Abstract
Previously, we identified a form of epithelial-stromal metabolic coupling, in which cancer cells induce aerobic glycolysis in adjacent stromal fibroblasts, via oxidative stress, driving autophagy and mitophagy. In turn, these cancer-associated fibroblasts provide recycled nutrients to epithelial cancer cells, "fueling" oxidative mitochondrial metabolism and anabolic growth. An additional consequence is that these glycolytic fibroblasts protect cancer cells against apoptosis, by providing a steady nutrient stream of to mitochondria in cancer cells. Here, we investigated whether these interactions might be the basis of tamoxifen-resistance in ER(+) breast cancer cells. We show that MCF7 cells alone are Tamoxifen-sensitive, but become resistant when co-cultured with hTERT-immortalized human fibroblasts. Next, we searched for a drug combination (Tamoxifen + Dasatinib) that could over-come fibroblast-induced Tamoxifen-resistance. Importantly, we show that this drug combination acutely induces the Warburg effect (aerobic glycolysis) in MCF7 cancer cells, abruptly cutting off their ability to use their fuel supply, effectively killing these cancer cells. Thus, we believe that the Warburg effect in tumor cells is not the "root cause" of cancer, but rather it may provide the necessary clues to preventing chemo-resistance in cancer cells. Finally, we observed that this drug combination (Tamoxifen + Dasatinib) also had a generalized anti-oxidant effect, on both co-cultured fibroblasts and cancer cells alike, potentially reducing tumor-stroma co-evolution. Our results are consistent with the idea that chemo-resistance may be both a metabolic and stromal phenomenon that can be overcome by targeting mitochondrial function in epithelial cancer cells. Thus, simultaneously targeting both (1) the tumor stroma and (2) the epithelial cancer cells, with combination therapies, may be the most successful approach to anti-cancer therapy. This general strategy of combination therapy for overcoming drug resistance could be applicable to many different types of cancer.
Introduction
We and others have previously identified a loss of stromal caveolin-1 (Cav-1) as a single independent predictive biomarker in women with primary breast cancers.Citation1–Citation6 In ER(+) tumors, loss of stromal Cav-1 is associated with lymph-node involvement, early tumor recurrence and Tamoxifen-resistance.Citation4 Loss of stromal Cav-1 is also associated with recurrence and progression to invasive breast cancer in ER(+) ductal carcinoma in situ (DCIS).Citation2
In order to understand the biological processes that underlie a loss of stromal Cav-1 and Tamoxifen-resistance, we developed a novel co-culture system to recapitulate this phenomenon in vitro.Citation7 In this co-culture system, ER(+) breast cancer cells (MCF7) are co-cultured with hTERT-immortalized human fibroblasts.Citation7 During co-culture, the MCF7 cells induce oxidative stress in adjacent fibroblasts, resulting in the destruction of Cav-1 via lysosomal degradation and autophagy, resulting in the onset of a cancer-associated fibroblast (CAF) phenotype.Citation7–Citation11 Thus, a loss of stromal Cav-1 is a marker for oxidative stress and autophagy, in the tumor microenvironment.Citation12,Citation13
Interestingly, these glycolytic/autophagic Cav-1-deficient fibroblasts also protect MCF7 cancer cells against apoptotic cell death, likely by providing recycled nutrients and high-energy metabolites, to better fuel the anabolic survival of cancer cells.Citation8,Citation9,Citation14
Recently, we have studied the metabolic changes that occur during this co-culture process, using a quantitative approach.Citation15 For example, when these two cell types are cultured individually, MCF7 cells alone are glycolytic and fibroblasts alone are oxidative.Citation15 Conversely, in co-culture, a role-reversal occurs: MCF7 cells become oxidative and fibroblasts become glycolytic.Citation15 This metabolic shift occurs because MCF7 cells actively produce and secrete hydrogen peroxide, thereby driving aerobic glycolysis in fibroblasts, via oxidative stress and mitophagy.Citation15 In turn, the glycolytic fibroblasts produce lactate which is sufficient to induce mitochondrial biogenesis in adjacent cancer cells.Citation8,Citation9 We have termed this newly discovered type of “parasitic” cancer metabolism the “reverse Warburg effect,”Citation16 since we proposed that aerobic glycolysis occurs in fibroblasts, and that cancer cells rely predominantly on oxidative metabolism, the opposite of Warburg's original prediction.Citation12,Citation17,Citation18
Here, we applied these metabolic findings to specifically address the issue of Tamoxifen-resistance. Our results indicate that MCF7 cells are sensitive to Tamoxifen, when cultured alone, and are metabolically glycolytic. However, MCF7 cells become resistant to tamoxifen, when co-cultured with fibroblasts, and when their metabolism is largely oxidative. Thus, we hypothesized that Tamoxifen-resistance is conferred by this metabolic shift in MCF7 cells from a glycolytic to an oxidative state, which is dependent on mitochondrial biogenesis and oxidative phosphorylation in cancer cells. A corollary of this hypothesis is that we should be able to overcome Tamoxifen-resistance, by shifting MCF7 cells back to their glycolytic state.
To test this hypothesis, we developed a Tamoxifen-based drug combination that effectively kills MCF7 cells in the presence of fibroblasts. This drug combination (Tamoxifen + Dasatinib) induces aerobic glycolysis (i.e., the Warburg effect) in MCF7 cells, resulting in their apoptotic cell death. Induction of this form of “synthetic lethality” may be a new treatment strategy for overcoming resistance in the clinic.
Results
Fibroblasts confer Tamoxifen-resistance in MCF7 cells.
To investigate the role of stromal fibroblasts in conferring Tamoxifen-resistance, we first established the conditions under which Tamoxifen effectively kills MCF7 cells, a well-established ER(+) breast cancer cell line. shows that Tamoxifen-treatment (12 µM) of MCF7 cells for 48 h is sufficient to induce >80% cell death, as quantitatively measured by FACS analysis with PI and Annexin-V staining.
Then, virtually identical experiments were performed with MCF7-fibroblast co-cultures, which showed complete resistance to Tamoxifen at the same concentration, under nearly identical culture conditions ().
Dasatinib effectively reverses the chemo-resistance conferred by cancer-associated fibroblasts, in combination with tamoxifen.
Thus, we systematically searched for a well-known drug that is currently used in the clinic to treat cancer patients, to over-come the Tamoxifen-resistance conferred by fibroblasts.
One of the triggers for initiating and maintaining autophagy in cancer-associated fibroblasts is the loss of the caveolin-1 (Cav-1) protein, via lysosomal degradation.Citation7 Thus, we searched for a drug that could prevent the degradation of Cav-1, which is initiated by the onset of oxidative stress in cancer-associated fibroblasts. It is already known that, in response to oxidative stressors, such as hydrogen peroxide, Cav-1 undergoes tyrosine phosphorylationCitation19 and is targeted intracellularly to lysosomes for degradation.Citation20,Citation21 A loss of stromal Cav-1 is also a new biomarker for Tamoxifen-resistance in human breast cancer patients.Citation4
With this in mind, we tested a number of tyrosine kinase inhibitors, to prevent loss of Cav-1 in cancer-associated fibroblasts. shows that treatment with Dasatinib prevents a loss of stromal Cav-1, as predicted. However, treatment with Dasatinib did not increase the expression of Cav-1 when fibroblasts were cultured alone, in the absence of cancer cells (). Thus, the effects of Dasatinib were specific for fibroblasts during co-culture with cancer cells; we have previously documented that these co-culture conditions are sufficient to induce oxidative stress in fibroblasts.Citation8,Citation11
Importantly, Dasatinib (2.5 nM) has no effect on MCF7 cells when they are cultured alone, in the absence of fibroblasts. Also, the combination of Tamoxifen (12 µM) and Dasatinib (2.5 nM) is not synergistic in MCF7 cell mono-cultures (). However, the combination of Tamoxifen plus Dasatinib (T + D) selectively kills MCF7 cells in co-culture, resulting in nearly 80% cell death (reflecting a two- to three-fold increase) ().
As such, by using a new paradigm for cancer metabolism, termed the “reverse Warburg effect,”Citation16 we have rationally identified a new drug combination that overcomes metabolically-induced drug resistance. This drug combination selectively kills MCF7 cancer cells in the presence of fibroblasts, while each drug alone has little or no effect. Thus, this drug-pair is synthetically-lethal with the “reverse Warburg effect.”
Our findings with fibroblasts in co-culture are included for comparison (), indicating that Dasatinib preferentially induces apoptosis in the cancer-associated fibroblast population, whereas Tamoxifen has only a modest effect.
Tamoxifen plus Dasatinib induces aerobic glycolysis in MCF7 cells, and shows an generalized anti-oxidant effect.
When MCF7 cells are cultured alone, they show a reduced mitochondrial mass, are predominantly glycolytic, and thus show the traditional Warburg effect.Citation8,Citation9,Citation15 However, when co-cultured with fibroblasts, they undergo a shift toward oxidative mitochondrial metabolism, with increased mitochondrial mass and decreased glucose uptake.Citation8,Citation9,Citation15 We have termed this change in metabolism the “reverse Warburg effect,” as aerobic glycolysis takes place in cancer-associated fibroblast to provide metabolic substrates to “feed” the TCA cycle in adjacent cancer cells.
In , we showed that MCF7 cells cultured alone are sensitive to Tamoxifen alone, when they are undergoing aerobic glycolysis (the Warburg effect). Thus, we reasoned that the combination of Tamoxifen plus Dasatinib might return cocultured MCF7 cells to a “Warburg-like state,” thus preventing them from using the high-energy nutrients derived from fibroblasts.
To test this hypothesis, we measured glucose uptake and mitochondrial activity in MCF7-fibroblast co-cultures, with and without drug treatment.Citation15 shows that the combination of Tamoxifen plus Dasatinib significantly increases glucose uptake (∼four-fold) in co-cultured MCF7 cells, indicative of a shift toward aerobic glycolysis. Similarly, we see that this drug combination normalizes the glucose uptake ratio (Fibro/MCF7), such that fibroblasts and MCF7 cells take up almost the same amount of glucose (). Usually, in coculture, fibroblasts take up more glucose (∼two- to three-fold) than MCF7 cells, as the fibroblasts are undergoing aerobic glycolysis.Citation15
In accordance with our results from the glucose uptake studies, we observed that Tamoxifen plus Dasatinib reduces mitochondrial activity >six-fold, as measured using MitoTracker, in cocultured MCF7 cells (). In addition, this drug combination normalizes the mitochondrial activity ratio (MCF7/Fibro), such that MCF7 cells show reduced mitochondrial activity, relative to fibroblasts (). Note that, normally in co-culture, MCF7 cells show ∼2.6-fold more mitochondrial activity than fibroblasts, and this is reduced to 1.5-fold. Furthermore, Tamoxifen plus Dasatinib also reduces mitochondrial activity (∼3.7-fold) in cocultured fibroblasts (), suggesting that this drug combination generally reduces mitochondrial function in both cell types.
Consistent with overall reduced mitochondrial activity, we observed decreased ROS production in both co-cultured MCF7 cells and fibroblasts ( and ). This represents a 3.3-fold reduction in ROS in co-cultured MCF7 cells and a near 14-fold reduction in ROS in co-cultured fibroblasts.
Thus, Tamoxifen plus Dasatinib has an overall anti-oxidant effect, likely via reductions in mitochondrial function.
Discussion
Here, we show that fibroblasts confer Tamoxifen-resistance upon ER(+) breast cancer cells, using MCF7-fibroblast co-cultures as a model system. In order to over-come such Tamoxifen-resistance, we identified a second drug, a tyrosine kinase inhibitor (Dasatinib), which acts synergistically with Tamoxifen, to induce MCF7 cancer cell death in the presence of fibroblasts. Mechanistically, we observed that this drug combination was sufficient to induce the Warburg effect in co-cultured MCF7 cells, resulting in decreased mitochondrial activity and increased glucose uptake (). In addition, this drug combination also significantly reduced ROS production in both MCF7 cells and stromal fibroblasts, acting effectively as a generalized antioxidant. Since ROS production results in enhanced DNA damage,Citation22 this drug combination may also help retard tumor-stroma co-evolution.Citation23
As such, our results are consistent with the idea that chemoresistance may be both a metabolic and stromal phenomenon that can be overcome by reducing mitochondrial function in both cell types. Thus, the “Warburg effect” may not be “root cause” of cancer, as previously proposed.Citation17,Citation18 Instead, we believe that the “Warburg effect” could be the therapeutic answer to drug resistance.
Interestingly, combining tyrosine kinase inhibitors with anti-estrogen therapy is not a new concept for preventing drug resistance, and has already been successfully tested experimentally in animal models.Citation24,Citation25 However, these authors proposed a completely different mechanism for their synergistic action, based on the positive role of the Src-tyrosine kinase in potentiating ER-α signaling in epithelial cancer cells. In contrast, our data suggest that the target of tyrosine kinase inhibitors (such as Dastatinib) is predominantly the cancer-associated fibroblast compartment (see and ), resulting in severe metabolic consequences for both cancer cells and adjacent fibroblasts ().
Materials and Methods
Materials.
Antibodies were as follows: anti-Cav-1 (N20, Santa Cruz), anti-cytokeratin 8/18 (20R-CP004, Fitzgerald Industries International). Other reagents were as follows: DAPI and Prolong Gold Antifade mounting reagent from Invitrogen, Tamoxifen from Sigma, Dasatinib (CAS No.: 302962-49-8) from Euroasian Chemicals (www.euroasiarnd.com).
Cell culture.
The MCF7 human breast cancer cell line was from ATCC. Human skin fibroblasts immortalized with human telomerase reverse transcriptase (hTERT-BJ1) were purchased originally from Clontech, Inc. All cells were maintained in DMEM, with 10% Fetal Bovine Serum (FBS) and Penicillin 100 units/mL-Streptomycin 100 µg/mL (Invitrogen). Co-culture experiments were performed as previously described in references 7, 8 and 15. Fibroblasts and MCF7 cells were co-plated in 12-well plates in complete media. Fibroblasts were seeded first and MCF7 cells were plated within 2 h. The fibroblast-to-epithelial cell ratio was 5:1, with a total of 1 × 105 cells per well. As controls, cultures of fibroblasts and cancer cells alone were plated in parallel, using the same number of a given cell population as the corresponding co-cultures. The day after, the media was changed to DMEM with 10% NuSerum I (a low protein alternative to FBS; BD Biosciences) and Pen-Strep. Cells were maintained in this media for 2 to 5 days, until further analysis. Both mono-cultures and co-cultures were maintained at 37°C in a humidified atmosphere containing 5% CO2.
Drug treatment.
For immuno-fluorescence analysis, day 4 co-cultures and corresponding control cells were treated with Dasatinib at a final concentration of 5 nM for 24 h. Vehicle alone (DMSO) treated cells were used as control. For the apoptosis and metabolic studies, co-cultures were established. The next day, the media was changed to DMEM-10% NuSerum I and the drugs were added at that time. Cells were treated either with Tamoxifen (final concentration 12 µM), Dasatinib (final concentration 2.5 nM), or with the drug combinations for 48 h. Tamoxifen was dissolved in ethanol and further diluted in media. Dasatinib was diluted in DMSO and further diluted in media. Cells treated with the vehicles alone (ethanol and DMSO) were used as critical negative controls.
Immunofluorescence (IF).
Cells were fixed for 30 min at room temperature in 2% paraformaldehyde diluted in PBS. Then, cells were rinsed with PBS/CM (PBS with 0.1 mM Calcium Chloride and 1 mM Magnesium Chloride), and permeabilized for 10 min at room temperature with immunofluorescence (IF) buffer (PBS/CM, 0.2% BSA, 0.1% TritonX-100). Then, cells were incubated with NH4Cl in PBS to quench free aldehyde groups. Primary antibodies were incubated in IF buffer for 1 h at room temperature. After washing with IF buffer (3x, 10 min each), cells were incubated for 30 min at room temperature with fluorocrome-conjugated secondary antibodies diluted in IF buffer. Finally, cells were washed with IF buffer (3x, 10 min each), counterstained with DAPI, and mounted with Prolong Gold Antifade reagent.
Confocal microscopy.
Images were collected with a Zeiss LSM510 meta confocal system using a 405 nm Diode excitation laser with a band pass filter of 420–480 nm, a 488 nm Argon excitation laser with a band pass filter of 505–550 nm and a 543 nm HeNe excitation laser with a 561–604 nm filter. Images were acquired with a 40x objective.
Apoptosis assay.
Cell death was quantified by flow cytometry using propidium iodide (PI) and Annexin-V-APC (Apoptosis Detection Kit, BD Biosciences), as previously described in reference Citation8 and Citation9. Briefly, MCF7-GFP cells were plated either alone or as co-cultures with fibroblasts. Next day, cells were treated either with Tamoxifen (12 µM) or Dasatinib (2.5 nM), or the combination of Tamoxifen and Dasatinib, or vehicles alone, all in DMEM-10% NuSerum. After 48 h, cells were collected by centrifugation and re-suspended in 500 µL of Annexin-V Binding Buffer. Then, the annexin V-APC conjugate (BD Biosciences 550474) (4 µL) and PI (1 µL) was added and incubated in the dark at room temperature for 5 min. Cells were then analyzed by flow cytometry using a GFP signal detector (to detect MCF7-GFP cells), a PE Texas Red signal detector (to detect PI) and an APC signal detector (to detect Annexin V positive cells). Data was analyzed using FlowJo 8.8 software.
Glucose uptake.
Glucose uptake was monitored using 2-NBDG (N13195, Invitrogen).Citation15 MCF7-RFP cell-fibroblasts co-cultures were treated with Tamoxifen (12 µM) or Dasatinib (2.5 nM), or combination of Tamoxifen and Dasatinib or vehicles alone for 48 h. Then, cells were incubated with pre-warmed 2-NBDG solution (diluted in serum-free DMEM to a final concentration of 200 µM) for 15 min at 37°C. All subsequent steps were performed in the dark. Cells were washed in PBS, harvested and re-suspended in 500 µL of Binding Buffer (BD Biosciences). Cells were then analyzed by flow cytometry using a GFP signal detector (to detect 2-NBDG) and a PE Texas Red signal detector (to detect RFP-positive MCF7 cells). Thus, fibroblasts in co-culture were the RFP-negative population. Data analysis was performed using FlowJo 8.8 software.
Mitochondrial activity.
To measure mitochondrial activity, cells were stained with MitoTracker Orange (CMTMRos, M7510, Invitrogen).Citation15 Briefly, co-cultures of GFP-MCF7 cells and fibroblasts were placed in DMEM with 10% NuSerum and immediately treated with Tamoxifen (12 µM) or Dasatinib (2.5 nM), or combination of Tamoxifen and Dasatinib or vehicles alone. After 48 h, cells were incubated with pre-warmed MitoTracker staining solution (diluted in serum free DMEM to a final concentration of 35 nM) for 10 min at 37°C. All subsequent steps were performed in the dark. Cells were washed in PBS, harvested and re-suspended in 500 µL of Binding Buffer (BD Biosciences). Cells were then analyzed by flow cytometry using a GFP signal detector (to detect GFP-positive MCF7 cells) and a PE Texas Red signal detector (to detect the MitoTracker staining). Thus, fibroblasts in co-culture were the GFP-negative population. Data was analyzed using FlowJo 8.8 software.
ROS measurement.
To measure intracellular ROS, the cell-permeable molecule CM-H2DCF-DA (C369, Invitrogen) was used as per manufacturer's instructions.Citation15 Briefly, MCF7-RFP cells were co-cultured with fibroblasts and treated with the indicated drugs for 48 h in DMEM-10% NuSerum. Then, cells were washed twice with HBSS buffer and incubated with 10 µM CM-H2DCF-DA for 15 min at 37°C. All subsequent steps were performed in the dark. Cells were washed and incubated with Phenol-red free media supplemented with 10% FBS for 15 min at 37°C. Cells were then washed in PBS, harvested and re-suspended in 500 µL of Binding Buffer (BD Biosciences). Cells were then analyzed by flow cytometry using a GFP signal detector (to detect DCF-DA), and a PE Texas Red signal detector (to detect RFP-positive MCF7 cells). Thus, fibroblasts in co-culture were the RFP-negative population. Data analysis was performed using FlowJo 8.8 software.
Statistical analysis.
Data were analyzed using the Student's t-test; p values < 0.05 were considered statistically significant.
Figures and Tables
Figure 1 Tamoxifen-induced apoptosis in MCF7 cells cultured alone: Dasatinib has no synergistic effect. MCF7 breast cancer cells were cultured alone, in the absence of fibroblasts and subjected to drug treatment with Tamoxifen (T; 12 µM) or Dasatinib (D; 2.5 nM), individually or in combination. Cell death in MCF7 cells was quantitated by FACS analysis via Annexin-V and PI staining. Note that Tamoxifen (T) treatment induces significant cell death in MCF7 cells. However, Dasatinib (D) has little or no appreciable effect. Tamoxifen plus Dasatinib (T + D) does not have a synergistic effect. In (A), “cell death” represents the percentage of cells that were Annexin-V(+) and/or PI(+), the sum of all three quadrants. In (B), only the Annexin-V(+) positivity is shown, yielding similar results. CTRL (control), represents MCF7 cells alone, treated with vehicle alone.
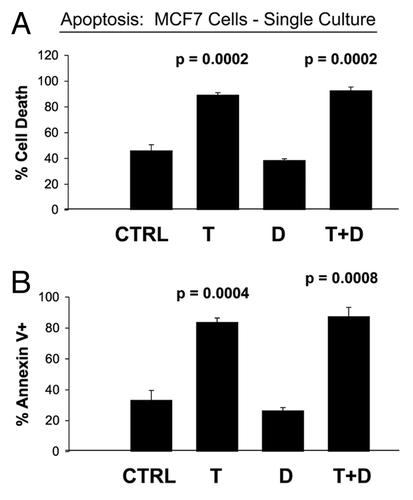
Figure 2 MCF7 cells co-cultured with fibroblasts are Tamoxifen-resistant: Dasatinib overcomes Tamoxifen-resistance. MCF7 breast cancer cells were co-cultured with fibroblasts, and then subjected to drug treatment with Tamoxifen (T; 12 µM) or Dasatinib (D; 2.5 nM), individually or in combination. Cell death in MCF7 cells was quantitated by FACS analysis via Annexin-V and PI staining. Note that individually Tamoxifen (T) or Dasatinib (D) treatment has little or no effect on MCF7 cell apoptosis. However, Tamoxifen plus Dasatinib (T + D) does have a clear synergistic effect, significantly killing MCF7 cells in co-culture. In (A), “cell death” represents the percentage of cells that were Annexin-V(+) and/or PI(+), the sum of all three quadrants. In (B), only the Annexin-V(+) positivity is shown, yielding similar results. CTRL (control), represents co-cultured MCF7 cells, treated with vehicle alone.
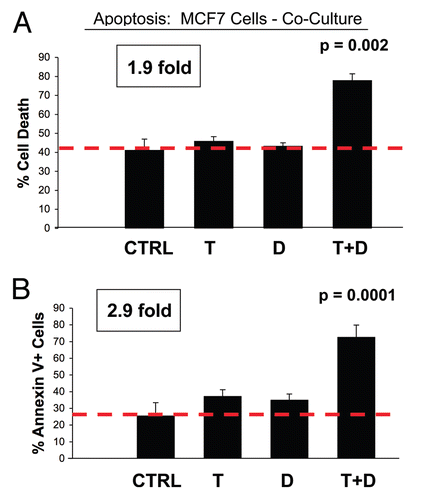
Figure 3 Dasatinib prevents a loss of Cav-1 expression in cancer-associated fibroblasts. MCF7 cells were co-cultured with fibroblasts, in the presence or absence of Dasatinib (2.5 or 5 nM). Then, these co-cultures were immuno-stained with antibody probes directed against Cav-1 (to detect fibroblasts) and Keratin-8/18 (to detect MCF7 epithelial cancer cells). Nuclei were visualized via DAPI staining. Note that Dasatinib treatment rescues the expression of Cav-1, that is normally downregulated in fibroblasts by co-culture with MCF7 cells. Similar results were obtained with both 2.5 and 5 nM Dasatinib. Control represents MCF7-co-cultures treated with vehicle alone.
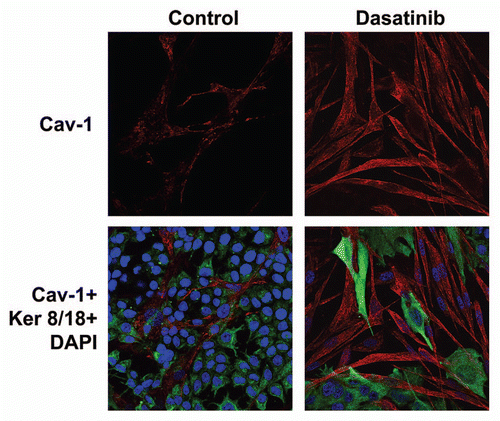
Figure 4 Dasatinib has no effect on Cav-1 levels in stromal fibroblasts cultured alone. As in , except that fibroblasts were cultured alone, in the absence of MCF7 cancer cells. Note that Dasatinib treatment does not affect expression of Cav-1. Control represents fibroblasts alone treated with vehicle alone.
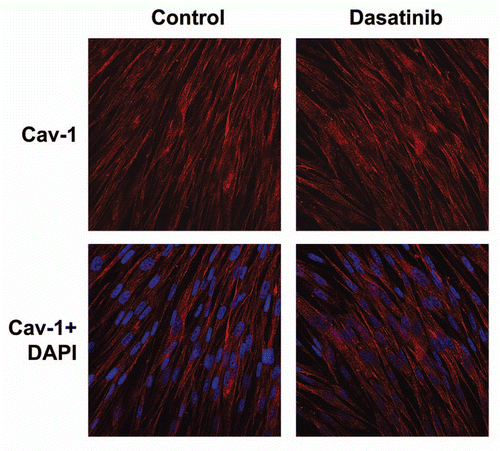
Figure 5 Dasatinib preferentially affects fibroblasts in co-culture. MCF7 breast cancer cells were co-cultured with fibroblasts, and then subjected to drug treatment with Tamoxifen (T; 12 µM) or Dasatinib (D; 2.5 nM), individually or in combination. Cell death in fibroblasts was quantitated by FACS analysis via Annexin-V and PI staining. Note that Dasatinib (D) preferentially affects fibroblasts in co-culture, while Tamoxifen (T) has only minor effects. In (A), “cell death” represents the percentage of cells that were Annexin-V(+) and/or PI(+), the sum of all three quadrants. In (B), only the Annexin-V(+) positivity is shown, yielding similar results. CTRL (control), represents co-cultured fibroblasts, treated with vehicle alone.
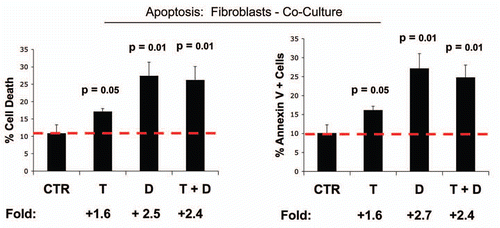
Figure 6 Tamoxifen plus Dasatinib increases glucose uptake in MCF7 cells co-cultured with fibroblasts. MCF7 breast cancer cells were co-cultured with fibroblasts, and then subjected to drug treatment with Tamoxifen (T; 12 µM) or Dasatinib (D; 2.5 nM), individually or in combination. Glucose uptake in MCF7 cells was quantitated by FACS analysis, using NBD-2-deoxy-glucose as a probe. Tamoxifen plus Dasatinib (T + D) has a clear synergistic effect, significantly increasing glucose uptake nearly 4-fold in MCF7 cells in co-culture. CTRL (control), represents co-cultured MCF7 cells, treated with vehicle alone.

Figure 7 Tamoxifen plus Dasatinib normalizes the glucose uptake ratio in co-cultures (Fibroblasts/MCF7 Cells). As in , except that glucose uptake was measured in both co-cultured MCF7 cells and fibroblasts. Note that Tamoxifen plus Dasatinib (T + D) has a clear synergistic effect, significantly normalizing the glucose uptake ratio (Fibro/MCF7) from nearly 2, to less than 1.
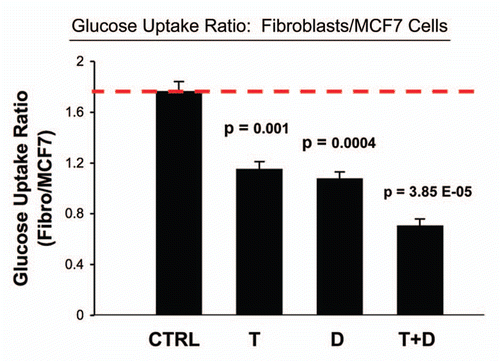
Figure 8 Tamoxifen plus Dasatinib reduces mitochondrial activity in MCF7 cells co-cultured with fibroblasts. MCF7 breast cancer cells were co-cultured with fibroblasts, and then subjected to drug treatment with Tamoxifen (T; 12 µM) or Dasatinib (D; 2.5 nM), individually or in combination. Mitochondrial activity in MCF7 cells was quantitated by FACS analysis, using MitoTracker as a probe. Tamoxifen plus Dasatinib (T + D) has a clear synergistic effect, significantly decreasing mitochondrial activity >six-fold in MCF7 cells in co-culture. CTRL (control), represents co-cultured MCF7 cells, treated with vehicle alone.
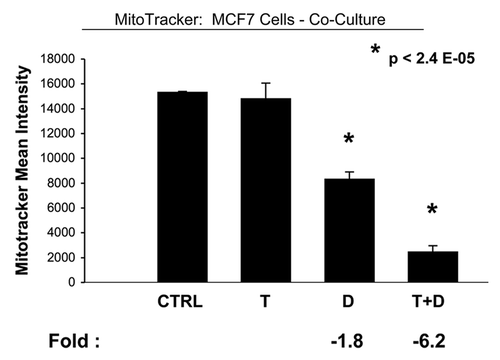
Figure 9 Tamoxifen plus Dasatinib normalizes the mitochondrial activity ratio in co-cultures (MCF7 cells/fibroblasts). As in , except that mitochondrial activity was measured in both co-cultured MCF7 cells and fibroblasts. Note that Tamoxifen plus Dasatinib (T + D) has a synergistic effect, significantly normalizing the mitochondrial activity ratio (MCF7/Fibro) from greater than 2.5, to less than 1.5.
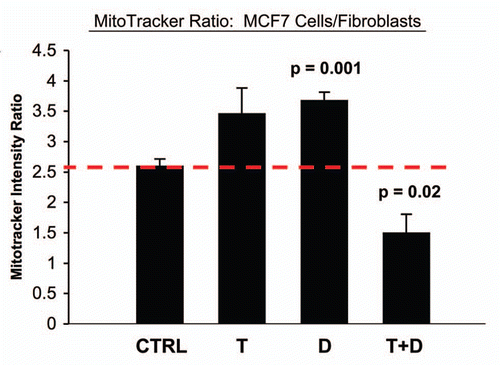
Figure 10 Tamoxifen plus Dasatinib reduces mitochondrial activity in fibroblasts co-cultured with MCF7 cells. MCF7 breast cancer cells were co-cultured with fibroblasts, and then subjected to drug treatment with tamoxifen (T; 12 µM) or Dasatinib (D; 2.5 nM), individually or in combination. Mitochondrial activity in fibroblasts was then quantitated by FACS analysis, using Mitotracker as a probe. Tamoxifen plus Dasatinib (T + D) has a clear synergistic effect, significantly decreasing mitochondrial activity nearly four-fold in fibroblasts in co-culture. CTRL (control), represents co-cultured fibroblasts, treated with vehicle alone.
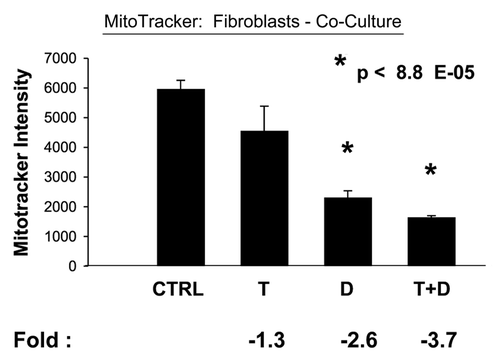
Figure 11 Tamoxifen plus Dasatinib reduces ROS activity in MCF7 cells co-cultured with fibroblasts. MCF7 breast cancer cells were co-cultured with fibroblasts, and then subjected to drug treatment with Tamoxifen (T; 12 µM) or Dasatinib (D; 2.5 nM), individually or in combination. ROS production in MCF7 cells was then quantitated by FACS analysis, using DCF-DA as a probe. Tamoxifen plus Dasatinib (T + D) has a clear synergistic anti-oxidant effect, significantly decreasing ROS activity ∼three-fold in MCF7 cells in co-culture. CTRL (control), represents co-cultured MCF7 cells, treated with vehicle alone.
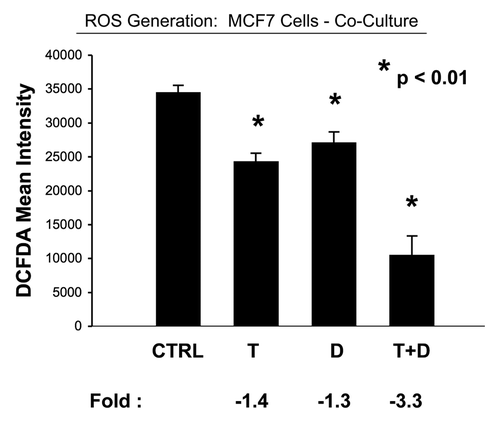
Figure 12 Tamoxifen plus Dasatinib reduces ROS activity in fibroblasts co-cultured with MCF7 cells. MCF7 breast cancer cells were co-cultured with fibroblasts, and then subjected to drug treatment with Tamoxifen (T; 12 µM) or Dasatinib (D; 2.5 nM), individually or in combination. ROS production in fibroblasts was then quantitated by FACS analysis, using DCF-DA as a probe. tamoxifen plus Dasatinib (T + D) has a clear synergistic anti-oxidant effect, significantly decreasing ROS activity ∼14-fold in fibroblasts in co-culture. CTRL (control), represents co-cultured fibroblasts, treated with vehicle alone.
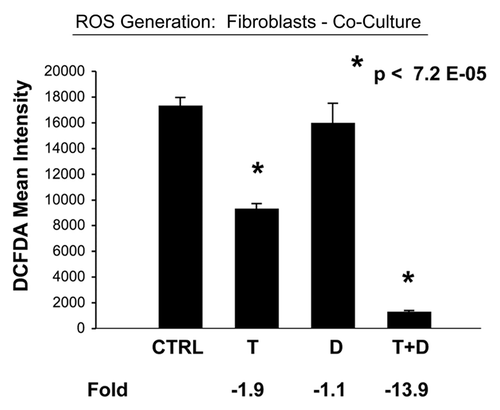
Figure 13 Understanding the metabolic basis of drug resistance: therapeutic induction of the Warburg Effect kills cancer cells. (A) In summary, our results indicate that MCF7 cells are sensitive to Tamoxifen (T), when they are cultured alone, and are metabolically glycolytic. However, MCF7 cells become resistant to Tamoxifen, when they are co-cultured with fibroblasts, and become oxidative (Upper). Thus, Tamoxifen-resistance may conferred by this metabolic shift in MCF7 cells from a glycolytic to an oxidative state, which is dependent on mitochondrial oxidative phosphorylation in cancer cells. As a consequence, we should be able to overcome Tamoxifen-resistance, by shifting MCF7 cells back to their glycolytic state. For this purpose, we developed a Tamoxifen-based drug combination that induces aerobic glycolysis (i.e., the Warburg Effect) in co-cultured MCF7 cells, resulting in their apoptotic cell death (Lower). As such, this drug combination (Tamoxifen + Dasatinib; T + D) is synthetically lethal with the “reverse Warburg effect” in ER(+) breast cancer cells. This new strategy for overcoming drug resistance should be generally applicable to many different types of cancer. The blue arrow indicates the direction of net energy flow, from fibroblasts to cancer cells, due to the “reverse Warburg effect.” (B) Targeting both the tumor stroma and epithelial cancer cells with a combination therapy. Cancer cells use oxidative stress as a “weapon” to extract recycled nutrients (via autophagy/mitophagy) and high-energy metabolites (via aerobic glycolysis) from adjacent stromal fibroblasts. Combination therapy with Tamoxifen plus Dasatinib may interrupt this vicious metabolic cycle, by simultaneously targeting both epithelial cancer cells and their “food supply,” the fibroblasts.
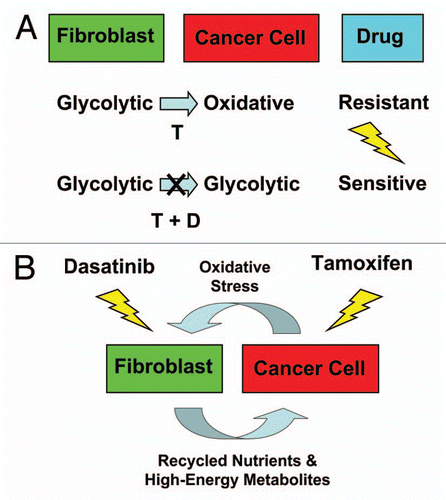
Acknowledgments
M.P.L. and his laboratory were supported by grants from the NIH/NCI (R01-CA-080250; R01-CA-098779; R01-CA-120876; R01-AR-055660), and the Susan G. Komen Breast Cancer Foundation. A.K.W. was supported by a Young Investigator Award from Breast Cancer Alliance, Inc., and a Susan G. Komen Career Catalyst Grant. F.S. was supported by grants from the W.W. Smith Charitable Trust, the Breast Cancer Alliance (BCA) and a Research Scholar Grant from the American Cancer Society (ACS). Funds were also contributed by the Margaret Q. Landenberger Research Foundation (to M.P.L.). R.G.P. was supported by grants from the NIH/NCI (R01-CA-70896, R01-CA-75503, R01-CA-86072 and R01-CA-107382) and the Dr. Ralph and Marian C. Falk Medical Research Trust. The Kimmel Cancer Center was supported by the NIH/NCI Cancer Center Core grant P30-CA-56036 (to R.G.P.).
This project is funded, in part, under a grant with the Pennsylvania Department of Health (to M.P.L. and F.S.). The Department specifically disclaims responsibility for any analyses, interpretations or conclusions. This work was also supported, in part, by a Centre grant in Manchester from Breakthrough Breast Cancer in the UK (to A.H.) and an Advanced ERC Grant from the European Research Council.
References
- Witkiewicz AK, Casimiro MC, Dasgupta A, Mercier I, Wang C, Bonuccelli G, et al. Towards a new “stromal-based” classification system for human breast cancer prognosis and therapy. Cell Cycle 2009; 8:1654 - 1658; PMID: 19448435; http://dx.doi.org/10.4161/cc.8.11.8544
- Witkiewicz AK, Dasgupta A, Nguyen KH, Liu C, Kovatich AJ, Schwartz GF, et al. Stromal caveolin-1 levels predict early DCIS progression to invasive breast cancer. Cancer Biol Ther 2009; 8:1071 - 1079; PMID: 19502809; http://dx.doi.org/10.4161/cbt.8.11.8874
- Witkiewicz AK, Dasgupta A, Sammons S, Er O, Potoczek MB, Guiles F, et al. Loss of stromal caveolin-1 expression predicts poor clinical outcome in triple negative and basal-like breast cancers. Cancer Biol Ther 2010; 10:135 - 143; PMID: 20431349; http://dx.doi.org/10.4161/cbt.10.2.11983
- Witkiewicz AK, Dasgupta A, Sotgia F, Mercier I, Pestell RG, Sabel M, et al. An absence of stromal caveolin-1 expression predicts early tumor recurrence and poor clinical outcome in human breast cancers. Am J Pathol 2009; 174:2023 - 2034; PMID: 19411448; http://dx.doi.org/10.2353/ajpath.2009.080873
- Witkiewicz AK, Kline J, Queenan M, Brody JR, Tsirigos A, Bilal E, et al. Molecular profiling of a lethal tumor microenvironment, as defined by stromal caveolin-1 status in breast cancers. Cell Cycle 2011; 10:1794 - 1809; PMID: 21521946
- Sloan EK, Ciocca DR, Pouliot N, Natoli A, Restall C, Henderson MA, et al. Stromal cell expression of caveolin-1 predicts outcome in breast cancer. Am J Pathol 2009; 174:2035 - 2043; PMID: 19411449; http://dx.doi.org/10.2353/ajpath.2009.080924
- Martinez-Outschoorn UE, Pavlides S, Whitaker-Menezes D, Daumer KM, Milliman JN, Chiavarina B, et al. Tumor cells induce the cancer associated fibroblast phenotype via caveolin-1 degradation: Implications for breast cancer and DCIS therapy with autophagy inhibitors. Cell Cycle 2010; 9:2423 - 2433; PMID: 20562526; http://dx.doi.org/10.4161/cc.9.12.12048
- Martinez-Outschoorn UE, Balliet RM, Rivadeneira DB, Chiavarina B, Pavlides S, Wang C, et al. Oxidative stress in cancer-associated fibroblasts drives tumor-stroma co-evolution: A new paradigm for understanding tumor metabolism, the field effect and genomic instability in cancer cells. Cell Cycle 2010; 9:3256 - 3276; PMID: 20814239; http://dx.doi.org/10.4161/cc.9.16.12553
- Martinez-Outschoorn UE, Trimmer C, Lin Z, Whitaker-Menezes D, Chiavarina B, Zhou J, et al. Autophagy in cancer-associated fibroblasts promotes tumor cell survival: Role of hypoxia, HIF1 induction and NFkappaB activation in the tumor stromal micro-environment. Cell Cycle 2010; 9:3515 - 3533; PMID: 20855962; http://dx.doi.org/10.4161/cc.9.17.12928
- Martinez-Outschoorn UE, Whitaker-Menezes D, Lin Z, Flomenberg N, Howell A, Pestell RG, et al. Cytokine production and inflammation drive autophagy in the tumor microenvironment: Role of stromal caveolin-1 as a key regulator. Cell Cycle 2011; 10:1784 - 1793; PMID: 21566463
- Whitaker-Menezes D, Martinez-Outschoorn UE, Lin Z, Ertel A, Flomenberg N, Witkiewicz AK, et al. Evidence for a stromal-epithelial “lactate shuttle” in human tumors: MCT4 is a marker of oxidative stress in cancer-associated fibroblasts. Cell Cycle 2011; 10:1772 - 1783; PMID: 21558814
- Martinez-Outschoorn UE, Pavlides S, Howell A, Pestell RG, Tanowitz HB, Sotgia F, Lisanti MP. Stromal-epithelial metabolic coupling in cancer: Integrating autophagy and metabolism in the tumor microenvironment. Int J Biochem Cell Biol 2011; 43:1045 - 1051; PMID: 21300172; http://dx.doi.org/10.1016/j.biocel.2011.01.023
- Martinez-Outschoorn UE, Whitaker-Menezes D, Pavlides S, Chiavarina B, Bonuccelli G, Casey T, et al. The Autophagic Tumor Stroma Model of Cancer or “Battery-Operated Tumor Growth”: A Simple Solution to the Autophagy Paradox. Cell Cycle 2010; 9:4297 - 4306; PMID: 21051947; http://dx.doi.org/10.4161/cc.9.21.13817
- Martinez-Outschoorn UE, Prisco M, Ertel A, Tsirigos A, Lin Z, Pavlides S, et al. Ketones and lactate increase cancer cell “stemness,” driving recurrence, metastasis and poor clinical outcome in breast cancer: Achieving personalized medicine via Metabolo-Genomics. Cell Cycle 2011; 10:1271 - 1286; PMID: 21512313; http://dx.doi.org/10.4161/cc.10.8.15330
- Martinez-Outschoorn UE, Lin Z, Flomenberg N, Wang C, Pavlides S, Pestell RG, et al. Cancer Cells “Fertilize” the Tumor Microenvironment with Hydrogen Peroxide, Driving the Reverse Warburg Effect: Implications for PET Imaging of Human Tumors. Cell Cycle 2011; 10:2504 - 2520
- Pavlides S, Whitaker-Menezes D, Castello-Cros R, Flomenberg N, Witkiewicz AK, Frank PG, et al. The reverse Warburg effect: aerobic glycolysis in cancer-associated fibroblasts and the tumor stroma. Cell Cycle 2009; 8:3984 - 4001; PMID: 19923890; http://dx.doi.org/10.4161/cc.8.23.10238
- Warburg O. On respiratory impairment in cancer cells. Science 1956; 124:269 - 270; PMID: 13351639
- Warburg O. On the origin of cancer cells. Science 1956; 123:309 - 314; PMID: 13298683; http://dx.doi.org/10.1126/science.123.3191.309
- Volonté D, Galbiati F, Pestell RG, Lisanti MP. Cellular stress induces the tyrosine phosphorylation of caveolin-1 (Tyr(14)) via activation of p38 mitogen-activated protein kinase and c-Src kinase. Evidence for caveolae, the actin cytoskeleton and focal adhesions as mechanical sensors of osmotic stress. J Biol Chem 2001; 276:8094 - 8103; PMID: 11094059; http://dx.doi.org/10.1074/jbc.M009245200
- Hayer A, Stoeber M, Ritz D, Engel S, Meyer HH, Helenius A. Caveolin-1 is ubiquitinated and targeted to intralumenal vesicles in endolysosomes for degradation. J Cell Biol 2010; 191:615 - 629; PMID: 21041450; http://dx.doi.org/10.1083/jcb.201003086
- Peterson TE, Guicciardi ME, Gulati R, Kleppe LS, Mueske CS, Mookadam M, et al. Caveolin-1 can regulate vascular smooth muscle cell fate by switching platelet-derived growth factor signaling from a proliferative to an apoptotic pathway. Arterioscler Thromb Vasc Biol 2003; 23:1521 - 1527; PMID: 12816877; http://dx.doi.org/10.1161/01.ATV.0000081743.35125.05
- Lisanti MP, Martinez-Outschoorn UE, Pavlides S, Whitaker-Menezes D, Pestell RG, Howell A, et al. Accelerated aging in the tumor microenvironment: Connecting aging, inflammation and cancer metabolism with personalized medicine. Cell Cycle 2011; 10:2059 - 2063; PMID: 21654190; http://dx.doi.org/10.4161/cc.10.13.16233
- Lisanti MP, Martinez-Outschoorn UE, Chiavarina B, Pavlides S, Whitaker-Menezes D, Tsirigos A, et al. Understanding the “lethal” drivers of tumor-stroma co-evolution: emerging role(s) for hypoxia, oxidative stress and autophagy/mitophagy in the tumor micro-environment. Cancer Biol Ther 2010; 10:537 - 542; PMID: 20861671; http://dx.doi.org/10.4161/cbt.10.6.13370
- Vallabhaneni S, Nair BC, Cortez V, Challa R, Chakravarty D, Tekmal RR, et al. Significance of ER-Src axis in hormonal therapy resistance. Breast Cancer Res Treat 2010; In press PMID: 21184269; http://dx.doi.org/10.1007/s10549-010-1312-2
- Hiscox S, Barrett-Lee P, Borley AC, Nicholson RI. Combining Src inhibitors and aromatase inhibitors: a novel strategy for overcoming endocrine resistance and bone loss. Eur J Cancer 2010; 46:2187 - 2195; PMID: 20471823; http://dx.doi.org/10.1016/j.ejca.2010.04.012