Abstract
Caveolin proteins are structural components of caveolae and are involved in the regulation of many biological processes. Recent studies have shown that caveolin-1 modulates inflammatory responses and is important for sepsis development. In the present study, we show that caveolin-1 and caveolin-2 have opposite roles in lipopolysaccharide (LPS)-induced sepsis using caveolin-deficient (Cav-1-/- and Cav-2-/-) mice for each of these proteins. While Cav-1-/- mice displayed delayed mortality following challenge with LPS, Cav-2-/- mice were more sensitive to LPS compared to wild-type (WT). With Cav-2-/- mice, this effect was associated with increased intestinal injury and increased intestinal permeability. This negative outcome was also correlated with enhanced expression of iNOS in epithelial intestinal cells, and enhanced production of nitric oxide (NO). By contrast, Cav-1-/- mice demonstrated a decrease in iNOS expression with decreased NO production, but no alteration in intestinal permeability. The differential expression of iNOS was associated with a significant increase of STAT-1 activation in these mice. Intestinal cells of Cav-2-/- mice showed increased phosphorylation of STAT-1 at tyrosine 701 compared to wild-type. However, Cav-1-/- mice-derived intestinal cells showed decreased levels of phosphorylation of STAT-1 at tyrosine 701. Since caveolin-2 is almost completely absent in Cav-1-/- mice, we conclude that it is not just the absence of caveolin-2 that is responsible for the observed effects, but that the balance between caveolin-1 and caveolin-2 is important for iNOS expression and ultimately for sepsis outcome.
Introduction
Caveolins are integral constituents of caveolae that are 50–100 nm invaginations of the plasma membrane. The family of caveolin proteins is composed of caveolin-1 (Cav-1), caveolin-2 (Cav-2) and caveolin-3 (Cav-3). While Cav-1 and Cav-2 show ubiquitous expression, Cav-3 expression is restricted to muscle cells.Citation1,Citation2 Cav-1 is the most studied caveolin isoform. It has been shown that besides its plasma membrane structural role, Cav-1 is an important activity modulator of a large variety of proteins involved in the regulation of signal transduction. Cav-1 can interact with proteins through its caveolin scaffolding domain (CSD) that is recognized by the caveolin binding domain (CBD), which has been identified in numerous caveolar proteins. Examples of caveolin-interacting proteins are protein kinase C (PKC), protein kinase A (PKA), phosphatidylinositol-3-kinase (PI3K), epidermal growth factor receptor (EGFR) and endothelial synthase (eNOS). Usually, this interaction leads to the inactivation of proteins bound to Cav-1.Citation3,Citation4 Cav-1 has also been involved in many biological processes, such as transcytosis, potocytosis, cholesterol metabolism and insulin signaling.Citation4
Cav-3 aminoacid sequence is 65% identical and 85% similar to that of Cav-1 (human proteins), and it also contains a CSD that confers the ability to interact with, and inactivate other proteins. On the other hand, Cav-2 aminoacid sequence is more divergent from that of Cav-1 (38% identical and 58% similar to caveolin-1 for the human forms),Citation5 and its CSD does not appear to have any regulatory function, but may rather allow Cav-2 to localize to the Golgi apparatus.Citation6
Cav-1 and Cav-3 can form homo-oligomers and are essential for caveolae formation in non-muscle and muscle cells, respectively. Cav-2 does not form homo-oligomers, but can hetero-oligomerize with Cav-1. This association between the proteins appears to induce the formation of deeper caveolae.Citation7 Other studies have shown that phosphorylation of Cav-2 at serines 23 and 36 favors Cav-1-dependent formation of caveolae.Citation8 Despite being highly co-regulated and having cooperative roles in caveolae formation, Cav-1 and Cav-2 can also display opposite activities. Shmuel and collaborators have shown that Cav-2 inhibits endocytosis of mAChR via a clathrin-mediated pathway, a process inhibited by ectopic Cav-2 expression. Co-expression of Cav-2 with Cav-1 rescues Cav-2 inhibitory effect.Citation9 Nonetheless, information about the roles of Cav-2 is still scarce.
Sepsis is a condition that results from an exacerbated response of the host against infections. It is a major health problem responsible for the high rate of mortality in critically ill patients. Gramnegative bacteria are important inducers of sepsis, which is caused by inflammatory responses triggered by components of the bacteria cell wall, such as lipopolyssacharide (LPS), also referred to as endotoxin. In vivo administration of LPS to mice provokes endotoxemia, and is widely used as a model for sepsis research. LPS can provoke local inflammation, shock or even death, depending on the dose utilized. Among the LPS-induced-responses that may be relevant for the outcome of the septic shock, cytokine release, leukocyte recruitment, nitric oxide (NO) production and tissue damage play an important role.
Sepsis induces the activation of a very complex network of biological events, and a large variety of molecules are involved in this pathway. Genetically engineered mice deficient in specific molecules have been used to study the involvement of proteins in different processes that integrate biological responses resulting in sepsis development. Using a mouse model of acute endotoxemia, Garrean and collaborators have shown that Cav-1-deficient mice are more resistant to LPS-induced mortality. Furthermore, the absence of Cav-1 was associated with reduced vascular permeability, attenuated neutrophil recruitment and edema formation in the lung. These observations were attributed to an increase in eNOS-derived NO production and the subsequent suppression of NFκB activity and iNOS transcription.Citation10 In contrast, Medina and collaborators have shown that Cav-1-deficient mice are more susceptible to Salmonella enterovar infection. Cav-1-/- mice were shown to produce inflammatory cytokines and NO more effectively compared to wild-type.Citation11 These contradictory results may be related to the model used, i.e., purified LPS versus whole bacteria as the inflammation inducer. LPS can mimic many inflammatory reactions triggered by Gram-negative bacteria, but biological effects, such as phagocytosis and subsequent cellular signaling, are not induced. Some cellular responses, including cytokine production, are at least in part, dependent on phagocytosis of the whole bacteria.Citation12
In 2000, Lei and Morrison have shown for the first time that Cav-1 expression is modulated by LPS in various macrophage cell lines.Citation13 The role of Cav-1 in LPS signaling has recently been examined in more details. LPS was shown to enhance Cav-1 expression in a proteasome and p38-MAP kinase-dependent manner.Citation14 Furthermore, Cav-1 was shown to play a role in LPS signaling, inducing an anti-inflammatory phenotype, characterized by the increased production of IL-10 and decreased production of IL-6 and TNFα by macrophages. This effect appears to be dependent on p38-MAP kinase and involves the reduction of NFκB and AP-1 activation.Citation1 Recently, it was demonstrated that the production of IL-6 and TNFα is inhibited by TLR4 bound to Cav-1, and that the inhibitory effect of Cav-1 involves HO-1 trafficking to caveolae, a process that is inhibited by p38-MAP kinase.Citation15
In the present study, we decided to examine the role of Cav-1 and Cav-2 in a mouse model of endotoxemia. The role of Cav-2 in lung function and its association with Cav-1 may suggest a potential role for this protein in the regulation of sepsis development. This work was undertaken to better explore the role of Cav-1 and Cav-2 in sepsis development.
Results
Cav-2-/- mice are highly sensitive to lethal doses of LPS.
Lethal endotoxemia was induced by i.p. injection of 20 mg/kg LPS in wild-type, and Cav-1-/- or Cav-2-/- mice. Experimental groups were composed of 10 mice for each genotype. Cav-2-/- mice were significantly more sensitive to LPS lethality compared to wildtype or Cav-1 deficient mice ().
Plasma levels of inflammatory mediators are similar in Cav-2-/- and wild-type mice.
Inflammatory cytokines and chemokines are important mediators of the LPS-response leading to septic shock. We examined the levels of inflammatory mediators in the plasma of wild-type and Cav-2-/- mice injected with saline or LPS. We did not find any significant change in any of the mediators analyzed in Cav-2-/- mice in comparison to wild-type. Therefore, the higher sensitivity to LPS exhibited by Cav-2-/- mice could not be explained by an exacerbated production of cytokines and chemokines. Instead, Cav-2-/- mice produce equal or diminished levels of inflammatory mediators in comparison to wild-type mice (). In conclusion, the increased LPS-induced mortality among Cav-2-/- mice is not provoked by an inflammatory dysfunction in these mice.
The increased susceptibility of Cav-2-/- mice to LPS is associated with enhanced tissue damage of the gut.
One of the consequences of bacterial infection that may result in sepsis is tissue damage and organ failure. Tissue damage was evaluated in distinct organs 12 h after LPS challenge in order to investigate whether Cav-2 could affect distinct organs differentially. This experiment was also performed to identify the cause for the increased LPS sensitivity of Cav-2-/- mice. No difference was observed in the lungs, liver, kidneys, spleen and heart of Cav-2-/- mice and Cav-1-/- mice, when compared to wild-type mice. However, the lack of Cav-2 was associated with increased LPS-induced damage of the small intestine. During sepsis, evidence of intestinal damage was demonstrated by shortened and distorted villi. This effect was more prominent in the ileum of Cav-2-/- mice compared to wild-type mice (). Damage of the small intestine can be measured by the ratio of the length of villi over the length of crypts. In this experiment, tissue injury was demonstrated by a decrease of this ratio value. The ileum of Cav-2-/- mice was more damaged than those of wild-type mice based on this parameter (). In conclusion, Cav-2-deficient mice showed increased ileum damage in comparison to wildtype mice. This effect was correlated with enhanced sensitivity to LPS-induced mortality.
Electron microscopic analysis of Cav-2-deficient epithelial cells.
One well-known effect of septic shock induced by LPS is intestinal injury that can result in barrier dysfunction of the epithelium with subsequent bacterial translocation, loss of fluid and diarrhea. Altered permeability may be a result of dysfunctions in paracellular and/or transcellular transport. Barrier integrity of the intestinal epithelium was examined by electron microscopy. Tight junctions of ileal epithelial cells of Cav-2 deficient mice were intact, ruling out the hypothesis that increased permeability could be the result of the loss of tight junction barrier function of epithelial cells (). However, epithelial cells of Cav-2 deficient mice had large cytoplasmic vacuoles that were not observed in ileal epithelial cells of wild-type mice (). This finding may indicate a more active transcellular transport of fluid through Cav-2-/-cells in comparison to wild-type cells.
Electron microscopy also revealed that in LPS challenged mice, epithelial cells of the ileum showed swollen mitochondria, which are indicative of increased cytotoxicity. This effect was more pronounced in Cav-2-/- mice than in wild-type mice ().
Cav-2-/- mice show increased permeability of the intestine.
We also determined whether permeability was differentially altered in LPS-treated mice, since it has been reported in the literature that Cav-1-/- mice or Cav-1-/- derived cells show altered permeability in various models. For this purpose, 45 minutes before sacrifice, mice were retro-orbitally injected with Evans Blue dye 12 h after LPS injection. This dye has a high affinity for albumin and is widely used to study transport of plasma substances. After sacrifice, mice were perfused with saline in order to remove Evans Blue dye from the circulation and organs were collected for elution of the dye. Permeability was not altered in the liver, heart, kidney, lung and spleen in Cav-2-/- mice in comparison to wild-type mice (). Cav-1-/- mice only show altered permeability in the lungs, where permeability was decreased compared to that observed in wild-type mice. In contrast, Cav-2-/- mice showed increased permeability in the ileum and colon in comparison to wild-type mice ().
The differential sensitivity of Cav-2-/- mice to LPS-induced death is associated with increased intestinal damage and increased permeability in this organ compared to wild-type and Cav-1-/- mice.
Cav-2-/- mice display increased production of NO compared to wild-type mice.
A putative mediator of enhanced intestinal injury and permeability is NO. NO is upregulated in endotoxic animals and is a modulator of vascular permeability and mucosal integrity.Citation17–Citation20 In order to evaluate whether nitric oxide is involved in the increased susceptibility of Cav-2 deficient mice, peritoneal lavages of Cav-2 deficient and wild-type mice injected with LPS or saline were collected to assess nitric oxide levels. NO levels in Cav-2-deficient mice were significantly increased compared to those observed in wild-type mice (). In contrast, Cav-1-/- mice showed decreased levels of NO after LPS challenge compared to WT mice. The increase in NO levels in Cav-2-deficient mice correlated with increased LPS-induced intestinal mucosal permeability, ileum damage and LPS-induced mortality.
iNOS expression is increased in Cav-2-/- mice compared to wild-type mice.
NO may be protective or harmful to tissues depending on its concentration. High concentrations of NO lead to enhanced epithelial permeability and cytotoxicity and are generated by the inducible form of NO synthase (iNOS). Importantly, increased iNOS expression and NO production have been implicated in sepsis and inflammatory bowel diseases.Citation17–Citation19,Citation21 iNOS protein expression levels were therefore examined by immunoblot analysis. We found that, in colon or small intestine, expression of iNOS was enhanced in Cav-2-/- mice compared to WT mice. Cav-1-/- mice showed decreased levels of iNOS, in agreement with the decreased levels of NO detected in the peritoneal lavage of LPS-challenged mice (). In conclusion, Cav-2-/- mice showed increased iNOS protein expression that correlates with increased NO levels in the peritoneal cavity of these mice.
STAT1 is hyperactivated in Cav-2-/- mice after LPS injection.
We next examined the activation of signal transduction pathways involved in the regulation of iNOS protein levels. STAT1 is activated through the Janus kinase-STAT pathway and is involved in LPS-induced iNOS expression.Citation22,Citation23 Our results show that STAT1 is hyperactivated in intestine of Cav-1-/- mice compared to intestine obtained from wild-type mice. In contrast, Cav-1-/- mice showed decreased levels of phosphorylated-STAT1 (). These results correlate well with the levels of iNOS protein expression and NO levels observed in these mice.
Discussion
Caveolins are structural proteins of caveolae that are involved in many biological processes. Cav-1 and Cav-2 are often coexpressed, interact and have cooperative roles in caveolae formation. However, Cav-1 and Cav-2 also display contrasting roles, as in the case of endocytosis of the M1 muscarinic receptor endocytosis.Citation9 In the present study, we have explored the roles of Cav-1 and Cav-2 in LPS-induced sepsis and found that both proteins contribute to sepsis development in opposite manners.
Recent studies have shown that Cav-1 is implicated in sepsis development in a study using Cav-1-/- mice.Citation10 However, since these mice do not express Cav-2 as well, it is therefore not possible to differentiate the effects related to the absence of Cav-1 or Cav-2. Garrean and collaborators showed that Cav-1-/- mice are less sensitive to LPS-induced mortality in the first 12 hours. However, later time points were not analyzed. We analyzed the survival of mice until day 6 after LPS injection, and we observed the same rate of mortality among Cav-1-/- and WT mice. By constrast, Cav-2-/- mice, showed a striking increased sensitivity to LPS compared to WT mice.
The molecular basis for the effects described by Garrean and collaborators was attributed to an increase in eNOS-derived NO, with subsequent decreased NFκB activity and decreased iNOS transcription levels. In this study, the differential sensitivity of Cav-1-/- mice and Cav-2-/- mice is also correlated with altered iNOS expression and NO production. After LPS treatment, Cav-1-/- mice display reduced instestinal iNOS protein levels compared to WT mice and produce less NO. On the other hand, Cav-2-/- mice, which are more sensitive to LPS, display increased iNOS protein levels and produce more NO than WT mice. NO is produced by distinct isoforms of NO-synthases (NOS). eNOS (a.k.a. NOS3) and nNOS (a.k.a. NOS1) are Ca2+/calmodulin-dependent constitutive isoforms that produce NO in the nanomolar range. Both enzymes are usually involved in house-keeping reactions. High levels of NO may be produced by iNOS (aka, NOS2). This is not a Ca2+/calmodulin-dependent enzyme and it is usually induced during the development of an inflammatory reaction. iNOS expression is frequently associated with tissue damage, while eNOS expression may have a protective role.Citation24 Large amounts of NO are responsible for many deleterious effects observed in the intestine in several disorders, such as Crohn disease, ulcerative colitis and sepsis.Citation25,Citation26 These effects include hypotension, cytotoxicity, inhibition of cytokine production and intestinal barrier dysfunction. Our results indicate that Cav-1 and Cav-2 expression differentially affect iNOS expression. We show that absence of Cav-1 and Cav-2 is associated with diminished iNOS expression, while the absence of Cav-2 alone is associated with increased iNOS expression. STAT1 has been shown to play an important role in the regulation of iNOS expression.Citation27–Citation29 In addition, it was shown to be specifically involved in iNOS expression in the intestine after LPS stimulation.Citation30 In agreement with these studies, we show that the levels of iNOS expression correlate with the level of STAT1 activation in Cav-1-/-, Cav-2-/- and WT mice.
As Cav-1, Cav-2 and eNOS are expressed in endothelial cells and iNOS in epithelial cells, we infer that the effect of caveolins on iNOS expression is paracrine. This effect is possibly related to eNOS expression and/or activity in WT, Cav-1-/- mice and Cav-2-/- mice. eNOS is expressed in endothelial cells and its activity is known to be negatively regulated by Cav-1. Garrean and collaborators have shown that Cav-1-deficient lungs produce less eNOS-derived NO. They also showed that there was a suppression of NFκB activity and diminished iNOS expression in Cav-1-/- mice. These effects were attributed to the fact that low concentrations of eNOS-derived NO regulates iNOS expression through NFκB activation, while high concentrations of NO have the opposite effect.Citation10,Citation31 We can speculate that the opposite outcome observed in Cav-1-/- mice and Cav-2-/- mice may be correlated with the production of eNOS-derived NO that would then affect iNOS expression in Cav-1-/-, Cav-2-/- and WT mice in an opposite manner. The activity of eNOS in Cav-1-deficient cells is expected to be upregulated according to various studies.Citation16,Citation32–Citation34 Increased eNOS-derived NO may be the basis for the decreased iNOS expression that we observed in Cav-1-/- mice in comparison to wild-type mice.Citation10 Regarding Cav-2, there is to our knowledge no information about this protein having a role in the regulation of eNOS activity. Cav-2-/- mice express Cav-1, so that eNOS can be regulated by Cav-1. Interestingly, Cav-2-/- mice show the highest levels of iNOS protein and NO. At high concentrations, NO is able to provoke cell damage, as evidenced by the swollen mitochondria and increased transcellular transport observed in intestine obtained from Cav-2-/- mice. Further studies will be necessary to understand how the absence of Cav-2 affects iNOS expression. These effects may possibly be related to an effect of Cav-2 on eNOS expression and/or activity.
The differential effects of a lethal dose of LPS in Cav-1-/- mice and Cav-2-/- mice indicate that Cav-2 plays a role in the response to LPS. Moreover, our results indicate that the balance of Cav-1 and Cav-2 could define the outcome of sepsis, since Cav-1-/- mice do not express Cav-2 either. Cav-1 was shown to interact with STAT3 and regulate its activity in several cell types.Citation35 Similarly, our data suggest that Cav-2 may interact with STAT1 and regulates its activation. This finding is important as it would be the first to suggest a functional role for Cav-2.
Our results also indicate an inhibitory role for Cav-2 in the regulation of endocytosis, since Cav-2-/- mice show increased permeability to Evans Blue associated with the observation that there are more cytoplasmic vacuoles in intestinal epithelial cells. Cav-1 may counteract Cav-2 inhibitory effect and, because Cav-1-/- mice do not express Cav-2, they do not show enhanced intestinal permeability. These findings are in agreement with the study of Schubert and collaborators who showed that Cav-1-/- mice are deficient in the transcellular transport of albumin.Citation16 Taken together, our data show an opposite role for caveolins in the regulation of endocytosis. While Cav-2 acts as an inhibitory modulator, Cav-1 counteracts this action, as already shown by Schmuel and collaborators.Citation9
In summary, we show that Cav-2 has a crucial role in LPS-induced sepsis. This study indicates a novel role for Cav-2 as a component of the system leading to NO production with consequences for sepsis outcome. Moreover, Cav-2 is implicated in the transcytosis process involved in LPS-induced permeability. Interestingly, Cav-2 and Cav-1 appear to act in opposite manners during these processes. These data suggest that the balance of Cav-1 and Cav-2 protein levels and/or their sub-cellular localization may be determinant for sepsis development and outcome.
Methods
Materials.
LPS from Escherichia coli serotype 0111: B4, thioglycollate, Evans Blue and phosphatase inhibitors were purchased from Sigma-Aldrich (St. Louis, MO). Protease inhibitors were from Roche Applied Science (Indianapolis, IN) and LSAB2 System Kit from Dako (Carpinteria, CA). Antibodies were as follows: β-actin (cat # A1978, Sigma-Aldrich, St. Louis, MO), Cav-1 (cat #sc-894, Santa Cruz Biotechnology, Inc., Santa Cruz, CA), Cav-2 (cat # 610684, BD Biosciences, San Jose, CA), iNOS (cat # 610332, BD Biosciences, San Jose, CA), Phospho-STAT1 (cat # 612132, BD Biosciences, San Jose, CA), STAT1 (cat # 610115, BD Biosciences, San Jose, CA).
Animal studies.
All animals were housed and maintained in a pathogen-free environment/barrier facility at the Kimmel Cancer Center at Thomas Jefferson University. Mice were kept on a 12-hour light/dark cycle with ad libitum access to chow diet and water. Cav-1-/- and Cav-2-/- mice were generated as previously described in references Citation4 and Citation16. Only male WT, Cav-1-/- and Cav-2-/- mice in the C57Bl/6 genetic background were utilized for these studies. Experimental procedures involving animals were approved by the Institutional Animal Care and Use Committee (IACUC) of Thomas Jefferson University.
Survival study.
LPS injections were performed in male 10–12-wk-old C57BL/6 (25–30 g body weight). For the endotoxemia model, mice were intraperitoneally (i.p.) injected with LPS (20 mg/kg weight) from E. coli serotype 0111:B4 dissolved in sterile saline. Control animals received equivalent volumes of sterile saline. All mice challenged with LPS appeared acutely ill and displayed lethargy, piloerection, shivering and diarrhea. Survival was assessed every day for a total of 6 days.
Measurement of inflammatory mediators levels in plasma.
Plasma samples were obtained at 6 h, 12 h and 18 h after LPS administration. Mice were euthanized by CO2 inhalation and blood was collected by cardiac puncture. The plasma fraction was separated from the cellular components in tubes containing 50 µL of 3.2% sodium citrate by centrifugation at 800 g for 6 min at 4°C. The plasma fraction was stored at −80°C until assayed. Cytokines and chemokines were measured by a multiplex system (Thermo Fisher Scientific, Rockford, IL).
Histology.
Eighteen hours after LPS injection, mice were sacrificed and organs were collected, rinsed in PBS, and placed in 10% buffered neutral formalin for fixation. Twenty-four hours later, formalin was substituted with 70% ethanol. Tissue samples were paraffin-embeded, sectioned and stained with hematoxylin and eosin. Histological sections were examined with a Zeiss phase contrast microscope. Intestinal crypt and villi length were measured using the Image J program developed by Dr. Wayne Rasband (National Institutes of Health, Bethesda, MD; available at www.rsb.info.nih.gov/ij).
Permeability assay.
Mice were retro-orbitally injected with Evans blue dye (30 mg/kg weight) in PBS. Forty-five min later, mice were perfused via heart puncture with PBS. Organs (heart, lung, liver, kidney, spleen, jejunum, ileum and colon) were harvested and incubated in formamide for 3 days to elute Evans blue dye. OD of formamide solution was measured at 620 nm.
Electron microscopy.
Twelve-hours after LPS injection, mice were sacrificed and ileum and colon samples were collected, rinsed in PBS and placed in 2% glutaraldehyde for fixation. Fixed samples were rinsed three times in 0.1 M phosphate buffer pH 7.4 and exposed to 2% osmium tetra oxide for one hour in phosphate buffer. Samples were then rinsed in deionized water four times for 5 minutes each and stained with 0.1% uranyl acetate for 15 minutes. Samples were later rinsed with two changes of deionized water and dehydrated in graded steps of acetone. Finally, samples were infiltrated with Spurrs embedding media and blocks were polymerized overnight in a 65°C convection oven. The resulting blocks were thin sectioned (80 nm) with a diamond knife on a Leica Ultracut UCT and sections were collected on bare copper grids. Following post-staining with uranyl acetate and sodium bismuth the sections were observed on a Hitachi 7000 STEM and the electron micrographs were recorded on Kodak 4489 film.
Measurement of nitrite levels in the peritoneal lavage.
Twelve hours after saline or LPS injections, mice were sacrificed and their peritoneal cavity was washed with 3 mL of saline solution. The peritoneal fluid was centrifuged at 1,000 rpm for 5 min and stored at −80°C until assayed. NO was measured by spectrophotometry on the basis of the Griess reaction using the Nitrate/Nitrite Colorimetric Assay kit (Cayman Chemicals Company, Ann Arbor, MI) according to the manufacturer instructions.
Immunoblot.
For protein isolation from tissue, 1 mm2 ileum or colon tissue was isolated, promptly incubated in PBS with protease inhibitors, rinsed to remove the intestine content, minced, frozen in liquid nitrogen and stored at −80°C. The frozen tissue samples were then homogenized in an appropriate volume of lysis buffer (10 mmol/L Tris, pH 7.5, 150 mmol/L NaCl, 1% Triton X-100 and 60 mM n-octyl-glucoside), containing protease and phosphatase inhibitors. Tissue lysates were then centrifuged at 12,000x g for 10 minutes, at 4°C, to remove insoluble debris. Protein concentration was determined using the BCA protein assay (Pierce, Inc., Rockford, IL), and the volume required for 50 µg of protein was determined. Samples were then separated by sodium dodecyl sulfate-polyacrylamide gel electrophoresis (12% polyacrylamide) and transferred to nitrocellulose. All subsequent washing buffers contained 10 mmol/L Tris, pH 8.0, 150 mmol/L NaCl, 0.05% Tween 20, which was supplemented with 5% nonfat dry milk for the blocking solution or 1% bovine serum albumin for the antibody diluent. Horseradish peroxidase-conjugated secondary antibodies [anti-mouse 1:6,000 dilution (Pierce, Inc., Rockford, IL) or anti-rabbit 1:5,000 (Transduction Laboratories, Lexington, KY)] were used to visualize bound primary antibodies with the Supersignal chemiluminescence substrate (Pierce, Inc., Rockford, IL). When phospho-specific antibody probes were used, nonfat dry milk was omitted from the blocking and primary antibody solutions.
Statistical analysis.
Values in figures and text are presented as mean ± SEM. Result sets were examined by oneway analysis of variance and individual group means were compared using the unpaired Student's t-test. A p value of less than 0.05 was considered significant.
Abbreviations
AP-1 | = | activator protein 1 |
Cav-1 | = | caveolin-1 |
Cav-2 | = | caveolin-2 |
CSD | = | caveolin scaffolding domain |
CBD | = | caveolin binding domain |
eNOS | = | endothelial nitric oxide synthase |
iNOS | = | inducible nitric oxide synthase |
LPS | = | lipopolysaccharide |
MAP kinase | = | mitogen-activated protein kinase |
mAChR | = | muscarinic acetylcholine receptor |
NFκB | = | nuclear factor kappa-light-chain-enhancer of activated B cells |
NO | = | nitric oxide |
PBS | = | phosphate buffered saline |
STAT-1 | = | signal transducers and activators of transcription-1 |
IL-10 | = | interleukin-10 |
IL-6 | = | interleukin-6 |
TLR4 | = | toll-like receptor 4 |
TNFα | = | tumor necrosis factor |
Figures and Tables
Figure 1 Cav-2-/- mice are more sensitive to a lethal dose of LPS. Mice were injected intraperitoneally with 20 mg/kg of LPS, and the percentage of surviving mice was determined daily for six consecutive days. Cav-2-/- mice (circles) exhibited significantly accelerated mortality compared to WT (squares) and Cav-1-/- mice (triangles) (**p = 0.003). Each genotype group was composed of ten mice. This graph shows the result of one representative experiment out of three independent experiments.
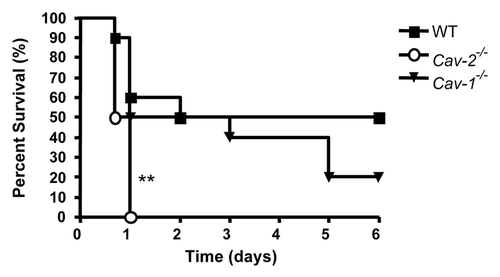
Figure 2 Cav-2-/- mice exhibit more tissue damage than WT mice in the ileum after LPS injection. (A) Groups of five mice for each genotype were intraperitoneally injected with saline or LPS (20 mg/kg). Organs were processed for histological analysis, and stained with hematoxilin and eosin. LPS-induced damage was observed in the ileum, characterized by shortened and distorted villi (arrows). (B) Damage was quantified by measuring the ratio of villi length over crypt length. LPS induced shortening and distortion of villi in WT and Cav-2-/- mice. This effect was more prominent in Cav-2-/- mice than wild-type mice (*p < 0.05).
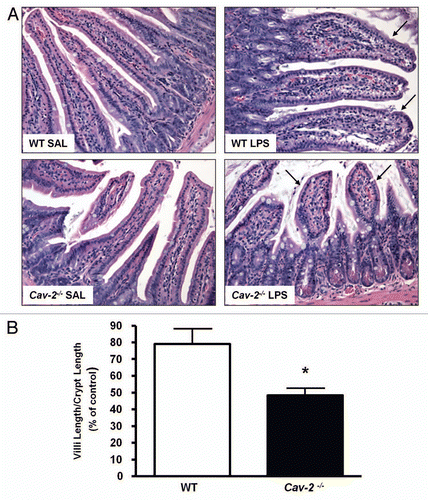
Figure 3 Ileum mucosal barrier in Cav-2-/- mice remains intact after LPS injection. Mice from each genotype were intraperitoneally injected with LPS (20 mg/kg). Instestine were processed for electron microscopy analysis. Despite increased permeability in the ileum of Cav-2-/- mice, tight junctions (arrows) and desmosomes (arrowheads) of these cells remained intact (arrows).
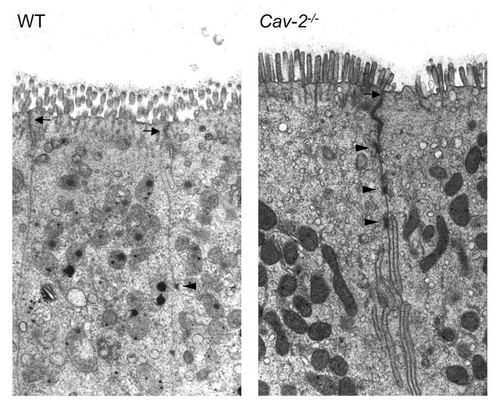
Figure 4 Epithelial cells of Cav-2-/- mice ileum show increased cytoplasmic fluid accumulation. Portions of the ileum of mice from each mouse injected with saline (SAL) or LPS (20 mg/kg i.p.) were collected and processed for electron microscopy. Representative fields of (A) WT SAL, (B) Cav-2-/- SAL, (C) WT LPS and (D) Cav-2-/- LPS are shown. Cav-2-/- mice exhibited increased fluid accumulation in epithelial cell cytoplasm of the ileum (arrows).
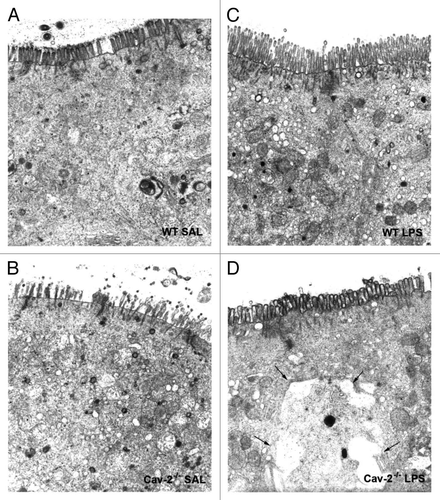
Figure 5 Cav-2-/- epithelial cells of the ileum exhibit swollen mitochondria. Portions of the ileum of mice from each mouse injected with LPS (20 mg/kg i.p.) were collected and processed for electron microscopy. Injection of LPS induced mitochondria swelling, an effect that was more prominent in (B) Cav-2-/- mice (arrows) compared to (A) WT mice (arrowheads).
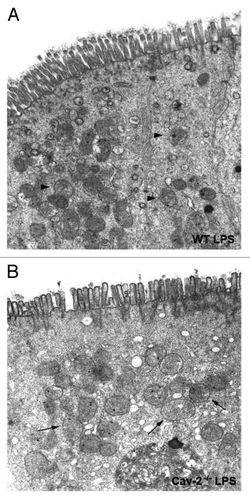
Figure 6 Cav-2-/- mice exhibit increased intestinal permeability. Cav-2-/- and WT mice were injected intraperitoneally with saline or LPS (20 mg/kg). Forty-five minutes before sacrifice (12 hours after saline or LPS injection), mice were retro-orbitally injected with a solution of 1% Evans Blue diluted in HBSS. Mice were then perfused; organs were weighted and incubated in formamide for three days to elute the Evans Blue dye. Quantification of Evans blue extravasation was determined spectrophotometrically at 620 nm. (A) Liver, heart, kidney, lung and spleen of Cav-2-/- and WT mice did not exhibit significant difference in permeability. n = 17 (WT), n = 11 (Cav-1-/-) and n = 14 (Cav-2-/-) (*p < 0.05; **p < 0.01). (B) Ileum and colon of Cav-2-/- mice showed increased permeability compared to WT mice. Ileum: n = 17 (WT), n = 11 (Cav-1-/-) and n = 14 (Cav-2-/-); Colon: n = 15 (WT), n = 9 (Cav-1-/-) and n = 13 (Cav-2-/-).
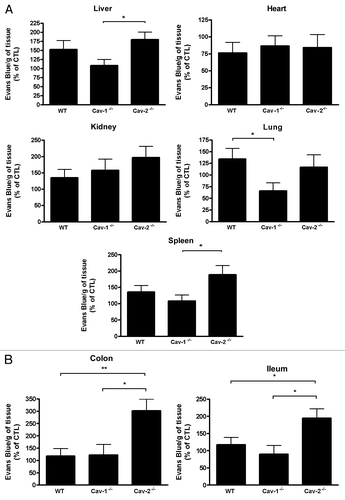
Figure 7 NO levels in the peritoneal lavage correlate with the permeability levels in WT and Cav-2-/- mice. Twelve hours after saline or LPS injection, HBSS was used to wash the peritoneal cavity of Cav-1-/-, Cav-2-/- or WT mice. The peritoneal lavage was submitted to a NO assay. Each group was composed of 6–7 mice. Cav-2-/- mice showed increased levels of NO in the peritoneal lavage compared to WT mice (*p < 0.05). Basal values: WT-22.60 ± 5.67 µM, Cav-1-/--20.89 ± 4.42 µM, Cav-2-/--26.60 ± 4.67 µM.
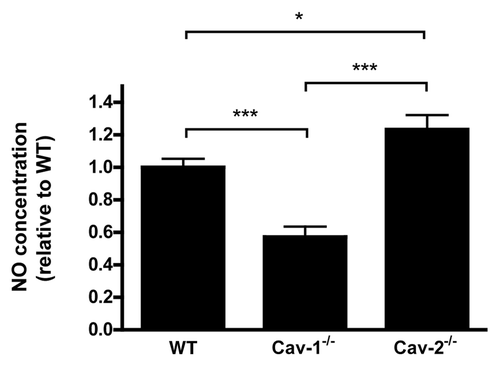
Figure 8 LPS-treated Cav-2-/- mice exhibit increased iNOS expression levels compared to LPS-treated WT and Cav-1-/- mice. (A) iNOS expression was detected by immunoblot of colon lysates obtained from mice injected with saline (SAL) or LPS (20 mg/kg) using a specific antibody for iNOS. (B) Densitometry analysis of the bands detected with iNOS antibody were performed with the Image J analysis software. Each group was composed of five mice. Results of LPS-treated WT were considered to be 100%.
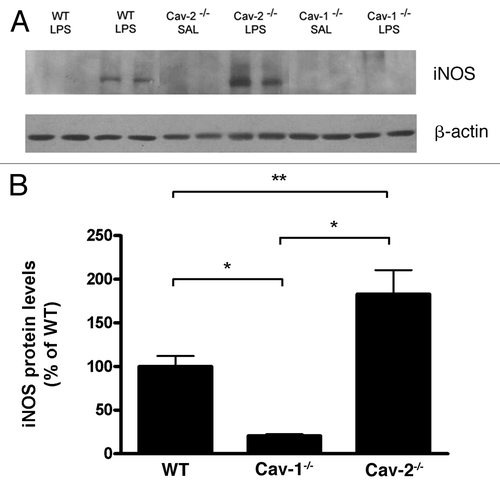
Figure 9 LPS-treated Cav-2-/- mice exhibit increased levels of tyrosinephosphorylated STAT1 compared to LPS-treated WT and Cav-1-/- mice. (A) The level of tyrosine-phosphorylated STAT1 (Tyr701) and total STAT1 were detected by immunoblot of colon lysates obtained from mice injected with saline (SAL) or LPS (20 mg/kg). (B) Densitometry analysis of the bands detected with iNOS antibody were performed with the Image J analysis software. Each group was composed of five mice. Results of LPS-treated WT were considered to be 100%.
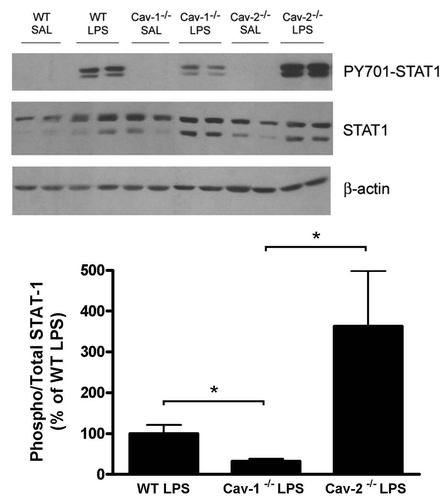
Table 1 Cav-2-/- and WT mice produce similar levels of cytokines and chemokines upon challenge with LPS
Acknowledgments
C.J.A. was supported by a Fogarty International Training Grant (1D43TW007129). M.P.L. and his laboratory were supported by grants from the NIH/NCI (R01-CA-080250; R01-CA-098779; R01-CA-120876; R01-AR-055660), and the Susan G. Komen Breast Cancer Foundation. Funds were also contributed by the Margaret Q. Landenberger Research Foundation (to M.P.L.). P.G.F. is supported by grants from the Jane Barsumian/Mary Lyons Trust, and the Susan G. Komen Foundation. J.F.J. is supported by grants from the American Lung Association and the Susan G. Komen Foundation.
References
- Wang XM, Kim HP, Song R, Choi AM. Caveolin-1 confers antiinflammatory effects in murine macrophages via the MKK3/p38 MAPK pathway. Am J Respir Cell Mol Biol 2006; 34:434 - 442
- Scherer PE, Lewis RY, Volonte D, Engelman JA, Galbiati F, Couet J, et al. Cell-type and tissue-specific expression of caveolin-2. Caveolins 1 and 2 co-localize and form a stable hetero-oligomeric complex in vivo. J Biol Chem 1997; 272:29337 - 29346
- Couet J, Li S, Okamoto T, Ikezu T, Lisanti MP. Identification of peptide and protein ligands for the caveolin-scaffolding domain. Implications for the interaction of caveolin with caveolae-associated proteins. J Biol Chem 1997; 272:6525 - 6533
- Park DS, Lee H, Frank PG, Razani B, Nguyen AV, Parlow AF, et al. Caveolin-1-deficient mice show accelerated mammary gland development during pregnancy, premature lactation and hyperactivation of the Jak-2/STAT5a signaling cascade. Mol Biol Cell 2002; 13:3416 - 3430
- Cohen AW, Hnasko R, Schubert W, Lisanti MP. Role of caveolae and caveolins in health and disease. Physiol Rev 2004; 84:1341 - 1379
- Breuza L, Corby S, Arsanto JP, Delgrossi MH, Scheiffele P, Le Bivic A. The scaffolding domain of caveolin 2 is responsible for its Golgi localization in Caco-2 cells. J Cell Sci 2002; 115:4457 - 4467
- Fujimoto T, Kogo H, Nomura R, Une T. Isoforms of caveolin-1 and caveolar structure. J Cell Sci 2000; 113:3509 - 3517
- Sowa G, Pypaert M, Fulton D, Sessa WC. The phosphorylation of caveolin-2 on serines 23 and 36 modulates caveolin-1-dependent caveolae formation. Proc Natl Acad Sci USA 2003; 100:6511 - 6516
- Shmuel M, Nodel-Berner E, Hyman T, Rouvinski A, Altschuler Y. Caveolin 2 regulates endocytosis and trafficking of the M1 muscarinic receptor in MDCK epithelial cells. Mol Biol Cell 2007; 18:1570 - 1585
- Garrean S, Gao XP, Brovkovych V, Shimizu J, Zhao YY, Vogel SM, et al. Caveolin-1 regulates NFkappaB activation and lung inflammatory response to sepsis induced by lipopolysaccharide. J Immunol 2006; 177:4853 - 4860
- Medina FA, de Almeida CJ, Dew E, Li J, Bonuccelli G, Williams TM, et al. Caveolin-1-deficient mice show defects in innate immunity and inflammatory immune response during Salmonella enterica serovar Typhimurium infection. Infect Immun 2006; 74:6665 - 6674
- Moore KJ, Andersson LP, Ingalls RR, Monks BG, Li R, Arnaout MA, et al. Divergent response to LPS and bacteria in CD14-deficient murine macrophages. J Immunol 2000; 165:4272 - 4280
- Lei MG, Morrison DC. Differential expression of caveolin-1 in lipopolysaccharide-activated murine macrophages. Infect Immun 2000; 68:5084 - 5089
- Lei MG, Tan X, Qureshi N, Morrison DC. Regulation of cellular caveolin-1 protein expression in murine macrophages by microbial products. Infect Immun 2005; 73:8136 - 8143
- Wang XM, Kim HP, Nakahira K, Ryter SW, Choi AM. The heme oxygenase-1/carbon monoxide pathway suppresses TLR4 signaling by regulating the interaction of TLR4 with caveolin-1. J Immunol 2009; 182:3809 - 3818
- Razani B, Engelman JA, Wang XB, Schubert W, Zhang XL, Marks CB, et al. Caveolin-1 null mice are viable but show evidence of hyperproliferative and vascular abnormalities. J Biol Chem 2001; 276:38121 - 38138
- Boughton-Smith NK, Evans SM, Hawkey CJ, Cole AT, Balsitis M, Whittle BJ, et al. Nitric oxide synthase activity in ulcerative colitis and Crohn's disease. Lancet 1993; 342:338 - 340
- Unno N, Wang H, Menconi MJ, Tytgat SH, Larkin V, Smith M, et al. Inhibition of inducible nitric oxide synthase ameliorates endotoxin-induced gut mucosal barrier dysfunction in rats. Gastroenterology 1997; 113:1246 - 1257
- Forsythe RM, Xu DZ, Lu Q, Deitch EA. Lipopolysaccharide-induced enterocyte-derived nitric oxide induces intestinal monolayer permeability in an autocrine fashion. Shock 2002; 17:180 - 184
- Vallance BA, Dijkstra G, Qiu B, van der Waaij LA, van Goor H, Jansen PL, et al. Relative contributions of NOS isoforms during experimental colitis: endothelial-derived NOS maintains mucosal integrity. Am J Physiol Gastrointest Liver Physiol 2004; 287:865 - 874
- Kubes P, McCafferty DM. Nitric oxide and intestinal inflammation. Am J Med 2000; 109:150 - 158
- Gao JJ, Filla MB, Fultz MJ, Vogel SN, Russell SW, Murphy WJ. Autocrine/paracrine IFN-alphabeta mediates the lipopolysaccharide-induced activation of transcription factor Stat1alpha in mouse macrophages: pivotal role of Stat1alpha in induction of the inducible nitric oxide synthase gene. J Immunol 1998; 161:4803 - 4810
- Jacobs AT, Ignarro LJ. Lipopolysaccharide-induced expression of interferon-beta mediates the timing of inducible nitric-oxide synthase induction in RAW 264.7 macrophages. J Biol Chem 2001; 276:47950 - 47957
- Albrecht EW, Stegeman CA, Heeringa P, Henning RH, van Goor H. Protective role of endothelial nitric oxide synthase. J Pathol 2003; 199:8 - 17
- Hokari R, Kato S, Matsuzaki K, Kuroki M, Iwai A, Kawaguchi A, et al. Reduced sensitivity of inducible nitric oxide synthase-deficient mice to chronic colitis. Free Radic Biol Med 2001; 31:153 - 163
- Palatka K, Serfozo Z, Vereb Z, Hargitay Z, Lontay B, Erdodi F, et al. Changes in the expression and distribution of the inducible and endothelial nitric oxide synthase in mucosal biopsy specimens of inflammatory bowel disease. Scand J Gastroenterol 2005; 40:670 - 680
- Guo FH, Comhair SA, Zheng S, Dweik RA, Eissa NT, Thomassen MJ, et al. Molecular mechanisms of increased nitric oxide (NO) in asthma: evidence for transcriptional and post-translational regulation of NO synthesis. J Immunol 2000; 164:5970 - 5980
- Ohmori Y, Hamilton TA. Requirement for STAT1 in LPS-induced gene expression in macrophages. J Leukoc Biol 2001; 69:598 - 604
- Samardzic T, Jankovic V, Stosic-Grujicic S, Trajkovic V. STAT1 is required for iNOS activation, but not IL-6 production in murine fibroblasts. Cytokine 2001; 13:179 - 182
- Stempelj M, Kedinger M, Augenlicht L, Klampfer L. Essential role of the JAK/STAT1 signaling pathway in the expression of inducible nitric-oxide synthase in intestinal epithelial cells and its regulation by butyrate. J Biol Chem 2007; 282:9797 - 9804
- Connelly L, Palacios-Callender M, Ameixa C, Moncada S, Hobbs AJ. Biphasic regulation of NFkappaB activity underlies the pro- and anti-inflammatory actions of nitric oxide. J Immunol 2001; 166:3873 - 3881
- Michel JB, Feron O, Sacks D, Michel T. Reciprocal regulation of endothelial nitric-oxide synthase by Ca2+-calmodulin and caveolin. J Biol Chem 1997; 272:15583 - 15586
- Feron O, Dessy C, Moniotte S, Desager JP, Balligand JL. Hypercholesterolemia decreases nitric oxide production by promoting the interaction of caveolin and endothelial nitric oxide synthase. J Clin Invest 1999; 103:897 - 905
- Bucci M, Gratton JP, Rudic RD, Acevedo L, Roviezzo F, Cirino G, et al. In vivo delivery of the caveolin-1 scaffolding domain inhibits nitric oxide synthesis and reduces inflammation. Nat Med 2000; 6:1362 - 1367
- Shah M, Patel K, Fried VA, Sehgal PB. Interactions of STAT3 with caveolin-1 and heat shock protein 90 in plasma membrane raft and cytosolic complexes. Preservation of cytokine signaling during fever. J Biol Chem 2002; 277:45662 - 45669