Abstract
Treatment of recurrent and metastatic cervical cancer remains a challenge, especially in developing countries, which lack efficient screening programs. In recent years, artemisinin and its derivatives, such as dihydroartemisinin (DHA), which were traditionally used as anti-malarial agent, have been shown to inhibit tumor growth with low toxicity to normal cells. In this study, we investigated mechanisms underlying the anti-tumor effect of DHA in cervical cancer. We evaluated the role of DHA on the expression of bcl-2 and Raf kinase inhibitor protein (RKIP), which is a suppressor of metastasis. The MTT assay was used to compare the proliferation of untreated and DHA-treated Hela and Caski cervical cancer cells. Flow cytometry was used to determine the percentage of cells at each stage of the cell cycle in untreated and DHA-treated cells. We used RT-PCR and western blots to determine the expression of bcl-2 and RKIP mRNA and proteins. We evaluated the effect of DHA treatment in nude mice bearing Hela or Caski tumors. DHA-treated cells showed a time- and dose-dependent inhibition of proliferation and a significant increase in apoptosis. The expression of RKIP was significantly upregulated and the expression of bcl-2 was significantly downregulated in DHA-treated cells compared with control cells. DHA treatment caused (1) a significant inhibition of tumor growth and (2) a significant increase in the apoptotic index in nude mice bearing Hela or Caski tumors. Our data suggest that DHA inhibits cervical cancer growth via upregulation of RKIP and downregulation of bcl-2.
Introduction
Cervical cancer is the third most commonly diagnosed cancer worldwide and accounts for 9% of all new cancer cases.Citation1 Efficient screening programs involving the use of Papanicolaou (Pap) smears and detection of HPV DNA have resulted in a significant reduction in cervical cancer mortality in developed countries.Citation2,Citation3 The high incidence and mortality rates seen in developing countries are due to a lack of such extensive screening programs that allow detection of precancerous lesions and early stage disease.Citation4
Alteration in the expression of genes such as EGFR, MYC, ERBB2, CCND1, and HRAS has been shown to contribute to the etiology of cervical cancer.Citation5 However, since persistent infection with high-risk types of the human papilloma virus (HPV) is a major etiological factor, HPV-based screening tests are extensively used for diagnostic purposes and targeting HPV-infected cells has become an important therapeutic strategy.Citation6 A bivalent vaccine protecting against HPV 16 and HPV 18 and a quadrivalent vaccine protecting against HPV 6, 11, 16, and 18 have shown efficacy in clinical trials.Citation7
Early stage cervical cancer is mainly treated surgically or with chemo-radiation in cases when surgery in contraindicated.Citation7 Strategies for treatment of advanced disease have limited value and are mostly palliative, since only one-third of women with metastatic disease respond to chemotherapy and this response is short-lived. It is therefore imperative to develop novel therapeutic strategies to address the challenges of clinical management of advanced cervical cancer in developing countries.
There has been a recent focus on the use of Chinese medicinal herbs to treat a number of diseases. Artemisinin, the active constituent of Artemisia annua L., along with its derivatives has been used effectively as an anti-malarial agent.Citation8-Citation10 One of the main active metabolites of arteminisin is dihydroartemisin (DHA) which has been reported to inhibit the proliferation of a number of cancer cells in vitro and also inhibited viral-induced tumor formation in mice.Citation9,Citation11-Citation13
A number of studies recently showed that the anti-tumor effect of DHA in different cell lines was associated with DHA-mediated apoptosis and modulation of the cell cycle.Citation14-Citation16 DHA in combination with doxorubicin had a synergistic anti-proliferative effect on breast cancer cells,Citation14 potentiated the effect of gemcitabine in pancreatic cancer cells,Citation17 and inhibited angiogenesis in human umbilical vein endothelial cells (HUVEC).Citation11 However, there is limited information on role of DHA in cervical cancer. DHA was shown to induce apoptosis via a p53-independent, caspase 9-mediated pathway in HPV-expressing cervical cancer cells.Citation12 DHA was also shown to abrogate the G2 checkpoint and induce radiosensitivity in Hela cells.Citation16
Raf kinase inhibitor protein (RKIP), a suppressor of metastasis, is downregulated in highly metastatic breast,Citation18 prostate,Citation19 and ovarian cancers.Citation20 It has recently been suggested that in addition to HPV screening, RKIP can be used as a marker to identify HPV-infected samples with abnormal cytology.Citation21 RKIP is thought to inhibit the RAF-MEK-ERK pathway to modulate a number of cellular processes including proliferation, apoptosis, and cell cycle progression.Citation22 We and others previously showed that RKIP was significantly downregulated in metastatic cervical cancer and this downregulation was associated with an aggressive phenotype and chemotherapy resistance.Citation23,Citation24
In this study, we investigated the effect of DHA on cervical cancer in vitro and in vivo. We also dissected the mechanisms underlying the anti-tumor effect of DHA in cervical cancer cells by evaluating the role of DHA in proliferation and apoptosis.
Results
DHA inhibited proliferation of Hela cells and Caski cells
We used MTT assay to show that the IC50 of DHA was 22.08 μmol/L for Hela cells and 18.20 μmol/L for Caski cells treated with DHA for 48 h (). We showed a dose-dependent inhibition of proliferation (from 1 µmol/L to 60 µmol/L DHA concentrations) in Hela and Caski cells (all P values < 0.001). We treated Hela and Caski cells with 20 μmol/L DHA for 12, 24, 36, 48, and 60 h. We showed that DHA exerted a time-dependent inhibition of proliferation in both cell lines () (all P < 0.001).
Figure 1. DHA inhibits proliferation of Hela and Caski cells in a dose- and time-dependent manner. (A) Hela cells were treated with different doses of DHA for 48 h. (B) Caski cells were treated with different doses of DHA for 48 h. (C) Hela cells were treated with 20 μmol/L of DHA for varying amounts of time (D) Caski cells were treated with 20 μmol/L of DHA for varying amounts of time.
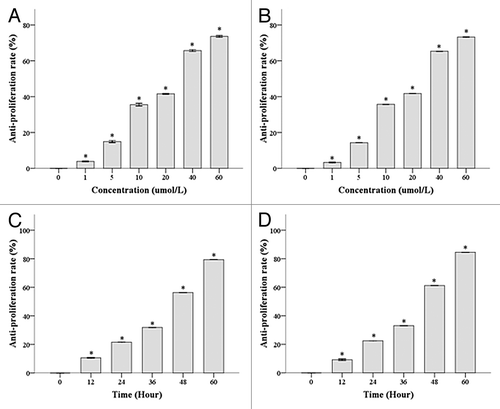
DHA treatment induced apoptosis in Hela and Caski cells
We used fluorescent microscopy to evaluate apoptosis in AO/EB stained cells. Normal, viable cells had green nuclear chromatin and a normal morphology. Early apoptotic cells (VA) had green nuclear chromatin, which appeared bead-like, while late apoptotic cells (NVA) had orange nuclear chromatin, which appeared bead-like. Necrotic cells (NVN) had orange nuclear chromatins and an abnormal morphology (). DHA-treated Hela and Caski cells had a significantly higher apoptotic index (AI) compared with untreated cells (80.30 ± 6.01% and 83.32 ± 3.28% vs. 11.39 ± 2.32% and 12.17 ± 2.89%, respectively; both P < 0.001) ().
Figure 2. DHA-treated Hela and Caski cell line were subjected to AO/EB fluorescent staining. (A) Morphology of Hela and Caski cell treated with 20 μM treatment of DHA for 48 h after AO/EB fluorescent staining. (1) Hela Control; (2) Hela DHA; (3) Caski Control; (4) Caski DHA. Up arrow, NV; star, VA; circle, NVA; triangle, NVN. (B) Bar representation of AO/EB fluorescent staining. *P < 0.05 indicates a significant difference between control and DHA groups for each cell line.
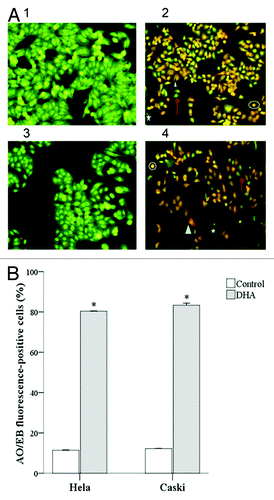
DHA treatment altered the cell cycle profile of Hela and Caski cells
We used flow cytometry to compare the cell cycle profiles of DHA-treated Hela and Caski cells with untreated cells (). We showed that a significantly higher percentage of DHA-treated Hela cells were in the G0/G1 phase compared with untreated cells (64.47 ± 5.42 vs. 37.23 ± 2.36). In contrast, a significantly lower percentage of DHA-treated Hela cells were in the S phase compared with the untreated group (21.06 ± 2.33 vs. 57.54 ± 4.83; both P < 0.001). Similarly, a higher percentage of DHA-treated Caski cells were in the G0/G1 and G2/M phases compared with untreated cells (66.19 ± 5.65 and 4.38 ± 3.98 vs. 40.24 ± 4.12 and 3.82 ± 2.92, respectively), and a significantly lower percentage were in the S phase compared with untreated cells (15.26 ± 2.06 vs. 55.63 ± 4.96; all P < 0.001).
Figure 3. DHA effects on cell cycle profiles of untreated and DHA-treated Hela and Caski cells. *P < 0.05 indicates a significant difference between control and DHA groups for each cell line. †P < 0.05 indicates a significant difference between Hela and Caski control groups. ‡P < 0.05 indicates a significant difference between Hela and Caski DHA groups.
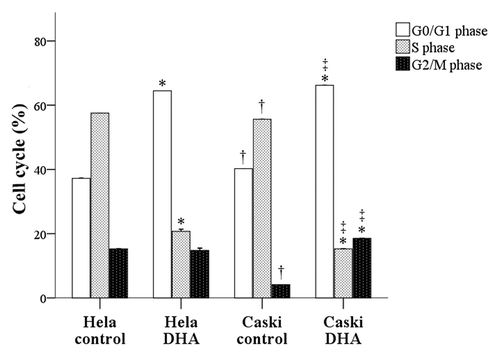
A comparison of the two cell lines showed that a significantly higher percentage of untreated Caski cells were in the G0/G1 phase compared with untreated Hela cells (40.24 ± 4.12 vs. 37.23 ± 2.36; P < 0.001). In contrast, a significantly lower percentage of untreated Caski cells were in the S (55.63 ± 4.96 vs. 57.54 ± 4.83) and G2/M phases (3.82 ± 2.92 vs. 5.14 ± 1.38) compared with untreated Hela cells (P < 0.001). Compared with DHA-treated Hela cells, a significantly higher percentage of DHA-treated Caski cells were in the G0/G1 phase (66.19 ± 5.65 vs. 64.47 ± 5.42) and the G2/M phase (4.38 ± 3.98 vs. 3.08 ± 1.15; P < 0.001), and a lower percentage were in the S phase (15.26 ± 2.06 vs. 21.06 ± 2.33; P < 0.001) ().
DHA treatment upregulated RKIP and downregulated bcl-2 expression in Hela cells and Caski cells
We used RT-PCR to determine the expression of RKIP mRNA () and showed a significantly higher expression of RKIP mRNA in DHA-treated Caski as well as Hela cells compared with untreated cells (both P < 0.001) (). However, the expression of bcl-2 mRNA was significantly lower in DHA-treated Caski and Hela cells compared with untreated cells (both P < 0.001) ().
Figure 4. mRNA expression of RKIP and Bcl-2 in untreated and DHA-treated Hela and Caski cells. (A) Representative electrophoresis images; (B) mRNA expression of RKIP normalized to that of GAPDH; (C) mRNA expression of bcl-2 normalized to that of GAPDH. *P < 0.05 indicates a significant difference between control and DHA groups for each cell line.
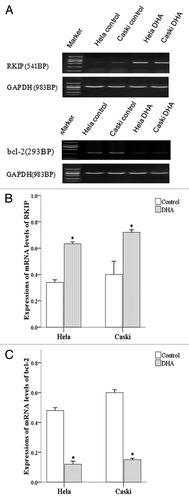
We also used western blots to show that the protein expression of RKIP was significantly upregulated in DHA-treated Hela and Caski cells () (both P < 0.001). However, the protein expression of bcl-2 was significantly downregulated in DHA-treated Hela and Caski cells () (P < 0.001).
Figure 5. Protein expression of RKIP and Bcl-2 in untreated and DHA-treated Hela and Caski cells. (A) Representative images of western blots protein gels; (B) Protein expression of RKIP normalized to that of GAPDH; (C) Protein expression of bcl-2 normalized to that of GAPDH. *P < 0.05 indicates a significant difference between control and DHA groups for each cell line.
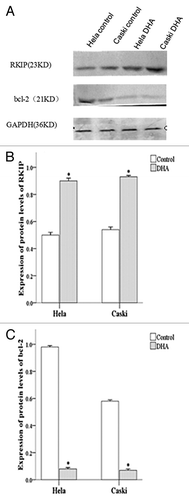
DHA treatment inhibited tumor size in nude mice
Subcutaneous tumors were observed in all nude mice (n = 20) inoculated with Hela or Caski cells (tumorigenicity rate of 100%). Tumor-bearing mice treated with DHA exhibited a significant reduction in tumor volume compared with untreated mice. Tumor volumes were 999.40 ± 0.6 mm3 and 190.98 ± 4.2 mm3 in untreated and DHA-treated mice bearing Hela tumors. Tumor volumes were 1094.2 ± 3.0 mm3 and 184.0 ± 4.8 mm3 in untreated and DHA-treated mice bearing Caski tumors ().
Table 1. Tumor volumes in the Hela and Caski cell line
DHA treatment did not affect morphology of important organs
Untreated cancer cells and their nuclei were uneven in morphology and size and staining intensity. With increase in tumor volume, we found focal necrosis at the center of the tumors. Tissue from DHA-treated mice in both Hela and Caski groups exhibited massive necrosis at the peripheral area of the tumors (). We used hematoxylin and eosin stain (HE) staining to evaluate the morphology of the heart, liver, and kidney. The heart appeared normal. The glomeruli and renal tubules of the kidney appeared normal and showed no vascular expansion or congestion. No necrotic cells were observed. The hepatocytes and hepatic lobules of the liver appeared normal. We used Wright–Giemsa staining to show that the bone marrow morphology was normal. Red blood cells showed active proliferation, and found no myelosuppression ().
Figure 6. HE staining of tumors in vivo. (A) HE staining of tissue from untreated Hela tumors (100×); (B) HE staining of tissue from untreated Hela tumors (400×); (C) HE staining of tissue from DHA-treated Hela tumors (100×); (D) HE staining of tissue from DHA-treated Hela tumors (400×); (E) HE staining of tissue from untreated Caski tumors (100×); (F) HE staining of tissue from untreated Caski tumors (400×); (G) HE staining of tissue from DHA-treated tumors (100×); (H) HE staining of tissue from DHA-treated Caski tumors (400×). Arrows show focal hemorrhage and necrosis at the center of the tumor in control groups.
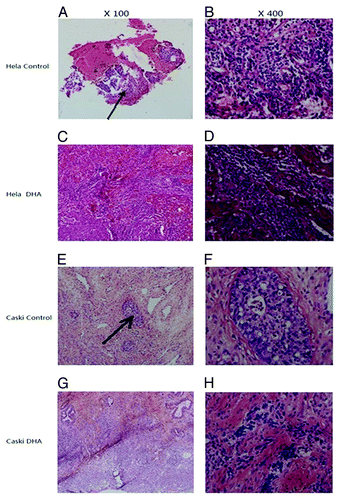
Increased apoptotic index (AI) in DHA-treated mice
We used TUNEL staining to evaluate the apoptotic index in tumor tissue from untreated and DHA-treated mice (). We showed that tumor tissue from DHA-treated mice had a significantly higher AI value compared with control mice in both the Hela and Caski groups (both P < 0.001).
Figure 8. Effect of DHA on cell death in Hela and Caski cell lines. (A) TUNEL-staining of untreated and DHA-treated Hela and Caski cells. (B) Apoptotic index of untreated and DHA-treated Hela and Caski cells. *P < 0.05 indicates a significant difference between control and DHA groups for each cell line.
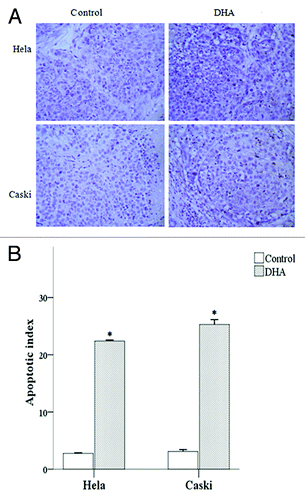
Discussion
In this study, we demonstrated that DHA efficiently inhibited the proliferation of cervical cancer cells in a dose-dependent and time-dependent manner. DHA treatment induced apoptosis and was associated with a significant upregulation of RKIP mRNA and protein and a significant downregulation of bcl-2 mRNA and protein. When mice bearing Hela or Caski tumors were treated with DHA, there was a significant inhibition of tumor growth compared with untreated mice and this was associated with a significant increase in the apoptotic index.
The morbidity and mortality of cervical cancer has decreased in developed countries due to efficient screening programs and better prognosis for early stage cervical cancer.Citation2,Citation25 However, treatment of recurrent and metastatic disease remains a challenge, especially in developing countries where screening programs are not extensively used.Citation25 In recent years, artemisinin and its derivatives have been shown to inhibit tumor growth with low toxicity to normal cells.Citation8
The anti-tumor effect of artemisinin and its derivatives has been attributed to ROS and carbon-centered radicals and their cytotoxic efficiency was enhanced in the presence of iron (II) salts alone or with transferrin.Citation26 However, these compounds have also been shown to work via ROS-independent mechanisms such as activation of the p38MAPK pathway.Citation27 Although artemisinin and its derivatives are known to induce apoptosis via the intrinsic or cytochrome C-mediated pathway, the exact mechanisms are not completely understood.Citation28 In this study, we showed that DHA inhibited the proliferation of two cervical cancer cells in a time- and dose-dependent manner. Interestingly, DHA treatment altered the cell cycle profile of Hela and Caski cells. DHA treatment of Hela cells resulted in a significant decrease in the percentage of S phase cells and a significant increase in the percentage of cells G0/G1 phase cells. Similarly, DHA treatment of Caski cells resulted in a significant decrease in the percentage of S phase cells and a significant increase in the percentage of G0/G1 and G2/M phase cells. Our data agreed with previous studies which showed a dose-dependent inhibition of proliferation in DHA-treated ovarian cancer and pancreatic cancer cells.Citation13,Citation17
RKIP, an inhibitor of the RAF/MEK/ERK pathway, has been reported to play an important role in modulating a number of signaling pathways which regulate proliferation, motility, and epithelial–mesenchymal transition (EMT).Citation29-Citation31 Downregulation of RKIP was shown to be associated with increased viability and proliferation, increased migration, and increased vascularization.Citation24 We previously reported that downregulation of RKIP was associated with metastasis of cervical cancer and speculated that the tumor suppressor function of RKIP could potentially be mediated via inhibition of tumor cell migration.Citation23 RKIP was also shown to inhibit NF-κB signaling by binding the upstream kinases TAK1, NIK, and IKK.Citation32 Interestingly, RKIP-mediated radiosensitization of tumor cells is thought to be the result of inhibition of NFκB signaling, which leads to alterations in the expression of anti-apoptotic and pro-apoptotic genes.Citation33
A number of cancers are characterized by altered expression of the anti-apoptotic Bcl-2 gene.Citation34-Citation37 Overexpression of bcl-2 increased the resistance of ALL cells to cytotoxic drugs, while knockdown of bcl-2 expression sensitized them to the cytotoxic drugs.Citation38 In this study, we investigated the effect of DHA treatment on RKIP and bcl-2 because (1) the anti-tumor effect of DHA has been reported to be associated with regulation of apoptosis and cell cycle signaling, (2) RKIP has been shown to inhibit the RAF-MEK-ERK pathway to regulate apoptosis and cell proliferation, and (3) Bcl-2 is an important anti-apoptotic gene whose expression is altered in a large number of cancers. We evaluated the effect of DHA on the mRNA and protein expression of RKIP and bcl-2. We showed a significant upregulation in the levels of RKIP mRNA and protein in DHA-treated Hela and Caski cells respectively. Our data suggested that DHA could function by upregulating the metastasis suppressor, RKIP, in order to inhibit metastasis and progression of cervical cancer. We also showed a significant downregulation of bcl-2 mRNA and protein levels in DHA-treated Hela and Caski cells. Our data suggested that the anti-tumor effect of DHA in cervical cancer cells could be due to bcl-2-dependent induction of apoptosis.
We showed that DHA safely and efficiently inhibited tumor growth in vivo. Body weight has been used as an indicator of effectiveness and safety of anti-tumor drugs. Our data showed that the body weights of untreated and DHA-treated mice were comparable. We found no ischemia, cell swelling or necrosis in the heart, liver, kidney, and bone marrow, and the bone marrow cells had no nuclei suggesting the absence of myelosuppression. The absence of toxicity or damage to the major organs strongly suggested that DHA treatment was safe.
Changes in tumor volume and inhibition of tumor growth reflect the anti-tumor effect of DHA. DHA-treated mice showed an initial increase in tumor growth for 3 weeks after inoculation, followed by growth inhibition. The inhibition rate remained at 70–80% until the end of study, suggesting that five-week duration of DHA treatment may be necessary to exert a stable anti-tumor effect. We used TUNEL staining to show that DHA-treated cervical cancer cells had a significantly higher apoptotic index compared with untreated cells. Based on our in vitro data, we speculate that the increased apoptotic index in DHA-treated mice could be associated with upregulation in RKIP and downregulation in bcl-2 levels. One limitation of this study is that we did not explore if DHA-mediated upregulation of RKIP and downregulation of Bcl-2 was dose-dependent. We plan to address this in future studies. Since the anti-tumor activity of DHA could be mediated via ROS-independent mechanisms such as activation of the p38MAPK pathway,Citation27 and RKIP was recently shown to activate glycogen synthase kinase 3β (GSK3β) via a ROS-activated p38 MAPK pathway,Citation39 we consider it important to dissect a number of such signaling pathways in more detail in untreated and DHA-treated tumor-bearing mice. We also plan to validate our results by evaluating the effects of DHA treatment in cervical cancer cells where RKIP expression is silenced.
In summary, we showed that DHA inhibited the proliferation of Hela and Caski cervical cancer cells in a dose-dependent and time-dependent manner. Apoptosis was significantly upregulated in DHA-treated cells and this upregulation of apoptosis was associated with an increase in the levels of RKIP and a decrease in the levels of bcl-2. DHA inhibited tumor growth in nude mice bearing Hela or Caski tumors. This study is the first to demonstrate that anti-apoptotic protein, bcl-2, and RKIP, an important regulator of cervical cancer metastasis, could play a role in DHA-mediated apoptosis. However, it will be important to understand in greater detail the mechanisms underlying DHA-mediated inhibition of tumor growth in vitro and in vivo.
Materials and Methods
Cell culture and animals
Cervical adenocarcinoma Hela cells and cervical squamous cell carcinoma Caski cells were purchased from the Cell Bank of the Chinese Academy of Sciences. Cells were maintained at 37 °C in RPMI 1640 medium supplemented with 10% FBS, 100 U/mL penicillin, and 100 mg/L streptomycin in an environment with 5% CO2.
Female BALB/c nude mice aged 4 weeks and weighing 12.5 g (n = 20) were purchased from the Experimental Animal Center of Military Medical Academy. The mice were housed in a specific pathogen free (SPF) environment for about 4 d. The relative humidity was 40–60% and the temperature was 25 ± 1 °C. The mice were subjected to 10 h light and 14 h dark cycles every day. All study protocols were approved by the Ethics Committee of the Fourth Affiliated Hospital of Harbin Medical University.
MTT assay to test the anti-tumor activity of DHA
Dihydroartemisinin (DHA) (Sigma) was dissolved in 160 mmol/L of DMSO. Hela and Caski cells were plated in 96 well plates at a density of 1 × 104 cells/well and treated with 0, 1, 5, 10, 20, 40, and 60 μM of DHA for 48 h. The optical density (OD) was measured at 546 nm and growth inhibition of cancer cells was calculated as follows: inhibition rate (%) = (ODcontrol – ODtreatment)/ODcontrol × 100%). A dose response curve was made based on the linear regression equation, and the dose of DHA at which cell viability reduced by 50% was calculated as the lethal concentration 50 (IC50) at a specific time point. All subsequent experiments were performed using 20 μmol/L DHA for 48 h.
AO/EB fluorescence staining to detect apoptosis in DHA-treated Hela cells and Caski cells
Hela and Caski cells were plated on coverslips in 24-well plates at a density of 1 × 104 cells/well. Logarithmically growing cells were treated with DHA as described above. The numbers of normal viable cells (VNA), early apoptotic cells (VA), late apoptotic cells (NVA), and necrotic cells (NVNA) were determined in untreated and in DHA-treated cells using the acridine orange/ethidium bromide staining (AO/EB) kit purchased from Nanjing Saihongrui Biotech Co., Ltd. The proportion of apoptotic cells was calculated as follows: apoptosis rate (%) = (VA + NVA)/Total cells × 100%
PI staining to detect cell cycle in DHA-treated Hela and Caski cells
Hela and Caski cells were plated in triplicate at a density of 1 × 106 cells and treated with DHA as described above. Untreated and DHA-treated cells were harvested, washed in PBS, and fixed in pre-cooled 75% ethanol. Single cell suspensions were prepared, fixed in pre-chilled 75% ethanol, and incubated at 37 °C for 30 min. After addition of 200 μl of PI solution, the samples were incubated at 37 °C for 30 min in the dark. Cells were filtered through a 400-mesh filter and the cell cycle profile was determined by flow cytometry.
RT-PCR and western blotting to determine the expression of RKIP and bcl-2 in Hela cells and Caski cells
Hela and Caski cells were plated in 96-well plates at a density of 1 × 106/well. Cells were treated with DHA as described above. Total RNA was extracted from untreated and DHA-treated cells using Trizol reagent (Invitrogen) according to the manufacturer’s recommendations. cDNA was prepared from 4 µg of total RNA using TRI Reagent RNA Isolation Reagent kit (Sigma) according to the manufacturer’s recommendations. RKIP and bcl-2 were PCR amplified from 2 µg of cDNA using primer sequences shown in according to GenBank accession number NM_002567.2 and GenBank accession number X94216. PCR was performed according to the manufacturer’s recommendations. PCR products (5 μl) were analyzed by agarose gel electrophoresis and the ratio of optical density of the target gene (RKIP or bcl-2) to that of GAPDH was expressed as the relative expression of target genes. All experiments were performed three times.
Table 2. The primers for RKIP, bcl-2, and GAPDH
Hela cells and Caski cells were plated in 96-well plates at a density of 1 × 107 cells/well and treated with DHA as described above. Total protein was extracted from untreated and DHA-treated cells using lysis buffer (0.5× TBE) and protein concentration was determined using the BCA method. Protein samples (100 µg) were electrophoresed on 5% SDS-PAGE gels and transferred to PVDF membranes for western blotting. Membranes were blocked overnight with blocking solution, and incubated with a 1:200 dilution of rabbit anti-human RKIP primary antibody, or a dilution of bcl-2 antibody (Sigma). The blots were washed and incubated with a secondary antibody (Sigma). Protein bands were detected using an ECL kit (Sigma).
Establishment of tumors in mice
Balb/c nude mice were exposed to gammaray irradiation at 400 cGy/mice for 3 d and inoculated in the right, lower limb with Hela cells or Caski cells (5 × 106 cells). When the tumor diameter was 3–4 mm, the mice were divided into 4 groups, (1) Hela control group, (2) Hela DHA group, (3) Caski control group, and (4) Caski DHA group (n = 5 per group). Mice in Hela DHA group and Caski DHA group were intraperitoneally injected with 100 μl of 20 μmol/L DHA. Mice in the Hela control group and Caski control group were intraperitoneally injected with 100 μl of PBS twice weekly.
Tumor growth inhibition
The tumor volume was evaluated every other day. The maximal length (a) and the perpendicular width (b) of the tumor were measured with vernier calipers. The tumor volume was calculated as follows: V = ab2/2 (mm3). In addition, the tumor was collected and weighed, and the growth inhibition rate was calculated as follows: inhibition rate (IR) (%) = (1 − Vtreatment/Vcontrol) × 100%. At 3 d after last treatment, nude mice were sacrificed by cervical dislocation.
Histological examination
The kidney, heart, liver, and bone marrow were collected from mice and subjected to HE and Wright-Giemsa staining to evaluate the damage to these organs in DHA treated mice.
Detection of apoptosis by TUNEL staining
The TUNEL staining kit was purchased from Beijing Zhongshan Biotech. TUNEL staining was performed according to the manufacturer’s instructions. Positive (apoptotic) cells presented under a light microscope as brown granules (blue granules after counterstaining). These cells had shrunken bodies, karyopyknosis, and apoptotic bodies. The number of apoptotic cells was determined in a total of 500 cells and the apoptosis index (AI) was calculated.
Statistical analysis
The continuous variables are presented as mean and standard deviation. One-way analysis of variance (ANOVA) was performed to determine dose effects and one-way repeated measures ANOVA was performed to determine time effects. The Student t test was used to compare the DHA and control groups. SAS software package, version 9.2 (SAS Institute Inc.) was used for all statistical analyses. All statistic assessments were evaluated at a two-sided P value of 0.05.
Disclosure of Potential Conflicts of Interest
No potential conflicts of interest were disclosed.
References
- Jemal A, Bray F, Center MM, Ferlay J, Ward E, Forman D. Global cancer statistics. CA Cancer J Clin 2011; 61:69 - 90; http://dx.doi.org/10.3322/caac.20107; PMID: 21296855
- Peralta-Zaragoza O, Deas J, Gómez-Cerón C, García-Suastegui WA, Fierros-Zárate GdelS, Jacobo-Herrera NJ. HPV-Based Screening, Triage, Treatment, and Followup Strategies in the Management of Cervical Intraepithelial Neoplasia. Obstet Gynecol Int 2013; 2013:912780; http://dx.doi.org/10.1155/2013/912780; PMID: 23690785
- Luhn P, Wentzensen N. HPV-based Tests for Cervical Cancer Screening and Management of Cervical Disease. Curr Obstet Gynecol Rep 2013; 2:76 - 85; http://dx.doi.org/10.1007/s13669-013-0040-0; PMID: 23705102
- Mathew A, George PS. Trends in incidence and mortality rates of squamous cell carcinoma and adenocarcinoma of cervix--worldwide. Asian Pac J Cancer Prev 2009; 10:645 - 50; PMID: 19827887
- Iida K, Nakayama K, Rahman MT, Rahman M, Ishikawa M, Katagiri A, Yeasmin S, Otsuki Y, Kobayashi H, Nakayama S, et al. EGFR gene amplification is related to adverse clinical outcomes in cervical squamous cell carcinoma, making the EGFR pathway a novel therapeutic target. Br J Cancer 2011; 105:420 - 7; http://dx.doi.org/10.1038/bjc.2011.222; PMID: 21730982
- Kawana K, Adachi K, Kojima S, Kozuma S, Fujii T. Therapeutic Human Papillomavirus (HPV) Vaccines: A Novel Approach. Open Virol J 2012; 6:264 - 9; http://dx.doi.org/10.2174/1874357901206010264; PMID: 23341862
- Denny L. Cervical cancer: prevention and treatment. Discov Med 2012; 14:125 - 31; PMID: 22935209
- Lee S. Artemisinin, promising lead natural product for various drug developments. Mini Rev Med Chem 2007; 7:411 - 22; http://dx.doi.org/10.2174/138955707780363837; PMID: 17430226
- Efferth T, Dunstan H, Sauerbrey A, Miyachi H, Chitambar CR. The anti-malarial artesunate is also active against cancer. Int J Oncol 2001; 18:767 - 73; PMID: 11251172
- Hien TT, White NJ. Qinghaosu. Lancet 1993; 341:603 - 8; http://dx.doi.org/10.1016/0140-6736(93)90362-K; PMID: 8094838
- Chen HH, Zhou HJ, Fang X. Inhibition of human cancer cell line growth and human umbilical vein endothelial cell angiogenesis by artemisinin derivatives in vitro. Pharmacol Res 2003; 48:231 - 6; http://dx.doi.org/10.1016/S1043-6618(03)00107-5; PMID: 12860439
- Disbrow GL, Baege AC, Kierpiec KA, Yuan H, Centeno JA, Thibodeaux CA, Hartmann D, Schlegel R. Dihydroartemisinin is cytotoxic to papillomavirus-expressing epithelial cells in vitro and in vivo. Cancer Res 2005; 65:10854 - 61; http://dx.doi.org/10.1158/0008-5472.CAN-05-1216; PMID: 16322232
- Chen H, Sun B, Pan S, Jiang H, Sun X. Dihydroartemisinin inhibits growth of pancreatic cancer cells in vitro and in vivo. Anticancer Drugs 2009; 20:131 - 40; http://dx.doi.org/10.1097/CAD.0b013e3283212ade; PMID: 19209030
- Wu GS, Lu JJ, Guo JJ, Huang MQ, Gan L, Chen XP, Wang YT. Synergistic anti-cancer activity of the combination of dihydroartemisinin and doxorubicin in breast cancer cells. Pharmacol Rep 2013; 65:453 - 9; PMID: 23744430
- Du XX, Li YJ, Wu CL, Zhou JH, Han Y, Sui H, Wei XL, Liu L, Huang P, Yuan HH, et al. Initiation of apoptosis, cell cycle arrest and autophagy of esophageal cancer cells by dihydroartemisinin. Biomed Pharmacother 2013; 67:417 - 24; http://dx.doi.org/10.1016/j.biopha.2013.01.013; PMID: 23582790
- Luo J, Chen X, Chen G, Zhou X, Lu X, Ling Y, Zhang S, Zhu W, Cao J. Dihydroartemisinin induces radiosensitivity in cervical cancer cells by modulating cell cycle progression. Saudi Med J 2013; 34:254 - 60; PMID: 23475089
- Wang SJ, Gao Y, Chen H, Kong R, Jiang HC, Pan SH, Xue DB, Bai XW, Sun B. Dihydroartemisinin inactivates NF-kappaB and potentiates the anti-tumor effect of gemcitabine on pancreatic cancer both in vitro and in vivo. Cancer Lett 2010; 293:99 - 108; http://dx.doi.org/10.1016/j.canlet.2010.01.001; PMID: 20137856
- Hagan S, Al-Mulla F, Mallon E, Oien K, Ferrier R, Gusterson B, García JJ, Kolch W. Reduction of Raf-1 kinase inhibitor protein expression correlates with breast cancer metastasis. Clin Cancer Res 2005; 11:7392 - 7; http://dx.doi.org/10.1158/1078-0432.CCR-05-0283; PMID: 16243812
- Fu Z, Smith PC, Zhang L, Rubin MA, Dunn RL, Yao Z, Keller ET. Effects of raf kinase inhibitor protein expression on suppression of prostate cancer metastasis. J Natl Cancer Inst 2003; 95:878 - 89; http://dx.doi.org/10.1093/jnci/95.12.878; PMID: 12813171
- Li HZ, Wang Y, Gao Y, Shao J, Zhao XL, Deng WM, Liu YX, Yang J, Yao Z. Effects of raf kinase inhibitor protein expression on metastasis and progression of human epithelial ovarian cancer. Mol Cancer Res 2008; 6:917 - 28; http://dx.doi.org/10.1158/1541-7786.MCR-08-0093; PMID: 18567796
- Al-Awadhi R, Husain S, Chehadeh W, Al-Jassar W, Kapila K, Al-Mulla F. Immunocytochemical detection of raf kinase inhibitor protein and human papillomavirus profiling of normal and abnormal cervical ThinPrep samples. Acta Cytol 2013; 57:259 - 65; http://dx.doi.org/10.1159/000350249; PMID: 23636063
- Al-Mulla F, Bitar MS, Taqi Z, Yeung KC. RKIP: much more than Raf kinase inhibitory protein. J Cell Physiol 2013; 228:1688 - 702; http://dx.doi.org/10.1002/jcp.24335; PMID: 23359513
- Hu CJ, Zhou L, Zhang J, Huang C, Zhang GM. Immunohistochemical detection of Raf kinase inhibitor protein in normal cervical tissue and cervical cancer tissue. J Int Med Res 2011; 39:229 - 37; http://dx.doi.org/10.1177/147323001103900125; PMID: 21672326
- Martinho O, Pinto F, Granja S, Miranda-Gonçalves V, Moreira MA, Ribeiro LF, di Loreto C, Rosner MR, Longatto-Filho A, Reis RM. RKIP inhibition in cervical cancer is associated with higher tumor aggressive behavior and resistance to cisplatin therapy. PLoS One 2013; 8:e59104; http://dx.doi.org/10.1371/journal.pone.0059104; PMID: 23527098
- Peralta-Zaragoza O, Bermúdez-Morales VH, Pérez-Plasencia C, Salazar-León J, Gómez-Cerón C, Madrid-Marina V. Targeted treatments for cervical cancer: a review. Onco Targets Ther 2012; 5:315 - 28; http://dx.doi.org/10.2147/OTT.S25123; PMID: 23144564
- Efferth T, Benakis A, Romero MR, Tomicic M, Rauh R, Steinbach D, Häfer R, Stamminger T, Oesch F, Kaina B, et al. Enhancement of cytotoxicity of artemisinins toward cancer cells by ferrous iron. Free Radic Biol Med 2004; 37:998 - 1009; http://dx.doi.org/10.1016/j.freeradbiomed.2004.06.023; PMID: 15336316
- Lu JJ, Meng LH, Cai YJ, Chen Q, Tong LJ, Lin LP, Ding J. Dihydroartemisinin induces apoptosis in HL-60 leukemia cells dependent of iron and p38 mitogen-activated protein kinase activation but independent of reactive oxygen species. Cancer Biol Ther 2008; 7:1017 - 23; http://dx.doi.org/10.4161/cbt.7.7.6035; PMID: 18414062
- Ferreira JF, Luthria DL, Sasaki T, Heyerick A. Flavonoids from Artemisia annua L. as antioxidants and their potential synergism with artemisinin against malaria and cancer. Molecules 2010; 15:3135 - 70; http://dx.doi.org/10.3390/molecules15053135; PMID: 20657468
- Zhang XM, Gu H, Yan L, Zhang GY. RKIP inhibits the malignant phenotypes of gastric cancer cells. Neoplasma 2012; Forthcoming http://dx.doi.org/10.4149/neo_2013_026; PMID: 23176280
- al-Mulla F, Bitar MS, Taqi Z, Rath O, Kolch W. RAF kinase inhibitory protein (RKIP) modulates cell cycle kinetics and motility. Mol Biosyst 2011; 7:928 - 41; http://dx.doi.org/10.1039/c0mb00208a; PMID: 21180766
- Baritaki S, Chapman A, Yeung K, Spandidos DA, Palladino M, Bonavida B. Inhibition of epithelial to mesenchymal transition in metastatic prostate cancer cells by the novel proteasome inhibitor, NPI-0052: pivotal roles of Snail repression and RKIP induction. Oncogene 2009; 28:3573 - 85; http://dx.doi.org/10.1038/onc.2009.214; PMID: 19633685
- Yeung KC, Rose DW, Dhillon AS, Yaros D, Gustafsson M, Chatterjee D, McFerran B, Wyche J, Kolch W, Sedivy JM. Raf kinase inhibitor protein interacts with NF-kappaB-inducing kinase and TAK1 and inhibits NF-kappaB activation. Mol Cell Biol 2001; 21:7207 - 17; http://dx.doi.org/10.1128/MCB.21.21.7207-7217.2001; PMID: 11585904
- Wu K, Bonavida B. The activated NF-kappaB-Snail-RKIP circuitry in cancer regulates both the metastatic cascade and resistance to apoptosis by cytotoxic drugs. Crit Rev Immunol 2009; 29:241 - 54; http://dx.doi.org/10.1615/CritRevImmunol.v29.i3.40; PMID: 19538137
- Certo M, Del Gaizo Moore V, Nishino M, Wei G, Korsmeyer S, Armstrong SA, Letai A. Mitochondria primed by death signals determine cellular addiction to antiapoptotic BCL-2 family members. Cancer Cell 2006; 9:351 - 65; http://dx.doi.org/10.1016/j.ccr.2006.03.027; PMID: 16697956
- Liu Z, Lu H, Jiang Z, Pastuszyn A, Hu CA. Apolipoprotein l6, a novel proapoptotic Bcl-2 homology 3-only protein, induces mitochondria-mediated apoptosis in cancer cells. Mol Cancer Res 2005; 3:21 - 31; PMID: 15671246
- Oltersdorf T, Elmore SW, Shoemaker AR, Armstrong RC, Augeri DJ, Belli BA, Bruncko M, Deckwerth TL, Dinges J, Hajduk PJ, et al. An inhibitor of Bcl-2 family proteins induces regression of solid tumours. Nature 2005; 435:677 - 81; http://dx.doi.org/10.1038/nature03579; PMID: 15902208
- VanBrocklin MW, Verhaegen M, Soengas MS, Holmen SL. Mitogen-activated protein kinase inhibition induces translocation of Bmf to promote apoptosis in melanoma. Cancer Res 2009; 69:1985 - 94; http://dx.doi.org/10.1158/0008-5472.CAN-08-3934; PMID: 19244105
- Schwarz CS, Evert BO, Seyfried J, Schaupp M, Kunz WS, Vielhaber S, Klockgether T, Wüllner U. Overexpression of bcl-2 results in reduction of cytochrome c content and inhibition of complex I activity. Biochem Biophys Res Commun 2001; 280:1021 - 7; http://dx.doi.org/10.1006/bbrc.2001.4242; PMID: 11162629
- Al-Mulla F, Bitar MS, Al-Maghrebi M, Behbehani AI, Al-Ali W, Rath O, Doyle B, Tan KY, Pitt A, Kolch W. Raf kinase inhibitor protein RKIP enhances signaling by glycogen synthase kinase-3β. Cancer Res 2011; 71:1334 - 43; http://dx.doi.org/10.1158/0008-5472.CAN-10-3102; PMID: 21303975