Abstract
Scar formation inhibits tissue repair and regeneration in the liver and central nervous system. Activation of hepatic stellate cells (HSCs) after liver injury or of astrocytes after nervous system damage is considered to drive scar formation. HSCs are the fibrotic cells of the liver, as they undergo activation and acquire fibrogenic properties after liver injury. HSC activation has been compared to reactive gliosis of astrocytes, which acquire a reactive phenotype and contribute to scar formation after nervous system injury, much like HSCs after liver injury. It is intriguing that a wide range of neuroglia-related molecules are expressed by HSCs. We identified an unexpected role for the p75 neurotrophin receptor in regulating HSC activation and liver repair. Here we discuss the molecular mechanisms that regulate HSC activation and reactive gliosis and their contributions to scar formation and tissue repair. Juxtaposing key mechanistic and functional similarities in HSC and astrocyte activation might provide novel insight into liver regeneration and nervous system repair.
Introduction
After injury, tissue repair is inhibited by cells that, upon activation from a quiescent state, deposit growth factors and cytokines that control cell survival in an autocrine and paracrine manner. Understanding the molecular mechanisms that govern the proliferation and activation of cells that regulate scar formation can provide insights into tissue repair and regeneration. Hepatic stellate cells (HSCs) regulate tissue repair after injury, while astrocytes do so after nervous system injury. HSCs and astrocytes share striking morphological and functional similarities. They both have spindled star-like shapes, as reflected in their names, which are derived from the Latin (stella) and Greek (astron) words for “star.” Like astrocytes, HSCs express glial fibrillary acidic protein (GFAP), a cytoskeletal protein well known for its role in astrocyte gliosisCitation1 (). Interestingly, HSCs and astrocytes are perivascular cells; both are intimately associated with the vasculature and help regulate the blood-tissue barrier, which can be critical in tissue homeostasis and reaction to injury.Citation2
HSCs fulfill a critical and dynamic function in regulating the liver's response to injury. In a normal healthy liver, HSCs are quiescent, a state in which they serve primarily to store vitamin A. In response to liver injury or disease, HSCs differentiate into myofibroblast-like cells and undergo changes in gene expression, morphology (e.g., increased size), proliferation and extracellular matrix (ECM) production.Citation3 Activated HSC myofibroblasts are characterized by the presence of specific cytoskeletal stress fibers, including α-smooth muscle actin, and by upregulated expression and secretion of profibrogenic factors such as transforming growth factorβ (TGFβ), ECM proteins such as collagens I and III, the ECM-remodeling enzymes matrix metalloproteinases and tissue inhibitors of matrix metalloproteinases.Citation4,Citation5
Astrocytes are glial cells found in gray and white matter in the brain and spinal cord. Their finely branching processes envelope all cellular components throughout the CNS to maintain nervous system functions. After CNS injury or disease, astrocytes become reactive, leading to cellular hypertrophy, upregulation of the intermediate filament GFAP (), changes in gene expression, some proliferation and inhibitory ECM production, leading to so-called reactive astrogliosis.Citation6–Citation8 Beside GFAP, activated astrocytes show enhanced expression of vimentin and re-expression of nestin, all of which contribute to formation of the intermediate filament network. Activated astrocytes secrete cytokines, such as interleukin (IL) 1 and IL-6; growth factors, such as TGFβ; ciliary neurotrophic factor and nerve growth factor (NGF); and ECM proteins, such as laminin and chondroitin sulfate proteoglycans (CSPGs).Citation8–Citation11
HSCs and astrocytes have both protective and damaging functions in tissue repair (). Activated HSCs and astrocytes can promote inflammation and cytokine release. HSCs secrete a scar-forming matrix that inhibits hepatocyte proliferation and liver regeneration,Citation5,Citation12,Citation13 and the scar-forming matrix released by astrocytes inhibits axonal regeneration.Citation6 Both HSC activation and astrocyte reactivity appear to be necessary for and contribute to tissue repair.Citation14–Citation18 Despite such striking similarities, HSC activation and reactive astrogliosis are not completely analogous. In this commentary, we highlight the molecular mechanisms that regulate the activation of HSCs and astrocytes and their effects on inhibitory or repair-promoting cross-talk with hepatocytes and neurons.
Molecular Mediators of Scar Formation Fibrosis in the Liver vs. Reactive Gliosis in the Nervous System
Scar formation is characterized by matrix deposition and localized inflammation. In the liver, fibrotic scarring is created by excess collagen secreted by activated HSCs. The dense collagen meshwork of the scar presents a barrier to hepatocyte growth and proliferation and inhibits liver regeneration after injury. In the CNS, scarring after traumatic injury is carried out by reactive astrocytes, which secrete a CSPG matrix that can persist for months.Citation7 CSPGs, along with myelin-based growth inhibitors, inhibit axonal growth and nerve regeneration after CNS injury.
A crucial question in both liver fibrosis and reactive gliosis is what causes HSCs to become activated and astrocytes to become reactive and initiate scar formation. Finding the molecular “scar inducers” that activate HSCs and astrocytes is important for identifying therapeutic targets for liver and nervous system injuries. One common major profibrotic factor that promotes HSC and astrocyte activation and scar formation is TGFβ.Citation19 In the liver, activation of HSCs by TGFβ stimulates these cells to produce and secrete collagen matrix. In activated HSCs, TGFβ expression increases, which initiates an autocrine signaling loop that perpetuates HSC activation. TGFβ secreted by activated HSCs stops hepatocyte proliferation, another barrier to liver regeneration.Citation13 The importance of TGFβ in HSC activation has been confirmed in vivo, as TGFβ1-null mice show minimal collagen expression in the liver after acute injury.Citation20 In HSCs and astrocytes, the major signaling molecule downstream of TGFβ is the transcription factor Smad3. In an in vivo model of acute liver injury and in vitro in culture-activated HSCs, Smad3 signaling is not necessary for HSC activation, as assessed by alpha-smooth muscle actin expression; however, it is essential for maximal expression of collagen type I in activated HSCs and for inhibition of HSC proliferation.Citation21,Citation22 In Smad3-null mice, scar formation is reduced after cortical stab wound injury. Thus, TGFβ signaling through Smad3 in astrocytes may contribute to scar formation after brain injury.Citation23
In the CNS, TGFβ expression increases in astrocytes after injury and localizes in areas of gliotic scar formation.Citation7,Citation24 Accordingly, astroglial overexpression of TGFβ1 strongly upregulates ECM proteins in transgenic miceCitation25 and increases CSPG expression by astrocytes in vitro.Citation26 Neutralization of TGFβ activity with antibodies against TGFβ1 and TGFβ2 reduces gliotic scarring after brain injury in vivo.Citation27 CSPGs are upregulated by reactive astrocytes soon after CNS injury, such as spinal cord injury.Citation7 Although vascular leakage had been associated with scar formation, the vascular derived signal that regulates TGFβ signaling and CSPG upregulation was unknown. We showed that the blood protein fibrinogen, which leaks into the CNS immediately after blood-brain barrier disruption or vascular damage,Citation28,Citation29 serves as an early signal for the induction of glial scar formation via the TGFβ/Smad signaling pathway.Citation30 Fibrinogen is a carrier of latent TGFβ and induces phosphorylation of Smad2 in astrocytes that leads to inhibition of neurite outgrowth. After cortical injury, mice genetically or pharmacologically depleted of fibrinogen have much lower levels of active TGFβ, reduced astrocytosis and less deposition of the CSPG neurocan. Therefore, fibrinogen initiates scar formation in the CNS by regulating the bioavailability of active TGFβ at sites of vascular damage.Citation30 In the CNS, fibrin is deposited early before the secretion of CSPGs.Citation30 Similarly, in the liver fibrin deposition precedes collagen secretion after injury. Moreover, impaired fibrin clearance results in persistent activation of HSCs.Citation31 It is therefore possible that fibrinogen might be regulating the bioavailability of active TGFβ and thus collagen deposition after liver damage.
Beside TGFβ, other inducers of scar formation in the liver and CNS after injury include IL-1, IL-6, interferon γ and fibroblast growth factor 2. In HSCs, IL-6 promotes collagen secretion,Citation32 while IL-1α may help promote changes in secretion of matrix-remodeling enzymes by activated HSCs.Citation33 Fibroblast growth factor 2 has no effect on HSC activation but may have a role in regulating collagen production.Citation34 IL-1β is upregulated immediately after CNS injury and in turn upregulates GFAP expression in astrocytes,Citation35 a hallmark of the transition to a reactive state. Transgenic expression of IL-6 or tumor necrosis factor α in the CNS can induce spontaneous astrogliosis, which can have both deleterious effects on CNS functionCitation36,Citation37 and beneficial effects after CNS injury.Citation38,Citation39 Administration of interferon γ increases gliotic scarring after traumatic brain injury.Citation40 Fibroblast growth factor 2 is a major regulator of GFAP induction in astrocytes, suggesting that it promotes their transition to a reactive state.Citation41
Other molecular pathways underlying scar formation in the CNS or liver have also been unraveled. Epidermal growth factor (EGF) is a major inducer of scar formation by astrocytes in the CNS and regulates hepatocyte proliferation in the liver. In primary astrocytes, treatment with EGF triggers the regulation of many genes associated with the reactive phenotype.Citation42 In cultured astrocytes, treatment with TGFα, EGF and heparin-binding EGF, all of which are ligands for the EGF receptor, increases CSPG secretion.Citation26,Citation43 The transcription factor Sox9 is involved in astrocyte production of pro- and antiregenerative matrix molecules. Overexpression of Sox9 induces the expression of key enzymes involved in CSPG synthesis but does not increase the expression of laminin, fibronectin or other ECM proteins. Correspondingly, SOX9 knockdown in primary astrocytes reduces expression of key CSPG synthesis enzymes and increases the expression of laminin and fibronectin. Thus, SOX9 levels may be pivotal in determining the balance of pro- and antiregenerative ECM proteins produced by astrocytes.Citation44 The role of SOX9 in liver scar formation has not been investigated.
A further major difference between scar formation in CNS injury versus liver injury is the physical area that the scarring encompasses. In CNS injury, the scar forms around the injury site, with reactive astrocytes surrounding the damaged tissue.Citation6,Citation45 The molecular regulation of the boundary is not understood. This localized scar formation may confine the postinjury inflammatory response to the damaged area, thereby minimizing damage to surrounding healthy tissue. In contrast, fibrotic scarring in the liver is often widespread, as many hepatic diseases and injuries are caused by systemic sources (e.g., viral hepatitis, chronic alcohol consumption) that activate HSCs throughout the liver.
Regulation of Blood-tissue Homeostasis by Astrocytes and HSCs
Activated HSCs remodel the sinusoidal wallCitation46 as well as necrotic areas, suggesting a possible function in blood-tissue homeostasis. Astrocytes fulfill a similar function in inducing and maintaining the blood-brain barrier.Citation47 Local neuronal activity leads to dynamic changes in cerebral blood flow and astrocytes are emerging as a key player in coordinating this neurovascular coupling.Citation48 Interestingly, activated HSCs regulate the contractility of blood vessels and their resistance to blood pressure.Citation49,Citation50 Metallothionein, a functional marker of the blood-brain barrier, is strongly expressed in HSCs during their activation under prolonged culture conditions,Citation2 further suggesting a role for HSCs in blood-tissue homeostasis.
Neuroglial Characteristics of HSCs
HSCs are thought to be fibroblastic mesenchymal cells,Citation51,Citation52 based upon the robust induction of α-smooth muscle actin, matrix molecules and matrix metalloproteinases during their activation.Citation53 However, HSCs also express many neuroglia-related molecules that contribute to their function. HSCs express all four neurotrophins, the TrkB and TrkC receptors, the p75 neurotrophin receptor (p75NTR), neuroglia-related cytoskeletal proteins such as GFAP and nestin, and a variety of molecules and receptors involved in neurotransmission and neuroendocrine signaling (). Additionally, HSCs can respond to several neurotransmitters, including acetylcholine and serotonin () and HSC processes can make synapse-like contacts with nerves.Citation54 Further evidence of the neural features of HSCs comes from in vitro studies with a pharmacologic inhibitor of the hedgehog pathway. Hedgehog, which provides neural survival signals required in developing and adult brain,Citation55 was shown to signal cell activation and viability of HSCs.Citation56 Although the exact functions of these neuroglial molecules in HSCs are not fully known, they have been implicated in functions as diverse as differentiation,Citation17 proliferation,Citation57 apoptosisCitation58 and chemotaxis.Citation59
We have shown that p75NTR regulates HSC activation to myofibroblasts.Citation17 p75NTR is a member of the TNF receptor superfamily of proteins originally studied for its role in neuronal death and survival.Citation60 It is widely expressed throughout the body,Citation61 and its expression can be upregulated by injury or disease, including liver cirrhosis.Citation58,Citation62 p75NTR acts through the small GTPase Rho to promote HSC differentiation into an activated myofibroblast state in a neurotrophin ligand-independent manner.Citation17 Unlike primary HSCs isolated from wildtype mice, HSCs from p75NTR−/− mice showed impaired differentiation into an activated form in culture. This ability was restored by adenoviral delivery of full-length p75NTR or its intracellular domain. p75NTR−/− HSCs also exhibited loss of Rho activation, which was completely restored by adenoviral delivery of constitutively active Rho. Additionally, inhibiting p75NTR-mediated Rho activation with the peptide inhibitor TAT-Pep5 prevented activation of wildtype HSCs—further evidence that a p75NTR/Rho signaling pathway is important in HSC differentiation.
The role of p75NTR/Rho signaling in later stages of liver injury is unknown. Although p75NTR signaling in HSCs contributes to hepatocellular growth after injury, p75NTR may have a dual role in liver injury and repair. Indeed, p75NTR can mediate HSC apoptosis upon stimulation with the neurotrophin ligand NGF.Citation17,Citation58,Citation63 At early stages of liver injury, p75NTR may regulate HSC differentiation through the Rho signaling pathway, and HSCs may secrete hepatocyte growth factor (HGF) to support hepatocyte proliferation. At later stages, however, secretion of pro-NGF or NGF by regenerating hepatocytes may lead to ligand-induced p75NTR-mediated activation of apoptotic pathways in HSCs.Citation64 Thus, p75NTR signaling in HSCs may act in a paracrine manner to regulate liver regeneration after injury. The bioavailability of neurotrophins and the balance between pro- and mature NGF might regulate the biological function of p75NTR in liver repair.
Another molecular mechanism proposed for p75NTR-mediated HSC activationCitation65 involves thyroid hormones implicated in liver cirrhosis. Binding of thyroid hormone receptor α1 to thyroid response elements in the p75NTR promoter induces p75NTR transcription.Citation65 The resulting increased levels of p75NTR activate Rho signaling involved in the transdifferentiation of HSCs to myofibroblasts.Citation65 Interestingly, thyroid hormone regulates several aspects of astrocyte differentiation and maturation, including the production of ECM proteins and growth factors.Citation66 The role of p75NTR in liver regeneration was also confirmed in the chloride tetracarbon model of liver cirrhosis.Citation67 In this study, the involvement of p75NTR in the regenerative response was attributed to regulation of hepatic myofibroblast apoptosis and proliferation by p75NTR.Citation67 Moreover, p75NTR is a marker of mesenchymal progenitors of HSCs at an early stage of liver development.Citation68 p75NTR-positive progenitor cells also express Jagged1, a Notch ligand that is crucial for inducing hepatoblasts to differentiate into cholangiocytes. Thus, p75NTR could participate in the commitment of liver progenitors and the differentiation of liver mesenchymal cells.Citation68
p75NTR/Rho signaling also contributes to neuron growth and nerve regeneration. p75NTR-mediated activation of Rho inhibits the regeneration of injured nerves.Citation69 TAT-Pep5 alleviates this growth inhibition and promotes neurite extension.Citation69 The function of p75NTR in astrocytes is poorly understood. p75NTR mRNA is expressed in primary astrocytes and is further upregulated by treatment with NGF, a p75NTR ligand.Citation70 In astrocyte cultures, activation of p75NTR by NGF attenuates proliferation induced by mitogens such as EGF or serum.Citation71 In astrocytes in the intact adult rat CNS, p75NTR expression is restricted to a small populationCitation72,Citation73 but is induced after different types of injury.Citation71,Citation74,Citation75 p75NTR is strongly upregulated by excitotoxic damage,Citation74 cerebral ischemiaCitation75 and seizures.Citation76
The role of astrocytic p75NTR in these pathological conditions is unknown.Citation77 Regulation of apoptosis has been excluded, as p75NTR expression does not correlate with apoptosis during development or in injury models.Citation74,Citation77,Citation78 However, p75NTR is expressed in cycling cells, suggesting a role in regulating the cell cycle, as cell cycle transitions are necessary for cell differentiation and activation.Citation77 A potential in vivo function of p75NTR in astrocytes has been substantiated by gene expression profiles of highly invasive and noninvasive tumor cell populations isolated from a human malignant glioma cell line after implantation into the brain of immunocompromised mice.Citation79 p75NTR was a critical regulator of glioma invasion through the regulation of RhoA activity and cytoskeletal rearrangement.Citation79 Interestingly, neurotrophin-dependent regulation of intramembrane proteolysis of p75NTR is required for p75NTR-mediated glioma invasion.Citation80 Indeed, p75NTR undergoes intramembrane proteolysis in malignant gliomas, whose highly invasive nature in vivo is markedly impaired by implantation of glioma cells expressing cleavage-resistant chimeric forms of p75NTR or by subcutaneous injection of γ-secretase inhibitors.Citation80 Although the exact role of p75NTR in cancer is poorly understood, p75NTR has been implicated in the metastatic progression of melanomas, and specifically those that metastasize to the brain.Citation81–Citation84
The Dual Roles of HSC Activation and Reactive Astrogliosis in Tissue Repair
Although many studies have documented the damaging roles of HSCs and astrocytes in tissue injury, these cells can also promote tissue repair. In the initial stages after liver injury, HSC activation is critical, as it leads to the generation of a provisional ECM scaffold and release of growth factors, such as HGF, that promote hepatocyte proliferation. HSCs are a major source of HGF.Citation13,Citation85 Moreover, activated HSCs are intimately associated with regenerating hepatocyte clusters through cell-to-cell contacts,Citation86,Citation87 which are believed to promote three-dimensional growth of hepatocytes after injury. In mice that are heterozygous for the transcription factor Foxf1, defective HSC activation impairs liver regeneration after injury.Citation16 Mice that lack p75NTR, which is critical for HSC activation, have exacerbated liver pathology and decreased hepatocyte proliferation in a genetic model of liver injury.Citation17
Like HSCs, reactive astrocytes have also been viewed as a negative component of the inflammatory response to brain injury. Reactive astrocytes secrete TGFβ, which induces scar formation and may contribute to cytotoxic death of neurons and glial cells by releasing nitric oxide.Citation6 However, in numerous studies of cultured neurons, TGFβ afforded protection against various toxins and injurious agents.Citation88,Citation89 In addition, reactive astrocytes support neuronal survival by releasing neurotrophic factors such as NGF and ciliary neurotrophic factor.Citation9,Citation10,Citation90
Reactive astrogliosis can also play a positive role in the response to CNS injury. Selective ablation of reactive astrocytes after spinal cord injury in mice increases neuronal degeneration and the amount and duration of inflammation, suggesting that the gliotic scar compartmentalizes and minimizes inflammation at the injury site.Citation14 These findings highlight a cytoprotective role of astrocytes after injury. In mice, conditional knockout of Stat3, an important intracellular mediator of cytokine-induced astrocyte reactivity, leads to widespread inflammatory cell infiltration, demyelination and limited functional recovery after spinal cord injury,Citation15,Citation91 further suggesting a protective role for reactive astrogliosis. Thus, HSC activation in liver injury and reactive astrogliosis in CNS injury seem to have dual roles. The balance of positive and negative effects of HSCs and astrocytes may be related to the stage and context of injury.
What the Liver and the Nervous System Can Learn from Each Other
Although parallels exist between the injury responses involved in HSC activation and reactive gliosis, the processes of scar formation after liver injury and after CNS injury are not totally analogous. Can we use knowledge about cell activation and scar formation in one organ to infer what may occur after injury in the other? Ideally, one would like to be able to manipulate scar formation to increase the opportunity for healing. In both CNS and hepatic injury, there are two major goals of treatment: to remove the scar using chemical or mechanical inhibitors of growth and to promote tissue regeneration and repair by fostering the growth of the key cell type (i.e., hepatocytes or neurons) that is most important for functional recovery of the tissue.
Combination therapy is a promising approach. In the nervous system, one strategy to promote repair is a neurotrophic factor-producing cell graft in the injured area combined with ways to alleviate the inhibitory effects of myelin-based growth inhibitors and CSPG matrix.Citation92,Citation93 A novel method to remove CSPGs from the gliotic scar was developed in transgenic mice, in which astrocytes were genetically modified to secrete a chondroitinase that digests CSPGs.Citation94 This technique, in conjunction with other therapies, holds promise for the treatment of CNS injury. A similar technique might be used to promote collagen removal from the injured liver; an HSC-specific promoter could be valuable for cell-specific gene therapy. Therapies for liver fibrosis aim at selectively inducing apoptosis of activated HSCs to remove the source of scar-forming matrix. Such therapies may potentially be used in conjunction with HGF to promote hepatocyte proliferation.
Investigators in both the liver field and the nervous system field have explored the de-differentiation of activated/reactive cells. HSC activation can be reversed in vitro;Citation95 however, it is not known whether it can be reversed in vivo. With further research, HSC and astrocyte “deactivation” may prove to be promising therapeutically. It would be especially interesting to see whether the two processes overlap in their molecular mechanisms. The identification of pathways that underlie HSC activation and astrogliosis is giving deep insights into the contribution of HSCs and astrocytes to scar formation after injury and offer a platform for the development of novel therapeutic targets. The parallels between scar formation in the liver and in the nervous system could stimulate researchers in both fields to unravel novel paradigms by looking beyond their tissue of choice.
Abbreviations
HSC | = | hepatic stellate cell |
GFAP | = | glial fibrillary acidic protein |
ECM | = | extracellular matrix |
TGFβ | = | transforming growth factorβ |
CNS | = | central nervous system |
IL | = | interleukin |
NGF | = | nerve growth factor |
CSPG | = | chondroitin sulfate proteoglycan |
EGF | = | epidermal growth factor |
HGF | = | hepatocyte growth factor |
Figures and Tables
Figure 1 Expression of GFAP in HSCs and astrocytes. Differentiation of HSCs into activated myofibroblasts (left) is a hallmark of fibrotic liver disease of different etiologies, such as viral hepatitis and chronic alcohol consumption.Citation96 In chronic liver disease, perpetuation of HSC activation leads to excessive collagen and ECM deposition, resulting in liver fibrosis. Thus, sustained activation of HSCs is a target for the treatment of liver fibrosis.Citation5 Astrocytes (right) become reactive in response to all forms of CNS injury or disease, causing cellular hypertrophy, scar formation and production of inhibitory CSPGs.Citation6,Citation7 CSPG removal from the injured CNS area offers promise for therapies to improve axonal regeneration after CNS injury.Citation94
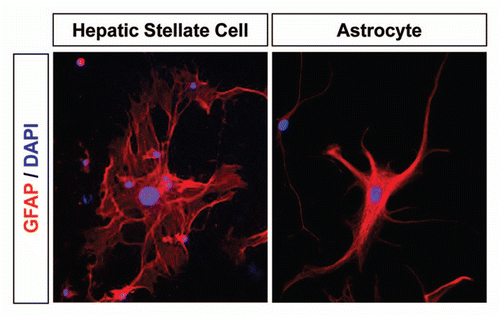
Figure 2 Dual role of HSCs and astrocytes in tissue repair. HSC activation and reactive astrogliosis after tissue injury each lead to deposition of growth factors, cytokines and ECM proteins that control cell survival, proliferation, growth and regeneration in an autocrine and paracrine manner. In both liver and CNS injury there is a dual role for HSC activation and reactive astrogliosis, respectively. The balance of protective and damaging effects of HSCs and astrocytes may be related to the mechanisms that regulate their cross-talk with hepatocytes and neurons, respectively; as well as the stage and context of injury.
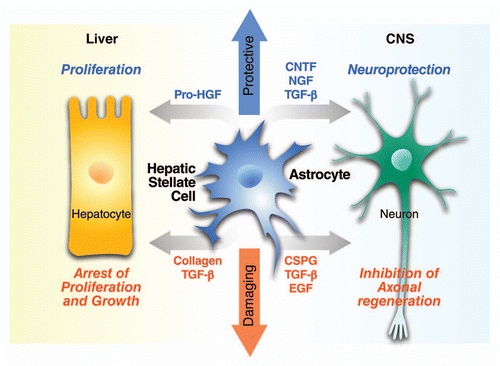
Table 1 Functions of neuroglial-related molecules expressed in HSCs
Acknowledgments
We thank Benjamin D. Sachs for discussions, John Carol for graphics and Stephen Ordway for editorial assistance. Supported by National Institutes of Health/National Institute of Neurological Disorders and Stroke Grants R01NS051470, R01NS52189 and R01NS066361 to K.A., the Pilot/Feasibility grant from the UCSF Liver Center (P30 DK026743) to K.A., the German Research Foundation (DFG) postdoctoral fellowship to C.S., and a National Multiple Sclerosis Society postdoctoral fellowship to N.L.M.
References
- Gard AL, White FP, Dutton GR. Extra-neural glial fibrillary acidic protein (GFAP) immunoreactivity in perisinusoidal stellate cells of rat liver. J Neuroimmunol 1985; 8:359 - 375
- Buniatian GH, Hartmann HJ, Traub P, Weser U, Wiesinger H, Gebhardt R. Acquisition of blood-tissue barrier-supporting features by hepatic stellate cells and astrocytes of myofibroblastic phenotype. Inverse dynamics of metallothionein and glial fibrillary acidic protein expression. Neurochem Int 2001; 38:373 - 383
- Geerts A. History, heterogeneity, developmental biology and functions of quiescent hepatic stellate cells. Semin Liver Dis 2001; 21:311 - 335
- Geerts A, Niki T, Hellemans K, De Craemer D, Van Den Berg K, Lazou JM, et al. Purification of rat hepatic stellate cells by side scatter-activated cell sorting. Hepatology 1998; 27:590 - 598
- Bataller R, Brenner DA. Hepatic stellate cells as a target for the treatment of liver fibrosis. Semin Liver Dis 2001; 21:437 - 451
- Sofroniew MV. Reactive astrocytes in neural repair and protection. Neuroscientist 2005; 11:400 - 407
- Silver J, Miller JH. Regeneration beyond the glial scar. Nat Rev Neurosci 2004; 5:146 - 156
- Eddleston M, Mucke L. Molecular profile of reactive astrocytes—implications for their role in neurologic disease. Neuroscience 1993; 54:15 - 36
- Yoshida K, Gage FH. Cooperative regulation of nerve growth factor synthesis and secretion in fibroblasts and astrocytes by fibroblast growth factor and other cytokines. Brain Res 1992; 569:14 - 25
- Asada H, Ip NY, Pan L, Razack N, Parfitt MM, Plunkett RJ. Time course of ciliary neurotrophic factor mRNA expression is coincident with the presence of protoplasmic astrocytes in traumatized rat striatum. J Neurosci Res 1995; 40:22 - 30
- Wu VW, Schwartz JP. Cell culture models for reactive gliosis: new perspectives. J Neurosci Res 1998; 51:675 - 681
- Pinzani M. Novel insights into the biology and physiology of the Ito cell. Pharmacol Ther 1995; 66:387 - 412
- Taub R. Liver regeneration: from myth to mechanism. Nat Rev Mol Cell Biol 2004; 5:836 - 847
- Bush TG, Puvanachandra N, Horner CH, Polito A, Ostenfeld T, Svendsen CN, et al. Leukocyte infiltration, neuronal degeneration and neurite outgrowth after ablation of scar-forming, reactive astrocytes in adult transgenic mice. Neuron 1999; 23:297 - 308
- Okada S, Nakamura M, Katoh H, Miyao T, Shimazaki T, Ishii K, et al. Conditional ablation of Stat3 or Socs3 discloses a dual role for reactive astrocytes after spinal cord injury. Nat Med 2006; 12:829 - 834
- Kalinichenko VV, Bhattacharyya D, Zhou Y, Gusarova GA, Kim W, Shin B, et al. Foxf1+/− mice exhibit defective stellate cell activation and abnormal liver regeneration following CCl4 injury. Hepatology 2003; 37:107 - 117
- Passino MA, Adams RA, Sikorski SL, Akassoglou K. Regulation of hepatic stellate cell differentiation by the neurotrophin receptor p75NTR. Science 2007; 315:1853 - 1856
- Voskuhl RR, Peterson RS, Song B, Ao Y, Morales LB, Tiwari-Woodruff S, et al. Reactive astrocytes form scar-like perivascular barriers to leukocytes during adaptive immune inflammation of the CNS. J Neurosci 2009; 29:11511 - 11522
- Gressner AM, Weiskirchen R. Modern pathogenetic concepts of liver fibrosis suggest stellate cells and TGFbeta as major players and therapeutic targets. J Cell Mol Med 2006; 10:76 - 99
- Hellerbrand C, Stefanovic B, Giordano F, Burchardt ER, Brenner DA. The role of TGFbeta1 in initiating hepatic stellate cell activation in vivo. J Hepatol 1999; 30:77 - 87
- Schnabl B, Kweon YO, Frederick JP, Wang XF, Rippe RA, Brenner DA. The role of Smad3 in mediating mouse hepatic stellate cell activation. Hepatology 2001; 34:89 - 100
- Uemura M, Swenson ES, Gaca MD, Giordano FJ, Reiss M, Wells RG. Smad2 and Smad3 play different roles in rat hepatic stellate cell function and alpha-smooth muscle actin organization. Mol Biol Cell 2005; 16:4214 - 4224
- Wang Y, Moges H, Bharucha Y, Symes A. Smad3 null mice display more rapid wound closure and reduced scar formation after a stab wound to the cerebral cortex. Exp Neurol 2007; 203:168 - 184
- Lagord C, Berry M, Logan A. Expression of TGFbeta2 but not TGFbeta1 correlates with the deposition of scar tissue in the lesioned spinal cord. Mol Cell Neurosci 2002; 20:69 - 92
- Wyss-Coray T, Feng L, Masliah E, Ruppe MD, Lee HS, Toggas SM, et al. Increased central nervous system production of extracellular matrix components and development of hydrocephalus in transgenic mice overexpressing transforming growth factor-beta1. Am J Pathol 1995; 147:53 - 67
- Asher RA, Morgenstern DA, Fidler PS, Adcock KH, Oohira A, Braistead JE, et al. Neurocan is upregulated in injured brain and in cytokine-treated astrocytes. J Neurosci 2000; 20:2427 - 2438
- Moon LD, Fawcett JW. Reduction in CNS scar formation without concomitant increase in axon regeneration following treatment of adult rat brain with a combination of antibodies to TGFbeta1 and beta2. Eur J Neurosci 2001; 14:1667 - 1677
- Adams RA, Passino M, Sachs BD, Nuriel T, Akassoglou K. Fibrin mechanisms and functions in nervous system pathology. Mol Interv 2004; 4:163 - 176
- Adams RA, Schachtrup C, Davalos D, Tsigelny I, Akassoglou K. Fibrinogen signal transduction as a mediator and therapeutic target in inflammation: Lessons from Multiple Sclerosis. Curr Med Chem 2007; 14:2925 - 2936
- Schachtrup C, Ryu JK, Helmrick MJ, Vagena E, Galanakis DK, Degen JL, et al. Fibrinogen triggers astrocyte scar formation by promoting the availability of active TGFbeta after vascular damage. J Neurosci 30:5843 - 5854
- Ng VL, Sabla GE, Melin-Aldana H, Kelley-Loughnane N, Degen JL, Bezerra JA. Plasminogen deficiency results in poor clearance of non-fibrin matrix and persistent activation of hepatic stellate cells after an acute injury. J Hepatol 2001; 35:781 - 789
- Nieto N. Oxidative-stress and IL-6 mediate the fibrogenic effects of rodent Kupffer cells on stellate cells. Hepatology 2006; 44:1487 - 1501
- Han YP, Zhou L, Wang J, Xiong S, Garner WL, French SW, et al. Essential role of matrix metalloproteinases in interleukin-1-induced myofibroblastic activation of hepatic stellate cell in collagen. J Biol Chem 2004; 279:4820 - 4828
- Yu C, Wang F, Jin C, Huang X, Miller DL, Basilico C, et al. Role of fibroblast growth factor type 1 and 2 in carbon tetrachloride-induced hepatic injury and fibrogenesis. Am J Pathol 2003; 163:1653 - 1662
- Herx LM, Yong VW. Interleukin-1beta is required for the early evolution of reactive astrogliosis following CNS lesion. J Neuropathol Exp Neurol 2001; 60:961 - 971
- Campbell IL, Abraham CR, Masliah E, Kemper P, Inglis JD, Oldstone MB, et al. Neurologic disease induced in transgenic mice by cerebral overexpression of interleukin 6. Proc Natl Acad Sci USA 1993; 90:10061 - 10065
- Akassoglou K, Bauer J, Kassiotis G, Pasparakis M, Lassmann H, Kollias G, et al. Oligodendrocyte apoptosis and primary demyelination induced by local TNF/p55TNF receptor signaling in the central nervous system of transgenic mice: models for multiple sclerosis with primary oligodendrogliopathy. Am J Pathol 1998; 153:801 - 813
- Penkowa M, Giralt M, Lago N, Camats J, Carrasco J, Hernandez J, et al. Astrocyte-targeted expression of IL-6 protects the CNS against a focal brain injury. Exp Neurol 2003; 181:130 - 148
- Mattson MP, Cheng B, Baldwin SA, Smith-Swintosky VL, Keller J, Geddes JW, et al. Brain injury and tumor necrosis factors induce calbindin D-28k in astrocytes: evidence for a cytoprotective response. J Neurosci Res 1995; 42:357 - 370
- Yong VW, Moumdjian R, Yong FP, Ruijs TC, Freedman MS, Cashman N, et al. Gamma-interferon promotes proliferation of adult human astrocytes in vitro and reactive gliosis in the adult mouse brain in vivo. Proc Natl Acad Sci USA 1991; 88:7016 - 7020
- Reuss B, Dono R, Unsicker K. Functions of fibroblast growth factor (FGF)-2 and FGF-5 in astroglial differentiation and blood-brain barrier permeability: evidence from mouse mutants. J Neurosci 2003; 23:6404 - 6412
- Liu B, Chen H, Johns TG, Neufeld AH. Epidermal growth factor receptor activation: an upstream signal for transition of quiescent astrocytes into reactive astrocytes after neural injury. J Neurosci 2006; 26:7532 - 7540
- Smith GM, Strunz C. Growth factor and cytokine regulation of chondroitin sulfate proteoglycans by astrocytes. Glia 2005; 52:209 - 218
- Gris P, Tighe A, Levin D, Sharma R, Brown A. Transcriptional regulation of scar gene expression in primary astrocytes. Glia 2007; 55:1145 - 1155
- Sofroniew MV. Molecular dissection of reactive astrogliosis and glial scar formation. Trends Neurosci 2009; 32:638 - 647
- Jin YL, Enzan H, Kuroda N, Hayashi Y, Nakayama H, Zhang YH, et al. Tissue remodeling following submassive hemorrhagic necrosis in rat livers induced by an intraperitoneal injection of dimethylnitrosamine. Virchows Arch 2003; 442:39 - 47
- Janzer RC, Raff MC. Astrocytes induce blood-brain barrier properties in endothelial cells. Nature 1987; 325:253 - 257
- Koehler RC, Roman RJ, Harder DR. Astrocytes and the regulation of cerebral blood flow. Trends Neurosci 2009; 32:160 - 169
- Thimgan MS, Yee HF Jr. Quantitation of rat hepatic stellate cell contraction: stellate cells' contribution to sinusoidal resistance. Am J Physiol 1999; 277:137 - 143
- Rockey DC, Weisiger RA. Endothelin induced contractility of stellate cells from normal and cirrhotic rat liver: implications for regulation of portal pressure and resistance. Hepatology 1996; 24:233 - 240
- Sato M, Suzuki S, Senoo H. Hepatic stellate cells: unique characteristics in cell biology and phenotype. Cell Struct Funct 2003; 28:105 - 112
- Magness ST, Bataller R, Yang L, Brenner DA. A dual reporter gene transgenic mouse demonstrates heterogeneity in hepatic fibrogenic cell populations. Hepatology 2004; 40:1151 - 1159
- Friedman SL. Stellate cells: a moving target in hepatic fibrogenesis. Hepatology 2004; 40:1041 - 1043
- Bioulac-Sage P, Lafon ME, Saric J, Balabaud C. Nerves and perisinusoidal cells in human liver. J Hepatol 1990; 10:105 - 112
- Rafuse VF, Soundararajan P, Leopold C, Robertson HA. Neuroprotective properties of cultured neural progenitor cells are associated with the production of sonic hedgehog. Neuroscience 2005; 131:899 - 916
- Sicklick JK, Li YX, Choi SS, Qi Y, Chen W, Bustamante M, et al. Role for hedgehog signaling in hepatic stellate cell activation and viability. Lab Invest 2005; 85:1368 - 1380
- Oben JA, Roskams T, Yang S, Lin H, Sinelli N, Torbenson M, et al. Hepatic fibrogenesis requires sympathetic neurotransmitters. Gut 2004; 53:438 - 445
- Trim N, Morgan S, Evans M, Issa R, Fine D, Afford S, et al. Hepatic stellate cells express the low affinity nerve growth factor receptor p75 and undergo apoptosis in response to nerve growth factor stimulation. Am J Pathol 2000; 156:1235 - 1243
- Hashmi AZ, Hakim W, Kruglov EA, Watanabe A, Watkins W, Dranoff JA, et al. Adenosine inhibits cytosolic calcium signals and chemotaxis in hepatic stellate cells. Am J Physiol Gastrointest Liver Physiol 2007; 292:395 - 401
- Chao MV. Neurotrophins and their receptors: a convergence point for many signalling pathways. Nat Rev Neurosci 2003; 4:299 - 309
- Lomen-Hoerth C, Shooter EM. Widespread Neurotrophin Receptor Expression in the Immune System and Other Nonneuronal Rat Tissues. Journal of Neurochemistry 1995; 64:1780 - 1789
- Cassiman D, Denef C, Desmet VJ, Roskams T. Human and rat hepatic stellate cells express neurotrophins and neurotrophin receptors. Hepatology 2001; 33:148 - 158
- Oakley F, Trim N, Constandinou CM, Ye W, Gray AM, Frantz G, et al. Hepatocytes express nerve growth factor during liver injury: evidence for paracrine regulation of hepatic stellate cell apoptosis. Am J Pathol 2003; 163:1849 - 1858
- Geerts A. The simple truth is seldom true and never simple: dual role for p75(NTR) in transdifferentation and cell death of hepatic stellate cells. Hepatology 2007; 46:600 - 601
- Zvibel I, Atias D, Phillips A, Halpern Z, Oren R. Thyroid hormones induce activation of rat hepatic stellate cells through increased expression of p75 neurotrophin receptor and direct activation of Rho. Lab Invest 2010; 90:674 - 684
- Trentin AG. Thyroid hormone and astrocyte morphogenesis. J Endocrinol 2006; 189:189 - 197
- Kendall TJ, Hennedige S, Aucott RL, Hartland SN, Vernon MA, Benyon RC, et al. p75 Neurotrophin receptor signaling regulates hepatic myofibroblast proliferation and apoptosis in recovery from rodent liver fibrosis. Hepatology 2009; 49:901 - 910
- Suzuki K, Tanaka M, Watanabe N, Saito S, Nonaka H, Miyajima A. p75 Neurotrophin receptor is a marker for precursors of stellate cells and portal fibroblasts in mouse fetal liver. Gastroenterology 2008; 135:270 - 281 3
- Yamashita T, Tohyama M. The p75 receptor acts as a displacement factor that releases Rho from Rho-GDI. Nat Neurosci 2003; 6:461 - 467
- Hutton LA, deVellis J, Perez-Polo JR. Expression of p75NGFR TrkA and TrkB mRNA in rat C6 glioma and type I astrocyte cultures. J Neurosci Res 1992; 32:375 - 383
- Cragnolini AB, Huang Y, Gokina P, Friedman WJ. Nerve growth factor attenuates proliferation of astrocytes via the p75 neurotrophin receptor. Glia 2009; 57:1386 - 1392
- Rudge JS, Li Y, Pasnikowski EM, Mattsson K, Pan L, Yancopoulos GD, et al. Neurotrophic factor receptors and their signal transduction capabilities in rat astrocytes. Eur J Neurosci 1994; 6:693 - 705
- Dougherty KD, Milner TA. p75NTR immunoreactivity in the rat dentate gyrus is mostly within presynaptic profiles but is also found in some astrocytic and postsynaptic profiles. J Comp Neurol 1999; 407:77 - 91
- Hanbury R, Charles V, Chen EY, Leventhal L, Rosenstein JM, Mufson EJ, et al. Excitotoxic and metabolic damage to the rodent striatum: role of the p75 neurotrophin receptor and glial progenitors. J Comp Neurol 2002; 444:291 - 305
- Oderfeld-Nowak B, Orzylowska-Sliwinska O, Soltys Z, Zaremba M, Januszewski S, Janeczko K, et al. Concomitant upregulation of astroglial high and low affinity nerve growth factor receptors in the CA1 hippocampal area following global transient cerebral ischemia in rat. Neuroscience 2003; 120:31 - 40
- Volosin M, Trotter C, Cragnolini A, Kenchappa RS, Light M, Hempstead BL, et al. Induction of proneurotrophins and activation of p75NTR-mediated apoptosis via neurotrophin receptor-interacting factor in hippocampal neurons after seizures. J Neurosci 2008; 28:9870 - 9879
- Cragnolini AB, Friedman WJ. The function of p75NTR in glia. Trends Neurosci 2008; 31:99 - 104
- Giuliani A, D'Intino G, Paradisi M, Giardino L, Calza L. p75(NTR)-immunoreactivity in the subventricular zone of adult male rats: expression by cycling cells. J Mol Histol 2004; 35:749 - 758
- Johnston AL, Lun X, Rahn JJ, Liacini A, Wang L, Hamilton MG, et al. The p75 neurotrophin receptor is a central regulator of glioma invasion. PLoS Biol 2007; 5:212
- Wang L, Rahn JJ, Lun X, Sun B, Kelly JJ, Weiss S, et al. Gamma-secretase represents a therapeutic target for the treatment of invasive glioma mediated by the p75 neurotrophin receptor. PLoS Biol 2008; 6:289
- Herrmann JL, Menter DG, Hamada J, Marchetti D, Nakajima M, Nicolson GL. Mediation of NGF-stimulated extracellular matrix invasion by the human melanoma low-affinity p75 neurotrophin receptor: melanoma p75 functions independently of trkA. Mol Biol Cell 1993; 4:1205 - 1216
- Shonukan O, Bagayogo I, McCrea P, Chao M, Hempstead B. Neurotrophin-induced melanoma cell migration is mediated through the actin-bundling protein fascin. Oncogene 2003; 22:3616 - 3623
- Walch ET, Albino AP, Marchetti D. Correlation of overexpression of the low-affinity p75 neurotrophin receptor with augmented invasion and heparanase production in human malignant melanoma cells. Int J Cancer 1999; 82:112 - 120
- Denkins Y, Reiland J, Roy M, Sinnappah-Kang ND, Galjour J, Murry BP, et al. Brain metastases in melanoma: roles of neurotrophins. Neuro Oncol 2004; 6:154 - 165
- Uyama N, Shimahara Y, Kawada N, Seki S, Okuyama H, Iimuro Y, et al. Regulation of cultured rat hepatocyte proliferation by stellate cells. J Hepatol 2002; 36:590 - 599
- Mabuchi A, Mullaney I, Sheard P, Hessian P, Zimmermann A, Senoo H, et al. Role of Hepatic Stellate Cells in the Early Phase of Liver Regeneration in Rat: Formation of Tight Adhesion to Parenchymal Cells. Comp Hepatol 2004; 3:29
- Mabuchi A, Mullaney I, Sheard PW, Hessian PA, Mallard BL, Tawadrous MN, et al. Role of hepatic stellate cell/hepatocyte interaction and activation of hepatic stellate cells in the early phase of liver regeneration in the rat. J Hepatol 2004; 40:910 - 916
- Krieglstein K, Strelau J, Schober A, Sullivan A, Unsicker K. TGFbeta and the regulation of neuron survival and death. J Physiol Paris 2002; 96:25 - 30
- Brionne TC, Tesseur I, Masliah E, Wyss-Coray T. Loss of TGFbeta1 leads to increased neuronal cell death and microgliosis in mouse brain. Neuron 2003; 40:1133 - 1145
- Rudge JS. Murphy S. Astrocyte-derived neurotrophic factors. Astrocytes: pharmacology and function 1993; New York Academic 267 - 305
- Herrmann JE, Imura T, Song B, Qi J, Ao Y, Nguyen TK, et al. STAT3 is a critical regulator of astrogliosis and scar formation after spinal cord injury. J Neurosci 2008; 28:7231 - 7243
- Lu P, Yang H, Jones LL, Filbin MT, Tuszynski MH. Combinatorial therapy with neurotrophins and cAMP promotes axonal regeneration beyond sites of spinal cord injury. J Neurosci 2004; 24:6402 - 6409
- Kadoya K, Tsukada S, Lu P, Coppola G, Geschwind D, Filbin MT, et al. Combined intrinsic and extrinsic neuronal mechanisms facilitate bridging axonal regeneration one year after spinal cord injury. Neuron 2009; 64:165 - 172
- Cafferty WB, Yang SH, Duffy PJ, Li S, Strittmatter SM. Functional axonal regeneration through astrocytic scar genetically modified to digest chondroitin sulfate proteoglycans. J Neurosci 2007; 27:2176 - 2185
- Tsukamoto H, She H, Hazra S, Cheng J, Miyahara T. Anti-adipogenic regulation underlies hepatic stellate cell transdifferentiation. J Gastroenterol Hepatol 2006; 21:102 - 105
- Kisseleva T, Brenner DA. Hepatic stellate cells and the reversal of fibrosis. J Gastroenterol Hepatol 2006; 21:84 - 87
- Niki T, Pekny M, Hellemans K, Bleser PD, Berg KV, Vaeyens F, et al. Class VI intermediate filament protein nestin is induced during activation of rat hepatic stellate cells. Hepatology 1999; 29:520 - 527
- Uyama N, Zhao L, Van Rossen E, Hirako Y, Reynaert H, Adams DH, et al. Hepatic stellate cells express synemin, a protein bridging intermediate filaments to focal adhesions. Gut 2006; 55:1276 - 1289
- Cassiman D, van Pelt J, De Vos R, Van Lommel F, Desmet V, Yap SH, et al. Synaptophysin: A novel marker for human and rat hepatic stellate cells. Am J Pathol 1999; 155:1831 - 1839
- Knittel T, Aurisch S, Neubauer K, Eichhorst S, Ramadori G. Cell-type-specific expression of neural cell adhesion molecule (N-CAM) in Ito cells of rat liver. Upregulation during in vitro activation and in hepatic tissue repair. Am J Pathol 1996; 149:449 - 462
- Lang A, Schrum LW, Schoonhoven R, Tuvia S, Solis-Herruzo JA, Tsukamoto H, et al. Expression of small heat shock protein alphaB-crystallin is induced after hepatic stellate cell activation. Am J Physiol Gastrointest Liver Physiol 2000; 279:1333 - 1342
- Nishi M, Takeshima H, Houtani T, Nakagawara K, Noda T, Sugimoto T. RhoN, a novel small GTP-binding protein expressed predominantly in neurons and hepatic stellate cells. Brain Res Mol Brain Res 1999; 67:74 - 81
- Chardin P. Function and regulation of Rnd proteins. Nat Rev Mol Cell Biol 2006; 7:54 - 62
- Dranoff JA, Ogawa M, Kruglov EA, Gaca MD, Sevigny J, Robson SC, et al. Expression of P2Y nucleotide receptors and ectonucleotidases in quiescent and activated rat hepatic stellate cells. Am J Physiol Gastrointest Liver Physiol 2004; 287:417 - 424
- Sohail MA, Hashmi AZ, Hakim W, Watanabe A, Zipprich A, Groszmann RJ, et al. Adenosine induces loss of actin stress fibers and inhibits contraction in hepatic stellate cells via Rho inhibition. Hepatology 2009; 49:185 - 194
- Ruddell RG, Oakley F, Hussain Z, Yeung I, Bryan-Lluka LJ, Ramm GA, et al. A role for serotonin (5-HT) in hepatic stellate cell function and liver fibrosis. Am J Pathol 2006; 169:861 - 876
- Ebrahimkhani MR, Kiani S, Oakley F, Kendall T, Shariftabrizi A, Tavangar SM, et al. Naltrexone, an opioid receptor antagonist, attenuates liver fibrosis in bile duct ligated rats. Gut 2006; 55:1606 - 1616
- Siegmund SV, Uchinami H, Osawa Y, Brenner DA, Schwabe RF. Anandamide induces necrosis in primary hepatic stellate cells. Hepatology 2005; 41:1085 - 1095
- Teixeira-Clerc F, Julien B, Grenard P, Tran Van Nhieu J, Deveaux V, Li L, et al. CB1 cannabinoid receptor antagonism: a new strategy for the treatment of liver fibrosis. Nat Med 2006; 12:671 - 676
- Julien B, Grenard P, Teixeira-Clerc F, Van Nhieu JT, Li L, Karsak M, et al. Antifibrogenic role of the cannabinoid receptor CB2 in the liver. Gastroenterology 2005; 128:742 - 755
- Oben JA, Yang S, Lin H, Ono M, Diehl AM. Acetylcholine promotes the proliferation and collagen gene expression of myofibroblastic hepatic stellate cells. Biochem Biophys Res Commun 2003; 300:172 - 177
- Siegmund SV, Qian T, de Minicis S, Harvey-White J, Kunos G, Vinod KY, et al. The endocannabinoid 2-arachidonoyl glycerol induces death of hepatic stellate cells via mitochondrial reactive oxygen species. FASEB J 2007; 21:2798 - 2806
- Oben JA, Yang S, Lin H, Ono M, Diehl AM. Norepinephrine and neuropeptide Y promote proliferation and collagen gene expression of hepatic myofibroblastic stellate cells. Biochem Biophys Res Commun 2003; 302:685 - 690