Abstract
BRCA1 C-terminal (BRCT) domains are integral signaling modules in the DNA damage response (DDR). Aside from their established roles as phospho-peptide binding modules, BRCT domains have been implicated in phosphorylation-independent protein interactions, DNA binding and poly(ADP-ribose) (PAR) binding. These numerous functions can be attributed to the diversity in BRCT domain structure and architecture, where domains can exist as isolated single domains or assemble into higher order homo- or hetero- domain complexes. In this review, we incorporate recent structural and biochemical studies to demonstrate how structural features allow single and tandem BRCT domains to attain a high degree of functional diversity.
BRCT Domains in DNA Damage Response
The DNA damage response (DDR) is an elaborate signaling network that orchestrates the cellular response to maintain genomic integrity. Such signaling cascades rely heavily on protein phosphorylation by serine/threonine kinases that include the phosphoinositide-3-kinase-related kinases (PIKKs) (ATM, ATR, DNA-PK)Citation1,Citation2 and checkpoint effector kinases (Chk1, Chk2 and MK2).Citation3 Phosphorylated proteins are recognized by specialized 14-3-3, forkhead associated (FHA) and BRCA1 C-terminal (BRCT) domains present in downstream DDR proteins. Interestingly, these phospho-peptide binding modules commonly match the conserved phospho-peptide motifs targeted by their cognate kinases. 14-3-3 domains preferentially bind the targets of checkpoint kinases, while the BRCT and FHA domains can bind to the phosphorylated targets of ATM/ATR.Citation4 While the kinases involved show little or no substrate specificity for serine or threonine residues, the binding modules can discriminate between their phospho-threonine or phospho-serine targets. For example, FHA domains show profound specificity for pT-peptides, while certain BRCT domains show specificity for pS-peptides.
The BRCT domain was originally identified in the tumor suppressor protein, Breast cancer associated 1 (BRCA1). Truncation and missense mutations in this region correlate with an increased risk for breast and ovarian cancers, underscoring its importance in the tumor suppressor function of BRCA1.Citation5,Citation6 Subsequent bioinformatics analysis revealed that BRCT domains exist in a myriad of proteins, most of which have roles in DNA metabolism and repair.Citation7,Citation8 The conserved phospho-peptide binding function was later identified in BRCA1 and other tandem BRCT domains, as well as various single BRCT domains.Citation9,Citation10 For example, the tandem BRCT domain pair in BRCA1 recognizes the phosphorylated peptide motif pS-P-T-F in various protein partners such as BRCA1-associated C-terminal helicase/Fanconi anemia group J protein (BACH1/FANCJ),Citation9,Citation10 CtIPCitation11 and Abraxas/Coiled-coil domain-containing protein 98 (CCDC98),Citation12–Citation14 and the ability of BRCA1 to recognize different binding partners in DDR signaling regulates BRCA1 recruitment and function.
It is now clear that BRCT domains possess an array of activities other than phosphorylated protein interactions. Besides the conserved phospho-peptide recognition exhibited by tandem BRCT domains, BRCT domains have also been implicated in phosphorylation-independent protein interactions, DNA binding and poly(ADP-ribose) (PAR) binding. This diversity can be credited not only to variations in sequence and structure within a single BRCT domain, but also to the unique ability of BRCT domains to assemble as multi-domain complexes with other BRCT domains or even other functional domains, adding another level of complexity, specificity and regulation. In light of structural and biochemical findings in recent years, we analyze the structural aspects of single and tandem BRCT domains that contribute to their functional versatility in DDR signaling.
BRCT Domain Structure and Assembly
The BRCT domain fold was first revealed in the crystal structure of the X-ray repair cross-complementing protein 1 (XRCC1) N-terminal BRCT domain.Citation15 The fold is comprised of a central 4-stranded β-sheet flanked by a single α-helix (α2) on one side and two α-helices (α1 and α3) on the opposite side. Comparison of various BRCT domain structures illustrate that deviations in structure and sequence are mainly localized to the connecting loops, whereas conserved residues are situated in the hydrophobic core and in residues involved in recognizing the phosphorylated amino acid in phospho-peptide targets.Citation16,Citation17
Interestingly, the domain architecture of BRCT domains is remarkably diverse. BRCT domains range from isolated individual domains to multiple tandem BRCT repeats, or even as fusions with other functional domains (). Single BRCT domains represent a large class of BRCT domains that exist as single copies in proteins such as Poly(ADP-ribose) polymerase 1 (PARP-1) and DNA Ligase III (Lig3). They can also be found in multiple but isolated copies, such as in XRCC1, where two distinct single BRCT domains are separated by 135 amino acids in sequence. In tandem BRCT repeats, multiple BRCT domains are separated by a variable linker region. The initial crystal structure of the tandem BRCT domains in BRCA1 shed light on the canonical BRCT-BRCT domain packing, which occurs through a hydrophobic interface consisting of the α2 helix of the N-terminal BRCT and α1′ and α3′ helices of the C-terminal BRCT.Citation18 Mutations at this hydrophobic interface in BRCA1 (M1775R and A1708E) are linked to breast and ovarian cancer, highlighting the requirement for an intact tandem BRCT interface for normal function.Citation5,Citation6 This conserved BRCT-BRCT domain packing has since been observed in numerous BRCT repeat structures such as in Mediator of DNA damage checkpoint protein 1 (MDC1), BRCA1-associated RING domain protein 1 (BARD1), Topoisomerase IIβ binding protein 1 (TopBP1) BRCT7/8, S. pombe Crb2 and S. pombe Brc1, and their functional role in phospho-peptide binding is well established.Citation19–Citation25 However, variations in tandem BRCT domain structure also exist. For example, the tandem BRCT domains of DNA Ligase IV (LigIV) do not pack together and are separated by a significantly longer inter-BRCT linker, which ultimately ensures its unique mode of recognition for X-ray repair cross-complementing protein 4 (XRCC4).Citation26–Citation28 Recently, a triple BRCT domain module was confirmed in TopBP1 BRCT0/1/2, suggesting even higher order of tandem BRCT domain architecture. Interestingly, the packing of BRCT0/BRCT1 and BRCT1/BRCT2 at the interface are significantly different from canonical tandem BRCT domains.Citation29,Citation30 This unconventional BRCT domain interface is likely a consequence of the shorter inter-BRCT linker regions, suggesting a role for the inter-BRCT linker in driving the packing of tandem BRCT domains. Whereas longer linker lengths ranging from 30–60 amino acids permit the parallel juxtaposition characteristic of canonical tandem BRCT domain packing, shorter linkers restrict the packing of adjacent BRCT repeats such that they interact in a twisted orientation.
The diversity in BRCT domain architecture extends to other functional domains, although the significance of these hetero-domain modules is still unclear. A single BRCT domain is packed alongside an N-terminal fibronectin type III (FN3) domain in S. cerevisiae Chs5p, a component of the exomer coat complex. Recent studies in yeast suggest that both the FN3 and BRCT domain act as a single module and are both required for localization and cargo delivery function.Citation31 The tandem BRCT repeats in the DNA double-stranded break repair protein Nijmegen breakage syndrome protein 1 (NBS1) are coupled to an N-terminal FHA domain, another phospho-peptide binding domain.Citation32,Citation33 This unique FHA-BRCT-BRCT domain setup may present an intriguing platform for cross-talk between the two adjacent functional phospho-peptide interacting modules.
Phosphorylation-Dependent Interactions
Since the original discovery of single and tandem BRCT domains as phospho-peptide binding modules,Citation9,Citation10 the ability for tandem BRCT domains to recognize phospho-peptides has been well characterized, both functionally and structurally, in a number of proteins. However, the basis of phospho-peptide recognition by single BRCT domains remains unclear.
Single BRCT domains.
The implication of phospho-peptide binding mediated by single BRCT domains was initially demonstrated in vitro using a degenerate peptide library. Using single BRCT domains in Terminal deoxynucleotidyl transferase (TDT), S. cerevisiae REV1 and Lig3, Yu et al. showed that these domains preferentially interact with a phospho-peptide library compared with the non-phosphorylated counterpart and that TopBP1 BRCT6 and hFCP1 BRCT specifically binds to cognate E2F1 and RNA polymerase II phospho-peptides, respectively.Citation10 However, subsequent work on FCP1 and TopBP1 single BRCT domains provides conflicting evidence for their roles as phospho-peptide binding modules. Although studies in S. pombe and A. thaliana indicate that the FCP1 BRCT domain is important for FCP1 C-terminal domain (CTD) phosphatase activity,Citation34,Citation35 structural and biochemical data from Ghosh et al. revealed that the BRCT phosphate-binding pocket is dispensable for FCP1 function.Citation36 Rather as part of a multi-domain complex, the BRCT domain may have an indirect role in supporting critical residues in the neighboring catalytic N-terminal FCP1 homology (FCPH) domain. The interaction between TopBP1 BRCT6 and E2F1 phosphorylated at S31 represses E2F1 activity in response to DNA damage.Citation37,Citation38 Conversely, the crystal structure of TopBP1 BRCT6 suggests that it lacks a functional phosphate-binding pocket and is unlikely to foster a stable phospho-peptide interaction on its own.Citation39
Given the less extensive binding surface, one might suspect that the recognition of phospho-peptides by single BRCT domains could be weaker than tandem BRCT domains. Single BRCT domain interactions may participate in more dynamic signaling processes or may require additional contacts provided within oligomeric complexes and/or from other protein domains, to stabilize these interactions. Clearly, more structural characterization will be required to elucidate the mechanism of single BRCT domain phospho-peptide interactions.
Tandem BRCT domains.
Through biochemical and structural studies of a number of BRCT repeats with their cognate phospho-peptides, we now understand not only the conserved mode of phospho-peptide binding in BRCT repeats, but also the differences that allow tandem BRCT domains to achieve such a high degree of specificity for a wide range of phospho-peptide targets.
Crystal structures of the BRCA1, MDC1, S. pombe Brc1, S. pombe Crb2 and TopBP1 BRCT7/8 BRCT repeats bound to their respective phospho-peptides reveal a conserved bipartite recognition involving two distinct pockets: a pS/pT binding pocket in the N-terminal BRCT and a secondary specificity pocket at the BRCT-BRCT interface that generally provides selectivity for a hydrophobic residue at the +3 position in the phospho-peptide with respect to the pS ().Citation16,Citation21–Citation24,Citation40–Citation44 Two different classes of phosphate binding pockets have been identified to date. The phosphate-binding pockets of BRCA1 and MDC1 serve as prototypes for the first class, which are selective for pS (; left part). In this class, the phosphate moiety interacts with two side chains (S1655/T1898, K1702/K1936 in BRCA1/MDC1, respectively) and a main chain NH (G1656/G1899 in BRCA1/MDC1). The recent investigations of the interaction of TopBP1 BRCT7/8 with phosphorylated BACH1 revealed a second class of phosphate binding pocket with additional specificity for pThr peptide targets.Citation45 In addition to interactions that are conserved with the class I pocket that involve S1273, K1317 and the main chain NH of S1274, the phosphate group is also recognized by the side chain guanidinium of R1280 (; right part). The combination of differences in the peptide backbone orientation and coordination of the pThr residue, likely facilitated by additional interactions with the conserved TopBP1 R1280, allows incorporation of the γ-methyl group without steric hindrance in the pocket.Citation22
The conserved minimal phospho-peptide sequence bound by BRCT repeats consists of four residues (pS/pT-X-X-X), in which the +3 residue dictates the majority of the binding specificity.Citation46 The +3 residue sits in a cleft situated at the hydrophobic interface between the two BRCT domains, and conserved residues at the BRCT-BRCT interface determine the binding specificity for the +3 residue. In particular, a conserved arginine residue (R1699/R1933 in BRCA1/MDC1, respectively) has a pivotal role in recognizing the peptide main chain at the +3 position (). In BARD1, the conserved arginine is substituted for a serine residue (S616), suggesting that the phosphopeptide binding properties of BARD1 may be different.Citation20,Citation25,Citation47,Citation48 Interestingly, both BRCA1 and MDC1 have peptide targets that are internal (BACH1/CtIP/ACCA for BRCA1, MMSET for MDC1)Citation9–Citation11,Citation49,Citation50 or terminate (Abraxas for BRCA1, γH2AX for MDC1)Citation51,Citation52 at the +3 position. Peptide binding studies have shown that the presence of a C-terminal carboxylate increases binding affinity to BRCA1 and MDC1, although the differences between the carboxy and amidated phospho-peptides are more profound for MDC1.Citation19,Citation24,Citation46 Comparison of the peptide-bound structures suggests that positioning of the +3 side chain determines the angle of incidence of the C-terminal carboxylate, which is more favorable in MDC1, and thereby tunes the degree of preference for the carboxylate.Citation46
Alterations in the hydrophobic packing interface between BRCT repeats may also present a means to alter phosphopeptide specificity. Due to the extent of hydrophobic interactions between α2-α1′-α3′ helices, the BRCT domain pair is typically held rigid and only show small deviations in domain movement upon peptide binding. This is perhaps reflected in the absolute conservation of the cognate phospho-peptide motif sequence for BRCA1, where all validated binding partners contain the consensus motif, pS-P-T-F.Citation6,Citation9–Citation11,Citation13,Citation14,Citation49,Citation52 In contrast, the significantly smaller hydrophobic interface in TopBP1 BRCT7/8 allows for a dramatic domain rotation (23°) at the BRCT-BRCT interface upon phospho-peptide binding in order to shape the larger +3/+4 pocket, as well as the phosphate-binding pocket to a lesser extent.Citation22 Since TopBP1 BRCT7/8 has also been implicated in binding another TopBP1 phospho-peptide motif with a divergent sequence (pS-N-L-Q-W-P-S),Citation38 the plasticity at the BRCT-BRCT interface in TopBP1 BRCT7/8 could potentially cater to a larger variety of peptide targets.
In another layer of complexity, tandem BRCT domain phospho-peptide binding may also be further regulated by interactions with neighboring domains, such as in the NBS1 FHA-BRCT-BRCT hetero-domain module. Structural studies of S. pombe NBS1 with the Ctp1 phospho-peptide reveal a structural rearrangement in the tandem BRCT domain interface, reminiscent of TopBP1 BRCT7/8 but in an orthogonal direction.Citation33 The NBS1 conformational change is triggered by an arginine switch located at the FHA-BRCT1 domain interface that is activated upon FHA domain binding to its phospho-peptide. Since both the NBS1 FHA domain and BRCT repeat are capable of binding to the phosphorylated MDC1 SDT motifs,Citation32,Citation53–Citation56 interaction from the FHA domain may regulate the phospho-peptide binding ability of the adjacent BRCT repeat by initiating a tandem BRCT domain structural rearrangement.
There is also the rare scenario where the putative phosphate-binding pocket exists in the C-terminal BRCT rather than the N-terminal BRCT domain of a BRCT repeat, such as in TopBP1 BRCT4/5 and PTIP BRCT1/2. TopBP1 BRCT5 is required for TopBP1 localization to IR-induced fociCitation57 and interacts with phosphorylated SDTD motifs in MDC1 at stalled replication forks.Citation58 In addition, TopBP1 BRCT4/5 has been shown to bind 53BP1, an interaction that is important for 53BP1-mediated checkpoint control in response to DSBs in G1.Citation59 It is likely that the phosphatebinding pocket in BRCT5 is involved in these interactions. However, the absence of a downstream +3 hydrophobic pocket implies a smaller peptide binding surface and potentially a weaker interaction compared with canonical tandem BRCT domain interactions.
The structure of the N-terminal region of TopBP1 shows an unexpected packing of BRCT1/2 with another BRCT domain at the N-terminus, forming a triple BRCT repeat module. This region interacts with Rad9, a component of the trimeric Rad9-Hus1-Rad1 (9-1-1) clamp, which is a prerequisite for stimulating ATR activity and subsequent DNA replication checkpoint activation.Citation60,Citation61 Interestingly, both BRCT1 and BRCT2 contain putative phosphate-binding pockets, but peptide binding data suggests that only the BRCT1 pocket is responsible for the phosphorylation-dependent interaction with Rad9.Citation29,Citation30 The presence of a distal functional phosphate-binding pocket in BRCT2, as indicated by the coordination of a sulfate ion in the crystal structure,Citation29 may imply an intriguing basis for the recognition of other phospho-peptide targets for TopBP1 BRCT0/1/2. In addition, deletion of BRCT0 only slightly decreases binding to the Rad9 phospho-peptide, suggesting that BRCT0 may only have a minor role in phospho-peptide interactions.Citation30 Indeed, further work will be needed to elucidate the potential significance of BRCT0 in the triple BRCT domain.
Phosphorylation-Independent Protein Interactions
Single BRCT domains.
Single BRCT domains have shown the ability to interact with single BRCT domains in other proteins via interfaces that are distinct from the hydrophobic BRCT-BRCT interface in tandem BRCT domains. Perhaps the best examples are in interactions involving the two single BRCT domains in XRCC1. The interaction between the C-terminal BRCT (BRCT2) of XRCC1 and Lig3 BRCT domain is well established in short-patch base excision repair.Citation62–Citation65 Although a crystal structure is unavailable (see Note added in proof), models have been proposed for the XRCC1-Lig3 complex based on a number of studies. A combination of the homodimer crystal structure of XRCC1 BRCT2 and mutational analysis of key residues in α1 helix provides a structural model for a dimerization interface.Citation15,Citation66 However, it remains unclear whether this interface is responsible for homo or hetero-dimerization and other data support a hetero-tetrameric complex, comprising of a homodimer of XRCC1 BRCT2 interacting with a homodimer of Lig3 BRCT domains.Citation64,Citation67,Citation68 A less characterized single BRCT-BRCT interaction involves the N-terminal BRCT of XRCC1 (BRCT1) and PARP-1 BRCT, which negatively regulates PARP-1 activity.Citation67,Citation69 Like XRCC1 BRCT1, TopBP1 BRCT6 also interacts with the PARP-1 BRCT domain,Citation70 providing evidence for a common mode of interaction mediated by the BRCT domain of PARP-1. The recent crystal structure of the N-terminal BRCT domain of Microcephalin (MCPH1/BRIT1) provides insight for another potential protein-protein interaction surface that is required for MCPH1 BRCT1-dependent centrosomal localization and prevention of abnormal chromosome condensation.Citation71,Citation72 The conserved phosphate-binding residues (S1655 and K1702 in BRCA1) are absent in MCPH1 (E14 and T59, respectively) and the pocket is instead replaced by a larger, more acidic surface that is conserved in MCPH1 homologs. Mutations at this pocket also correlate to defective regulation of chromosomal condensation. Thus, the distinct surface that substitutes for the phosphate-binding pocket in MCPH1 BRCT1 may represent another contact surface for protein-protein interactions mediated by single BRCT domains.
Tandem BRCT domains.
Tandem BRCT domains can also interact with other proteins independent of phosphorylation. As demonstrated in crystal structures involving the BRCT repeats of LigIV, S. pombe Crb2 and 53BP1 in complex with their respective protein binding partners, a common theme observed in each of these interactions is the presence of a β-hairpin motif in the inter-BRCT linker, which is responsible for extensive contacts at the protein binding interface.Citation73
Interactions between the 53BP1 BRCT repeats and the p53 DNA binding domain (DBD) enhance the transactivation potential of p53.Citation74–Citation76 In the 53BP1 BRCT1/2-p53 DBD complex crystal structure, the 53BP1 BRCT-BRCT linker adopts an extended β-hairpin loop that, together with the α4 helix, contacts the conserved L2 and L3 loops of the p53 DBD ().Citation77,Citation78 A distal salt bridge between the 53BP1 BRCT1 α3 helix residue D1833 and p53 R181 also makes a minor contribution to the overall binding interface.
The S. pombe Crb2 checkpoint function relies on the BRCT repeat-mediated homodimerization of Crb2.Citation79,Citation80 Biochemical and structural evidence for Crb2 BRCT1/2 further confirms the oligomeric status of the Crb2 tandem BRCT domains.Citation21 At the interface of the Crb2 dimer, the extended β-hairpin loop of the Crb2 BRCT-BRCT linker from each protomer participates in an extensive symmetrical hydrogen bonding network (). Mutations in the β-hairpin (S666R and C663R) also confer checkpoint functional defects,Citation81,Citation82 which further supports the biological role of Crb2 homodimerization.
The LigIV-XRCC4 interaction is critical for non-homologous end joining (NHEJ) and in V(D)J recombination.Citation83–Citation86 Interestingly, the tandem BRCT domains of LigIV contain an unusually long linker region, and packing of the tandem BRCT domains is not observed. The crystal structures of the human and yeast ortholog of XRCC4 in complex with the tandem BRCT domains of LigIV provide a possible rationale for the unique LigIV BRCT repeat structure.Citation26–Citation28 Besides contacts mediated by the β-hairpin of the linker region in LigIV and α1 helix of LigIV BRCT2 on opposite ends of the coiled-coil tails of the XRCC4 homodimer, the extended LigIV linker forms a distinct helix-loop-helix (HLH) clamp structure that wraps around the linear XRCC4 coiled-coil tails to stabilize the interaction ().
The inter-BRCT domain linker has evolved a role in facilitating protein-protein interactions in addition to its role in dictating the orientation and packing of tandem BRCT repeats. Whereas shorter linker lengths affect the overall tandem BRCT domain structure by restricting the BRCT packing orientation, as evident in the TopBP1 BRCT0/1/2 structure,Citation29,Citation30 the flexibility of longer linkers to form common structure motifs, such as a β-hairpin and/or HLH, can be utilized for protein-protein interactions.
DNA Binding
Although DNA-binding activity has been proposed for a number of different single and tandem BRCT domains, there is still uncertainty as to the specificity of these interactions for different types of DNA structures and their relevance to the biological functions of BRCT proteins. Recent biochemical and structural studies of the replication factor C (RFC) BRCT domain have provided molecular insight into how a subgroup of single BRCT domains are selective for DNA ends.
Single BRCT domains.
The initial studies on the DNA binding activity of BRCT domains by Yamane et al.Citation87,Citation88 demonstrated that the single BRCT domains in TopBP1 and XRCC1 can bind DNA strand breaks and termini in vitro, although the relevance of these interactions to the cellular function of TopBP1 and XRCC1 is still unclear. In contrast, significant progress has been made to characterize the DNA binding activity in the BRCT domain of Replication factor C (RFC) p140, a subunit of the five protein RFC complex that functions in DNA replication and repair.Citation89–Citation91 The RFC BRCT domain selectively recognizes the 5′-phosphate DNA terminus within a 3′ overhanging DNA end.Citation92,Citation93 Structural investigations by NMR spectroscopy shed light on the mechanism of DNA recognition, and involves not only the phosphate-binding pocket but an α-helix N-terminal to the BRCT domain that inserts into the major groove of the DNA ( and B).Citation93,Citation94 Analogous to the recognition of phosphopeptides, conserved residues in the phosphate-binding pocket of RFC (T415, G416, K458, R423) coordinate the recessed 5′-phosphate (). These conserved residues are also absolutely conserved with the BRCT domain of the bacterial NAD+-dependent DNA ligase (LigA), which is highly similar to RFC (53% identity) and constitutes a distinct BRCT subgroup with RFC and PARP-1.Citation7 As in RFC, the BRCT domain of LigA has DNA binding activity and mutations in these conserved residues (T599, G600) markedly reduce DNA binding and ligation activity.Citation95,Citation96 In light of this evidence, the mechanism of DNA recognition by the RFC BRCT domain may represent a conserved mode of DNA recognition for this subset of single BRCT domains.
Tandem BRCT domains.
As with the single BRCT domains of TopBP1 and XRCC1, tandem BRCT domains in BRCA1 and TopBP1 can also bind DNA strand breaks and ends in vitro.Citation87,Citation88 In the case of BRCA1, BRCT repeat-mediated DNA-binding activity is enhanced by formation of the BRCA1-BARD1 heterodimer. 97 Interestingly, TopBP1 BRCT7/8 also displays unique specificity for damaged DNA containing bulky lesions. Using an in vitro partially reconstituted checkpoint system, Choi et al. demonstrated that a C-terminal fragment of TopBP1, containing BRCT7/8 and the ATR-activation domain (AAD), preferentially binds benzo[a]pyrene diol epoxide (BPDE)-treated DNA and stimulates ATR activity,Citation98 which is critical for DNA replication checkpoint activation in response to DNA replication stress. TopBP1 BRCT7/8 binding also favors longer stretches of DNA and, contrary to the previous studies by Yamane et al.Citation87,Citation88 is not specific for DNA ends. The implication of BRCT domains directly detecting damaged DNA offers intriguing possibilities that proteins with BRCT domains can act as DNA damage sensors in addition to transducers of DDR signaling.
Poly(ADP-Ribose) Binding
Single BRCT domains.
Although still poorly understood, single BRCT domains have been implicated in binding poly(ADP-ribose) (PAR), a post-translational modification catalyzed by poly(ADP-ribose) polymerases in DDR signaling. A consensus PAR-binding motif is found in TopBP1 BRCT6 and the N-terminal BRCT of XRCC1,Citation99 although there is more evidence supporting XRCC1 BRCT1 as a validated PAR-binding module. XRCC1 BRCT1 preferentially recognizes ribosylated PARP-1 and a peptide of the XRCC1 PAR-binding sequence binds to PAR in vitro.Citation69,Citation99–Citation102 In contrast, the PAR?binding activity of XRCC1 does not appear to be conserved in TopBP1, as indicated by the absence of PAR-binding by TopBP1 BRCT6 in vitro.Citation39 Further comparison of the structures of TopBP1 BRCT6 and XRCC1 BRCT1 reveal that their respective PAR-binding motifs are not structurally conserved and that the PAR-binding motif in TopBP1 BRCT6 is partially buried in the protein core ().Citation39
Conclusions
BRCT domains have proven to be remarkably versatile binding modules. Starting from the single BRCT domain building block, the ability to incorporate different functions by varying or adding different structural features grants unprecedented advantages to establish regulation and specificity for various targets such as phospho-peptides, proteins, DNA and PAR (). Although the phospho-peptide and phosphorylation-independent protein binding functions of tandem BRCT domains has been well characterized both structurally and functionally, less is clear about the particular role of single BRCT domains, aside from the DNA binding activity of the RFC BRCT domain. Indeed, further work is needed to elucidate the mechanisms underlying the multitude of functions associated with BRCT domains.
Note added in proof
The structural basis for XRCC1-Lig3 BRCT interactions has recently been revealed in a crystallographic study (Cuneo MJ, Gabel SA, Krahn JM, Ricker MA, London RE. The structural basis for partitioning of the XRCC1/DNA ligase III-a BRCT-mediated dimer complexes. Nucleic Acids Res 2011; In press; PMID: 21652643.
Figures and Tables
Figure 1 Phospho-peptide binding of tandem BRCT domains. (A) Conserved phospho-peptide recognition of BRCA1 BRCT1/2 consists of a phosphate-binding pocket (blue) and +3 binding pocket (brown). The surface representation of BRCA1 BRCT1/2 is shown and the BACH1 peptide pS and +3 side chains are represented as sticks and labeled. (B) pS coordination by BRCA1 (blue) and MDC1 (beige) (left part) and pT coordination by TopBP1 BRCT7/8 (right part). Phosphate contact residues for BRCA1/MDC1 (top/bottom) and TopBP1 are labeled. Equivalent hydrogen bonding and electrostatic interactions are indicated by black dotted lines and additional contacts by TopBP1 R1280 are shown as red dotted lines. (C) +3 phospho-peptide main chain recognition at the BRCT-BRCT interface in BRCA1 (blue), MDC1 (beige) and BARD1 (pink). The equivalent Arg residue in BRCA1/MDC1/BARD1 (top/middle/bottom) is labeled.
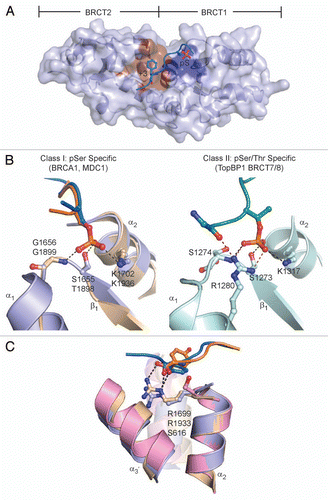
Figure 2 Tandem BRCT domain linker-mediated protein interactions. The inter-BRCT linker is shown in orange and contact regions are highlighted in red. Secondary structure motifs are labeled. (A) Structure of 53BP1 BRCT repeats (gray) in complex with p53 DBD (teal). (B) Structure of the S. pombe Crb2 homodimer. Each protomer is labeled (A and B). (C) LigIV (gray) in complex with XRCC4 homodimer (light cyan).
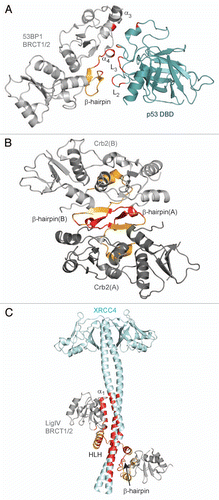
Figure 3 Model of the RFC p140 BRCT domain in complex with dsDNA. (A) Representative ensemble by Kobayashi et al.94 based on the four best structures from HADDOCK. The N-terminal α-helix is shown in purple and the 5′-phosphate is represented as a gray sphere. (B) Surface representation of RFC BRCT domain. Binding surfaces with the DNA major groove (purple) and 5′-phosphate (orange) are marked. (C) Conserved phosphate-binding pocket of RFC BRCT domain, as represented by one model in the ensemble. The 5′-phosphate group and contact residues are represented as sticks and labeled.
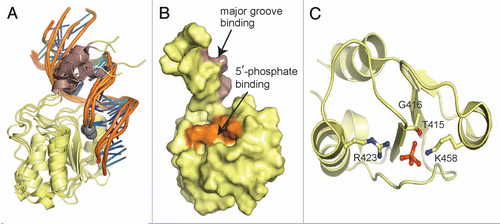
Figure 4 The consensus PAR-binding motif is not conserved in TopBP1 and XRCC1. The PAR-binding motif (cyan) is mapped in the structures of TopBP1 BRCT6 (left) and XRCC1 BRCT1 (right).
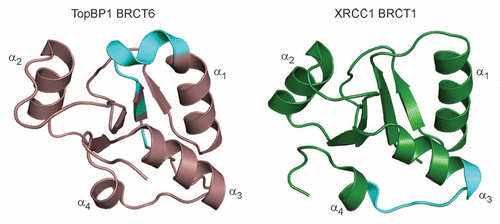
Table 1 BRCT domain architecture found in proteins
Acknowledgments
We thank members of the Glover lab for their helpful discussion and comments on the manuscript. This work was supported by grants from the Canadian Cancer Society, National Institutes of Health, and the Howard Hughes Medical Institute International Scholar Program.
References
- Shiloh Y. ATM and related protein kinases: safeguarding genome integrity. Nat Rev Cancer 2003; 3:155 - 168; PMID: 12612651; http://dx.doi.org/10.1038/nrc1011
- Bakkenist CJ, Kastan MB. Initiating cellular stress responses. Cell 2004; 118:9 - 17; PMID: 15242640; http://dx.doi.org/10.1016/j.cell.2004.06.023
- Reinhardt HC, Yaffe MB. Kinases that control the cell cycle in response to DNA damage: Chk1, Chk2 and MK2. Curr Opin Cell Biol 2009; 21:245 - 255; PMID: 19230643; http://dx.doi.org/10.1016/j.ceb.2009.01.018
- Mohammad D, Yaffe MB. 14-3-3 proteins, FHA domains and BRCT domains in the DNA damage response. DNA Repair (Amst) 2009; 8:1009 - 1017; PMID: 19481982; http://dx.doi.org/10.1016/j.dnarep.2009.04.004
- Futreal PA, Liu Q, Shattuck-Eidens D, Cochran C, Harshman K, Tavtigian S, et al. BRCA1 mutations in primary breast and ovarian carcinomas. Science 1994; 266:120 - 122; PMID: 7939630; http://dx.doi.org/10.1126/science.7939630
- Miki Y, Swensen J, Shattuck-Eidens D, Futreal PA, Harshman K, Tavtigian S, et al. A strong candidate for the breast and ovarian cancer susceptibility gene BRCA1. Science 1994; 266:66 - 71; PMID: 7545954; http://dx.doi.org/10.1126/science.7545954
- Bork P, Hofmann K, Bucher P, Neuwald AF, Altschul SF, Koonin EV. A superfamily of conserved domains in DNA damage-responsive cell cycle checkpoint proteins. FASEB J 1997; 11:68 - 76; PMID: 9034168
- Callebaut I, Mornon JP. From BRCA1 to RAP1: a widespread BRCT module closely associated with DNA repair. FEBS Lett 1997; 400:25 - 30; PMID: 9000507; http://dx.doi.org/10.1016/S0014-5793(96)01312-9
- Manke Ia, Lowery DM, Nguyen A, Yaffe MB. BRCT repeats as phosphopeptide-binding modules involved in protein targeting. Science 2003; 302:636 - 639; PMID: 14576432; http://dx.doi.org/10.1126/science.1088877
- Yu X, Chini CCS, He M, Mer G, Chen J. The BRCT domain is a phospho-protein binding domain. Science 2003; 302:639 - 642; PMID: 14576433; http://dx.doi.org/10.1126/science.1088753
- Yu X, Chen J. DNA damage-induced cell cycle checkpoint control requires CtIP, a phosphorylation-dependent binding partner of BRCA1 C-terminal domains. Mol Cell Biol 2004; 24:9478 - 9486; PMID: 15485915; http://dx.doi.org/10.1128/MCB.24.21.9478-86.2004
- Wang B, Matsuoka S, Ballif Ba, Zhang D, Smogorzewska A, Gygi SP, et al. Abraxas and RAP80 form a BRCA1 protein complex required for the DNA damage response. Science (New York, NY) 2007; 316:1194 - 1198
- Kim H, Huang J, Chen J. CCDC98 is a BRCA1-BRCT domain-binding protein involved in the DNA damage response. Nat Struct Mol Biol 2007; 14:710 - 715; PMID: 17643122; http://dx.doi.org/10.1038/nsmb1277
- Liu Z, Wu J, Yu X. CCDC98 targets BRCA1 to DNA damage sites. Nat Struct Mol Biol 2007; 14:716 - 720; PMID: 17643121; http://dx.doi.org/10.1038/nsmb1279
- Zhang X, Moréra S, Bates PA, Whitehead PC, Coffer aI, Hainbucher K, et al. Structure of an XRCC1 BRCT domain: a new protein-protein interaction module. EMBO J 1998; 17:6404 - 6411; PMID: 9799248; http://dx.doi.org/10.1093/emboj/17.21.6404
- Glover JNM, Williams RS, Lee MS. Interactions between BRCT repeats and phosphoproteins: tangled up in two. Trends Biochem Sci 2004; 29:579 - 585; PMID: 15501676; http://dx.doi.org/10.1016/j.tibs.2004.09.010
- Rodriguez MC, Songyang Z. BRCT domains: phosphopeptide binding and signaling modules. Front Biosci 2008; 13:5905 - 5915; PMID: 18508631; http://dx.doi.org/10.2741/3125
- Williams RS, Green R, Glover JN. Crystal structure of the BRCT repeat region from the breast cancer-associated protein BRCA1. Nat Struct Biol 2001; 8:838 - 842; PMID: 11573086; http://dx.doi.org/10.1038/nsb1001-838
- Lee MS, Edwards RA, Thede GL, Glover JN. Structure of the BRCT repeat domain of MDC1 and its specificity for the free COOH-terminal end of the gamma-H2AX histone tail. J Biol Chem 2005; 280:32053 - 32056; PMID: 16049003; http://dx.doi.org/10.1074/jbc.C500273200
- Edwards RA, Lee MS, Tsutakawa SE, Williams RS, Nazeer I, Kleiman FE, et al. The BARD1 C-terminal domain structure and interactions with polyadenylation factor CstF-50. Biochemistry 2008; 47:11446 - 11456; PMID: 18842000; http://dx.doi.org/10.1021/bi801115g
- Kilkenny ML, Doré AS, Roe SM, Nestoras K, Ho JCY, Watts FZ, et al. Structural and functional analysis of the Crb2-BRCT2 domain reveals distinct roles in checkpoint signaling and DNA damage repair. Genes Dev 2008; 22:2034 - 2047; PMID: 18676809; http://dx.doi.org/10.1101/gad.472808
- Leung CC, Gong Z, Chen J, Glover JN. Molecular basis of BACH1/FANCJ recognition by TopBP1 in DNA replication checkpoint control. J Biol Chem 2010; 286:4292 - 4301
- Williams JS, Williams RS, Dovey CL, Guenther G, Tainer JA, Russell P. gammaH2A binds Brc1 to maintain genome integrity during S-phase. EMBO J 2010; 29:1136 - 1148; PMID: 20094029; http://dx.doi.org/10.1038/emboj.2009.413
- Stucki M, Clapperton Ja, Mohammad D, Yaffe MB, Smerdon SJ, Jackson SP. MDC1 directly binds phosphorylated histone H2AX to regulate cellular responses to DNA double-strand breaks. Cell 2005; 123:1213 - 1226; PMID: 16377563; http://dx.doi.org/10.1016/j.cell.2005.09.038
- Birrane G, Varma AK, Soni A, Ladias Jaa. Crystal structure of the BARD1 BRCT domains. Biochemistry 2007; 46:7706 - 7712; PMID: 17550235; http://dx.doi.org/10.1021/bi700323t
- Doré AS, Furnham N, Davies OR, Sibanda BL, Chirgadze DY, Jackson SP, et al. Structure of an Xrcc4-DNA ligase IV yeast ortholog complex reveals a novel BRCT interaction mode. DNA Repair (Amst) 2006; 5:362 - 368; PMID: 16388993; http://dx.doi.org/10.1016/j.dnarep.2005.11.004
- Sibanda BL, Critchlow SE, Begun J, Pei XY, Jackson SP, Blundell TL, et al. Crystal structure of an Xrcc4-DNA ligase IV complex. Nat Struct Biol 2001; 8:1015 - 1019; PMID: 11702069; http://dx.doi.org/10.1038/nsb725
- Wu PY, Frit P, Meesala S, Dauvillier S, Modesti M, Andres SN, et al. Structural and functional interaction between the human DNA repair proteins DNA ligase IV and XRCC4. Mol Cell Biol 2009; 29:3163 - 3172; PMID: 19332554; http://dx.doi.org/10.1128/MCB.01895-08
- Rappas M, Oliver AW, Pearl LH. Structure and function of the Rad9-binding region of the DNA-damage checkpoint adaptor TopBP1. Nucleic Acids Res 2011; 39:313 - 324; PMID: 20724438; http://dx.doi.org/10.1093/nar/gkq743
- Huo YG, Bai L, Xu M, Jiang T. Crystal structure of the N-terminal region of human Topoisomerase IIβ binding protein 1. Biochem Biophys Res Commun 2010; 401:401 - 405; PMID: 20858457
- Martín-García R, de León N, Sharifmoghadam MR, Curto MÁ, Hoya M, Bustos-Sanmamed P, et al. The FN3 and BRCT motifs in the exomer component Chs5p define a conserved module that is necessary and sufficient for its function. Cellular and molecular life sciences: CMLS 2010; In press
- Lloyd J, Chapman JR, Clapperton JA, Haire LF, Hartsuiker E, Li J, et al. A supramodular FHA/BRCT-repeat architecture mediates Nbs1 adaptor function in response to DNA damage. Cell 2009; 139:100 - 111; PMID: 19804756; http://dx.doi.org/10.1016/j.cell.2009.07.043
- Williams RS, Dodson GE, Limbo O, Yamada Y, Williams JS, Guenther G, et al. Nbs1 flexibly tethers Ctp1 and Mre11-Rad50 to coordinate DNA double-strand break processing and repair. Cell 2009; 139:87 - 99; PMID: 19804755; http://dx.doi.org/10.1016/j.cell.2009.07.033
- Bang W, Kim S, Ueda A, Vikram M, Yun D, Bressan Ra, et al. Arabidopsis carboxyl-terminal domain phosphatase-like isoforms share common catalytic and interaction domains but have distinct in planta functions. Plant Physiol 2006; 142:586 - 594; PMID: 16905668; http://dx.doi.org/10.1104/pp.106.084939
- Hausmann S, Shuman S. Characterization of the CTD phosphatase Fcp1 from fission yeast. Preferential dephosphorylation of serine 2 versus serine 5. J Biol Chem 2002; 277:21213 - 21220; PMID: 11934898; http://dx.doi.org/10.1074/jbc.M202056200
- Ghosh A, Shuman S, Lima CD. The structure of Fcp1, an essential RNA polymerase II CTD phosphatase. Mol Cell 2008; 32:478 - 490; PMID: 19026779; http://dx.doi.org/10.1016/j.molcel.2008.09.021
- Liu K, Lin FT, Ruppert JM, Lin WC. Regulation of E2F1 by BRCT domain-containing protein TopBP1. Mol Cell Biol 2003; 23:3287 - 3304; PMID: 12697828; http://dx.doi.org/10.1128/MCB.23.9.3287-3304.2003
- Liu K, Paik JC, Wang B, Lin FT, Lin WC. Regulation of TopBP1 oligomerization by Akt/PKB for cell survival. EMBO J 2006; 25:4795 - 4807; PMID: 17006541; http://dx.doi.org/10.1038/sj.emboj.7601355
- Leung CCY, Kellogg E, Kuhnert A, Hänel F, Baker D, Glover JNM. Insights from the crystal structure of the sixth BRCT domain of topoisomerase IIbeta binding protein 1. Protein Sci 2010; 19:162 - 167; PMID: 19937654
- Clapperton JA, Manke IA, Lowery DM, Ho T, Haire LF, Yaffe MB, et al. Structure and mechanism of BRCA1 BRCT domain recognition of phosphorylated BACH1 with implications for cancer. Nat Struct Mol Biol 2004; 11:512 - 518; PMID: 15133502; http://dx.doi.org/10.1038/nsmb775
- Shen Y, Tong L. Structural evidence for direct interactions between the BRCT domains of human BRCA1 and a phospho-peptide from human ACC1. Biochemistry 2008; 47:5767 - 5773; PMID: 18452305; http://dx.doi.org/10.1021/bi800314m
- Shiozaki EN, Gu L, Yan N, Shi Y. Structure of the BRCT repeats of BRCA1 bound to a BACH1 phosphopeptide: implications for signaling. Mol Cell 2004; 14:405 - 412; PMID: 15125843; http://dx.doi.org/10.1016/S1097-2765(04)00238-2
- Varma AK, Brown RS, Birrane G, Ladias Jaa. Structural basis for cell cycle checkpoint control by the BRCA1-CtIP complex. Biochemistry 2005; 44:10941 - 10946; PMID: 16101277; http://dx.doi.org/10.1021/bi0509651
- Williams RS, Lee MS, Hau DD, Glover JN. Structural basis of phosphopeptide recognition by the BRCT domain of BRCA1. Nat Struct Mol Biol 2004; 11:519 - 525; PMID: 15133503; http://dx.doi.org/10.1038/nsmb776
- Gong Z, Kim JE, Leung CCY, Glover JNM, Chen J. BACH1/FANCJ acts with TopBP1 and participates early in DNA replication checkpoint control. Mol Cell 2010; 37:438 - 446; PMID: 20159562; http://dx.doi.org/10.1016/j.molcel.2010.01.002
- Campbell SJ, Edwards RA, Glover JNM. Comparison of the structures and peptide binding specificities of the BRCT domains of MDC1 and BRCA1. Structure 2010; 18:167 - 176; PMID: 20159462; http://dx.doi.org/10.1016/j.str.2009.12.008
- Thanassoulas A, Nomikos M, Theodoridou M, Yannoukakos D, Mastellos D, Nounesis G. Thermodynamic study of the BRCT domain of BARD1 and its interaction with the -pSER-X-X-Phemotif-containing BRIP1 peptide. Biochim Biophys Acta 2010; 1804:1908 - 1916
- Rodriguez M, Yu X, Chen J, Songyang Z. Phosphopeptide binding specificities of BRCA1 COOH-terminal (BRCT) domains. J Biol Chem 2003; 278:52914 - 52918; PMID: 14578343; http://dx.doi.org/10.1074/jbc.C300407200
- Ray H, Moreau K, Dizin E, Callebaut I, Venezia ND. ACCA phosphopeptide recognition by the BRCT repeats of BRCA1. J Mol Biol 2006; 359:973 - 982; PMID: 16698035; http://dx.doi.org/10.1016/j.jmb.2006.04.010
- Pei H, Zhang L, Luo K, Qin Y, Chesi M, Fei F, et al. MMSET regulates histone H4K20 methylation and 53BP1 accumulation at DNA damage sites. Nature 2011; 470:124 - 128; PMID: 21293379; http://dx.doi.org/10.1038/nature09658
- Stewart GS, Wang B, Bignell CR, Taylor AM, Elledge SJ. MDC1 is a mediator of the mammalian DNA damage checkpoint. Nature 2003; 421:961 - 966; PMID: 12607005; http://dx.doi.org/10.1038/nature01446
- Wang B, Matsuoka S, Ballif BA, Zhang D, Smogorzewska A, Gygi SP, et al. Abraxas and RAP80 form a BRCA1 protein complex required for the DNA damage response. Science 2007; 316:1194 - 1198; PMID: 17525340; http://dx.doi.org/10.1126/science.1139476.
- Hari FJ, Spycher C, Jungmichel S, Pavic L, Stucki M. A divalent FHA/BRCT-binding mechanism couples the MRE11-RAD50-NBS1 complex to damaged chromatin. EMBO Rep 2010; 11:387 - 392; PMID: 20224574; http://dx.doi.org/10.1038/embor.2010.30
- Melander F, Bekker-Jensen S, Falck J, Bartek J, Mailand N, Lukas J. Phosphorylation of SDT repeats in the MDC1 N terminus triggers retention of NBS1 at the DNA damage-modified chromatin. J Cell Biol 2008; 181:213 - 226; PMID: 18411307; http://dx.doi.org/10.1083/jcb.200708210
- Spycher C, Miller ES, Townsend K, Pavic L, Morrice Na, Janscak P, et al. Constitutive phosphorylation of MDC1 physically links the MRE11-RAD50-NBS1 complex to damaged chromatin. J Cell Biol 2008; 181:227 - 240; PMID: 18411308; http://dx.doi.org/10.1083/jcb.200709008
- Chapman JR, Jackson SP. Phospho-dependent interactions between NBS1 and MDC1 mediate chromatin retention of the MRN complex at sites of DNA damage. EMBO Rep 2008; 9:795 - 801; PMID: 18583988; http://dx.doi.org/10.1038/embor.2008.103
- Yamane K, Wu X, Chen J. A DNA damage-regulated BRCT-containing protein, TopBP1, is required for cell survival. Mol Cell Biol 2002; 22:555 - 566; PMID: 11756551; http://dx.doi.org/10.1128/MCB.22.2.555-66.2002
- Wang J, Gong Z, Chen J. MDC1 collaborates with TopBP1 in DNA replication checkpoint control. J Cell Biol 2011; 193:267 - 273; PMID: 21482717; http://dx.doi.org/10.1083/jcb.201010026
- Cescutti R, Negrini S, Kohzaki M, Halazonetis TD. TopBP1 functions with 53BP1 in the G1 DNA damage checkpoint. EMBO J 2010; 29:3723 - 3732; PMID: 20871591; http://dx.doi.org/10.1038/emboj.2010.238
- Lee J, Kumagai A, Dunphy WG. The Rad9-Hus1-Rad1 checkpoint clamp regulates interaction of TopBP1 with ATR. J Biol Chem 2007; 282:28036 - 28044; PMID: 17636252; http://dx.doi.org/10.1074/jbc.M704635200
- Delacroix S, Wagner JM, Kobayashi M, Yamamoto Ki, Karnitz LM. The Rad9-Hus1-Rad1 (9-1-1) clamp activates checkpoint signaling via TopBP1. Genes Dev 2007; 21:1472 - 1477; PMID: 17575048; http://dx.doi.org/10.1101/gad.1547007
- Caldecott KW, McKeown CK, Tucker JD, Ljungquist S, Thompson LH. An interaction between the mammalian DNA repair protein XRCC1 and DNA ligase III. Mol Cell Biol 1994; 14:68 - 76; PMID: 8264637
- Nash RA, Caldecott KW, Barnes DE, Lindahl T. XRCC1 protein interacts with one of two distinct forms of DNA ligase III. Biochemistry 1997; 36:5207 - 5211; PMID: 9136882; http://dx.doi.org/10.1021/bi962281m
- Taylor RM, Wickstead B, Cronin S, Caldecott KW. Role of a BRCT domain in the interaction of DNA ligase III-alpha with the DNA repair protein XRCC1. Curr Biol 1998; 8:877 - 880; PMID: 9705932; http://dx.doi.org/10.1016/S0960-9822(07)00350-8
- Thornton KH, Krishnan VV, West MG, Popham J, Ramirez M, Thelen MP, et al. Expression, purification and biophysical characterization of the BRCT domain of human DNA ligase IIIalpha. Protein Expr Purif 2001; 21:401 - 11; PMID: 11281714; http://dx.doi.org/10.1006/prep.2001.1391
- Dulic A, Bates PA, Zhang X, Martin SR, Freemont PS, Lindahl T, et al. BRCT domain interactions in the heterodimeric DNA repair protein XRCC1-DNA ligase III. Biochemistry 2001; 40:5906 - 5913; PMID: 11352725; http://dx.doi.org/10.1021/bi002701e
- Beernink PT, Hwang M, Ramirez M, Murphy MB, Doyle SA, Thelen MP. Specificity of protein interactions mediated by BRCT domains of the XRCC1 DNA repair protein. J Biol Chem 2005; 280:30206 - 30213; PMID: 15987676; http://dx.doi.org/10.1074/jbc.M502155200
- Krishnan VV, Thornton KH, Thelen MP, Cosman M. Solution structure and backbone dynamics of the human DNA ligase IIIalpha BRCT domain. Biochemistry 2001; 40:13158 - 13166; PMID: 11683624; http://dx.doi.org/10.1021/bi010979g
- Masson M, Niedergang C, Schreiber V, Muller S, Menissier-de Murcia J, de Murcia G. XRCC1 is specifically associated with poly(ADP-ribose) polymerase and negatively regulates its activity following DNA damage. Mol Cell Biol 1998; 18:3563 - 3571; PMID: 9584196
- Wollmann Y, Schmidt U, Wieland GD, Zipfel PF, Saluz HP, Hänel F. The DNA topoisomerase IIbeta binding protein 1 (TopBP1) interacts with poly (ADPribose) polymerase (PARP-1). J Cell Biochem 2007; 102:171 - 182; PMID: 17340632; http://dx.doi.org/10.1002/jcb.21292
- Richards MW, Leung JW, Roe SM, Li K, Chen J, Bayliss R. A pocket on the surface of the N-terminal BRCT domain of Mcph1 is required to prevent abnormal chromosome condensation. J Mol Biol 2010; 395:908 - 915; PMID: 19925808; http://dx.doi.org/10.1016/j.jmb.2009.11.029
- Jeffers LJ, Coull BJ, Stack SJ, Morrison CG. Distinct BRCT domains in Mcph1/Brit1 mediate ionizing radiation-induced focus formation and centrosomal localization. Oncogene 2008; 27:139 - 144; PMID: 17599047; http://dx.doi.org/10.1038/sj.onc.1210595
- Watts FZ, Brissett NC. Linking up and interacting with BRCT domains. DNA Repair (Amst) 2010; 9:103 - 108; PMID: 19945358; http://dx.doi.org/10.1016/j.dnarep.2009.10.010
- Datta B, Li B, Choubey D, Nallur G, Lengyel P. P202, an Interferon-Inducible Modulator of Transcription, Inhibits Transcriptional Activation By the p53 Tumor Suppressor Protein, and a Segment From the P53-Binding Protein 1 That Binds To P202 Overcomes This Inhibition. J Biol Chem 1996; 271:27544 - 27555; PMID: 8910340; http://dx.doi.org/10.1074/jbc.271.44.27544
- Iwabuchi K, Bartel PL, Li B, Marraccino R, Fields S. Two cellular proteins that bind to wild-type but not mutant p53. Proc Natl Acad Sci USA 1994; 91:6098 - 7102; PMID: 8016121; http://dx.doi.org/10.1073/pnas.91.13.6098
- Thukral SK, Blain GC, Chang KK, Fields S. Distinct residues of human p53 implicated in binding to DNA, simian virus 40 large T antigen, 53BP1 and 53BP2. Mol Cell Biol 1994; 14:8315 - 8321; PMID: 7969167
- Derbyshire DJ, Basu BP, Serpell LC, Joo WS, Date T, Iwabuchi K, et al. Crystal structure of human 53BP1 BRCT domains bound to p53 tumour suppressor. EMBO J 2002; 21:3863 - 3872; PMID: 12110597; http://dx.doi.org/10.1093/emboj/cdf383
- Joo WS, Jeffrey PD, Cantor SB, Finnin MS, Livingston DM, Pavletich NP. Structure of the 53BP1 BRCT region bound to p53 and its comparison to the Brca1 BRCT structure. Genes Dev 2002; 16:583 - 593; PMID: 11877378; http://dx.doi.org/10.1101/gad.959202
- Du LL, Moser BA, Russell P. Homo-oligomerization is the essential function of the tandem BRCT domains in the checkpoint protein Crb2. J Biol Chem 2004; 279:38409 - 38414; PMID: 15229228; http://dx.doi.org/10.1074/jbc.M403326200
- Soulier J, Lowndes NF. The BRCT domain of the S. cerevisiae checkpoint protein Rad9 mediates a Rad9-Rad9 interaction after DNA damage. Curr Biol 1999; 9:551 - 554; PMID: 10339432; http://dx.doi.org/10.1016/S0960-9822(99)80242-5
- Mochida S, Esashi F, Aono N, Tamai K, O'Connell MJ, Yanagida M. Regulation of checkpoint kinases through dynamic interaction with Crb2. EMBO J 2004; 23:418 - 428; PMID: 14739927; http://dx.doi.org/10.1038/sj.emboj.7600018
- Willson J, Wilson S, Warr N, Watts FZ. Isolation and characterization of the Schizosaccharomyces pombe rhp9 gene: a gene required for the DNA damage checkpoint but not the replication checkpoint. Nucleic Acids Res 1997; 25:2138 - 2146; PMID: 9153313; http://dx.doi.org/10.1093/nar/25.11.2138
- Li Z, Otevrel T, Gao Y, Cheng HL, Seed B, Stamato TD, et al. The XRCC4 gene encodes a novel protein involved in DNA double-strand break repair and V(D) J recombination. Cell 1995; 83:1079 - 1089; PMID: 8548796; http://dx.doi.org/10.1016/0092-8674(95)90135-3
- Critchlow SE, Bowater RP, Jackson SP. Mammalian DNA double-strand break repair protein XRCC4 interacts with DNA ligase IV. Curr Biol 1997; 7:588 - 598; PMID: 9259561; http://dx.doi.org/10.1016/S0960-9822(06)00258-2
- Grawunder U, Wilm M, Wu X, Kulesza P, Wilson TE, Mann M, et al. Activity of DNA ligase IV stimulated by complex formation with XRCC4 protein in mammalian cells. Nature 1997; 388:492 - 495; PMID: 9242410; http://dx.doi.org/10.1038/41358
- Grawunder U, Zimmer D, Kulesza P, Lieber MR. Requirement for an interaction of XRCC4 with DNA ligase IV for wild-type V(D)J recombination and DNA double-strand break repair in vivo. J Biol Chem 1998; 273:24708 - 24714; PMID: 9733770; http://dx.doi.org/10.1074/jbc.273.38.24708
- Yamane K, Katayama E, Tsuruo T. The BRCT regions of tumor suppressor BRCA1 and of XRCC1 show DNA end binding activity with a multimerizing feature. Biochem Biophys Res Commun 2000; 279:678 - 684; PMID: 11118345; http://dx.doi.org/10.1006/bbrc.2000.3983
- Yamane K, Tsuruo T. Conserved BRCT regions of TopBP1 and of the tumor suppressor BRCA1 bind strand breaks and termini of DNA. Oncogene 1999; 18:5194 - 5203; PMID: 10498869; http://dx.doi.org/10.1038/sj.onc.1202922
- Burbelo PD, Utani A, Pan ZQ, Yamada Y. Cloning of the large subunit of activator 1 (replication factor C) reveals homology with bacterial DNA ligases. Proc Natl Acad Sci USA 1993; 90:11543 - 11547; PMID: 8265586; http://dx.doi.org/10.1073/pnas.90.24.11543
- Fotedar R, Mossi R, Fitzgerald P, Rousselle T, Maga G, Brickner H, et al. A conserved domain of the large subunit of replication factor C binds PCNA and acts like a dominant negative inhibitor of DNA replication in mammalian cells. EMBO J 1996; 15:4423 - 4433; PMID: 8861969
- Tsurimoto T, Stillman B. Replication factors required for SV40 DNA replication in vitro. II. Switching of DNA polymerase alpha and delta during initiation of leading and lagging strand synthesis. J Biol Chem 1991; 266:1961 - 1968; PMID: 1671046
- Allen BL, Uhlmann F, Gaur LK, Mulder BA, Posey KL, Jones LB, et al. DNA recognition properties of the N-terminal DNA binding domain within the large subunit of replication factor C. Nucleic Acids Res 1998; 26:3877 - 3882; PMID: 9705493; http://dx.doi.org/10.1093/nar/26.17.3877
- Kobayashi M, Figaroa F, Meeuwenoord N, Jansen LET, Siegal G. Characterization of the DNA binding and structural properties of the BRCT region of human replication factor C p140 subunit. J Biol Chem 2006; 281:4308 - 4317; PMID: 16361700; http://dx.doi.org/10.1074/jbc.M511090200
- Kobayashi M, Ab E, Bonvin AMJJ, Siegal G. Structure of the DNA-bound BRCA1 C-terminal region from human replication factor C p140 and model of the protein-DNA complex. J Biol Chem 2010; 285:10087 - 10097; PMID: 20081198; http://dx.doi.org/10.1074/jbc.M109.054106
- Feng H, Parker JM, Lu J, Cao W. Effects of deletion and site-directed mutations on ligation steps of NAD+-dependent DNA ligase: a biochemical analysis of BRCA1 C-terminal domain. Biochemistry 2004; 43:12648 - 12659; PMID: 15449954; http://dx.doi.org/10.1021/bi049451c
- Wilkinson A, Smith A, Bullard D, Lavesa-Curto M, Sayer H, Bonner A, et al. Analysis of ligation and DNA binding by Escherichia coli DNA ligase (LigA). Biochimica et biophysica acta 2005; 1749:113 - 122
- Simons AM, Horwitz AA, Starita LM, Griffin K, Williams RS, Glover JN, et al. BRCA1 DNA-binding activity is stimulated by BARD1. Cancer Res 2006; 66:2012 - 2018; PMID: 16489000; http://dx.doi.org/10.1158/0008-5472.CAN-05-3296
- Choi JH, Lindsey-Boltz La, Sancar A. Cooperative activation of the ATR checkpoint kinase by TopBP1 and damaged DNA. Nucleic Acids Res 2009; 37:1501 - 1509; PMID: 19139065; http://dx.doi.org/10.1093/nar/gkn1075
- Pleschke JM, Kleczkowska HE, Strohm M, Althaus FR. Poly(ADP-ribose) binds to specific domains in DNA damage checkpoint proteins. J Biol Chem 2000; 275:40974 - 40980; PMID: 11016934; http://dx.doi.org/10.1074/jbc.M006520200
- Dantzer F, de La Rubia G, Ménissier-De Murcia J, Hostomsky Z, de Murcia G, Schreiber V. Base excision repair is impaired in mammalian cells lacking Poly(ADP-ribose) polymerase-1. Biochemistry 2000; 39:7559 - 7569; PMID: 10858306; http://dx.doi.org/10.1021/bi0003442
- El-Khamisy SF, Masutani M, Suzuki H, Caldecott KW. A requirement for PARP-1 for the assembly or stability of XRCC1 nuclear foci at sites of oxidative DNA damage. Nucleic Acids Res 2003; 31:5526 - 5533; PMID: 14500814; http://dx.doi.org/10.1093/nar/gkg761
- Schreiber V, Amé JC, Dollé P, Schultz I, Rinaldi B, Fraulob V, et al. Poly(ADP-ribose) polymerase-2 (PARP-2) is required for efficient base excision DNA repair in association with PARP-1 and XRCC1. J Biol Chem 2002; 277:23028 - 23036; PMID: 11948190; http://dx.doi.org/10.1074/jbc.M202390200