Abstract
DNA methylation at the 5 position of cytosine (5-mC) has emerged as a key epigenetic marker that plays essential roles in various biological and pathological processes. 5-mC can be converted to 5-hydroxymethylcytosine (5-hmC) by the ten–eleven translocation (TET) family proteins, which is now widely recognized as the “sixth base” in the mammalian genome, following 5-mC, the “fifth base”. 5-hmC is detected to be abundant in brain and embryonic stem cells, and is also distributed in many different human tissues. Emerging evidence has shown that 5-hmC and TET family might serve unique biological roles in many biological processes such as gene control mechanisms, DNA methylation regulation, and involved in many diseases, especially cancers. In this paper we provide an overview of the role of 5-hmC as a new sight of epigenetics in human cancer.
Keywords: :
Introduction
Methylation of DNA at the 5-position of cytosines (5-methylcytosine [5-mC]) is an epigenetic mark that is across the spectrum of mammalian DNA. 5-mC has been extensively studied and found playing important roles as an epigenetic modification in various biological processes such as in gene regulation, genomic imprinting, X-chromosome inactivation, long-term silencing of transposons, and cancer development.Citation1-Citation3 In nearly half a century, 5-mC has been regarded as the “fifth base” in mammalian DNA to illustrate its importance and heritability. Recently, the researches for this methylation process moved to a new direction. In 2009, two reports in Science showed that mammalian genomic DNA contains not only 5-mC but also 5-hydroxymethylcytosine (5-hmC), which is now widely accepted as the “sixth base” in mammalian genome.Citation4,Citation5 Although 5-hmC was discovered in 1952, it is known for a long time as a component of special bacteriophages DNA.Citation6 However, 40 y ago, it was found in mammalian DNA.Citation7 5-hmC constitutes a small but significant fraction of cytosines in the DNA derived from Purkinje neurons and brain tissue. Moreover, the ten–eleven translocation (TET) family proteins can enzymatically convert 5-mC to 5-hmC. The discovery is a milestone for epigenetic research.
The Distribution and Function of 5-hmC in Mammalian Genomes
5-hmC, the hydroxylated form of 5-mC, was first reported in the DNA of T-even bacteriophage.Citation6 When it was first reported in mammalian DNA in 1972, this modified base accounted for 0–15% of the total cytosines in DNA extracted from the brains of adult rats, mice, and frogs.Citation7 However, this finding could not be reproduced until in 2009 Kriaucionis and Heintz found that 5-hmC accounts for 0.6% of the total nucleotides in Purkinje cells and 0.2% of the nucleotides in granule cells by using the thin-layer chromatography assay.Citation4 With the same method, Tahiliani et al. examined mouse embryonic stem (ES) cells and found that 5-hmC accounts for 0.03% of total nucleotides. Moreover, further research with genome-wide mapping of 5-hmC in mouse ES cells revealed that 5-hmC was relatively abundant within intragenic regions of genome at high and medium levels, as well as promoter regions of transcriptionally inactive genes. Furthermore, researchers used antibodies against 5-hmC and 5-mC together with high throughput sequencing to determine genome-wide patterns of methylation and hydroxymethylation in mouse wild-type and mutant ES cells and differentiating embryoid bodies which found that 5-hmC is mostly associated with euchromatin and enriched associated with increased transcriptional levels. Declining levels of TETs during differentiation are associated with decreased hydroxymethylation levels in the promoters of ES cell-specific genes together with increased methylation and gene silencing, which investigated a dynamic regulation of 5-hmC in mouse ES cells during differentiation.Citation8-Citation10 The study in human ES cells revealed that 5-hmC may be abundant in certain genomic regions such as enhancers associated with histone modifications and other protein–DNA interaction sites based both on the information of gene expression and sequence composition.Citation11
The distribution of 5-hmC has been investigated in several mouse tissues and found to be at high levels in central nervous system and the spinal cord (0.3–0.7%); medium levels in kidney, heart, lung, bladder, nasal epithelium, and skeletal muscle (0.15–0.17%); and low levels in liver, spleen, and the endocrine gland (0.03–0.06%).Citation12 Moreover, 5-hmC levels were increased from 0.1% of total nucleotides at postnatal day 7 to 0.4% of total nucleotides in adult mouse during the mouse cerebellum development.Citation13 In addition, the distribution of 5-hmC in various human tissues was also examined and shown a tiny difference from mouse tissues. In human, the brain tissue contains the highest level of 5-hmC (0.67%), and the rectum (0.57%), liver (0.46%), colon (0.45%), and kidney (0.38%) present relatively high abundance of 5-hmC. 5-hmC content was relatively low in lung (0.14%) and very low in placenta (0.06%) and breast (0.05%).Citation14 Unlike the content of 5-mC in human tissues (which is only 1- to 2.5-fold difference), significant difference of 5-hmC between different tissues (which is 13-fold greater difference) was observed. Further studies indicated that 5-hmC patterns are tissue-specific, unsupervised hierarchical clustering solely based on 5-hmC patterns groups from independent biological samples determined by tissue type. Using quantitative 5-hmC-qPCR, it was found that the absolute levels of 5-hmC for any given gene are primarily determined by tissue type. Gene expression is a secondary influence on 5-hmC levels. All these highlighted that tissue type is a major modifier of the 5-hydroxymethylcytosine content of human genes.Citation15
There are more evidences indicating that hydroxymethylation may be related with actively transcribed genes, although the exact biochemical mechanisms still remain enigmatic.Citation10,Citation16,Citation17 Recognized as the sixth base of DNA, 5-hmC was suggested to have some biological functions rather than just as an epigenetic mark. These functions may include alteration of chromatin structure or contribution to the recruitment or exclusion of DNA-binding proteins. It has been found that during the embryo cleavage, the maternal genome undergoes demethylation without DNA-methyltransferase 1 (DNMT1) during DNA replication.Citation18 In addition, 5-hmC was found to prevent DNMT1-medicated methylation of the target cytosine,Citation19 which was on the mammalian DNA glycosylase specific for 5-hmC.Citation20 Therefore, 5-hmC was proposed to lead an active demethylation.Citation21 Damage of 5-hmC at methyl-CpG sequence interfered several methyl-CpG-binding proteins to bind to these sequences, which may affect the transcriptional activity of genes with significant levels of 5-hmC in promoter regions.Citation22,Citation23 Later research in mouse ES cells found that 5-hmC was relatively enriched within intragenic regions as well as promoter regions.Citation8 It was also found located in enhancers and gene bodies, and tended to locate in other protein–DNA interaction sites such as transcription factor binding sites (TFBSs) in human ES cells.Citation11 All these evidences suggested a role of 5-hmC in gene regulation.
The Relationship between 5-mC and 5-hmC
5-mC, which is formed by post-replicative addition of a methyl group to cytosine through the action of DNA methyltransferase (DNMT), is one of the key epigenetic marks that has been implicated in a variety of biological process, especially in cell differentiation and gene regulation.Citation24-Citation26 Its close interrelation with 5-hmC has been widely studied. Early researches showed that 5-hmC may be associated with 5-mC sites in normal DNA in response to oxidative stress. With series of vitro studies about treatment of 5-mC under UV light or Fenton conditions (Fe2+/Cu2+ and H2O2) or with benzoylperoxide in CBrCl3 can yielded 5-hmC,Citation27,Citation28 which suggested that 5-hmC could be one of the major oxidative degradation products of 5-mC. However, its oxidative formation could not be confirmed under in vivo condition. 5-hmC can be further oxidized mainly to 5-formylcytosine (5-fC) and 5-carboxylcytosine (5-caC) ().
Figure 1. The relationship between 5-mc and 5-hmC. 5-mC is formed by post-replicative addition of methyl group to cytosine through the action of DNA methyltransferase (DNMT) which using S-adenosyl methionine (SAM) as methyl donor. Tet family proteins (TET1/2/3) catalyze 5-mC oxidation to 5-hmC in the presence of O2 and iron, 5-hmC can be further oxidized mainly to 5-fC and 5-caC. 5-hmC can be converted to 5-hmU via activation-induced deaminase (AID), which in turn can be converted to cytosine following base-excision repair (BER) pathway-mediated demethylation.
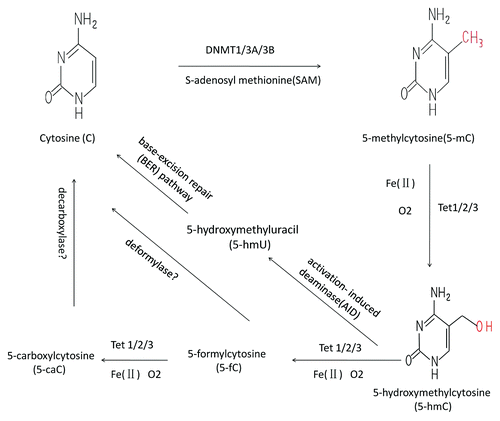
With outcomes that 5-hmC was not accompanied by the accumulation of other damage, the oxidative damage was suggested as the driving force.Citation4 Therefore Tahiliani et al. identified human ten–eleven translocation 1 (TET1) as a dioxygenase responsible for converting 5-mC to 5-hmC.Citation5 They found the levels of 5-hmC were reduced in TET1-lacking cells while the levels of 5-mC were increased. The levels of 5-hmC depended upon RNAi-mediated depletion of TET1 in ES cells. They also demonstrated that TET1 is able to catalyze the conversion of 5-mC to 5-hmC in both methylated and hemi-methylated DNA in cultured cells. Further studies showed that TET1 can control DNA methylation by not only binding to CpG rich regions to prevent unwanted DNA methyltransferase activity, but also converting 5-mC to 5-hmC through hydroxylase activity.Citation29 TET family has now become significant family of proteins in regulation of DNA methylation. The mammalian TET family contains three members: TET1, TET2, and TET3, and all of which share a high degree of homology with their C-terminal catalytic domain, where can be regulated by CFP1 (CxxC finger protein 1), MLL (mixed lineage leukemia protein), KDM (lysine demethylase) 2A, and KDM2B, which suggest that this family of enzymes take part in a potentially novel mechanism for regulation of DNA methylation.Citation30-Citation32 Recent studies showed that not only TET1 but all three TET proteins have the capacity to convert 5-mC to 5-hmC. However, TET1 could be more important in regulation of stem cell self-renewal and maintenance. Some studies found that without TET1 not TET2 or TET3, it will cause impairment of self-renewal and maintenance in mouse ES cells.Citation16 Meanwhile, other researches showed only a partial reduction in 5-hmC levels after the TET1 mutant, and may not affect pluripotency in ES cells.Citation29,Citation33,Citation34 TET3 type-3 ZF-CxxC domain can even recognize of non-methylated cytosine bases in either a CpG or a non-CpG context on the Xenopus.Citation32 Although it seems that the lack of TET2 or TET3 did not confer any obvious phenotype in ES cells, they may play important roles in other cell types.Citation35 Investigation found that TET family members were differentially expressed in mouse tissues except for TET2 that was most widely expressed.Citation36,Citation37 All these show that the TET family is the key molecules closely connecting 5-mC and 5-hmC, and has its potential function in many biological processes. The modulation mechanism of TET family is more and more popular. Myunggon Ko et al. showed that TET2 protein can be downregulated by domain protein IDAX, while TET3 can also be regulated by this.Citation38 Meanwhile, Yufei Xu et al. found that the TET3 CXXC domain is also critical for specific TET3 targeting.Citation39 N-acetylglucosamine (O-GlcNAc) transferase (OGT), an enzyme which catalyzes the addition of O-GlcNAc onto the serine and threonine residues, was found to be associated with TET proteins, especially TET2 and TET3.Citation40,Citation41 It associate with TET2 at transcription start sites and can regulate gene transcriptions together with TET2 by epigenetic modification on both DNA and histones.Citation41 TET and OGT tend to bind SET domain containing 1A (SETD1A), a methyl transferase component, to chromatin, which is necessary for methylation of histone H3 lysine 4 (H3K4me3) and subsequent transcriptional activation. In the same time, their activity is very important for the integrity of H3Ke methyl transferase, SET1/COMPASS complex.Citation40
5-hmC and Tet Family in Human Cancer
The traditional view is that tumor is due to gene mutation, deletion, and amplification caused by carcinogenic factors. These changes could lead to the disorder of cells growth and differentiation. However, in recent years, with further understanding of cancer, researchers found that regulatory mechanism of DNA sequences outside of abnormalities plays a more important role in the occurrence and the development of tumor such as DNA methylation, histone modification and chromatin structure, which are all found to alter in many human cancers.Citation42-Citation46 5-mC has been investigated to examine whether it has correlated with the initiation, progression, histological grade or poor prognosis of human cancers.Citation47-Citation49 However, the biological significance of 5-hmC in human cancer still remains elusive. The recent discovery of Tet proteins, which are capable of converting 5-mC to 5-hmC, may give a great breakthrough to this epigenetic research field.Citation5,Citation16,Citation33 Nevertheless, other co-pathways also take part in the hydroxylation to 5-hmC together with Tet proteins. Isocitrate dehydrogenase (IDH2) is significantly downregulated in melanomas.Citation50 And 2-hydroxyglutarate (2HG) produced by mutant IDH might inhibit the hydroxylation reaction of 5-methylcytosine by TET2.Citation51 Furthermore, the isocitrate dehydrogenase activity of IDH2 plays an important role in maintaining proper levels of 5-hmC in melanocytes (and may function as a putative tumor suppressor for melanoma progression).Citation50 Other co-factor like ascorbase also takes part in the regulation of 5-hmC generation.Citation52
Myeloid Malignancies
Nearly ten years ago, it was found that TET1 fused to the mixed lineage leukemia (MLL) gene in acute myeloid leukemia as a result of a chromosome translocation.Citation36,Citation53 But the exact role of TET1 in hematopoietic development and leukemogenesis is ambiguous at that time. Later studies identified that TET proteins as mammalian homologs of the trypanosome proteins Janus kinase-binding protein 1 (JBP1) and JBP2, were able to oxidize the 5-methyl of thymine. In addition, TET1 was shown to catalyze conversion of 5-mC to 5-hmC and be a fusion partner of MLL gene in acute myeloid leukemia as a 2-oxoglutarate (2OG)-and Fe (II)-dependent enzyme.Citation5
Another member of Tet family (TET2) is the most widely studied member in many kinds of myeloid malignancies. In 2009, sequencing of TET2 in some paired tumor/normal samples identified that gene mutations of TET2 occurred in myeloproliferative neoplasms (MPN), chronic myelomonocytic leukemia (CMML), and acute myeloid leukemia (AML).Citation54 Since then researches on TET2 and myeloid malignancies became more and more thorough. People showed that TET2 mutations were associated with myeloid malignancies compromising the TET2 catalytic activity. Patients with TET2 mutations displayed uniformly low level of 5-hmC in genomic DNA.Citation55 Recent investigations showed that TET2 played a negatively role in regulating the differentiation of mice hematopoietic stem cells (HSCs). If the conversion of 5-mC to 5-hmC was inhibited due to mutation of TET2 gene, it might disturb the differentiation of human hematopoietic progenitors.Citation35,Citation56 TET2 disruption affects 5-hmC levels in human myeloid cells and may participates in the pathogenesis of myeloid malignancies through the disturbance of myeloid differentiation, which may explain the reason why TET2 gene expression decreases during the progression of chronic myeloid leukemia (CML). Therefore TET2 mutation or loss of function is closely related to 5-mC and 5-hmC in patients with CMML and CML.Citation57-Citation60
Brain Neoplasms
Brain tumor is a kind of solid tumor that associated with 5-hmC, which has been currently studied. Researchers used immunohistochemistry (IHC) and isotope-based liquid chromatography mass spectrometry (LC-MS) to investigate the presence and distribution of 5-hmC in human brain during different periods of development as well as in a large series of human brain tumors, and found that cells express 5-hmC in brain tumors at a very low levels as compared with normal brain. During development, 5-hmC levels are high in more differentiated compartments like the fetal cortex, but low in the periventricular progenitor cell regions. In brain tumors, 5-hmC levels were relatively high in low-grade glioma and related to tumor differentiation and the WHO grade. However, it did not exhibit any correlation with isocitrate dehydrogenase 1 (IDH1) mutation status, which was thought to have a great relationship with many tumors.Citation61-Citation64 Moreover, a recent research found out that the number of 5-hmC positive cells was significantly correlated with IDH1 mutations in diffuse and anaplastic astrocytomas but not in glioblastomas.Citation64
Other Solid Tumors
Besides the brain tumor, 5-hmC levels are decreased in a large broad of other solid tumors compared with the normal tissues including cancerous colorectal tissues, liver, kidney, lung, skeletal muscle, prostate, breast cancer, and melanoma, and even decreased to an undetectable level in colon cancer cell lines. These findings suggest that the loss of 5-hmC is a common phenomenon in tumor tissues.Citation50,Citation65,Citation66 These indicated that 5-hmC may negatively regulate cancer formation and development. As abundant evidences showed that methylation mediated silencing of tumor suppression and genes apoptosis are closely related to cancer formation and progression, 5-hmC had been shown to prevent DNA methylation by blocking DNMT1 and involved the maintenance of gene expression by turnover of methylation.Citation16,Citation19 It might suggest a possibility that 5-hmC reduction in cancerous tissues damages the reactivation of these genes through 5-hmC-mediated methylation turnover, which would help the cancer cells to escape from tumor suppression and apoptosis caused by products of these genes, but the real mechanism is still unclear. It was also found that the highest 5-hmC level was observed in terminally differentiated cells, while less differentiated tissue stem/progenitor cell compartments had very low 5-hmC levels. All these findings showed a special relationship between 5-hmC and cancers, suggesting a distinct role for 5-hmC in tissue differentiation and epigenetic cancer therapyCitation67 ().
Table 1. Summarization of relationships between 5-hmC and cancer
Conclusion
DNA methylation at the 5-position of cytosines (5-mC) represents epigenetic functions in embryonic development and tumor biology. 5-hmC is generated from 5-mC by the Tet protein family. The 5-hmC modifications are known to be prevalent in DNA of embryonic stem cells and many other issues. Its function is involved in many human diseases especially cancers. However, the exploration of the relationship between 5-hmC and tumor is still in the initial stage. Since the level of 5-hmC in cancer is significantly reduced and changed in different types of tumor, 5-hmC may play a role in tumorigenesis and development process. The levels of 5-hmC may be downregulated by the mutations of TET2 and other relevant pathway as the tumors are getting less differentiated with the advance of malignancy. Nevertheless, there may be different ways and regulatory mechanisms in the process of different kinds of tumorigenesis. The exact role and molecular mechanism of 5-hmC are still remaining unclear. With the development of methods on methylation analysis and detection especially with the use of gene sequencing and microarray analysis, there will be more convenient and economical way for quantification of single gene locus so that horizontal comparison between these gene loci can be more widely studied. And the relationship between 5-hmC and tumor development will be further revealed. Signaling pathway will still be a popular point to the relationship between 5-hmC and tumor. Moreover, other new research areas such as cell circle may deserve more attention. The 5-hmC as a kind of new modified epigenetic mark is expected to become the marker of early diagnosis and prognosis of cancer.
Abbreviations: | ||
5-mC | = | 5-methylcytosine |
5-hmC | = | 5-hydroxymethylcytosine |
TET | = | ten–eleven translocation |
ES | = | embryonic stem |
DNMT1 | = | DNA-methyltransferase 1 |
MPN | = | myeloproliferative neoplasms |
CML | = | chronic myeloid leukemia |
CMML | = | chronic myelomonocytic leukemia |
AML | = | acute myeloid leukemia |
TFBSs | = | transcription factor binding sites |
MLL | = | mixed lineage leukemia |
HSC | = | hematopoietic stem cells |
IHC | = | immunohistochemistry |
LC-MS | = | liquid chromatography mass spectrometry |
IDH1 | = | isocitrate dehydrogenase 1 |
JBP | = | Janus kinase-binding protein |
Disclosure of Potential Conflicts of Interest
No potential conflicts of interest were disclosed.
References
- Lim DH, Maher ER. Genomic imprinting syndromes and cancer. Adv Genet 2010; 70:145 - 75; http://dx.doi.org/10.1016/B978-0-12-380866-0.60006-X; PMID: 20920748
- Jones PA, Baylin SB. The epigenomics of cancer. Cell 2007; 128:683 - 92; http://dx.doi.org/10.1016/j.cell.2007.01.029; PMID: 17320506
- Grønbaek K, Hother C, Jones PA. Epigenetic changes in cancer. APMIS 2007; 115:1039 - 59; http://dx.doi.org/10.1111/j.1600-0463.2007.apm_636.xml.x; PMID: 18042143
- Kriaucionis S, Heintz N. The nuclear DNA base 5-hydroxymethylcytosine is present in Purkinje neurons and the brain. Science 2009; 324:929 - 30; http://dx.doi.org/10.1126/science.1169786; PMID: 19372393
- Tahiliani M, Koh KP, Shen Y, Pastor WA, Bandukwala H, Brudno Y, Agarwal S, Iyer LM, Liu DR, Aravind L, et al. Conversion of 5-methylcytosine to 5-hydroxymethylcytosine in mammalian DNA by MLL partner TET1. Science 2009; 324:930 - 5; http://dx.doi.org/10.1126/science.1170116; PMID: 19372391
- Wyatt GR, Cohen SS. A new pyrimidine base from bacteriophage nucleic acids. Nature 1952; 170:1072 - 3; http://dx.doi.org/10.1038/1701072a0; PMID: 13013321
- Penn NW, Suwalski R, O’Riley C, Bojanowski K, Yura R. The presence of 5-hydroxymethylcytosine in animal deoxyribonucleic acid. Biochem J 1972; 126:781 - 90; PMID: 4538516
- Wu H, D’Alessio AC, Ito S, Wang Z, Cui K, Zhao K, Sun YE, Zhang Y. Genome-wide analysis of 5-hydroxymethylcytosine distribution reveals its dual function in transcriptional regulation in mouse embryonic stem cells. Genes Dev 2011; 25:679 - 84; http://dx.doi.org/10.1101/gad.2036011; PMID: 21460036
- Pastor WA, Pape UJ, Huang Y, Henderson HR, Lister R, Ko M, McLoughlin EM, Brudno Y, Mahapatra S, Kapranov P, et al. Genome-wide mapping of 5-hydroxymethylcytosine in embryonic stem cells. Nature 2011; 473:394 - 7; http://dx.doi.org/10.1038/nature10102; PMID: 21552279
- Ficz G, Branco MR, Seisenberger S, Santos F, Krueger F, Hore TA, Marques CJ, Andrews S, Reik W. Dynamic regulation of 5-hydroxymethylcytosine in mouse ES cells and during differentiation. Nature 2011; 473:398 - 402; http://dx.doi.org/10.1038/nature10008; PMID: 21460836
- Stroud H, Feng S, Morey Kinney S, Pradhan S, Jacobsen SE. 5-Hydroxymethylcytosine is associated with enhancers and gene bodies in human embryonic stem cells. Genome Biol 2011; 12:R54; http://dx.doi.org/10.1186/gb-2011-12-6-r54; PMID: 21689397
- Globisch D, Münzel M, Müller M, Michalakis S, Wagner M, Koch S, Brückl T, Biel M, Carell T. Tissue distribution of 5-hydroxymethylcytosine and search for active demethylation intermediates. PLoS One 2010; 5:e15367; http://dx.doi.org/10.1371/journal.pone.0015367; PMID: 21203455
- Song CX, Szulwach KE, Fu Y, Dai Q, Yi C, Li X, Li Y, Chen CH, Zhang W, Jian X, et al. Selective chemical labeling reveals the genome-wide distribution of 5-hydroxymethylcytosine. Nat Biotechnol 2011; 29:68 - 72; http://dx.doi.org/10.1038/nbt.1732; PMID: 21151123
- Li W, Liu M. Distribution of 5-hydroxymethylcytosine in different human tissues. J Nucleic Acids 2011; 2011:870726; http://dx.doi.org/10.4061/2011/870726; PMID: 21772996
- Nestor CE, Ottaviano R, Reddington J, Sproul D, Reinhardt D, Dunican D, Katz E, Dixon JM, Harrison DJ, Meehan RR. Tissue type is a major modifier of the 5-hydroxymethylcytosine content of human genes. Genome Res 2012; 22:467 - 77; http://dx.doi.org/10.1101/gr.126417.111; PMID: 22106369
- Ito S, D’Alessio AC, Taranova OV, Hong K, Sowers LC, Zhang Y. Role of Tet proteins in 5mC to 5hmC conversion, ES-cell self-renewal and inner cell mass specification. Nature 2010; 466:1129 - 33; http://dx.doi.org/10.1038/nature09303; PMID: 20639862
- Thalhammer A, Hansen AS, El-Sagheer AH, Brown T, Schofield CJ. Hydroxylation of methylated CpG dinucleotides reverses stabilisation of DNA duplexes by cytosine 5-methylation. Chem Commun (Camb) 2011; 47:5325 - 7; http://dx.doi.org/10.1039/c0cc05671e; PMID: 21451870
- Rougier N, Bourc’his D, Gomes DM, Niveleau A, Plachot M, Pàldi A, Viegas-Péquignot E. Chromosome methylation patterns during mammalian preimplantation development. Genes Dev 1998; 12:2108 - 13; http://dx.doi.org/10.1101/gad.12.14.2108; PMID: 9679055
- Valinluck V, Sowers LC. Endogenous cytosine damage products alter the site selectivity of human DNA maintenance methyltransferase DNMT1. Cancer Res 2007; 67:946 - 50; http://dx.doi.org/10.1158/0008-5472.CAN-06-3123; PMID: 17283125
- Cannon SV, Cummings A, Teebor GW. 5-Hydroxymethylcytosine DNA glycosylase activity in mammalian tissue. Biochem Biophys Res Commun 1988; 151:1173 - 9; http://dx.doi.org/10.1016/S0006-291X(88)80489-3; PMID: 3355548
- Zhang P, Su L, Wang Z, Zhang S, Guan J, Chen Y, Yin Y, Gao F, Tang B, Li Z. The involvement of 5-hydroxymethylcytosine in active DNA demethylation in mice. Biol Reprod 2012; 86:104; http://dx.doi.org/10.1095/biolreprod.111.096073; PMID: 22262693
- Jin SG, Kadam S, Pfeifer GP. Examination of the specificity of DNA methylation profiling techniques towards 5-methylcytosine and 5-hydroxymethylcytosine. Nucleic Acids Res 2010; 38:e125; http://dx.doi.org/10.1093/nar/gkq223; PMID: 20371518
- Valinluck V, Tsai HH, Rogstad DK, Burdzy A, Bird A, Sowers LC. Oxidative damage to methyl-CpG sequences inhibits the binding of the methyl-CpG binding domain (MBD) of methyl-CpG binding protein 2 (MeCP2). Nucleic Acids Res 2004; 32:4100 - 8; http://dx.doi.org/10.1093/nar/gkh739; PMID: 15302911
- Bird A. DNA methylation patterns and epigenetic memory. Genes Dev 2002; 16:6 - 21; http://dx.doi.org/10.1101/gad.947102; PMID: 11782440
- Cedar H, Bergman Y. Linking DNA methylation and histone modification: patterns and paradigms. Nat Rev Genet 2009; 10:295 - 304; http://dx.doi.org/10.1038/nrg2540; PMID: 19308066
- Suzuki MM, Bird A. DNA methylation landscapes: provocative insights from epigenomics. Nat Rev Genet 2008; 9:465 - 76; http://dx.doi.org/10.1038/nrg2341; PMID: 18463664
- Privat E, Sowers LC. Photochemical deamination and demethylation of 5-methylcytosine. Chem Res Toxicol 1996; 9:745 - 50; http://dx.doi.org/10.1021/tx950182o; PMID: 8831819
- Adams TB, Hallagan JB, Putnam JM, Gierke TL, Doull J, Munro IC, Newberne P, Portoghese PS, Smith RL, Wagner BM, et al. The FEMA GRAS assessment of alicyclic substances used as flavour ingredients. Food Chem Toxicol 1996; 34:763 - 828; http://dx.doi.org/10.1016/S0278-6915(96)00051-8; PMID: 8972877
- Xu Y, Wu F, Tan L, Kong L, Xiong L, Deng J, Barbera AJ, Zheng L, Zhang H, Huang S, et al. Genome-wide regulation of 5hmC, 5mC, and gene expression by Tet1 hydroxylase in mouse embryonic stem cells. Mol Cell 2011; 42:451 - 64; http://dx.doi.org/10.1016/j.molcel.2011.04.005; PMID: 21514197
- Iyer LM, Tahiliani M, Rao A, Aravind L. Prediction of novel families of enzymes involved in oxidative and other complex modifications of bases in nucleic acids. Cell Cycle 2009; 8:1698 - 710; http://dx.doi.org/10.4161/cc.8.11.8580; PMID: 19411852
- Loenarz C, Schofield CJ. Oxygenase catalyzed 5-methylcytosine hydroxylation. Chem Biol 2009; 16:580 - 3; http://dx.doi.org/10.1016/j.chembiol.2009.06.002; PMID: 19549596
- Long HK, Blackledge NP, Klose RJ. ZF-CxxC domain-containing proteins, CpG islands and the chromatin connection. Biochem Soc Trans 2013; 41:727 - 40; http://dx.doi.org/10.1042/BST20130028; PMID: 23697932
- Koh KP, Yabuuchi A, Rao S, Huang Y, Cunniff K, Nardone J, Laiho A, Tahiliani M, Sommer CA, Mostoslavsky G, et al. Tet1 and Tet2 regulate 5-hydroxymethylcytosine production and cell lineage specification in mouse embryonic stem cells. Cell Stem Cell 2011; 8:200 - 13; http://dx.doi.org/10.1016/j.stem.2011.01.008; PMID: 21295276
- Dawlaty MM, Ganz K, Powell BE, Hu YC, Markoulaki S, Cheng AW, Gao Q, Kim J, Choi SW, Page DC, et al. Tet1 is dispensable for maintaining pluripotency and its loss is compatible with embryonic and postnatal development. Cell Stem Cell 2011; 9:166 - 75; http://dx.doi.org/10.1016/j.stem.2011.07.010; PMID: 21816367
- Ko M, Bandukwala HS, An J, Lamperti ED, Thompson EC, Hastie R, Tsangaratou A, Rajewsky K, Koralov SB, Rao A. Ten-Eleven-Translocation 2 (TET2) negatively regulates homeostasis and differentiation of hematopoietic stem cells in mice. Proc Natl Acad Sci U S A 2011; 108:14566 - 71; http://dx.doi.org/10.1073/pnas.1112317108; PMID: 21873190
- Lorsbach RB, Moore J, Mathew S, Raimondi SC, Mukatira ST, Downing JR. TET1, a member of a novel protein family, is fused to MLL in acute myeloid leukemia containing the t(10;11)(q22;q23). Leukemia 2003; 17:637 - 41; http://dx.doi.org/10.1038/sj.leu.2402834; PMID: 12646957
- Langemeijer SM, Aslanyan MG, Jansen JH. TET proteins in malignant hematopoiesis. Cell Cycle 2009; 8:4044 - 8; http://dx.doi.org/10.4161/cc.8.24.10239; PMID: 19923888
- Ko M, An J, Bandukwala HS, Chavez L, Aijö T, Pastor WA, Segal MF, Li H, Koh KP, Lähdesmäki H, et al. Modulation of TET2 expression and 5-methylcytosine oxidation by the CXXC domain protein IDAX. Nature 2013; 497:122 - 6; http://dx.doi.org/10.1038/nature12052; PMID: 23563267
- Xu Y, Xu C, Kato A, Tempel W, Abreu JG, Bian C, Hu Y, Hu D, Zhao B, Cerovina T, et al. Tet3 CXXC domain and dioxygenase activity cooperatively regulate key genes for Xenopus eye and neural development. Cell 2012; 151:1200 - 13; http://dx.doi.org/10.1016/j.cell.2012.11.014; PMID: 23217707
- Deplus R, Delatte B, Schwinn MK, Defrance M, Méndez J, Murphy N, Dawson MA, Volkmar M, Putmans P, Calonne E, et al. TET2 and TET3 regulate GlcNAcylation and H3K4 methylation through OGT and SET1/COMPASS. EMBO J 2013; 32:645 - 55; http://dx.doi.org/10.1038/emboj.2012.357; PMID: 23353889
- Chen Q, Chen Y, Bian C, Fujiki R, Yu X. TET2 promotes histone O-GlcNAcylation during gene transcription. Nature 2013; 493:561 - 4; http://dx.doi.org/10.1038/nature11742; PMID: 23222540
- Feinberg AP, Vogelstein B. Hypomethylation distinguishes genes of some human cancers from their normal counterparts. Nature 1983; 301:89 - 92; http://dx.doi.org/10.1038/301089a0; PMID: 6185846
- Gama-Sosa MA, Slagel VA, Trewyn RW, Oxenhandler R, Kuo KC, Gehrke CW, Ehrlich M. The 5-methylcytosine content of DNA from human tumors. Nucleic Acids Res 1983; 11:6883 - 94; http://dx.doi.org/10.1093/nar/11.19.6883; PMID: 6314264
- Jones PA, Baylin SB. The fundamental role of epigenetic events in cancer. Nat Rev Genet 2002; 3:415 - 28; PMID: 12042769
- Herman JG, Baylin SB. Gene silencing in cancer in association with promoter hypermethylation. N Engl J Med 2003; 349:2042 - 54; http://dx.doi.org/10.1056/NEJMra023075; PMID: 14627790
- Ushijima T. Detection and interpretation of altered methylation patterns in cancer cells. Nat Rev Cancer 2005; 5:223 - 31; http://dx.doi.org/10.1038/nrc1571; PMID: 15719030
- Sun L, Hui AM, Kanai Y, Sakamoto M, Hirohashi S. Increased DNA methyltransferase expression is associated with an early stage of human hepatocarcinogenesis. Jpn J Cancer Res 1997; 88:1165 - 70; http://dx.doi.org/10.1111/j.1349-7006.1997.tb00345.x; PMID: 9473734
- Peng DF, Kanai Y, Sawada M, Ushijima S, Hiraoka N, Kosuge T, Hirohashi S. Increased DNA methyltransferase 1 (DNMT1) protein expression in precancerous conditions and ductal carcinomas of the pancreas. Cancer Sci 2005; 96:403 - 8; http://dx.doi.org/10.1111/j.1349-7006.2005.00071.x; PMID: 16053511
- Nakagawa T, Kanai Y, Saito Y, Kitamura T, Kakizoe T, Hirohashi S. Increased DNA methyltransferase 1 protein expression in human transitional cell carcinoma of the bladder. J Urol 2003; 170:2463 - 6; http://dx.doi.org/10.1097/01.ju.0000095919.50869.c9; PMID: 14634451
- Lian CG, Xu Y, Ceol C, Wu F, Larson A, Dresser K, Xu W, Tan L, Hu Y, Zhan Q, et al. Loss of 5-hydroxymethylcytosine is an epigenetic hallmark of melanoma. Cell 2012; 150:1135 - 46; http://dx.doi.org/10.1016/j.cell.2012.07.033; PMID: 22980977
- Figueroa ME, Abdel-Wahab O, Lu C, Ward PS, Patel J, Shih A, Li Y, Bhagwat N, Vasanthakumar A, Fernandez HF, et al. Leukemic IDH1 and IDH2 mutations result in a hypermethylation phenotype, disrupt TET2 function, and impair hematopoietic differentiation. Cancer Cell 2010; 18:553 - 67; http://dx.doi.org/10.1016/j.ccr.2010.11.015; PMID: 21130701
- Minor EA, Court BL, Young JI, Wang G. Ascorbate induces ten-eleven translocation (Tet) methylcytosine dioxygenase-mediated generation of 5-hydroxymethylcytosine. J Biol Chem 2013; 288:13669 - 74; http://dx.doi.org/10.1074/jbc.C113.464800; PMID: 23548903
- Ono R, Taki T, Taketani T, Taniwaki M, Kobayashi H, Hayashi Y. LCX, leukemia-associated protein with a CXXC domain, is fused to MLL in acute myeloid leukemia with trilineage dysplasia having t(10;11)(q22;q23). Cancer Res 2002; 62:4075 - 80; PMID: 12124344
- Abdel-Wahab O, Mullally A, Hedvat C, Garcia-Manero G, Patel J, Wadleigh M, Malinge S, Yao J, Kilpivaara O, Bhat R, et al. Genetic characterization of TET1, TET2, and TET3 alterations in myeloid malignancies. Blood 2009; 114:144 - 7; http://dx.doi.org/10.1182/blood-2009-03-210039; PMID: 19420352
- Ko M, Huang Y, Jankowska AM, Pape UJ, Tahiliani M, Bandukwala HS, An J, Lamperti ED, Koh KP, Ganetzky R, et al. Impaired hydroxylation of 5-methylcytosine in myeloid cancers with mutant TET2. Nature 2010; 468:839 - 43; http://dx.doi.org/10.1038/nature09586; PMID: 21057493
- Pronier E, Almire C, Mokrani H, Vasanthakumar A, Simon A, da Costa Reis Monte Mor B, Massé A, Le Couédic JP, Pendino F, Carbonne B, et al. Inhibition of TET2-mediated conversion of 5-methylcytosine to 5-hydroxymethylcytosine disturbs erythroid and granulomonocytic differentiation of human hematopoietic progenitors. Blood 2011; 118:2551 - 5; http://dx.doi.org/10.1182/blood-2010-12-324707; PMID: 21734233
- Albano F, Anelli L, Zagaria A, Coccaro N, Minervini A, Rossi AR, Specchia G. Decreased TET2 gene expression during chronic myeloid leukemia progression. Leuk Res 2011; 35:e220 - 2; http://dx.doi.org/10.1016/j.leukres.2011.07.013; PMID: 21794915
- Pérez C, Martínez-Calle N, Martín-Subero JI, Segura V, Delabesse E, Fernandez-Mercado M, Garate L, Alvarez S, Rifon J, Varea S, et al. TET2 mutations are associated with specific 5-methylcytosine and 5-hydroxymethylcytosine profiles in patients with chronic myelomonocytic leukemia. PLoS One 2012; 7:e31605; http://dx.doi.org/10.1371/journal.pone.0031605; PMID: 22328940
- Mancini M, Veljkovic N, Leo E, Aluigi M, Borsi E, Galloni C, Iacobucci I, Barbieri E, Santucci MA. Cytoplasmatic compartmentalization by Bcr-Abl promotes TET2 loss-of-function in chronic myeloid leukemia. J Cell Biochem 2012; 113:2765 - 74; http://dx.doi.org/10.1002/jcb.24154; PMID: 22467095
- Li Z, Cai X, Cai CL, Wang J, Zhang W, Petersen BE, Yang FC, Xu M. Deletion of Tet2 in mice leads to dysregulated hematopoietic stem cells and subsequent development of myeloid malignancies. Blood 2011; 118:4509 - 18; http://dx.doi.org/10.1182/blood-2010-12-325241; PMID: 21803851
- Orr BA, Haffner MC, Nelson WG, Yegnasubramanian S, Eberhart CG. Decreased 5-hydroxymethylcytosine is associated with neural progenitor phenotype in normal brain and shorter survival in malignant glioma. PLoS One 2012; 7:e41036; http://dx.doi.org/10.1371/journal.pone.0041036; PMID: 22829908
- Jin SG, Jiang Y, Qiu R, Rauch TA, Wang Y, Schackert G, Krex D, Lu Q, Pfeifer GP. 5-Hydroxymethylcytosine is strongly depleted in human cancers but its levels do not correlate with IDH1 mutations. Cancer Res 2011; 71:7360 - 5; http://dx.doi.org/10.1158/0008-5472.CAN-11-2023; PMID: 22052461
- Shih AH, Levine RL. IDH1 mutations disrupt blood, brain, and barriers. Cancer Cell 2012; 22:285 - 7; http://dx.doi.org/10.1016/j.ccr.2012.08.022; PMID: 22975371
- Kraus TF, Globisch D, Wagner M, Eigenbrod S, Widmann D, Münzel M, Müller M, Pfaffeneder T, Hackner B, Feiden W, et al. Low values of 5-hydroxymethylcytosine (5hmC), the “sixth base,” are associated with anaplasia in human brain tumors. Int J Cancer 2012; 131:1577 - 90; http://dx.doi.org/10.1002/ijc.27429; PMID: 22234893
- Kudo Y, Tateishi K, Yamamoto K, Yamamoto S, Asaoka Y, Ijichi H, Nagae G, Yoshida H, Aburatani H, Koike K. Loss of 5-hydroxymethylcytosine is accompanied with malignant cellular transformation. Cancer Sci 2012; 103:670 - 6; http://dx.doi.org/10.1111/j.1349-7006.2012.02213.x; PMID: 22320381
- Haffner MC, Chaux A, Meeker AK, Esopi DM, Gerber J, Pellakuru LG, Toubaji A, Argani P, Iacobuzio-Donahue C, Nelson WG, et al. Global 5-hydroxymethylcytosine content is significantly reduced in tissue stem/progenitor cell compartments and in human cancers. Oncotarget 2011; 2:627 - 37; PMID: 21896958
- Tan L, Shi YG. Tet family proteins and 5-hydroxymethylcytosine in development and disease. Development 2012; 139:1895 - 902; http://dx.doi.org/10.1242/dev.070771; PMID: 22569552