Abstract
The Hedgehog (Hh) signaling pathway has been demonstrated to play a critical role in controlling embryonic development, tissue patterning, wound healing and a variety of cell functions. Aberrant activation of Hh signaling is implicated in the pathogenesis of many human cancers, and in angiogenesis. However, the role of this pathway in uveal melanoma (UM) carcinogenesis remains unknown. In this study, we investigated the effects of Hh inhibition using the specific Smoothened (Smo) antagonist cyclopamine to block Hh signaling in cultured human UM cell lines expressing Hh signaling components. Cyclopamine treatment effectively increased apoptosis and inhibited cell proliferation, migration, and epithelial-to-mesenchymal transition (EMT) by downregulating the Hh final arbiter glioblastoma 1 (Gli1), which regulates the transcription of target genes in the nucleus. Changes in gene and protein expression levels were detected by real-time PCR and by western blotting and immunocytochemistry, respectively. Cell cycle and apoptosis regulation induced by cyclopamine were demonstrated by flow cytometry. In addition, the migration capability of UM cells was reduced, as demonstrated by transwell migration and scratch assays. The effects of Hh inhibition on the levels of angiogenesis factors secreted by UM cells were examined by tube-formation assay. Conclusion: Blocking the Hh pathway by cyclopamine decreased cell viability, migration, EMT, and angiogenesis, increased apoptosis, and induced G1 phase cell cycle arrest in UM cells. Collectively, these results provide the first evidence of the significance of Gli1 activation downstream of Smo as a therapeutic target and the potential value of cyclopamine for the treatment of human UM.
Introduction
Uveal melanoma (UM) is the most common primary malignant tumor of the eye in adults.Citation1,Citation2 UM can affect any part of the uveal tract, though choroidal melanoma accounts for the majority of cases of UM, while iris and ciliary body melanomas are far less common.Citation3 In view of its characteristic cytogenetic alterations and a strong propensity toward fatal metastasis, UM is considered to differ from cutaneous melanoma.Citation4 Unfortunately, almost half of all patients diagnosed with UM develop metastases, usually to the liver,Citation5,Citation6 and the survival rate has remained unchanged for decades.Citation7 Despite improvements in the diagnosis and local control of UM, established metastases have proven refractory to conventional chemotherapy, and are associated with a median survival time of only several months from their discovery.Citation5,Citation8 Efficient strategies to improve the treatment of UM are thus urgently needed.
The Hedgehog (Hh) signaling pathway is known to be a master developmental regulator controlling embryogenesis,Citation9 tissue patterning,Citation10 adult tissue homeostasis,Citation11 stemness,Citation12 wound healing,Citation13 regulation of the epithelial–mesenchymal transition (EMT),Citation14,Citation15 and regulating a variety of cell functions such as proliferation,Citation11 apoptosis,Citation16 autophagy,Citation17 differentiation, and migration.Citation12,Citation18 Canonical activation of the Hh pathway is initiated by binding of Hh ligands to the 12-pass-transmembrane receptor Patched (Ptch1) in Hh-responsive cells. Ptch1 then becomes internalized, resulting in activation of the 7-transmembrane spanning protein Smoothened (Smo), which is released from Ptch1-independent suppression. Smo then translocates to the cilium to promote nuclear localization of the glioblastoma (Gli) zinc finger transcription factors, including Gli1,Citation19 Gli2, and Gli3,Citation20 which ultimately regulate downstream Hh target genes, including Ptch1 ().Citation10,Citation21
Figure 1. Schematic representation of the Hh signaling pathway in the balance between on-state and off-state. Activated Gli1/2, downstream of Smo, regulate target genes of Hh signaling pathway including cyclin D, cyclin E, Myc, Gli1, and PTCH1. Cyclopamine or si-SMO (targeting Smo) blocks the functions of downstream Gli activators, resulting in inhibition of tumor cells.
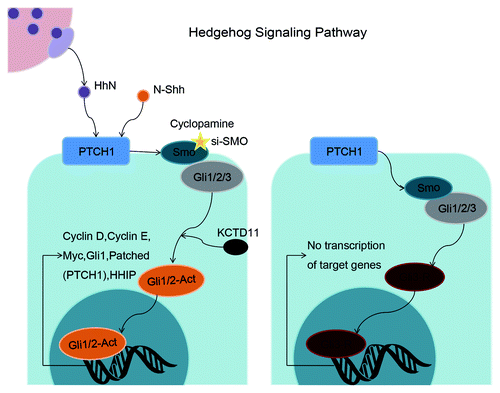
Most of the biological effects of the Hh signaling pathway are considered to be induced by transcriptional regulation of Gli1 target genes, the products of which effect critical cell fate decisions.Citation12 Although the precise role of Hh in these functions remains unclear, deregulation of Hh signaling results in the development of cancers and fibrosis. Aberrant activation of the Hh signaling pathway has been reported in the pathogenesis of several distinct cancers, including cancers of the skin, brain, muscle, and lymph, and carcinomas of the oral cavity,Citation22 stomach,Citation23 pancreas,Citation24 colon,Citation25 liver,Citation17 breast,Citation26 bladder,Citation21 ovary,Citation27 prostate,Citation28 and lung;Citation29 however, very little is known regarding the roles of Hh signaling in regulating cell functions and secretion in UM, and the downstream target genes related to the determination of cell fate.
Cyclopamine is a natural chemical belonging to the steroidal jerveratrum alkaloids, and acts as a Hh signaling pathway inhibitor by influencing the balance between the active and inactive forms of the Smo protein.Citation30 It is a teratogen that causes often fatal birth defects, including the development of a single eye (cyclopia), by preventing the fetal brain from dividing into two lobes. Cyclopamine is a useful tool for investigating the role of Hh signaling and as a potential treatment for patients with Hh-overexpressing cancers.Citation12,Citation31
Genes regulated downstream of Gli1,Citation32 which are either direct or indirect targets and involved in cell growth, include suppressor of fused homolog (SuFu),Citation26 Hedgehog-interacting protein (HHIP),Citation33 Ki67, cyclin D, and cyclin E. SuFu, a negative regulator of Hh signaling, decreases Gli1-mediated transactivation of target genes.Citation34 SuFu mutations adversely affect normal development and are associated with cancer-predisposing syndromes such as holoprosencephaly, basal cell nevus syndrome and Greig cephalopolysyndactyly syndrome.Citation35 SuFu has been shown to interact with Gli1, Gli3, and PEX26. It can detain Gli1 in the cytoplasm by combining with Gli1 directly, and can bind to the mRNA of target genes together with Gli1 to inhibit their transcription.Citation36 The regulatory component HHIP is located downstream of the Hh signaling pathway to form a negative feedback loop;Citation37 upregulation of HHIP occurs in the presence of surplus Hh ligands, suppressing their effects.Citation38 Other genes involved in EMT have also been detected,Citation34,Citation39 including genes for vimentin and epithelial cadherin (E-cadherin).Citation30 Invasion and metastasis of UM cells were inhibited through upregulation of E-cadherin and downregulation of vimentin.Citation40 In addition, the tube-forming capability of human umbilical vein endothelial cell (HUVEC) was decreased in association with reduced secretion of vascular endothelial growth factor (VEGF).Citation41
This study aimed to demonstrate the presence of aberrant activation of the Hh signaling pathway and to discuss the potential biological functions of this pathway in UM. We used cyclopamine to identify downstream targets of Gli1 responsible for regulating cellular functions in the context of UM, using two well-characterized human UM cell lines with high and low Smo expression levels, respectively. The results of this study provide evidence for the presence and regulation of several Hh components and suggest that blocking of aberrant Hh signaling pathway activation plays an inhibitory role in uveal cancer cells.
Results
Smo is expressed in human UM cell lines
We explored the effects of cyclopamine on the Hh pathway in two human UM and one normal cell lines by detecting the expression of Smo, a target gene of cyclopamine. The canonical Hh signaling pathway component Smo was expressed in the UM cell lines at the gene and protein levels, as determined by PCR () and western blotting analysis (), respectively. Smo expression was higher in OM431 cells compared with OCM290 cells, and these UM cell lines were thus used to investigate the influence of Smo expression level on the effects of cyclopamine, using ARPE19 as a normal control cell line.
Figure 2. Expression of Smo in human uveal melanoma and normal epithelial cells. (A) Expression levels of Smo mRNA in ARPE19, OCM290, and OM431 cells were detected by PCR, using β-actin as an endogenous control. (B) Grey value quantification of mRNA expression of Smo. (C) Expression levels of Smo protein were evaluated by western blotting analysis. Cellular proteins (60 μg) were separated by 10% SDS-PAGE and transferred to a PVDF membrane. Immunoblots were incubated with antibodies specific for Smo and probed with β-actin as a loading control. (D) Grey value quantification of protein expression of Smo.
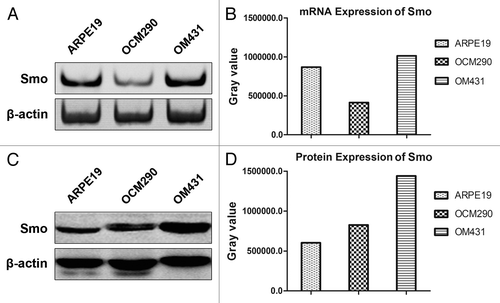
Cyclopamine downregulates expression of Gli1 and its target genes in UM cells
The effects of cyclopamine (20 μmol/L) on the mRNA expression levels of Gli1 and its target genesCitation32 in OM431 and OCM290 UM cells and control cells were determined by normal and real-time PCR after treatment for 72 h (). Smo expression was related to Ptch1, and a similar relationship existed between Gli1 and Smo. Inhibition of Hh signaling by cyclopamine downregulated the expression of Gli1, Ptch1, and Smo in all three cell lines. However, the cancer suppressor gene SuFu was increased, suppressing the viability of cancer cells. Immunocytochemistry assay and western blot analysis confirmed reduced protein expression levels of Ptch1, Smo, and Gli1, and upregulation of SuFu after 72 h culture with cyclopamine, supporting the Smo-mediated inhibitory effects of cyclopamine in human UM cells ().
Figure 3. Cyclopamine regulated the expression of genes in the Hh pathway in ARPE19, OM431, and OCM290 cells. Normal cell cultures with 0.2% DMSO were used as a negative control. (A and B) Exposure to cyclopamine (20 μmol/L; 72 h) downregulated Ptch1, Smo, and Gli1 mRNA levels and upregulated SuFu mRNA levels, as determined by PCR and real-time RT-PCR. The expression of each target gene was quantified using β-actin or GAPDH as a normalization control. Data represent the mean ± SD of three determinations (*P < 0.05; **P < 0.01). (C and D) Expression levels of cellular proteins were regulated by cyclopamine (20 μmol/L; 72 h), detected by immunocytochemistry and western blotting analysis. β-actin was used as an internal loading control. (E) Grey value quantification of protein expression on the basis of western blotting analysis.
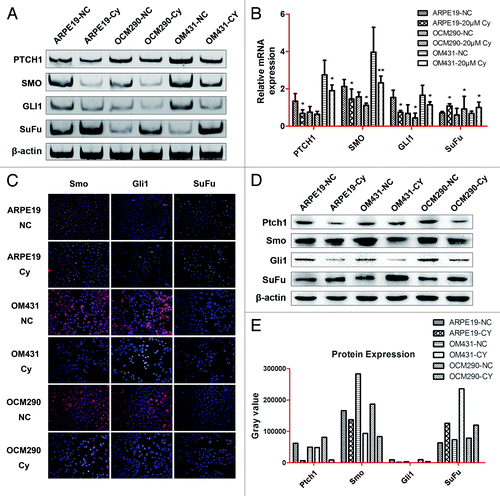
Cyclopamine reduces cell viability and proliferation and increases apoptosis in UM cells by targeting Smo
Previous studies targeting Smo with the natural inhibitor cyclopamine reported modest cytotoxicity in several human cancer cell models.Citation17,Citation30 However, its effects in human UM cell lines have not been investigated. We explored the effects of cyclopamine on cell viability and proliferation by MTT assay after treating cells with cyclopamine (20 μmol/L) or 0.2% dimethylsulfoxide (DMSO) for up to 72 h. Ki67 expression levels were detected by real-time PCR and western blot analysis, as described in Materials and Methods. Cell viability was decreased following exposure to cyclopamine in a dose-dependent manner, with maximal effect at the third day. The viabilities of the two UM cell lines were reduced by >40% at 72 h, while the reduction in ARPE19 cells was approximately 30% (). At the same time, Ki67 expression was significantly decreased by cyclopamine treatment at both the gene and protein levels, indicating that the proliferation abilities of all three cell lines were suppressed by cyclopamine. Cyclopamine was therefore employed in the subsequent mechanistic studies (). Very few apoptotic cells were observed in the attached cell population because of the rapid detachment of apoptotic cells (). Following cyclopamine treatment, floating cells in both the early and advanced stages of apoptosis were confirmed by flow cytometry (). Cyclopamine treatment thus decreased cell yields and induced apoptosis in the experimental cell lines.
Figure 4. Cyclopamine decreased viability and proliferation and increased apoptosis in uveal melanoma cells by targeting Smo. (A) ARPE19, OM431, and OCM290 cells were treated with different concentrations of cyclopamine (0, 10, and 20 μmol/L) for 4 d. Cell proliferation was lower in cells exposed to cyclopamine compared with normal controls, and the downtrends in both UM cell lines were greater than in ARPE19 cells. Six wells were set up for each concentration and the color intensity of DMSO-dissolved formazan was detected using a microplate reader at 490 nm. Data represent the mean ± SD of three determinations. (B) Ki67 mRNA expression levels were downregulated by exposure to cyclopamine (20 μmol/L; 72 h), as determined by real-time RT-PCR. GAPDH was used as a normalization control for gene expression. Data represent the mean ± SD of three determinations (*P < 0.05; **P < 0.01). (C) Ki67 protein expression levels were reduced following treatment with cyclopamine (20 μmol/L; 72 h), as detected by immunocytochemistry. Experiments were repeated three times. (D) Most UM cells that detached from the adherent monolayer and floated in the medium following cyclopamine treatment had undergone apoptosis. Photographs were taken at 100× magnification after 3 d treatment with 20 μmol/L cyclopamine. Experiments were repeated three times. (E) Dead cells in random visual fields were counted under the microscope. (F and G) Cells were pretreated as described before and stained with Annexin-V-FITC and PI. Apoptosis was analyzed by flow cytometry. Both early and advanced stages of apoptosis were increased in UM cells. Three independent experiments were performed.
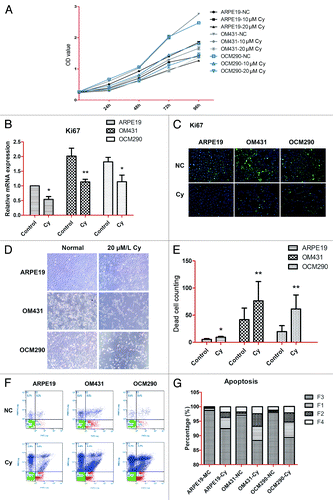
Cyclopamine inhibits colony-forming ability
The clonogenic assay is based on the principle that cell cycle arrest or cell death cause a decrease in colony numbers. This method therefore allows the evaluation of relatively long-term cell survival. Cells were treated in triplicate with 20 μmol/L cyclopamine for 72 h and the supernatant was removed and replaced with fresh medium for a further 3 d, then their clonogenic survival was determined (). OM431 and OCM290 cell colonies were reduced by >50% and ARPE19 colonies by almost 40% after 3 d exposure to cyclopamine (). Furthermore, colony size was smaller in cultures treated with cyclopamine.
Figure 5. Cyclopamine treatment increased cell death and reduced colony-forming ability in uveal melanoma cells. (A) Clonogenic assays were used to measure the effects of cyclopamine on cell proliferation in UM and normal control cells. Cyclopamine inhibited the clonogenic survival of human UM cells. Experiments were repeated three times. (B) Surviving colonies were counted in five random visual fields. Data represent the mean ± SD of three determinations (*P < 0.05; **P < 0.01).
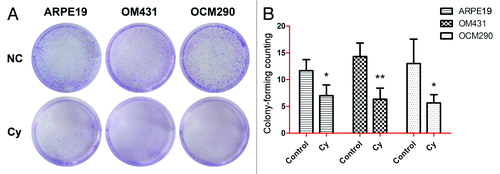
Cyclopamine affects cell cycle in UM cells
Cell lines were stained with propidium iodide (PI) to detect changes in the cell cycle by flow cytometry. The proportion of cells in G1 phase was significantly increased in cyclopamine-treated cells, indicating that Hh inhibition caused G1 phase arrest in UM cells (). In addition, cyclin D1 mRNA and protein expression levels detected by real-time PCR and immunocytochemistry assays, respectively, were reduced by exposure to cyclopamine (). Because of the special position of cyclin D1, which is located in crosstalk among different signaling pathways, the changes of mRNA and protein expression levels of cyclin D1 were not that large.
Figure 6. Cell cycle of uveal melanoma cells was influenced by cyclopamine. (A) Cell cycle changes in ARPE19, OM431, and OCM290 cells with or without cyclopamine were detected by flow cytometry. The proportion of G1 phase cells was significantly increased in UM cells. (B) Cyclin D1 mRNA expression levels were downregulated by exposure to cyclopamine (20 μmol/L; 72 h), as determined by real-time RT-PCR. GAPDH was used as a normalization control for gene expression. Data represent the mean ± SD of three determinations (*P < 0.05; **P < 0.01). (C) Cyclin D1 protein expression levels were reduced following treatment with cyclopamine (20 μmol/L; 72 h), detected by immunocytochemistry. Experiments were repeated three times.
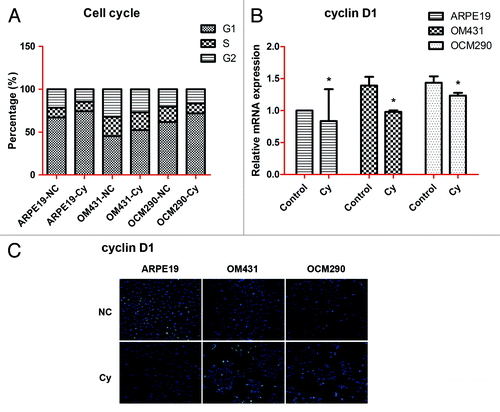
Migration and EMT of UM cells is influenced by cyclopamine
The capacity for migration contributes to the aggressiveness of UM. We assessed the effects of Hh pathway inhibition by cyclopamine on melanoma migration by in vitro transwell, scratch, and tube-formation assays. After 72 h treatment with cyclopamine, the number of cells transferred to the lower chamber in transwell experiments was reduced compared with control cultures (). With a weaker migration capability than other two tumor cell lines, there was no obvious transference of ARPE19 until the third day. Furthermore, the background of plates has a relatively steady OD value which accounts for the majority of reading at the first day and it might cover the tendency to some extent. With the passage of time, more and more cells have transferred to the lower chamber and the changes of their staining OD values would become obvious at the third and fifth day (). In addition, the rate of wound healing was reduced by cyclopamine (), as demonstrated by scratch assay. Furthermore, the tube-forming ability of HUVECs cultured with supernatant from UM cells treated with cyclopamine for 24 h was lower than that of cells with supernatant from untreated cells. Supernatant from ARPE19 cells either with or without cyclopamine treatment was unable to induce tube formation in HUVECs (). Immunocytochemistry assay showed that VEGF-α levels were reduced by cyclopamine, indicating that the secretion of tumor angiogenesis factors, such as VEGF-α, was downregulated by Hh inhibition ().
Figure 7. Effects of cyclopamine on uveal melanoma cell migration and VEGF-α secretion. (A) Representative images of transwell assays for ARPE19, OM431, and OCM290 cells, cultured with cyclopamine (20 μmol/L) or 0.2% DMSO. Cells transferred to the lower well were stained with crystal violet for 30 min before being photographed on days 1, 3, and 5. (B) The dye remaining in the cells was dissolved in 33% glacial acetic acid and migrating cells were quantified by detecting the differences in absorbance of crystal violet dilutions. The data were presented as the mean ± SD of three independent experiments. (C) Wound-healing assays were performed using ARPE19, OM431, and OCM290 cells pretreated with cyclopamine (20 μmol/L; 72 h). Cells cultured with 0.2% DMSO were used as negative control. Typical images were captured at the time wounding and 48 h later. Experiments were repeated three times (*P < 0.05; **P < 0.01). (D) Supernatants of ARPE19, OM431, and OCM290 cells treated with cyclopamine (20 μmol/L; 72 h) were used to induce tube formation in HUVECs. VEGF (10 and 100 ng/mL) served as a positive control. The tube-formation abilities of OM431 and OCM290 cells were significantly reduced by exposure to cyclopamine. Photographs were taken after 8 h under an inverted light microscope at 200× magnification. Experiments were repeated three times. (E) Expression of VEGF-α protein was reduced following treatment with cyclopamine (20 μmol/L; 72 h), detected by immunocytochemistry. Experiments were repeated three times.
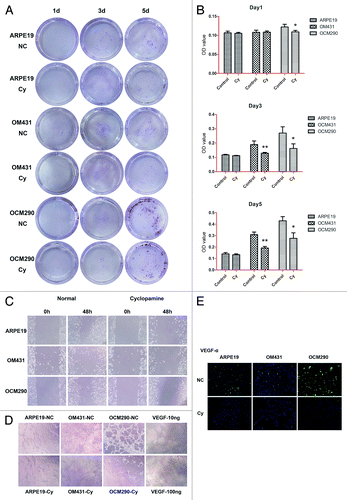
EMT is an important process in tumor progression and is characterized by the loss of cell–cell adhesion epithelial markers such as E-cadherin, and upregulation of mesenchymal marker such as vimentin.Citation39 We investigated the effects of cyclopamine on EMT using real-time PCR and immunocytochemistry to detect E-cadherin and vimentin expression at both the mRNA and protein levels. Cyclopamine (20 μmol/L) increased the expression of E-cadherin mRNA () and simultaneously decreased the expression of vimentin at both the mRNA and protein levels (). These results suggest that cyclopamine may inhibit UM progression through the Hh signaling pathway.
Figure 8. EMT was downregulated by cyclopamine in uveal melanoma cells. (A) Exposure to cyclopamine (20 μmol/L; 72 h) downregulated vimentin and upregulated E-cadherin mRNA, as determined by real-time RT-PCR. Gene expression was quantified using GAPDH as a normalization control. Data represent the mean ± SD of three determinations. (B) Vimentin protein expression was reduced following treatment with cyclopamine (20 μmol/L; 72 h), detected by immunocytochemistry.
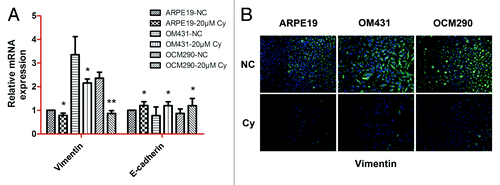
Overall, the results revealed that 20 μmol/L cyclopamine significantly inhibited the migration of ARPE19, OM431, and OCM290 cells, but had less effect on the rate of cell invasion of the less aggressive ARPE19 cells. We therefore conclude that inhibition of Hh pathway activation via blocking the function of Smo could reduce invasion and EMT in UM.
SMO-siRNA had similar results with cyclopamine in regulating expression of genes in Hh signaling pathway
To further confirm if Smo inhibition could influence UM cells through Hh pathway and siRNA targeting SMO have similar effects with cyclopamine, we used SMO-siRNA to knockdown SMO in three experimental cell lines and detected the transfection effenciency by fluorescence microscope. After counting of both transfected and non-transfected cells under microscope, we figured out the transfection efficiency of ARPE19, OM431, and OCM290 was 87.05%, 91.55%, and 91.85%, respectively (). RNAi interference on Smo downregulated Smo and Gli1 mRNA levels greater than cyclopamine did, as determined by PCR and real-time RT-PCR (). Furthermore, we used western blotting analysis to detect the protein expression levels of Smo and Gli1, regulated respectively by SMO-siRNA and cyclopamine (). And SMO-siRNA interference decreased Smo and Gli1 at both mRNA and protein levels in a larger degree than cyclopamine.
Figure 9. siRNA targeting SMO had similar results with cyclopamine in regulating the expression of genes in the Hh pathway in ARPE19, OM431, and OCM290 cells. Normal cell cultures with 0.2% DMSO, lipofectamine, and negative control siRNA were used as controls. (A and B) Transfection efficiency of siRNA in ARPE19, OM431, and OCM290 cells were observed by fluorescence microscope and calculated by cell counting of both transfected and non-transfected cells. (C and D) RNAi interference on Smo downregulated Smo and Gli1 mRNA levels greater than cyclopamine did, as determined by PCR and real-time RT-PCR. The expression of each target gene was quantified using β-actin or GAPDH as a normalization control. Data represent the mean ± SD of three determinations (*P < 0.05; **P < 0.01). (E and F) Expression levels of cellular proteins regulated respectively by si-SMO and cyclopamine were detected by western blotting analysis and corresponding gray value quantification. β-actin was used as an internal loading control.
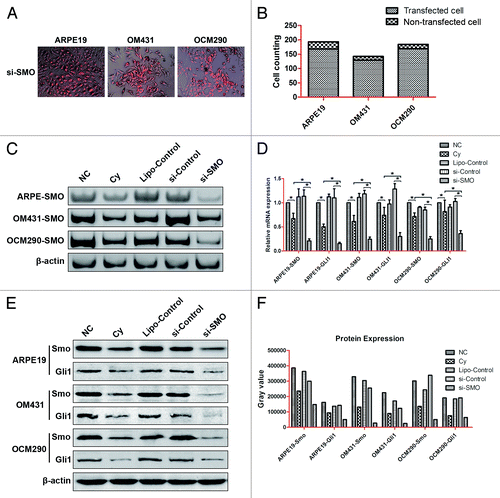
siRNA targeting SMO increased cell death as well as reduced colony-forming ability and cell migration in uveal melanoma cells
In ARPE19, OM431, and OCM290 cells, the number of survival cells after being treated with SMO-siRNA and cyclopamine were much smaller than control cultures, meanwhile, the number of dead cells increased significantly (). In addition, these three cell lines were respectively treated in triplicate with 20 μmol/L cyclopamine for 72 h and SMO-siRNA for 48 h. Their clonogenic survival was determined by colony-forming counting and no significant difference was observed between the effects caused by SMO-siRNA and cyclopamine (). We also assessed the effects of Hh pathway inhibition by SMO-siRNA and cyclopamine on melanoma migration by in vitro transwell assay. After 48 h treatment with SMO-siRNA or 72 h treatment with cyclopamine, the number of cells transferred to the lower chamber in transwell experiments was obviously reduced compared with control cultures ().
Figure 10. siRNA targeting SMO increased cell death as well as reduced colony-forming ability and cell migration in uveal melanoma cells. (A) Most UM cells that detached from the adherent monolayer and floated in the medium following siRNA transfection had undergone apoptosis. Photographs were taken at 100× magnification after 48 h treatment. Experiments were repeated three times. (B) Dead cells in random visual fields were counted under the microscope. (C) Clonogenic assays were used to measure the effects of si-SMO on cell proliferation in UM and normal control cells. siRNA targeting SMO inhibited the clonogenic survival of human UM cells. Experiments were repeated three times. (D) Surviving colonies were counted in five random visual fields. Data represent the mean ± SD of three determinations (*P < 0.05; **P < 0.01). (E) Representative images of transwell assays for ARPE19, OM431, and OCM290 cells, cultured with 0.2% DMSO, cyclopamine (20 μmol/L), si-SMO, si-Control, or lipofectamine. Cells transferred to the lower well were stained with crystal violet for 30 min before being photographed on day 3. (F) The dye remaining in the cells was dissolved in 33% glacial acetic acid and migrating cells were quantified by detecting the differences in absorbance of crystal violet dilutions. The data were presented as the mean ± SD of three independent experiments.
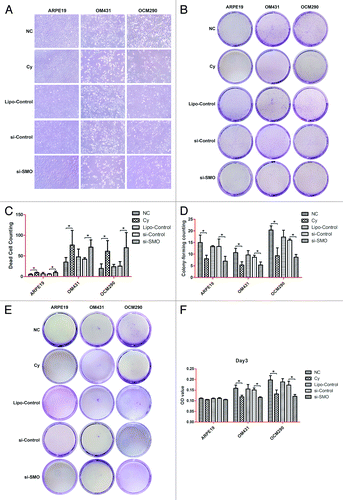
Discussion
In this study, we demonstrated that Hh signaling pathway components in UM cells were modulated by exposure to the pathway inhibitor cyclopamine. In addition, the results also suggest that the biological effects of the Hh pathway in UM cells are related to the control of cell fate and regulation of cytokine production. Hh signaling events are known to be implicated in cancer cell survival and growth in many human cancers,Citation42,Citation43 such as basal cell carcinoma,Citation44 subsets of medulloblastoma,Citation45 prostate cancer,Citation46 pancreatic cancer,Citation24 rhabdomyosarcoma,Citation47 and breast cancer.Citation26 Nevertheless, activation of the Hh pathway in UM cells under conditions of ligand blocking is unclear. We therefore investigated the role of Hh signaling in the homeostatic control of UM cell survival and function.
Cyclopamine is a natural alkaloid isolated from a member of the lily family, Veratrum californicum,Citation12,Citation31 which inhibits the Hh pathway specifically through suppressing Smo activity by binding to its heptahelical bundle.Citation48 The modulation of Hh pathway-related and other indirectly associated genes, including those for Ptch1, Smo, SuFu, cyclin D1, vimentin, E-cadherin, and importantly Gli1, were investigated at the mRNA and protein levels to reveal the mechanisms of cyclopamine effects on UM. The results provide compelling evidence to indicate that the overactivated Hh signaling pathway is suppressed by cyclopamine in UM cells in a Smo-independent manner, thus affecting UM cell fate.
The results of wounding-healing, transwell, and tube-formation assays indicated that cyclopamine could inhibit UM cell metastasis.Citation41 EMT is an important feature of human malignant tumorsCitation39 and decreased E-cadherin and increased vimentin expression are thought to play important roles in the EMT process in various human cancers.Citation40 Cyclopamine increased E-cadherin and reduced vimentin expression in UM cells, indicating that Hh signaling pathway inhibition reduced EMT. These results may explain the mechanisms whereby the small molecular inhibitor cyclopamine decreases UM metastasis and invasion.
In colony-forming and transwell assays, si-SMO interference achieved similar effects with cyclopamine, even better. In consideration of the functional mechanism of cyclopamine which acts as a Hh signaling pathway inhibitor by influencing the balance between the active and inactive forms of the Smo protein in concentration-dependent manner, while siRNA could decrease the mRNA expression directly, the results are considered reasonable. However, there would have some restrictions on applying RNAi interference, such as the cytotoxicity of transfection reagents and the different transfection efficiency vary with different cell lines. Furthermore, the inhibition of Hh pathway by targeting Smo is worked by means of regulating Gli1 functions, and there was research showed that inhibition of Gli1 could achieve better effect than inhibition of Smo.Citation30 We would carry out much deeper research later in order to remove some of the mystery about UM.
The inhibitors of Hh signaling pathway involves a wide range of types and several of them have already appeared in clinical applications. Cyclopamine, the first specific inhibitor of Hh signaling pathway, has already been researched in many different tumors intensively in the past.Citation49 In addition to this, Cur-61414 could induce apoptosis of basal cells by inhibiting Hh signal transduction through combining with Smo, what is more, it has no toxic side effect on normal skin cells.Citation50 Another small molecule compound GDC-0449 which could efficiently suppress Hh signaling activation, has already entered clinical trials.Citation51 Furthermore, itraconazole, a kind of antifungal drug used in the clinic for many years, also could inhibit Hh signaling pathway effectively.Citation52,Citation53 Accordingly, both Hh suppression and Hh specific inhibitors are rather promising and we bet that they are going to be applied in the clinic widely in the near future.
We therefore conclude that inhibition of the Hh pathway by cyclopamine would influence cell growth and migration in UM through downregulation of Gli1 expression.Citation28,Citation39 We verified that cyclopamine acts as an inhibitory modulator of the Hh signaling pathway through targeting the effects of Smo in human UM cells, and plays an important role in reducing metastasis and tumor cell EMT through decreasing Smo expression. Our results suggest that the Hh pathway component Smo represents a promising therapeutic target in UM and that its inhibitor cyclopamine has a potential role in future therapeutic strategies aimed at UM prevention and treatment.
Materials and Methods
Cell culture
The human normal retinal pigment epithelium cell line ARPE19 was generously provided by the Department of Ophthalmology, Ruijin Hospital, Shanghai Jiao Tong University School of Medicine, PR China.Citation54 The human UM cell line OM431 and OCM290 were kindly provided by Professor John F Marshall (Tumor Biology Laboratory, John Vane Science Centre, London, UK),Citation55 and the tumorigenicity of OM431 in mouse xenografts has been demonstrated previously.Citation56 Cell lines were tested biannually to confirm they were mycoplasma-free. Ham’s F-12, Dulbecco’s modified Eagle’s medium (DMEM), RPMI 1640 medium, fetal bovine serum (FBS), and penicillin–streptomycin were purchased from Gibco (Invitrogen Gibco). ARPE19 cells were maintained in F-12 supplemented with 10% FBS, OM431 cells were treated with DMEM contained 10% FBS, and OCM290 cells were grown in RPMI 1640 supplemented with 10% FBS. Culture media for all cells included penicillin (100 U/mL) and streptomycin (100 μg/mL). Cells in log phase growth were grown as monolayers in 25 cm2 culture flasks at 37 °C in a humidified atmosphere containing 5% CO2. After growing to confluence, cells were trypsinized, centrifuged and resuspended in culture medium, and some cells from each culture were frozen and maintained at −80 °C. Cells at a density of 1 × 106 cells/mL were treated specifically for each experiment in 6-, 12-, 24-, or 96-well microplates, as required. Supernatants were collected and stored at −80 °C for further measurements.
Reagents
The selective Hh inhibitor cyclopamine was provided by Selleck Chemicals and diluted in DMSO (Invitrogen) at 3 mM. Antibodies against Ptch1, cyclin D1, and β-actin were purchased from ProteinTech. Anti-Smo antibody was from Abcam, antibodies against Gli1 and SuFu were obtained from Epitomics, and antibodies against vimentin, E-cadherin, Ki67, and VEGF-α were from Boster (Wuhan Boster Biological Technology, Ltd.).
Cyclopamine treatment
Cyclopamine was dissolved in DMSO. Prior to treatment, ARPE19, OM431, and OCM290 cells were planted in flasks in triplicate and allowed to grow to approximately 30–40% confluence. Cells in the experimental group were treated with 20 μmol/L cyclopamine for 72 h and then trypsinized for counting and other analyses. Corresponding vehicle controls were prepared by adding 0.2% (v/v) DMSO.
RNA isolation and cDNA synthesis
SMO expression levels in ARPE19 and the two UM cell lines were validated by quantitative real-time RT-PCR (qRT-PCR). Total RNA was isolated from cultured cells using Trizol reagent (Sigma-Aldrich), following the manufacturer’s protocol. The RNA purity of the samples was determined using a Nanodrop 2000 UV-Vis Spectrophotometer (Thermo Scientific). The same amounts of total RNA were reverse transcribed into cDNA using PrimeScript RT reagent Kit (TaKaRa Biotechnology Co., Ltd.). Basal Smo levels were analyzed by 35 cycles of conventional PCR using the cDNA obtained previously. SMO-specific primer sequences were acquired from PrimerBank or Primer-Blast and used to generate the PCR product, which was subsequently resolved and visualized by 8% native-PAGE (PAGE).Citation57,Citation58
qRT-PCR
ARPE19, OM431, and OCM290 cells were left untreated (vehicle control, 0.2% DMSO) or treated with cyclopamine (20 μmol/L) dissolved in DMSO-containing medium. cDNA was acquired from these cells as described previously. Changes in mRNA levels were validated by real-time PCR using an ABI Prism 7500 (Applied Biosystems) for 40 cycles (95 °C for 15 s and 60 °C for 30 s) after an initial 10 min incubation at 95 °C, using Power SYBR green PCR Master Mix (Applied Biosystems). Genes for Ptch1, Smo, Gli-1, SuFu, cyclin D1, vimentin, E-cadherin, VEGF-α, and Ki67 were detected, and levels of mRNA were normalized to that of the control gene glyceraldehyde phosphate dehydrogenase (GAPDH).Citation59 The expression of each target gene was quantitated by the comparative C (T) method and determined in triplicate for each gene. Each experiment was also repeated at least three times.Citation60 Primer sequences for conventional and real-time RT-PCR were as follows:
GAPDH-F ACAACTTTGG TATCGTGGAA GG
GAPDH-R GCCATCACGC CACAGTTTC
PTCH1-F ACTTCAAGGG GTACGAGTAT GT
PTCH1-R TGCGACACTC TGATGAACCA C
SMO-F CTGTCCTGCG TCATCATCTT T
SMO-R CCACAGCAAG GATTGCCAC
GLI1-F GGGTGCCGGA AGTCATACTC
GLI1-R GCTAGGATCT GTATAGCGTT TGG
SUFU-F CACGCCATCT ACGGAGAGTG
SUFU-R GTACTTGACG ATAGCGGTAA CC
CCND1-F CAATGACCCC GCACGATTTC
CCND1-R CATGGAGGGC GGATTGGAA
VIMENTIN-F AGTCCACTGA GTACCGGAGA C
VIMENTIN-R CATTTCACGC ATCTGGCGTT C
E-CADHERIN-F ATTTTTCCCT CGACACCCGA T
E-CADHERIN-R TCCCAGGCGT AGACCAAGA
VEGFA-F AGGGCAGAAT CATCACGAAG T
VEGFA-R AGGGTCTCGA TTGGATGGCA
Ki67-F CAGTACTCGG AATGCAGCAA
Ki67-R CAGTCTTCAG GGGCTCTGTC
Immunocytochemistry assays
ARPE19, OM431, and OCM290 cells were treated with 0.2% DMSO or 20 μmol/L cyclopamine for 72 h, harvested and plated onto axenic glass coverslips in 24-well plates at 1 × 105 cells/well, and allowed to attach overnight. The coverslips with adhered cells were washed three times with phosphate-buffered saline (PBS) and fixed in 4% paraformaldehyde for 20 min. After rinsing three times in PBS on a shaker at very low speed, the coverslips were soaked in blocking buffer to block non-specific binding for 1 h. The blocking buffer was then replaced with different anti-human primary antibodies and incubated at 4 °C overnight, according to the manufacturer’s recommended concentrations (Smo, 1:200 dilution; Gli1 and SuFu, 1:250 dilution; vimentin and VEGF-α, 1:200 dilution). After washing three times in PBS, the coverlips were treated with the relevant isotype rabbit or mouse secondary antibodies (Invitrogen) for 1 h at room temperature and then washed three times in PBS and incubated in DAPI for 2 min. Five randomly selected visual fields on each coverslip were viewed under an Olympus DD70 BX51 microscope (Olympus). The excitation wavelengths were 546 nm for Smo, Gli1, and SuFu, 488 nm for Ki67, cyclin D1, vimentin, and VEGF-α, and 350 nm for DAPI.
Western blotting analysis
After treatment with 0.2% DMSO or cyclopamine, the medium was removed and the three types of cells were washed twice with PBS. Cells were lysed in RIPA lysis buffer (Beyotime Institute of Biology) and phenylmethanesulfonylfluoride mixture (100:1) for 30 min on ice. Protein extracts were purified by centrifugation and their concentrations were determined using a BCA Protein Assay Kit (Beyotime Institute of Biology). A 4× sodium dodecyl sulfate (SDS) protein loading buffer was added to the samples and boiled for 10 min. Equal amounts of proteins from all the samples were resolved by 10% SDS-PAGE and transferred to polyvinylidene difluoride membranes.Citation57 The membranes were incubated in 5% non-fat dry milk in Tris-buffered saline (TBS) for 60 min to block non-specific binding. Samples were incubated at 4 °C overnight with primary antibodies raised against Ptch1, Smo, Gli1, or SuFu, followed by three washings with TBS Tween (0.1%). The membranes were then incubated for 60 min with the corresponding secondary antibodies.Citation61 Immunoreactive proteins were detected using an Odyssey Infrared Imaging System (LI-COR Biosciences).
Measurement of cell viability
Cell viability was assessed using a toxicology assay to quantify the biofilm-forming abilities of the isolates, based on the reduction of 3-[4,5-dimetylthiazol-2-yl]-2,5-dipheniltetrazolium bromide (MTT) (Sigma-Aldrich) in vitro. Culture medium (100 μL) containing 3000 ARPE19, OM431, or OCM290 cells respectively, was seeded in 96-well plates, and the cells were treated with different concentrations of cyclopamine (0, 10, or 20 μmol/L) 12 h later for 4 d. Six wells were used for each concentration. At a selected time point each day, 10 μL of 10 mg/mL MTT solution was added into each well and incubated for 4 h in the dark at 37 °C. The supernatant was then removed and the formazan crystals were solubilized in 150 μL DMSO for 30 min at 37 °C.Citation62 The color intensity of the DMSO-dissolved formazan was detected using a microplate reader (Tecan Sunrise) at 490nm, which was shown to be the maximum absorbance wavelength. The absorbance values of the negative control were subtracted from the tested wells to eliminate false results caused by background interference. The mean value of three experiments was taken as the result.
Clonogenic assays
ARPE19, OM431, and OCM290 cells were plated at 3000 cells/well in 6-well plates. After overnight attachment, cells were fed with or without 20 μmol/L cyclopamine for 72 h, in triplicate. The supernatant was removed and replaced with fresh medium for a further 3 d. The cells were then washed three times with PBS and stained with crystal violet for 30 min.Citation25 The colonies were counted under a microscope at 100× magnification and analyzed.
Transwell migration assay
Cell migration assays were performed using 24-well transwell inserts (8 μm pore; Millipore). ARPE19, OM431 and OCM290 cells from the control and experimental groups were treated with 0.2% DMSO or 20 μmol/L cyclopamine for 3 d and then seeded into the upper chamber of a transwell insert in serum-free culture medium at a density of 5 × 104 cells/well. Medium containing 10% FBS as a chemoattractant was placed in the lower chamber. Cells that migrated through the membranes to the bottom surface of the plate were stained with 0.1% crystal violet for 30 min at room temperature after 1, 3, and 5 d incubation at 37 °C. Redundant crystal violet was washed off with PBS and the remaining dye was diluted with 33% glacial acetic acid. Migrating cells were quantified by detecting differences in absorbance of crystal violet dilutions. The assays were performed in triplicate and repeated three times.
Scratch assays
ARPE19, OM431, and OCM290 cells in the control and experimental groups were treated with 0.2% DMSO or 20 μmol/L cyclopamine for 3 d and then seeded in 6-well plates. For scratch wound assays, freshly confluent monolayers of cells were scraped off manually using a 200 μL sterile pipette tip. Care was taken to ensure that the scratch wounds were of similar widths (approximately 0.8 mm). The cell culture medium was then replaced with fresh medium containing 4 µg/mL mitomycin C (Sigma-Aldrich). Wound closure after 48 h was monitored under a phase contrast microscope at 200× magnification. Experiments were repeated three times.
Tube-formation assay
After 72 h culture with 0.2% DMSO or 20 μmol/L cyclopamine, the supernatants from ARPE19, OM431, and OCM290 cells were replaced with corresponding medium, without FBS. The new supernatants were collected 24 h later. A 24-well plate was coated with 200 µL Matrigel (BD Biosciences), which was allowed to solidify and polymerize at 37 °C for 30 min. HUVECs (1 × 105) were resuspended in 0.5 mL of the corresponding culture medium containing 1% FBS mixed with 0.5 mL supernatant and seeded into triplicate wells on top of the solidified matrigel. HUVECs treated with 10 and 100 ng/mL VEGF (R&D Systems) served as a positive control. Preliminary experiments showed that tube formation peaked after 8 h incubation, and this time point was therefore selected for further experiments. After cellular network structures were fully developed, photographs were taken under an inverted light microscope (Olympus) at 200× magnification.
Flow cytometric detection of cell cycle
ARPE19, OM431, and OCM290 cells cultured in medium at 37 °C in saturated humidity at 5% CO2 were seeded in 6-well plates at a concentration of 6 × 104 cells per well for attachment overnight. Cyclopamine (20 μmol/L) was then added to the culture medium in the wells of the experimental cells. After culturing for a further 72 h, cells from both the control and experimental groups were harvested, rinsed twice with PBS, fixed with 70% glacial ethanol, and then left at 4 °C for over 24 h, followed by centrifugation for 5 min at 1000 rpm to remove the ethanol. PI (BD Biosciences) was added to the cells, which were then incubated at 4 °C for 30 min in the dark. Flow cytometry was performed to detect the cell cycle phase. The results represent the mean values of three experiments.
Flow cytometric detection of apoptotic cells
Apoptosis was measured using an Annexin-V Apoptosis Detection Kit (eBioscience). Cells were treated as described for flow cytometric detection of the cell cycle, until harvesting. The cells were then collected by trypsinization, and washed once with PBS and binding buffer, separately. The cell pellet was resuspended in binding buffer to a concentration of 1–5 × 106 cells/mL, and 5 μL Annexin-V-fluorescein isothiocyanate (FITC) was added to 100 μL cell-resuspending solution followed by incubation for 15 min at room temperature in the dark. Then cells were washed and resuspended in 200 μL binding buffer with 5 μL PI and analyzed in a flow cytometer within 4 h, after storing at 2–8 °C in the dark.Citation25,Citation63 At least three experiments were performed.
RNAi transfections
siRNA against SMO (hs-SMO-si-1: 5′-CUAUGUCAGG CCAAUGUGAT T-3′, 5′-UCACAUUGGC CUGACAUAGT T-3′) and a negative control siRNA (NC: 5′-UUCUCCGAAC GUGUCACGUT T-3′, 5′-ACGUGACACG UUCGGAGAAT T-3′) were purchased from Biomics. Cells (1.5 × 106/well) seeded in six-well plates were transfected with 100 nM siRNA using Lipofectamine 2000 Reagent (Invitrogen) according to the manufacturer’s instructions. The cells were used for further experiments at 48 h after transfection.
Statistical analysis
The results were presented as mean ± standard deviation (SD) based on experiments conducted in triplicate. The data were analyzed using SPSS 13.0 (SPSS Inc.). The results of each assay were analyzed statistically by ANOVA. The Student t test was used to compare the means of independent samples. Statistical evaluation was performed using GraphPad Prism version 5. A P value <0.05 indicated a significant difference.
Disclosure of Potential Conflicts of Interest
No potential conflicts of interest were disclosed.
Acknowledgments
This work was supported by the Scientific Research Program of National Health and Family Planning Commission of China (201402014), the National Natural Science Foundation of China (81100676, 81372469), the Science and Technology Commission of Shanghai (11ZR1420000, 13JC1406202), and the Shanghai PuJiang Program (13PJ1405700).
References
- Mariani P, Servois V, Piperno-Neumann S. Therapeutic options in metastatic uveal melanoma. Dev Ophthalmol 2012; 49:166 - 81; http://dx.doi.org/10.1159/000328333; PMID: 22042020
- Cun B, Song X, Jia R, Zhao X, Wang H, Ge S, Fan X. Combination of oncolytic adenovirus and dacarbazine attenuates antitumor ability against uveal melanoma cells via cell cycle block. Cancer Biol Ther 2012; 13:77 - 84; http://dx.doi.org/10.4161/cbt.13.2.18436; PMID: 22336909
- Jovanovic P, Mihajlovic M, Djordjevic-Jocic J, Vlajkovic S, Cekic S, Stefanovic V. Ocular melanoma: an overview of the current status. Int J Clin Exp Pathol 2013; 6:1230 - 44; PMID: 23826405
- Marcoval J, Ferreres JR, Martín C, Gómez S, Penín RM, Ochoa de Olza M, Fabra Á. Patterns of visceral metastasis in cutaneous melanoma: a descriptive study. Actas Dermosifiliogr 2013; 104:593 - 7; http://dx.doi.org/10.1016/j.adengl.2012.12.006; PMID: 23876678
- Singh AD, Turell ME, Topham AK. Uveal melanoma: trends in incidence, treatment, and survival. Ophthalmology 2011; 118:1881 - 5; http://dx.doi.org/10.1016/j.ophtha.2011.01.040; PMID: 21704381
- Hu S, Luo Q, Cun B, Hu D, Ge S, Fan X, Chen F. The Pharmacological NF-κB Inhibitor BAY11-7082 Induces Cell Apoptosis and Inhibits the Migration of Human Uveal Melanoma Cells. Int J Mol Sci 2012; 13:15653 - 67; http://dx.doi.org/10.3390/ijms131215653; PMID: 23443086
- Virgili G, Gatta G, Ciccolallo L, Capocaccia R, Biggeri A, Crocetti E, Lutz JM, Paci E, EUROCARE Working Group. Survival in patients with uveal melanoma in Europe. Arch Ophthalmol 2008; 126:1413 - 8; http://dx.doi.org/10.1001/archopht.126.10.1413; PMID: 18852420
- Collaborative Ocular Melanoma Study Group.. Assessment of metastatic disease status at death in 435 patients with large choroidal melanoma in the Collaborative Ocular Melanoma Study (COMS): COMS report no. 15. Arch Ophthalmol 2001; 119:670 - 6; http://dx.doi.org/10.1001/archopht.119.5.670; PMID: 11346394
- Yu M, Gipp J, Yoon JW, Iannaccone P, Walterhouse D, Bushman W. Sonic hedgehog-responsive genes in the fetal prostate. J Biol Chem 2009; 284:5620 - 9; http://dx.doi.org/10.1074/jbc.M809172200; PMID: 19095649
- Kasper M, Regl G, Frischauf AM, Aberger F. GLI transcription factors: mediators of oncogenic Hedgehog signalling. Eur J Cancer 2006; 42:437 - 45; http://dx.doi.org/10.1016/j.ejca.2005.08.039; PMID: 16406505
- Ruiz i Altaba A, Mas C, Stecca B. The Gli code: an information nexus regulating cell fate, stemness and cancer. Trends Cell Biol 2007; 17:438 - 47; http://dx.doi.org/10.1016/j.tcb.2007.06.007; PMID: 17845852
- Varnat F, Duquet A, Malerba M, Zbinden M, Mas C, Gervaz P, Ruiz i Altaba A. Human colon cancer epithelial cells harbour active HEDGEHOG-GLI signalling that is essential for tumour growth, recurrence, metastasis and stem cell survival and expansion. EMBO Mol Med 2009; 1:338 - 51; http://dx.doi.org/10.1002/emmm.200900039; PMID: 20049737
- Lum L, Beachy PA. The Hedgehog response network: sensors, switches, and routers. Science 2004; 304:1755 - 9; http://dx.doi.org/10.1126/science.1098020; PMID: 15205520
- Siegel R, DeSantis C, Virgo K, Stein K, Mariotto A, Smith T, Cooper D, Gansler T, Lerro C, Fedewa S, et al. Cancer treatment and survivorship statistics, 2012. CA Cancer J Clin 2012; 62:220 - 41; http://dx.doi.org/10.3322/caac.21149; PMID: 22700443
- Hooper JE, Scott MP. Communicating with Hedgehogs. Nat Rev Mol Cell Biol 2005; 6:306 - 17; http://dx.doi.org/10.1038/nrm1622; PMID: 15803137
- Kobune M, Takimoto R, Murase K, Iyama S, Sato T, Kikuchi S, Kawano Y, Miyanishi K, Sato Y, Niitsu Y, et al. Drug resistance is dramatically restored by hedgehog inhibitors in CD34+ leukemic cells. Cancer Sci 2009; 100:948 - 55; http://dx.doi.org/10.1111/j.1349-7006.2009.01111.x; PMID: 19245435
- Wang Y, Han C, Lu L, Magliato S, Wu T. Hedgehog signaling pathway regulates autophagy in human hepatocellular carcinoma cells. Hepatology 2013; 58:995 - 1010; http://dx.doi.org/10.1002/hep.26394; PMID: 23504944
- Alexaki VI, Javelaud D, Van Kempen LC, Mohammad KS, Dennler S, Luciani F, Hoek KS, Juàrez P, Goydos JS, Fournier PJ, et al. GLI2-mediated melanoma invasion and metastasis. J Natl Cancer Inst 2010; 102:1148 - 59; http://dx.doi.org/10.1093/jnci/djq257; PMID: 20660365
- Rossi M, Magnoni L, Miracco C, Mori E, Tosi P, Pirtoli L, Tini P, Oliveri G, Cosci E, Bakker A. β-catenin and Gli1 are prognostic markers in glioblastoma. Cancer Biol Ther 2011; 11:753 - 61; http://dx.doi.org/10.4161/cbt.11.8.14894; PMID: 21321483
- Steg A, Amm HM, Novak Z, Frost AR, Johnson MR. Gli3 mediates cell survival and sensitivity to cyclopamine in pancreatic cancer. Cancer Biol Ther 2010; 10:893 - 902; http://dx.doi.org/10.4161/cbt.10.9.13252; PMID: 20814245
- Katoh Y, Katoh M. Hedgehog target genes: mechanisms of carcinogenesis induced by aberrant hedgehog signaling activation. Curr Mol Med 2009; 9:873 - 86; http://dx.doi.org/10.2174/156652409789105570; PMID: 19860666
- Snijders AM, Schmidt BL, Fridlyand J, Dekker N, Pinkel D, Jordan RC, Albertson DG. Rare amplicons implicate frequent deregulation of cell fate specification pathways in oral squamous cell carcinoma. Oncogene 2005; 24:4232 - 42; http://dx.doi.org/10.1038/sj.onc.1208601; PMID: 15824737
- Berman DM, Karhadkar SS, Maitra A, Montes De Oca R, Gerstenblith MR, Briggs K, Parker AR, Shimada Y, Eshleman JR, Watkins DN, et al. Widespread requirement for Hedgehog ligand stimulation in growth of digestive tract tumours. Nature 2003; 425:846 - 51; http://dx.doi.org/10.1038/nature01972; PMID: 14520411
- Bailey JM, Mohr AM, Hollingsworth MA. Sonic hedgehog paracrine signaling regulates metastasis and lymphangiogenesis in pancreatic cancer. Oncogene 2009; 28:3513 - 25; http://dx.doi.org/10.1038/onc.2009.220; PMID: 19633682
- Mazumdar T, DeVecchio J, Shi T, Jones J, Agyeman A, Houghton JA. Hedgehog signaling drives cellular survival in human colon carcinoma cells. Cancer Res 2011; 71:1092 - 102; http://dx.doi.org/10.1158/0008-5472.CAN-10-2315; PMID: 21135115
- Zhuang Z, Wang K, Cheng X, Qu X, Jiang B, Li Z, Luo J, Shao Z, Duan T. LKB1 inhibits breast cancer partially through repressing the Hedgehog signaling pathway. PLoS One 2013; 8:e67431; http://dx.doi.org/10.1371/journal.pone.0067431; PMID: 23861764
- Szkandera J, Kiesslich T, Haybaeck J, Gerger A, Pichler M. Hedgehog signaling pathway in ovarian cancer. Int J Mol Sci 2013; 14:1179 - 96; http://dx.doi.org/10.3390/ijms14011179; PMID: 23303278
- Sanchez P, Hernández AM, Stecca B, Kahler AJ, DeGueme AM, Barrett A, Beyna M, Datta MW, Datta S, Ruiz i Altaba A. Inhibition of prostate cancer proliferation by interference with SONIC HEDGEHOG-GLI1 signaling. Proc Natl Acad Sci U S A 2004; 101:12561 - 6; http://dx.doi.org/10.1073/pnas.0404956101; PMID: 15314219
- Bermudez O, Hennen E, Koch I, Lindner M, Eickelberg O. Gli1 mediates lung cancer cell proliferation and Sonic Hedgehog-dependent mesenchymal cell activation. PLoS One 2013; 8:e63226; http://dx.doi.org/10.1371/journal.pone.0063226; PMID: 23667589
- Li X, Ma Q, Xu Q, Liu H, Lei J, Duan W, Bhat K, Wang F, Wu E, Wang Z. SDF-1/CXCR4 signaling induces pancreatic cancer cell invasion and epithelial-mesenchymal transition in vitro through non-canonical activation of Hedgehog pathway. Cancer Lett 2012; 322:169 - 76; http://dx.doi.org/10.1016/j.canlet.2012.02.035; PMID: 22450749
- Qualtrough D, Buda A, Gaffield W, Williams AC, Paraskeva C. Hedgehog signalling in colorectal tumour cells: induction of apoptosis with cyclopamine treatment. Int J Cancer 2004; 110:831 - 7; http://dx.doi.org/10.1002/ijc.20227; PMID: 15170664
- Yoshimoto AN, Bernardazzi C, Carneiro AJ, Elia CC, Martinusso CA, Ventura GM, Castelo-Branco MT, de Souza HS. Hedgehog pathway signaling regulates human colon carcinoma HT-29 epithelial cell line apoptosis and cytokine secretion. PLoS One 2012; 7:e45332; http://dx.doi.org/10.1371/journal.pone.0045332; PMID: 23028941
- Götschel F, Berg D, Gruber W, Bender C, Eberl M, Friedel M, Sonntag J, Rüngeler E, Hache H, Wierling C, et al. Synergism between Hedgehog-GLI and EGFR signaling in Hedgehog-responsive human medulloblastoma cells induces downregulation of canonical Hedgehog-target genes and stabilized expression of GLI1. PLoS One 2013; 8:e65403; http://dx.doi.org/10.1371/journal.pone.0065403; PMID: 23762360
- Lei J, Ma J, Ma Q, Li X, Liu H, Xu Q, Duan W, Sun Q, Xu J, Wu Z, et al. Hedgehog signaling regulates hypoxia induced epithelial to mesenchymal transition and invasion in pancreatic cancer cells via a ligand-independent manner. Mol Cancer 2013; 12:66; http://dx.doi.org/10.1186/1476-4598-12-66; PMID: 23786654
- Stone DM, Murone M, Luoh S, Ye W, Armanini MP, Gurney A, Phillips H, Brush J, Goddard A, de Sauvage FJ, et al. Characterization of the human suppressor of fused, a negative regulator of the zinc-finger transcription factor Gli. J Cell Sci 1999; 112:4437 - 48; PMID: 10564661
- Law KK, Makino S, Mo R, Zhang X, Puviindran V, Hui CC. Antagonistic and cooperative actions of Kif7 and Sufu define graded intracellular Gli activities in Hedgehog signaling. PLoS One 2012; 7:e50193; http://dx.doi.org/10.1371/journal.pone.0050193; PMID: 23166838
- Bak M, Hansen C, Friis Henriksen K, Tommerup N. The human hedgehog-interacting protein gene: structure and chromosome mapping to 4q31.21-->q31.3. Cytogenet Cell Genet 2001; 92:300 - 3; http://dx.doi.org/10.1159/000056918; PMID: 11435703
- Martin ST, Sato N, Dhara S, Chang R, Hustinx SR, Abe T, Maitra A, Goggins M. Aberrant methylation of the Human Hedgehog interacting protein (HHIP) gene in pancreatic neoplasms. Cancer Biol Ther 2005; 4:728 - 33; http://dx.doi.org/10.4161/cbt.4.7.1802; PMID: 15970691
- Thiery JP, Acloque H, Huang RY, Nieto MA. Epithelial-mesenchymal transitions in development and disease. Cell 2009; 139:871 - 90; http://dx.doi.org/10.1016/j.cell.2009.11.007; PMID: 19945376
- Iwatsuki M, Mimori K, Yokobori T, Ishi H, Beppu T, Nakamori S, Baba H, Mori M. Epithelial-mesenchymal transition in cancer development and its clinical significance. Cancer Sci 2010; 101:293 - 9; http://dx.doi.org/10.1111/j.1349-7006.2009.01419.x; PMID: 19961486
- Bailey JM, Swanson BJ, Hamada T, Eggers JP, Singh PK, Caffery T, Ouellette MM, Hollingsworth MA. Sonic hedgehog promotes desmoplasia in pancreatic cancer. Clin Cancer Res 2008; 14:5995 - 6004; http://dx.doi.org/10.1158/1078-0432.CCR-08-0291; PMID: 18829478
- Yang L, Xie G, Fan Q, Xie J. Activation of the hedgehog-signaling pathway in human cancer and the clinical implications. Oncogene 2010; 29:469 - 81; http://dx.doi.org/10.1038/onc.2009.392; PMID: 19935712
- Pasca di Magliano M, Hebrok M. Hedgehog signalling in cancer formation and maintenance. Nat Rev Cancer 2003; 3:903 - 11; http://dx.doi.org/10.1038/nrc1229; PMID: 14737121
- Athar M, Li C, Tang X, Chi S, Zhang X, Kim AL, Tyring SK, Kopelovich L, Hebert J, Epstein EH Jr., et al. Inhibition of smoothened signaling prevents ultraviolet B-induced basal cell carcinomas through regulation of Fas expression and apoptosis. Cancer Res 2004; 64:7545 - 52; http://dx.doi.org/10.1158/0008-5472.CAN-04-1393; PMID: 15492281
- Ward RJ, Lee L, Graham K, Satkunendran T, Yoshikawa K, Ling E, Harper L, Austin R, Nieuwenhuis E, Clarke ID, et al. Multipotent CD15+ cancer stem cells in patched-1-deficient mouse medulloblastoma. Cancer Res 2009; 69:4682 - 90; http://dx.doi.org/10.1158/0008-5472.CAN-09-0342; PMID: 19487286
- Thiyagarajan S, Bhatia N, Reagan-Shaw S, Cozma D, Thomas-Tikhonenko A, Ahmad N, Spiegelman VS. Role of GLI2 transcription factor in growth and tumorigenicity of prostate cells. Cancer Res 2007; 67:10642 - 6; http://dx.doi.org/10.1158/0008-5472.CAN-07-2015; PMID: 18006803
- Ecke I, Rosenberger A, Obenauer S, Dullin C, Aberger F, Kimmina S, Schweyer S, Hahn H. Cyclopamine treatment of full-blown Hh/Ptch-associated RMS partially inhibits Hh/Ptch signaling, but not tumor growth. Mol Carcinog 2008; 47:361 - 72; http://dx.doi.org/10.1002/mc.20394; PMID: 17963245
- Wu JY, Xu XF, Xu L, Niu PQ, Wang F, Hu GY, Wang XP, Guo CY. Cyclopamine blocked the growth of colorectal cancer SW116 cells by modulating some target genes of Gli1 in vitro. Hepatogastroenterology 2011; 58:1511 - 8; http://dx.doi.org/10.5754/hge10765; PMID: 21940310
- Chen JK, Taipale J, Cooper MK, Beachy PA. Inhibition of Hedgehog signaling by direct binding of cyclopamine to Smoothened. Genes Dev 2002; 16:2743 - 8; http://dx.doi.org/10.1101/gad.1025302; PMID: 12414725
- Williams JA, Guicherit OM, Zaharian BI, Xu Y, Chai L, Wichterle H, Kon C, Gatchalian C, Porter JA, Rubin LL, et al. Identification of a small molecule inhibitor of the hedgehog signaling pathway: effects on basal cell carcinoma-like lesions. Proc Natl Acad Sci U S A 2003; 100:4616 - 21; http://dx.doi.org/10.1073/pnas.0732813100; PMID: 12679522
- Robarge KD, Brunton SA, Castanedo GM, Cui Y, Dina MS, Goldsmith R, Gould SE, Guichert O, Gunzner JL, Halladay J, et al. GDC-0449-a potent inhibitor of the hedgehog pathway. Bioorg Med Chem Lett 2009; 19:5576 - 81; http://dx.doi.org/10.1016/j.bmcl.2009.08.049; PMID: 19716296
- Kim J, Tang JY, Gong R, Kim J, Lee JJ, Clemons KV, Chong CR, Chang KS, Fereshteh M, Gardner D, et al. Itraconazole, a commonly used antifungal that inhibits Hedgehog pathway activity and cancer growth. Cancer Cell 2010; 17:388 - 99; http://dx.doi.org/10.1016/j.ccr.2010.02.027; PMID: 20385363
- Antonarakis ES, Heath EI, Smith DC, Rathkopf D, Blackford AL, Danila DC, King S, Frost A, Ajiboye AS, Zhao M, et al. Repurposing itraconazole as a treatment for advanced prostate cancer: a noncomparative randomized phase II trial in men with metastatic castration-resistant prostate cancer. Oncologist 2013; 18:163 - 73; http://dx.doi.org/10.1634/theoncologist.2012-314; PMID: 23340005
- Huang X, Wang L, Zhang H, Wang H, Zhao X, Qian G, Hu J, Ge S, Fan X. Therapeutic efficacy by targeting correction of Notch1-induced aberrants in uveal tumors. PLoS One 2012; 7:e44301; http://dx.doi.org/10.1371/journal.pone.0044301; PMID: 22937170
- Huang X, Jia R, Zhao X, Liu B, Wang H, Wang J, Zhou Y, Cun B, Ge S, Fan X. Recombinant oncolytic adenovirus H101 combined with siBCL2: cytotoxic effect on uveal melanoma cell lines. Br J Ophthalmol 2012; 96:1331 - 8; http://dx.doi.org/10.1136/bjophthalmol-2011-301470; PMID: 22843987
- Zhou Y, Song X, Jia R, Wang H, Dai L, Xu X, Gu P, Ge S, Fan X. Radiation-inducible human tumor necrosis factor-related apoptosis-inducing ligand (TRAIL) gene therapy: a novel treatment for radioresistant uveal melanoma. Pigment Cell Melanoma Res 2010; 23:661 - 74; http://dx.doi.org/10.1111/j.1755-148X.2010.00729.x; PMID: 20546535
- Zhang H, Jiao W, Sun L, Fan J, Chen M, Wang H, Xu X, Shen A, Li T, Niu B, et al. Intrachromosomal looping is required for activation of endogenous pluripotency genes during reprogramming. Cell Stem Cell 2013; 13:30 - 5; http://dx.doi.org/10.1016/j.stem.2013.05.012; PMID: 23747202
- Zhang H, Niu B, Hu JF, Ge S, Wang H, Li T, Ling J, Steelman BN, Qian G, Hoffman AR. Interruption of intrachromosomal looping by CCCTC binding factor decoy proteins abrogates genomic imprinting of human insulin-like growth factor II. J Cell Biol 2011; 193:475 - 87; http://dx.doi.org/10.1083/jcb.201101021; PMID: 21536749
- Schmittgen TD, Livak KJ. Analyzing real-time PCR data by the comparative C(T) method. Nat Protoc 2008; 3:1101 - 8; http://dx.doi.org/10.1038/nprot.2008.73; PMID: 18546601
- Yao Y, Wang L, Zhang H, Wang H, Zhao X, Zhang Y, Zhang L, Fan X, Qian G, Hu JF, et al. A novel anticancer therapy that simultaneously targets aberrant p53 and Notch activities in tumors. PLoS One 2012; 7:e46627; http://dx.doi.org/10.1371/journal.pone.0046627; PMID: 23071601
- Lim KJ, Bisht S, Bar EE, Maitra A, Eberhart CG. A polymeric nanoparticle formulation of curcumin inhibits growth, clonogenicity and stem-like fraction in malignant brain tumors. Cancer Biol Ther 2011; 11:464 - 73; http://dx.doi.org/10.4161/cbt.11.5.14410; PMID: 21193839
- Zhang H, Wang H, Zhang J, Qian G, Niu B, Fan X, Lu J, Hoffman AR, Hu JF, Ge S. Enhanced therapeutic efficacy by simultaneously targeting two genetic defects in tumors. Mol Ther 2009; 17:57 - 64; http://dx.doi.org/10.1038/mt.2008.236; PMID: 19018252
- Song X, Wang H, Jia R, Cun B, Zhao X, Zhou Y, Xu X, Qian G, Ge S, Fan X. Combined Treatment with an Oncolytic Adenovirus and Antitumor Activity of Vincristine against Retinoblastoma Cells. Int J Mol Sci 2012; 13:10736 - 49; http://dx.doi.org/10.3390/ijms130910736; PMID: 23109819