Abstract
We evaluated the capacity for supernatants (SNs) derived from Escherichia coli Nissle 1917 (EcN), cultured under different growth conditions, to prevent 5-fluorouracil (5-FU)-induced intestinal epithelial cell damage. EcN was cultured in: Luria Bertani (LB) broth, tryptone soya broth (TSB), de Man Rogosa Sharpe (MRS) broth, and M17 broth supplemented with 10% (v/v) lactose solution (M17). Intestinal epithelial cells (IEC-6) were treated with the following EcN SNs: LB+, TSB+, MRS+, and M17+ in the presence and absence of 5-FU (1.5 or 5 μM). Cell viability, apoptotic activity and cell monolayer permeability were measured by 3-(4,5-dimethylthiazol-2-yl)-2,5-diphenyltetrazolium bromide (MTT), flow cytometry, and transepithelial electrical resistance (TER) assays, respectively. 5-FU significantly reduced cell viability (P < 0.05) at both 24 and 48 h. However, only EcN SN produced from LB and M17 growth media significantly decreased cell death induced by 5-FU (by approximately 10% after 24 and 48 h; and 10% after 24 h, respectively [P < 0.05]). When measured by flow cytometry all EcN SNs in the presence of 5-FU increased the proportion of viable cells (by 3–5% for 24 h, 3–7% for 48 h, P < 0.05) and reduced late-apoptotic cells after 24 and 48 h, compared with 5-FU control. Moreover, all EcN SNs significantly reduced the disruption of IEC-6 cell barrier function induced by 5-FU by 7–10% (P < 0.05), compared with DMEM control. We conclude that EcN derived factors could potentially reduce the severity of intestinal mucositis.
Keywords: :
Introduction
Mucositis is a debilitating side effect of cancer chemotherapy, which results from mucosal injury to the alimentary tract.Citation1 Clinical studies have reported increased apoptosis in intestinal crypts, hypoplastic villous atrophy, and enterocyte tight junction disruption in patients following chemotherapy.Citation2 The clinical manifestation of mucositis include severe pain resulting from ulceration and bleeding along the gastrointestinal tract, nausea, and malnutrition, which in extreme cases may lead to death.Citation1 Moreover, depending on the dose of chemotherapy and nature of the neoplasm, 40–100% of all cancer patients are afflicted by mucositis.Citation3 Currently, mucositis cannot be treated effectively. New treatments are required which will relieve patients from the side effects of chemotherapy, but not compromise its effectiveness.
The probiotic bacterium, Escherichia coli Nissle 1917 (EcN), has been studied for its therapeutic potential against various intestinal disorders such as inflammatory bowel disease (IBD).Citation4 EcN has been found to be effective in the treatment of ulcerative colitisCitation4 and gut inflammationCitation5 in humans. EcN has also been reported to improve mucosal integrity in the colon in mice following dextran sodium sulfate (DSS)-induced damage by modulating the tight junction molecule, ZO-1, thereby reducing intestinal permeability.Citation6 EcN has been shown to regulate the intestinal epithelial cell differentiation factors Hes1, Hath1, and KLF4, Muc1 and HBD2 in mice, and in the LS174T colon adenocarcinoma cell line.Citation7 Moreover, EcN co-cultured with Caco-2 and mucin-producing LS-174T cells antagonized the activity of some strains of enterohemorrhagic Escherichia coli (EHEC), which is responsible for the hemolytic uremic syndrome.Citation8 In addition, EcN has demonstrated anti-pathogenic properties against Crohn disease-associated E. coli LF82Citation9 and its flagellum has been reported to play a pivotal role in competition against other pathogens.Citation10
Probiotic SNs are able to compete with pathogens, maintain intestinal integrity, and are involved in immune reactivity in vivo and in vitro, which may contribute to their applicability in bowel disorders.Citation11-Citation13 However, the potential utility of factors derived from EcN has been investigated to a far lesser extent. Supernatant (SN) from EcN partially protected the small intestine from 5-fluorouracil (5-FU)-induced damage in ratsCitation14 and in IEC-6 cells when grown in tryptone soya broth (TSB).Citation15 However, the underlying mechanisms of SN for these protective effects were not defined.Citation14,Citation15 Moreover, EcN SN has demonstrated promise in the treatment of human gastrointestinal motility disorders when grown in Standard-I-Bouillon growth medium.Citation16
It is becoming apparent that the composition of probiotic supernatants will likely depend on the composition of the growth medium. Different growth media could thereby promote the release of different factors from the same strain of probiotic, which in turn could result in differential efficacy in the context of intestinal damage. For example, the probiotic strain, Streptococcus thermophilus, grown in M17 broth supplemented with 1% lactose or in skim Marguerite milk, resulted in the production of different proteome profiles.Citation17 In addition, Streptococcus thermophilus grown in milk produced higher levels of enzymes such as BCAA aminotransferase, ketol-acid reductoisomerase, and pyruvate formate-lyase. These enzymes play important roles in purine biosynthesis associated with the growth of Streptococcus thermophilus.Citation17 However, these enzymes were barely detectable when this same probiotic was grown in M17 medium.
In the current study, IEC-6 cells, derived from rat ileum, were used as non-malignant intestinal cells.Citation18 IEC-6 cells have been used previously to investigate gastrointestinal toxicity induced by chemotherapeutic drugs such as 5-FU and methotrexate (MTX).Citation18,Citation19
The current study primarily aimed to identify a mechanism of action for EcN SNs when applied to IEC-6 cells in the presence and absence of the antimetabolite chemotherapy drug, 5-FU. Specifically, effects on IEC-6 cell viability, apoptosis, and monolayer permeability were sought. The second aim was to determine if EcN cultured in a range of different growth media resulted in the release of factors that would differentially impact on these parameters.
Results
Effects of different broths and EcN SNs on cell viability of IEC-6 cells in the presence and absence of 5-FU
The cell viability of IEC-6 cells cultured for up to 48 h in all broths and EcN SNs, at concentrations of 0.0001 to 100 μg/mL were not significantly different compared with Dulbecco’s modified Eagle medium (DMEM) controls (100%) (–). However, when the concentrations of broths and EcN SNs increased to 1 mg/mL, the numbers of viable cells differed. LB, TSB and M17 (1 mg/mL), at both 24 and 48 h, significantly reduced cell viability, compared with DMEM controls (,, and ). MRS at a concentration of 1 mg/mL significantly reduced IEC-6 cell viability at 48 h, compared with DMEM control ().
Figure 1. Combined effects of LB cultured EcN SNs and 5-FU (µM) on the viability of IEC-6 cells for 24 h (A) or 48 h (B) Cells were treated with DMEM, dried LB re-suspended in DMEM (LB-) to reach final concentrations of 0.0001, 0.01, 1, 100 µg/mL, and 1 mg/mL, or dried EcN SN grown in LB re-suspended in DMEM (LB+) to reach final concentrations of 0.0001, 0.01, 1, 100 µg/mL, and 1 mg/mL, either alone or in combination with 5-FU (1.25 µM). Data are expressed as percentage of viable cells relative to untreated cell controls. Data are presented as means ± SEM of three independent experiments (n = 9). Bar data not sharing the same letter are significantly different (P < 0.05). *Indicates a significant difference compared with 5-FU control in all 5-FU treatment groups (P < 0.05).
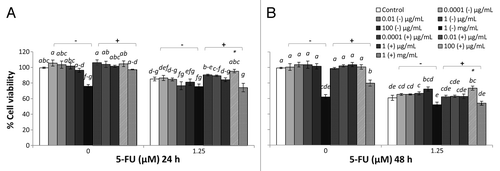
Figure 4. Combined effects of M17 broth supplemented with 10% (v/v) lactose solution (M17) broth cultured EcN SN and 5-FU (µM) on viability of IEC-6 cells for 24 h (A) or 48 h (B). Cells were treated with DMEM, dried M17 broth re-suspended in DMEM (M17-) to reach final concentrations of 0.0001, 0.01, 1, 100 µg/mL, and 1 mg/mL, or dried EcN SN had grown in M17 broth re-suspended in DMEM (M17+) to reach final concentrations of 0.0001, 0.01, 1, 100 µg/mL, and 1 mg/mL, either alone or in combination with 5-FU (1.25 µM). Data are expressed as percentage of viable cells relative to untreated cell controls. Data are presented as means ± SEM of three independent experiments (n = 9). Bar data not sharing the same letter are significantly different (P < 0.05). *Indicates a significant difference compared with 5-FU control in all 5-FU treatment groups (P < 0.05).
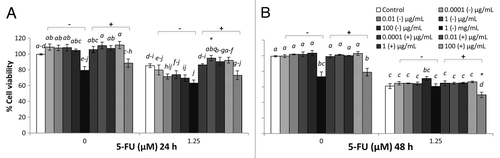
Figure 2. Combined effects of TSB-cultured EcN SN and 5-FU (µM) on the viability of IEC-6 cells for 24 h (A) or 48 h (B). Cells were treated with DMEM, dried TSB re-suspended in DMEM (TSB−) to reach final concentrations of 0.0001, 0.01, 1, 100 µg/mL, and 1 mg/mL, or dried EcN SN grown in TSB broth re-suspended in DMEM (TSB+) to reach final concentrations of 0.0001, 0.01, 1, 100 µg/mL, and 1 mg/mL, either alone or in combination with 5-FU (1.25 µM). Data are expressed as percentage of viable cells relative to untreated cell controls. Data are presented as means ± SEM of three independent experiments (n = 9). Bar data not sharing the same letter are significantly different (P < 0.05). *Indicates a significant difference compared with 5-FU control in all 5-FU treatment groups (P < 0.05).
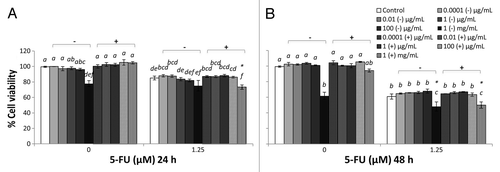
Figure 3. Combined effects of MRS broth cultured EcN SN and 5-FU (µM) on the viability of IEC-6 cells for 24 h (A) or 48 h (B). Cells were treated with DMEM, dried MRS broth re-suspended in DMEM (MRS−) to reach final concentrations of 0.0001, 0.01, 1, 100 µg/mL, and 1 mg/mL, or dried EcN SN grown in MRS broth re-suspended in DMEM (MRS+) to reach final concentrations of 0.0001, 0.01, 1, 100 µg/mL, and 1 mg/mL, either alone or in combination with 5-FU (1.25 µM). Data are expressed as percentage of viable cells relative to untreated cell controls. Data are presented as means ± SEM of three independent experiments (n = 9). Bar data not sharing the same letter are significantly different (P < 0.05). *Indicates a significant difference compared with 5-FU control in all 5-FU treatment groups (P < 0.05).
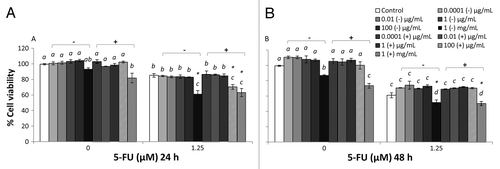
LB+ (1 mg/mL) did not affect the viability of IEC-6 cells (100%) at 24 h (). However, LB+ significantly reduced viability at 48 h (), compared with DMEM control, whereas TSB+ (1 mg/mL) did not reduce cell viability compared with DMEM controls at both 24 and 48 h (). MRS+ (1 mg/mL), significantly reduced IEC-6 cell viability at both 24 and 48 h (). Similar toxic effects on IEC-6 cells were also observed with 1 mg/mL M17+ at both 24 and 48 h, decreasing viability to approximately 80% ().
5-FU treatment reduced cell viability in all treatment groups compared with DMEM treated controls. The cell viability of 5-FU treated IEC-6 cells (5-FU control) was significantly reduced, to approximately 80% at 24 h and 60% at 48 h, compared with DMEM control (P < 0.05). All broths at a concentration range of 0.0001 μg/mL to 1 mg/mL, together with 5-FU, tended to decrease IEC-6 cell viability to approximately 80% at 24 h and 60% at 48 h, which was consistent with 5-FU control (–). TSB and MRS (1 mg/mL), together with 5-FU, significantly reduced cell viability to approximately 50% at 48 h and to 50–60% at both 24 and 48 h, respectively ( and ).
EcN SNs at a concentration range of 0.0001 μg/mL to 100 μg/mL, together with 5-FU, tended to decrease IEC-6 cell viability to approximately 80% at 24 h and 60% at 48 h, consistent with 5-FU control (–). However, LB+ (100 μg/mL) together with 5-FU significantly increased cell viability to 95% at 24 h, compared with 5-FU control (85%) () and 73% at 48 h, compared with 5-FU control (61%) (P < 0.05) (). Moreover, M17+ (0.01 μg/mL) together with 5-FU, significantly increased cell viability to 95%, compared with 5-FU control (85%) at 24h () (P < 0.05). Concentrations of M17+, ranging from 0.01 to 100 μg/mL, together with 5-FU, improved viability (92–95%) compared with 5-FU control (85%) at 24 h (). In contrast, MRS+ (100 μg/mL) together with 5-FU significantly reduced cell viability to 70% at 24 h, compared with 5-FU control (85%) (P < 0.05) (). All EcN SNs (1 mg/mL), together with 5-FU at both 24 and 48 h, tended to decrease cell viability compared with 5-FU controls (P < 0.05).
At the 24 h time-point, cell viability was decreased by EcN grown in TSB, when combined with 5-FU, compared with 5-FU controls (P < 0.05) (–). Similarly, EcN grown in MRS also decreased viability at this time-point, compared with 5-FU controls, although similar effects were evident for MRS broth alone. At the 48 h time-point, cell viability was decreased equally by EcN supernatants grown in TSB and MRS when combined with 5-FU, compared with 5-FU control. However, similar effects were obtained for TSB and MRS growth media in the absence of EcN. Only EcN grown in M17 medium decreased viability, when combined with 5-FU, compared with 5-FU controls. This effect was not evident with M17 medium alone (–).
Effects of different EcN SNs and 5-FU on apoptosis and necrosis in IEC-6 cells
Results from and indicated that LB+ and M17+ SNs containing EcN-released factors could potentially prevent cell death induced by 5-FU. This protective effect was not observed when media alone were used. Therefore, we only tested EcN SNs from all growth media.
The percentages of viable, apoptotic, and necrotic IEC-6 cells after treatment with DMEM (control) or 100 μg/mL of EcN (grown in all media), and/or 5-FU, for 24 and 48 h are displayed in and . All EcN SNs, excluding EcN grown in MRS, increased cell viability by up to approximately 95%, compared with DMEM control (86%) (P < 0.05) () when cultured with IEC-6 cells. Very few cells were undergoing early apoptosis (~3–4%) and necrosis (~3–5%) following treatment with DMEM and all EcN SNs. There were no significant differences among treatments at 24 h (). Accordingly, EcN SNs significantly reduced the proportion of late apoptotic/dead cells to approximately 2% compared with 6% for the DMEM control at 24 h (P < 0.05). At 48 h, all EcN SNs enhanced cell viability to ~84% compared with the DMEM control (79%). All EcN SNs produced similar proportions of early apoptotic cells (6%) and necrotic cells (4%) compared with the DMEM controls at 48 h. Importantly, all EcN SNs decreased the proportion of late apoptotic/dead cells to approximately 6%, compared with DMEM control (10%) after 48 h () (P < 0.05). On the other hand, at 24 h the proportion of viable IEC-6 cells (79%) in 5-FU treated 5-FU controls decreased, while the proportion of apoptotic cells (6%) and late apoptotic cells (10%) increased, compared with DMEM controls (). This became more apparent at 48 h, with 70% of viable cells and 17% of early apoptotic cells resulting from 5-FU treatment.
Figure 5. Annexin-PI staining of IEC-6 cells by flow cytometry. DMEM-treated IEC-6 cells are shown in (A) for 24 h and (C) for 48 h. 5-FU treated IEC-6 cells are shown in (B) for 24 h and (D) for 48 h. Results are presented by density plots and separated into four quadrants showing viable cells at lower left, early apoptotic at lower right, late apoptotic/dead at upper right and necrotic at upper left. Values are presented as means of two independent experiments.
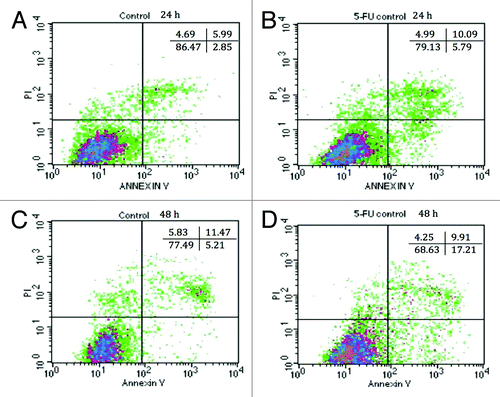
Table 1. Combined effects of EcN SNs and 5-FU (µM) on the percentage of viable (V), apoptotic (A), late apoptotic or dead (LA/D), and necrotic IEC-6 cells at 24 h or 48 h, as measured by flow cytometry
All EcN SNs in combination with 5-FU increased viable cells (83% at 24 h, 75% at 48 h) compared with 5-FU control (79% at 24 h, 70% at 48 h) at both 24 and 48 h (P < 0.05). In contrast, all EcN SNs together with 5-FU produced similar proportions of apoptotic cells (5%) compared with 5-FU control (6%) at 24 h. All EcN SNs together with 5-FU slightly decreased the proportion of early apoptotic cells to 12–17% at 48 h compared with 5-FU control (17%) (P > 0.05). All EcN SNs in the presence of 5-FU slightly decreased the proportion of late apoptotic/dead cells at both 24 and 48 h (6–8% at 24 h, 5–9% at 48 h) compared with their 5-FU controls (10% at both 24 and 48 h) (P > 0.05). Overall the necrotic cell numbers produced by 5-FU treatment were approximately 3–5% at both 24 and 48 h with no significant difference between treatments.
Effects of different EcN SNs and 5-FU on the IEC-6 cell barrier
IEC-6 cells were cultured in transwells for 8 d prior to treatment to allow cells to form a tight cell barrier (). On Day 8, Transepithelial electrical resistance (TER) values (650 Ω/cm2) were not significantly different compared with day 1 (600 Ω/cm2) () (P > 0.05). On Day 8, cells were treated with DMEM, EcN SNs (100 μg/mL), 5-FU alone, or EcN SNs and 5-FU, respectively. TER values 24, 48, 72, and 96 h after adding treatments are shown in . No significant changes were observed between all EcN SNs (674 to 706 Ω/cm2) compared with DMEM controls at 24 and 48 h (). TER values of EcN SN groups decreased slightly at 72 and 96 h, ranging from 627 to 685 Ω/cm2, compared with values at 24 and 48 h (). However, the TER value of the DMEM treatment decreased to 576 Ω/cm2 at 96 h () (P < 0.05).
Figure 6. Transepithelial resistance readings (Ω/cm2) of IEC-6 cells over 8 d. IEC-6 cells were cultured with DMEM supplemented with 10% FBS for 8 d. Media was changed every 2–3 d. Measurement was conducted every 2–3 d prior to media change.
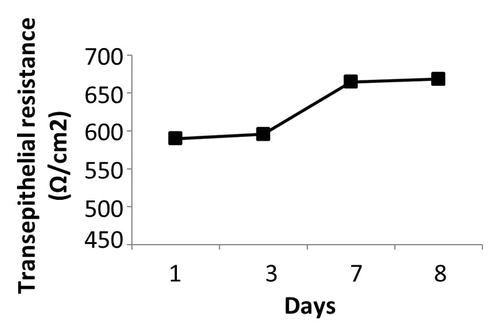
Figure 7. Combined effects of EcN SNs and 5-FU (µM) on the transepithelial resistance (Ω/cm2) of IEC-6 cells for 24 h (A), 48 h (B), 72 h (C), and 96 h (D). Cells were treated with DMEM, or dried EcN SNs (LB+, TSB+, MRS+, or M17+) re-suspended in DMEM to reach final concentration of 100 µg/mL, either alone or in combination with 5-FU (5 µM). TER values are expressed as means ± SEM of three independent experiments (n = 6). Bar data not sharing the same letter are significantly different (P < 0.05). *Indicates a significant difference compared with 5-FU control in all 5-FU treatment groups (P < 0.05).
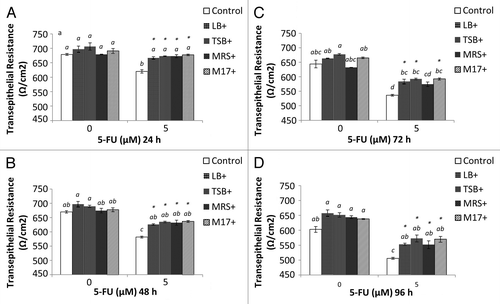
In contrast, 5-FU administration significantly decreased TER value (24 h, 619 Ω/cm2; 96 h, 506 Ω/cm2) compared with DMEM control (678 and 603 Ω/cm2; P < 0.05). Importantly, the TER values of all EcN SNs (excluding MRS+ at 72 h) and 5-FU significantly prevented 5-FU-induced disruption of the cell monolayer barrier at all time-points measured () (P < 0.05).
Protein contents of broths and ECN SNs
BSA equivalent protein contents in broths and EcN SNs are shown in . All media and EcN SNs had protein levels ranging from 2 to 76 μg/mL (). EcN SNs (LB+, TSB+, and M17+) possessed significantly higher protein contents compared with their corresponding growth media alone, indicating that at least some of the factors released from EcN were protein in nature. However, surprisingly, MRS+ (66 ± 2 μg/mL) comprised slightly lower protein concentration compared with MRS− (77 ± 1 μg/mL).
Figure 8. BSA equivalent protein content in media (LB−, TSB−, MRS−, and M17−) and EcN SNs (LB+, TSB+, MRS+, and M17+) were measured by Bradford assay. Data are expressed as means ± SEM of 3 independent experiment (n = 9). Standard error bars not sharing the same letter are significantly different P < 0.05. *Indicates a significant difference compared with other SNs.
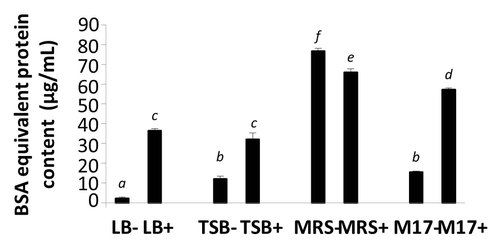
Discussion
Mucositis is one of the most debilitating side effects of cancer chemotherapyCitation20 and to date, there are no satisfactory clinical treatments available. Certain probiotics and probiotic-derived factors have been reported to possess anti-pathogenic and anti-apoptotic properties, combined with a promotion of cell proliferation, suggesting therapeutic utility against mucositis.Citation14,Citation15,Citation21 In the current study, the effects of probiotic-derived factors released from EcN grown in different broths were investigated on cell viability, apoptosis, and disruption of the cell barrier function in IEC-6 cells, in the presence and absence of 5-FU treatment.
The current study shows that none of the growth media and EcN SNs exerted any deleterious side effects on normal intestinal epithelial cells. These results were further confirmed by flow cytometry and TER assay. All EcN SNs produced slightly higher cell resistances compared with DMEM at all time-points measured. This result is consistent with the findings measured by flow cytometry in the current study, where an increase in the percentage of viable cells and a decrease in the late apoptotic/dead cells was observed in most of the EcN SN-treated IEC-6 cells, compared with the DMEM controls. This suggested that EcN SNs could promote cell proliferation and reduce late apoptosis, which may in turn have some impact on the prevention of 5-FU induced cell damage.
The current study was consistent with the findings of Cheah et al., who reported that 5-FU induced 26% of IEC-6 cell death at 24 h, as measured by the neutral red assay.Citation18 In the current study, 5-FU treatment induced a higher proportion of programmed cell death (early and late apoptosis) in IEC-6 cells compared with DMEM controls, as measured by flow cytometry. These results were supported by the findings of Prisciandaro et al. who also revealed that IEC-6 cells treated with 5-FU (5 μM) induced a significant increase in caspase 3 and 7 activation,Citation15 representing an important indicator of apoptosis.Citation22 At the same time, 5-FU caused significant damage to the cell barrier compared with DMEM control.
Media alone (LB, TSB, MRS, and M17) in the presence of 5-FU did not significantly protect IEC-6 cells from 5-FU induced cell death. However, EcN SNs grown in LB and M17, in combination with 5-FU, partially prevented cell death by up to 12%, compared with 5-FU alone. This indicated that EcN-derived factors from LB and M17 may have been the primary bioactive substances to interfere with 5-FU action and inhibit cell toxicity induced by 5-FU. Yan et al. reported that Lactobacillus rhamnosus GG-released factors (p45 and p70) found in SNs that promoted cell proliferation in human and mouse colonic epithelial cells.Citation21 Although untested in the current study, EcN SNs grown in LB and M17 media may have stimulated cell proliferation, which in turn could have reduced IEC-6 cell death associated with 5-FU treatment. Conversely, EcN SNs (100 μg/mL) derived from MRS, together with 5-FU at 24 h, significantly reduced cell viability compared with 5-FU control; whereas this effect was not observed with treatment by MRS medium alone or 5-FU. EcN SN grown in MRS therefore altered the experimental conditions in such a way that cell viability was negatively affected. It is possible that EcN could have released toxic compounds into the MRS broth, which may have resulted in the higher percentage of cell death. Indeed, there is a precedent for this postulate as cytotoxic activity has been described with other probiotic derived factors. For example, a few studies have addressed the anti-pathogenic properties of probiotic-derived factors, with limited reports of bacteriocin, reuterin, and some proteinaceous compounds.Citation23,Citation24 To this end, reuterin, secreted from Lactobacillus reuteri, has been reported to inhibit the growth of a wide range of microorganisms, including gram-negative and gram-positive bacteria, yeasts, molds, and protozoa.Citation11,Citation23
As only EcN SNs grown in LB and M17 showed protective effects against 5-FU-induced cell death as measured by MTT assay, we selected all EcN SNs in combination with 5-FU to further test their effects on apoptosis and the maintenance of cell barrier by flow cytometry and TER assay.
EcN SNs in combination with 5-FU decreased the proportion of late apoptotic/dead cells compared with 5-FU controls; however, EcN SNs combined with 5-FU yielded similar numbers of early apoptotic and necrotic cells, compared with 5-FU controls, as measured by flow cytometry. These results indicated that EcN SNs have the potential to modify the mechanism of late apoptosis and reduce the damage-induced by 5-FU. To date, little is known in regard to the apoptotic effects of probiotic-derived factors. LGG SNs have been reported to reduce TNF-α-induced epithelial cell apoptosis.Citation21 It is still unclear whether the antimicrobial effects of probiotic-derived factors are due to their apoptotic effects. Furthermore, manipulation of COX gene expression by probiotic SNs could indirectly have an impact on apoptosis. Inhibition of COX-2 expression is believed to be an important therapeutic approach in the prevention of gastrointestinal lesions and colon carcinogenesis.Citation25,Citation26 In human studies, it has been reported that EcN SNs, as well as other metabolic factors derived from the probiotic formulation VSL#3, significantly decreased COX-2 gene expression induced by gastrin or the proinflammatory cytokine TNF-α.Citation25 However, whether COX gene expression, and certain specific probiotic-derived substances have any impact on chemotherapy-induced apoptosis of both healthy and transformed cells requires further investigation.
Breaches to the intestinal barrier, accompanied by increases in intestinal permeability in cancer patients undergoing chemotherapy, are an important component of mucositis pathogenesis.Citation2 It is believed that chemotherapeutic drugs deteriorate the balance between cell proliferation and apoptosis leading to disruption of the gastrointestinal barrier. In the current study, a standard TER assay was performed to investigate the effects of EcN SNs on epithelial resistance of 5-FU challenged IEC-6 cells. IEC-6 cells form a relatively lower cell barrier resistance (approximately 700 Ω/cm2) compared with other large intestinal cell lines, such as Caco-2 cells. Indeed, colon cancer Caco-2 cells form a barrier resistance of 2000 Ω/cm2 after 20 d of culture (data not shown).
The TER value of IEC-6 cells cultured in DMEM supplemented with 10% FCS for up to 20 d, was not significantly altered compared with the initial TER value measured on day 1 (data not shown). Therefore, we cultured IEC-6 cells in a transwell for 7 d to give sufficient time for IEC-6 cells to form a healthy barrier. This is also consistent with the studies by Manoj et al., who cultured IEC-6 cells for 6 d prior to treatment.Citation27
As expected, in our study, 5-FU significantly reduced the TER value compared with DMEM controls. Surprisingly, all EcN SNs in combination with 5-FU prevented 5-FU induced disruption of the cell barrier from 24 to 96 h. This result was also consistent with a previous study of Caco-2 cell monolayer integrity, which reported that EcN SNs restored the disrupted epithelial barrier by decreasing 14C-mannitol permeability on Caco-2 cells.Citation28 Given the effect of EcN SNs on apoptosis and cell proliferation in the current study, it is proposed that the reduced late apoptosis and promoted cell proliferation may have contributed to an overall improvement in epithelial integrity. In addition, EcN has been reported to modulate tight junction molecules and to decrease intestinal permeability in DSS-challenged mice,Citation6 which could partially explain the preventive effects of EcN SNs on 5-FU-challenged IEC-6 cells in the current study.
It has been reported that Lactobacillus rhamnosus GG (LGG), which has been more widely studied compared with EcN-derived factors, prevented cell barrier disruption induced by 5-FU on IEC-6 cells and cell barrier dysfunction induced by alcohol on Caco-2 cells.Citation12,Citation15 More recently, LGG SN has been shown to restore the alcohol-damaged intestinal barrier in mice by reducing ileal claudin-1 mRNA levels, leading to the alteration of tight junction proteins.Citation13 In the same study, LGG SN was reported to modulate mRNA levels of hypoxia-inducible factor (HIF), P-glycoproten (P-gp), and cathelin-related antimicrobial peptide in the ileum of alcohol-challenged mice, which together play an important role in maintaining barrier function.Citation13,Citation29,Citation30 Future studies should investigate the underlying mechanism of specific components derived from EcN in regard to the maintenance of epithelial barrier function.
The current study revealed that total protein content significantly increased in all EcN SNs compared with their medium-only counterparts (LB−, TSB−, and M17−). This confirmed that at least some of the released factors derived from EcN were proteinaceous in nature. Interestingly, when EcN was grown in MRS medium, protein levels were significantly lower than in MRS medium alone. This may have been due to the composition of the MRS broth, which contains many constituents such as meat and yeast extract, glucose, peptone, and other minerals. These constituents may have interfered with the sensitivity of the Bradford assay, resulting in the lower protein content measured in EcN SNs grown in MRS, compared with MRS medium alone. Furthermore, there exists the possibility that EcN grown in MRS broth may have produced a protease, which could have digested proteins in the medium, resulting in the lower protein content in EcN SNs (MRS+). To date, the identity of the secreted factors from EcN is still not clear.
The identification and isolation of specific factors from EcN, and further investigation into their underlying mechanisms on tight junction expression and cell cytotoxicity, could provide a better understanding of their potential to prevent chemotherapy-induced mucositis. Identification of the bioactive constituents in EcN supernatants could have broader implications for the potential treatment of other gastrointestinal disorders characterized by compromised intestinal barrier function, such as inflammatory bowel disease and the spectrum of infective enteropathies.
Materials and Methods
EcN supernatant (SN) preparation
The method for preparing bacterial SNs has previously been described by Prisciandaro et al.Citation15 Briefly, E. coli Nissle 1917 (EcN) was purchased from Ardeypharm. EcN (25 ×107 colony forming units) was cultured separately in four different broth growth media: Luria Bertani (LB) (Gibco®, Life Technologies), tryptone soya (TSB), de Man Rogosa Sharpe (MRS), and M17 broth supplemented with 10% (v/v) lactose solution (Oxoid). EcN was incubated at 37 °C for 24 h and reached a concentration of approximately 109 CFU/mL. EcN supernatants were then centrifuged at 6000 rpm for 20 min. SN were then collected and buffered with HEPE to a final concentration of 20 mM (pH 7.2). SNs were then filtered through a 0.22 μm filter and stored at −20 °C.SN aliquots (0.5 mL) was transferred to a 1.5 mL eppendorf tube and the total moisture content was removed by using a Speedvac (Servant Speedvac SC110) for at least 24 h. Dried SNs were then weighed and suspended in serum free Dulbecco’s modified Eagle medium (DMEM) to reach final concentrations of 0.0001, 0.01, 1, 100 µg/mL, and 1 mg/mL. All SNs were re-filtered through a 0.22 μm filter prior to use. All growth media was prepared the same way as EcN SNs without previously growing EcN. The resultant treatment groups were as follows: five broths only including (1) DMEM (control) (1) LB (LB−), (2) TSB (TSB−), (3) MRS (MRS−), and (4) M17 (M17−). Symbol “−” represents broth only; four EcN SNs such as (6) LB (+), (7) TSB (+), (8) MRS (+), and (9) M17 (+). Symbol “+” indicates that each broth had previously grown EcN.
Cell culture
IEC-6 rat intestinal epithelial cells (passage 17–20) were cultured in medium containing DMEM supplemented with 10% (v/v) Fetal calf serum (FCS) (Gibco®, Life Technologies) and 1% (v/v) antibiotics (penicillin, gentamicin, and streptomycin, Gibco®, Life Technologies), respectively. All cells were maintained at 37 °C in a humidified incubator with 5% CO2 and grown in 75 cm2 vented tissue culture flasks (CELLSTAR®, Greiner Bio-One). Culture medium was changed twice weekly and passaged when it had reached 80% confluence.
Cell viability
IEC-6 cell viability was examined by MTT assay.Citation31,Citation32 Cells (1 × 105 cells/mL; 100 uL) were placed in each well of a 96-well tissue culture plate for 24 h for attachment to the bottom of the well. After 24 h, the culture medium was replaced with serum free DMEM comprising different concentrations of broths and SNs (µg/mL) and/or 5-FU (μM). The final concentrations of broth and SNs were 0.0001, 0.01, 1, 100 µg/mL, and 1 mg/mL. The final concentration of 5-FU was 1.25 μM. The cells were then further cultured for either 24 or 48 h. MTT powder (3-[4,5-dimethylthiazol-2-yl]-2,5-diphenyltetrazoliumbromide; Molecular Probes®, Life Technologies) was dissolved in Dulbecco’s phosphate-buffered saline (DPBS) (Gibco®, Life Technologies) to reach a final concentration of 1 mg/mL. The MTT solution (50 μL) was then sterilized by a 0.22 μm filter and added to each well. After the cells were incubated at 37 °C for 4 h, the medium was then replaced with 100 μL of dimethyl sulfoxide (DMSO) (Sigma-Aldrich) for 15 min with minor shaking. Absorbance was read at 570 nm by a spectrophotometer (Tecan infinite 200® PRO). Data were expressed as number of viable cells compared with the percentage of control cells treated with serum free DMEM.Citation32 Treatments were performed in three replicates, while the entire experiment was repeated three times (n = 9).
Detection of apoptotic, live, and dead cells by Annexin V/PI staining through flow cytometry
In order to determine whether the cell death induced by 5-FU was due to apoptosis or necrosis, and whether EcN SNs could have any effect on 5-FU-induced cell death in IEC-6 cells, a standard apoptosis assay was performed. This assay used an Annexin V/Dead cell apoptosis kit with Alexa® Fluor 488 annexin V and propidium iodide (PI) for flow cytometry (V13241, Invitrogen). This assay detects and differentiates the following four groups of cells: (1) early apoptotic cells (with only a green Annexin V stain); (2) necrotic cells (with only a PI stain); (3) late apoptotic/dead cells (with both positive stains); and (4) viable cells (with little or no stain).Citation27,Citation33,Citation34 IEC-6 cells (7.6 × 104 cells/mL) were plated in 12-well culture plates for 24 h to allow attachment. EcN SNs including LB+, TSB+, MRS+, and M17+ at a final concentration of 100 µg/mL and/or 5-FU at a final concentration of 5 µM were used to replace DMEM for a further 24 or 48 h. Cell preparation was performed according to the protocol recommended by the manufacturer (V13241, Invitrogen) with minor adjustments based on other relevant publications.Citation27,Citation33,Citation34 Briefly, after 24 or 48 h, all media were aspirated and cells harvested by trypsinization. Cells were then washed with 3 mL of DPBS and centrifuged at 1000 rpm for 5 min at room temperature (RT). Supernatants were then discarded and cell pellets were re-suspended with 1 × Annexin binding buffer (100 μL). Then, Annexin V (5 μL) and PI (1 μL; 100 μg/mL) were immediately added to the prepared cell suspension. Cell suspension was incubated in the dark for 15 min at RT and followed by addition of 1× Annexin binding buffer (400 μL) before analysis by flow cytometry (FACSCalibur, Becton Dickinson Biosciences). Results were presented by density plot and separated into four quadrants showing viable cells at lower left (both Annexin V- and PI-negative), early apoptotic at lower right (Annexin V-positive only), late apoptotic/dead (upper right) (both Annexin V- and PI-positive), and necrotic at upper left (PI-positive only). Quadrant statistics were analyzed by BD CellQuest ProTM software © 2002.
Transepithelial electrical resistance (TER) assay
IEC-6 cells were harvested from a 75 cm2 vented tissue culture flask. Cell suspension (200 μL) was added to the upper layer of 0.33 cm2 Transwell clear polyester permeable membranes (Corning Costar) at a density of 8 × 104 cells/well. Next, 600 μL of DMEM supplemented with 10% FCS and 1% antibodies (penicillin, gentamicin, and streptomycin, Gibco®, Life Technologies) were added to the bottom well. Cells were incubated at 37 °C in a humidified incubator with 5% CO2 for the duration of the study. Cells were cultured with DMEM supplemented with 10% FCS and 1% antibodies for the first seven days to allow sufficient time to form a monolayer barrier. Media were changed every two to three days prior to the beginning of the experiment. On day 8, for control treatment groups, media were replaced with DMEM control, LB+, TSB+, MRS+, or M17+ at a concentration of 100 μg/mL on the upper layer of the transwell and DMEM at the lower layer for all control groups. For 5-FU treatment groups, all media were replaced with DMEM control, or LB+, TSB+, MRS+, or M17+ at a concentration of 100 μg/mL on the upper layer of the transwell and 5-FU (5 μM) at the lower layer for all 5-FU treated groups. TER measurements were recorded prior to changes of media for the first 8 d and 24, 48, 72, and 96 h after the addition of treatments. Treatments were performed in duplicate and repeated three times for the entire experiment (n = 6). Monolayer resistance was measured by a millicell-ERS volt–ohm meter (Millipore) with electrodes. Values were expressed as ohms per square centimeter (Ω/cm2).Citation15
Bradford protein assay
Coomassie brilliant blue G-250 under acidic conditions binds to protein which is converted from its red form into a blue dye. The colorimetric protein assay was adapted from published methods and measured through absorption spectrum at 590 and 450 nm.Citation35,Citation36 Coomassie stock solution was prepared from 0.01% (w/v) Coomassie brilliant blue G-250, 4.7% (w/v) ethanol, and 8.5% (w/v) phosphoric acid. Bovine serum albumin (BSA) standards (2 mg/mL, Sigma-Aldrich) were diluted with milli-Q water to reach final concentrations of 20, 40, 80, 120, 160, and 200 µg/mL to generate a BSA standard curve.
Coomassie stock solution was added to either BSA standard or a SN into each eppendorf tube at a ratio of Coomassie Brillant Blue G-250 to samples of 9:1 (v/v). Samples including LB−/+, TSB−/+, MRS−/+, M17−/+, and DF−/+ were analyzed in their original liquefied form (not SpeedVac dried). All Coomassie and sample mixtures were mixed thoroughly and 100 μL was added to each well of a 96-well plate. Absorbance was measured immediately at 590 and 450 nm. The y axis of the BSA standard curve was the density of absorbance at 590 nm divided by absorbance at 450 nm (A590/A450). A linear equation was generated based on the plot and BSA equivalent protein content in a sample was calculated based on the equation. Treatments were performed in three replicates while the entire experiment was repeated three times (n = 9).
Statistical analysis
All data are expressed as mean ± standard error (SEM) and statistical analyses were performed using IBM SPSS Statistics for Windows, version 20.0 (SPSS Inc.). One-way ANOVA with a Tukey post hoc test was performed to determine the difference between means, with statistical significance set at P < 0.05.
Disclosure of Potential Conflicts of Interest
No potential conflicts of interest were disclosed.
Acknowledgments
The authors would like to thank Anne Chua for assistance with the TER assay, Gregory Valentine, Michael Papadimitrious, and Joseph Webster for assistance with flow cytometry, Dr Paul Grbin for access to the flow cytometer, and Yuanyuan Wang for assistance with the Bradford assay. The authors would also like to thank Dr David Stone, Anna and Scoresby Shepherd for their assistance in proofreading this manuscript. G.S.H. is supported by a South Australian Cancer Research Collaborative Senior Research Fellowship.
References
- Keefe DM, Schubert MM, Elting LS, Sonis ST, Epstein JB, Raber-Durlacher JE, Migliorati CA, McGuire DB, Hutchins RD, Peterson DE, Mucositis Study Section of the Multinational Association of Supportive Care in Cancer and the International Society for Oral Oncology. Updated clinical practice guidelines for the prevention and treatment of mucositis. Cancer 2007; 109:820 - 31; http://dx.doi.org/10.1002/cncr.22484; PMID: 17236223
- Keefe DM, Brealey J, Goland GJ, Cummins AG. Chemotherapy for cancer causes apoptosis that precedes hypoplasia in crypts of the small intestine in humans. Gut 2000; 47:632 - 7; http://dx.doi.org/10.1136/gut.47.5.632; PMID: 11034578
- Sonis ST. Mucositis as a biological process: a new hypothesis for the development of chemotherapy-induced stomatotoxicity. Oral Oncol 1998; 34:39 - 43; http://dx.doi.org/10.1016/S1368-8375(97)00053-5; PMID: 9659518
- Matthes H, Krummenerl T, Giensch M, Wolff C, Schulze J. Clinical trial: probiotic treatment of acute distal ulcerative colitis with rectally administered Escherichia coli Nissle 1917 (EcN). BMC Complement Altern Med 2010; 10:13; http://dx.doi.org/10.1186/1472-6882-10-13; PMID: 20398311
- Dylag K, Hubalewska-Mazgaj M, Surmiak M, Szmyd J, Brzozowski T. Probiotics in the mechanism of protection against gut inflammation and therapy of gastrointestinal disorders. Curr Pharm Des 2013; PMID: 23755726
- Ukena SN, Singh A, Dringenberg U, Engelhardt R, Seidler U, Hansen W, Bleich A, Bruder D, Franzke A, Rogler G, et al. Probiotic Escherichia coli Nissle 1917 inhibits leaky gut by enhancing mucosal integrity. PLoS One 2007; 2:e1308; http://dx.doi.org/10.1371/journal.pone.0001308; PMID: 18074031
- Becker S, Oelschlaeger TA, Wullaert A, Vlantis K, Pasparakis M, Wehkamp J, Stange EF, Gersemann M. Bacteria regulate intestinal epithelial cell differentiation factors both in vitro and in vivo. PLoS One 2013; 8:e55620; http://dx.doi.org/10.1371/journal.pone.0055620; PMID: 23418447
- Rund SA, Rohde H, Sonnenborn U, Oelschlaeger TA. Antagonistic effects of probiotic Escherichia coli Nissle 1917 on EHEC strains of serotype O104:H4 and O157:H7. Int J Med Microbiol 2013; 303:1 - 8; http://dx.doi.org/10.1016/j.ijmm.2012.11.006; PMID: 23312798
- Huebner C, Ding Y, Petermann I, Knapp C, Ferguson LR. The probiotic Escherichia coli Nissle 1917 reduces pathogen invasion and modulates cytokine expression in Caco-2 cells infected with Crohn’s disease-associated E. coli LF82. Appl Environ Microbiol 2011; 77:2541 - 4; http://dx.doi.org/10.1128/AEM.01601-10; PMID: 21317252
- Troge A, Scheppach W, Schroeder BO, Rund SA, Heuner K, Wehkamp J, Stange EF, Oelschlaeger TA. More than a marine propeller--the flagellum of the probiotic Escherichia coli strain Nissle 1917 is the major adhesin mediating binding to human mucus. Int J Med Microbiol 2012; 302:304 - 14; http://dx.doi.org/10.1016/j.ijmm.2012.09.004; PMID: 23131416
- Jones SE, Versalovic J. Probiotic Lactobacillus reuteri biofilms produce antimicrobial and anti-inflammatory factors. BMC Microbiol 2009; 9:35; http://dx.doi.org/10.1186/1471-2180-9-35; PMID: 19210794
- Wang Y, Kirpich I, Liu Y, Ma Z, Barve S, McClain CJ, Feng W. Lactobacillus rhamnosus GG treatment potentiates intestinal hypoxia-inducible factor, promotes intestinal integrity and ameliorates alcohol-induced liver injury. Am J Pathol 2011; 179:2866 - 75; http://dx.doi.org/10.1016/j.ajpath.2011.08.039; PMID: 22093263
- Wang Y, Liu Y, Sidhu A, Ma Z, McClain C, Feng W. Lactobacillus rhamnosus GG culture supernatant ameliorates acute alcohol-induced intestinal permeability and liver injury. Am J Physiol Gastrointest Liver Physiol 2012; 303:G32 - 41; http://dx.doi.org/10.1152/ajpgi.00024.2012; PMID: 22538402
- Prisciandaro LD, Geier MS, Butler RN, Cummins AG, Howarth GS. Probiotic factors partially improve parameters of 5-fluorouracil-induced intestinal mucositis in rats. Cancer Biol Ther 2011; 11:671 - 7; http://dx.doi.org/10.4161/cbt.11.7.14896; PMID: 21307648
- Prisciandaro LD, Geier MS, Chua AE, Butler RN, Cummins AG, Sander GR, Howarth GS. Probiotic factors partially prevent changes to caspases 3 and 7 activation and transepithelial electrical resistance in a model of 5-fluorouracil-induced epithelial cell damage. Support Care Cancer 2012; 20:3205 - 10; http://dx.doi.org/10.1007/s00520-012-1446-3; PMID: 22526145
- Bär F, Von Koschitzky H, Roblick U, Bruch HP, Schulze L, Sonnenborn U, Böttner M, Wedel T. Cell-free supernatants of Escherichia coli Nissle 1917 modulate human colonic motility: evidence from an in vitro organ bath study. Neurogastroenterol Motil 2009; 21:559 - 66, e16-7; http://dx.doi.org/10.1111/j.1365-2982.2008.01258.x; PMID: 19220758
- Derzelle S, Bolotin A, Mistou MY, Rul F. Proteome analysis of Streptococcus thermophilus grown in milk reveals pyruvate formate-lyase as the major upregulated protein. Appl Environ Microbiol 2005; 71:8597 - 605; http://dx.doi.org/10.1128/AEM.71.12.8597-8605.2005; PMID: 16332852
- Cheah KY, Howarth GS, Yazbeck R, Wright TH, Whitford EJ, Payne C, Butler RN, Bastian SE. Grape seed extract protects IEC-6 cells from chemotherapy-induced cytotoxicity and improves parameters of small intestinal mucositis in rats with experimentally-induced mucositis. Cancer Biol Ther 2009; 8:382 - 90; http://dx.doi.org/10.4161/cbt.8.4.7453; PMID: 19305141
- Horie T, Li T, Ito K, Sumi S, Fuwa T. Aged garlic extract protects against methotrexate-induced apoptotic cell injury of IEC-6 cells. J Nutr 2006; 136:Suppl 861S - 3S; PMID: 16484581
- Duncan M, Grant G. Oral and intestinal mucositis - causes and possible treatments. Aliment Pharmacol Ther 2003; 18:853 - 74; http://dx.doi.org/10.1046/j.1365-2036.2003.01784.x; PMID: 14616150
- Yan F, Cao H, Cover TL, Whitehead R, Washington MK, Polk DB. Soluble proteins produced by probiotic bacteria regulate intestinal epithelial cell survival and growth. Gastroenterology 2007; 132:562 - 75; http://dx.doi.org/10.1053/j.gastro.2006.11.022; PMID: 17258729
- Ashkenazi A, Dixit VM. Death receptors: signaling and modulation. Science 1998; 281:1305 - 8; http://dx.doi.org/10.1126/science.281.5381.1305; PMID: 9721089
- Talarico TL, Dobrogosz WJ. Chemical characterization of an antimicrobial substance produced by Lactobacillus reuteri.. Antimicrob Agents Chemother 1989; 33:674 - 9; http://dx.doi.org/10.1128/AAC.33.5.674; PMID: 2751282
- Howarth GS, Wang H. Role of endogenous microbiota, probiotics and their biological products in human health. Nutrients 2013; 5:58 - 81; http://dx.doi.org/10.3390/nu5010058; PMID: 23306189
- Otte JM, Mahjurian-Namari R, Brand S, Werner I, Schmidt WE, Schmitz F. Probiotics regulate the expression of COX-2 in intestinal epithelial cells. Nutr Cancer 2009; 61:103 - 13; http://dx.doi.org/10.1080/01635580802372625; PMID: 19116880
- Nurmi JT, Puolakkainen PA, Rautonen NE. Bifidobacterium Lactis sp. 420 up-regulates cyclooxygenase (Cox)-1 and down-regulates Cox-2 gene expression in a Caco-2 cell culture model. Nutr Cancer 2005; 51:83 - 92; http://dx.doi.org/10.1207/s15327914nc5101_12; PMID: 15749634
- Puthia MK, Sio SW, Lu J, Tan KS. Blastocystis ratti induces contact-independent apoptosis, F-actin rearrangement, and barrier function disruption in IEC-6 cells. Infect Immun 2006; 74:4114 - 23; http://dx.doi.org/10.1128/IAI.00328-06; PMID: 16790785
- Stetinova V, Smetanova L, Kvetina J, Svoboda Z, Zidek Z, Tlaskalova-Hogenova H. Caco-2 cell monolayer integrity and effect of probiotic Escherichia coli Nissle 1917 components. Neuro Endocrinol Lett 2010; 31:Suppl 2 51 - 6; PMID: 21187838
- Wang GL, Jiang BH, Rue EA, Semenza GL. Hypoxia-inducible factor 1 is a basic-helix-loop-helix-PAS heterodimer regulated by cellular O2 tension. Proc Natl Acad Sci U S A 1995; 92:5510 - 4; http://dx.doi.org/10.1073/pnas.92.12.5510; PMID: 7539918
- Colgan SP, Taylor CT. Hypoxia: an alarm signal during intestinal inflammation. Nat Rev Gastroenterol Hepatol 2010; 7:281 - 7; http://dx.doi.org/10.1038/nrgastro.2010.39; PMID: 20368740
- Huynh-Delerme C, Huet H, Noël L, Frigieri A, Kolf-Clauw M. Increased functional expression of P-glycoprotein in Caco-2 TC7 cells exposed long-term to cadmium. Toxicol In Vitro 2005; 19:439 - 47; http://dx.doi.org/10.1016/j.tiv.2004.08.003; PMID: 15826803
- Cheah KY. Grape Sourced Bioactives: A Potential New Treatment Strategy for Intestinal Mucositis and Colon Cancer. University of Adelaide. Adelaide, Australia, 2012.
- Vermes I, Haanen C, Steffens-Nakken H, Reutelingsperger C. A novel assay for apoptosis. Flow cytometric detection of phosphatidylserine expression on early apoptotic cells using fluorescein labelled Annexin V. J Immunol Methods 1995; 184:39 - 51; http://dx.doi.org/10.1016/0022-1759(95)00072-I; PMID: 7622868
- Wlodkowic D, Skommer J, Darzynkiewicz Z. Flow cytometry-based apoptosis detection. Methods Mol Biol 2009; 559:19 - 32; http://dx.doi.org/10.1007/978-1-60327-017-5_2; PMID: 19609746
- Bradford MM. A rapid and sensitive method for the quantitation of microgram quantities of protein utilizing the principle of protein-dye binding. Anal Biochem 1976; 72:248 - 54; http://dx.doi.org/10.1016/0003-2697(76)90527-3; PMID: 942051
- Zor T, Selinger Z. Linearization of the Bradford protein assay increases its sensitivity: theoretical and experimental studies. Anal Biochem 1996; 236:302 - 8; http://dx.doi.org/10.1006/abio.1996.0171; PMID: 8660509