Abstract
In 1889, Dr. Stephen Paget proposed the “seed and soil” hypothesis, which states that cancer cells (the seeds) need the proper microenvironment (the soil) for them to grow, spread and metastasize systemically. In this hypothesis, Dr. Paget rightfully recognized that the tumor microenvironment has an important role to play in cancer progression and metastasis. In this regard, a series of recent studies have elegantly shown that the production of hydrogen peroxide, by both cancer cells and cancer-associated fibroblasts, may provide the necessary “fertilizer,” by driving accelerated aging, DNA damage, inflammation and cancer metabolism, in the tumor microenvironment. By secreting hydrogen peroxide, cancer cells and fibroblasts are mimicking the behavior of immune cells (macrophages/neutrophils), driving local and systemic inflammation, via the innate immune response (NFκB). Thus, we should consider using various therapeutic strategies (such as catalase and/or other anti-oxidants) to neutralize the production of cancer-associated hydrogen peroxide, thereby preventing tumor-stroma co-evolution and metastasis. The implications of these findings for overcoming chemo-resistance in cancer cells are also discussed in the context of hydrogen peroxide production and cancer metabolism.
The “free radical theory of aging” states that progressive defects in mitochondrial function lead to the increased production of reactive oxygen species (ROS), such as hydrogen peroxide, resulting in accumulated DNA damage.Citation1–Citation3 Ultimately, this DNA damage also increases our susceptibility toward the onset of cancer.Citation4–Citation6
But what if cancer cells could facilitate this process too, by producing hydrogen peroxide themselves? In fact, oncogene-induced transformation of cells results in hydrogen peroxide and ROS productionCitation7–Citation10(). And treatment with antioxidants blocks oxidative stress, and in some cases is sufficient to reverse cell transformation.Citation11–Citation13 Similarly, non-transformed cells, including stem cells, require ROS production for cell proliferation.Citation14,Citation15
Normally, epithelial cells only produce hydrogen peroxide during wound healing, initiating the onset of inflammation and myofibroblast conversion.Citation16 In this regard, epithelial cells behave like inflammatory cells, such as macrophages and neutrophils, which are responsible for the vast majority of hydrogen peroxide production during the inflammatory immune response.Citation8–Citation10 Similarly, oxidative stress, via hydrogen peroxide production, is sufficient to convert normal fibroblasts to activated myofibroblasts.Citation17 These activated myofibroblasts then produce hydrogen peroxide themselves, propagating the inflammatory signal.Citation18
In normal wound healing, the production of hydrogen peroxide is shut off. However, in cancer cells and tumor tissues, the production of hydrogen peroxide continues, leading to further DNA damage, inflammation and changes in cellular metabolism. Hydrogen peroxide drives the onset of inflammation, via the activation of NFκB, a master regulator of the innate immune response.Citation19 Hydrogen peroxide also damages DNA, cellular membranes and organelles, resulting in the onset of autophagy and mitophagy, and HIF1 activation.Citation20–Citation24 Mitophagy (the autophagic destruction of mitochondria) results in aerobic glycolysis and lactate production under conditions of oxidative stress.Citation22,Citation25
Similarly, during scar formation, keloid fibroblasts produce hydrogen peroxide, undergo aerobic glycolysis and secrete lactate.Citation26–Citation28 Thus, myofibroblasts also have the capacity to undergo the Warburg Effect (i.e., aerobic glycolysis),Citation26,Citation28 a process that was previously thought to be confined to cancer cells.Citation29,Citation30
This is all consistent with the idea that cancers are wounds that do not heal,Citation31 likely due to the continued production of hydrogen peroxide.
Does Hydrogen Peroxide Functionas “Fertilizer” for Tumor Growth and Metastasis?
In the “seed and soil” hypothesis, the cancer cells (the seeds) require the proper local and systemic environment (the soil) to facilitate tumor growth and metastasis.Citation32–Citation34 Several independent lines of evidence now suggest that hydrogen peroxide may function as the “fertilizer” in this process, by driving accelerated aging, DNA damage, inflammation and cancer metabolism.Citation35–Citation39
Catalase is one of the major enzymes that detoxifies hydrogen peroxide in the body, functioning as a powerful catalytic anti-oxidant. Catalase converts hydrogen peroxide to water and oxygen. It is one of the most catalytically active enzymes known; one molecule of catalase can “neutralize” 40 million molecules of hydrogen peroxide per second.
Thus, it might be predicted that a catalase-deficiency predisposes toward the development of cancer, due to increased levels of hydrogen peroxide. Interestingly, by 9 months of age, female catalase-deficient mice spontaneously develop mammary tumors, which can be prevented by treatment of these mice with dietary Vitamin E, a known antioxidant.Citation40 In contrast, wild-type control mice do not show the development of mammary cancers.Citation40 Thus, the inability to properly detoxify hydrogen peroxide is sufficient to promote tumor initiation.Citation40 A catalase-deficiency also appears to contribute toward the onset of fibrosis,Citation41–Citation44 possibly further promoting tumor growth.
Conversely, in humans, the CC allele of the catalase gene is associated with increased serum catalase activity and a 20% reduction in breast cancers.Citation45 This reduction in breast cancer risk was increased to 40%, if women with the CC allele ate large amounts of vegetables and fruit, that contain antioxidants.Citation45 Similarly, treatment of breast cancer patients with antioxidants (Vitamins C/E) results in substantial reductions in both mortality and recurrence (approaching 40–50%), if patients with radiation therapy are excluded.Citation46
In Japan, a plethora of pre-clinical studies have now shown that “catalase therapy” (either via i.v. or i.p. injection of catalase) in rodent animal models is sufficient to almost completely prevent both tumor recurrence and metastasis.Citation47–Citation53
Transgenic overexpression of catalase in MMTV-PyMT mice (a well-established model of breast cancer) reduces tumor aggressiveness (from high-grade to low-grade) and reduces lung metastatic tumor burden by >12-fold.Citation54,Citation55
Glutathione peroxidase family members also function to detoxify hydrogen peroxide.Citation56 Of the eight known family members (GPX1-8), it appears that GPX4 is the most relevant to our discussion, as it is targeted to mitochondria. Interestingly, overexpression of GPX4 in fibroblasts protects against ROS production, NFκB activation and IL-6 secretion, as well as MMP production.Citation56 In addition, overexpression of GPX4 in cancer cells effectively reduces cell proliferation, tumor growth and metastasis.Citation56
Thus, it appears that hydrogen peroxide does function as “fertilizer” to promote tumor growth, progression and metastasis.
In accordance with this simple notion, hydrogen peroxide production and chronic inflammation (also due to viral and bacterial infections), may be the major drivers of tumor initiation. In fact, the most common “root causes” of cancer worldwide are thought to be due to infectious agents, such as in liver (HBV),Citation57 cervical (HPV),Citation58 nasopharyngeal (EBV),Citation59,Citation60 lung (TB),Citation61 and stomach (H. pylori) Citation62 cancers. Similarly, chronic inflammatory diseases, such as scleroderma,Citation63 ulcerative colitis,Citation64 and Crohn's disease,Citation65 all predispose to the onset of cancer. ROS-producing bacteria have even been implicated in the pathogenesis of colon cancer, which induce aneuploidy (genomic instability) in normal colonocytes via a “bystander effect.”Citation66
Likewise, H. pylori increases the local production of ROS species (including hydrogen peroxide) and RNS (such as nitric oxide) in the stomach.Citation62 This also fits well with the possibility that Wasabi consumption [a condiment that actively produces hydrogen peroxide (horseradish)] could explain the observation that Japanese men have the highest rate of gastric cancer in the world, ∼7–10 times higher than in the United States.Citation67
As discussed below, ROS-induced cytokine production and inflammationCitation65 also further drive autophagy (the production of high-energy nutrients) in the tumor microenvironment,Citation36 thereby producing “fuel” to feed “hungry” cancer cells.
Hydrogen Peroxide and the Warburg Effect in the Tumor Stroma: Metabolic Coupling
If cancer cells produce and secrete hydrogen peroxide, then this also has important metabolic consequences for the tumor microenvironment.
These stromal effects were recently observed by co-culturing MCF7 breast cancer cells with immortalized fibroblasts.Citation23,Citation24,Citation68 Interestingly, at day 2 of co-culture most of the ROS production occurred in MCF7 cells.Citation35 This ROS production was reduced to baseline levels by co-incubation with extracellular catalase, identifying the predominant ROS species as hydrogen peroxide.Citation35 In contrast, by day 5, most of the ROS production occurred in the cancer-associated fibroblasts.Citation23,Citation35 Thus, it appears that cancer cells initially secrete hydrogen peroxide, which then triggers oxidative stress in neighboring fibroblasts.
Importantly, we observed using this MCF7-fibroblast co-culture system, that hydrogen peroxide secretion activates NFκB and HIF1 in cancer-associated fibroblasts, driving stromal inflammation and aerobic glycolysis, as well as autophagy.Citation23,Citation24 Then, autophagy, mitophagy and aerobic glycolysis in cancer-associated fibroblasts provides high-energy nutrients and recycled building blocks (such as lactate, ketones and glutamine) to literally “feed” cancer cells.Citation23,Citation24 These high-energy nutrients (such as lactate) are sufficient to promote mitochondrial biogenesis and oxidative mitochondrial metabolism in cancer cells, thereby driving tumor growth.Citation23,Citation24,Citation69,Citation70 Under these conditions, most of the DNA damage occurs in the cancer-associated fibroblasts, as the MCF7 cancer cells effectively mount an anti-oxidant defense by upregulating key proteins, such as peroxiredoxin-1 and TIGAR.Citation23,Citation24 Thus, hydrogen peroxide helps produce “glycolytic fibroblasts” to feed hungry “oxidative cancer cells.”
In order to pheno-copy the effects of hydrogen peroxide on cancer-associated fibroblasts, we overexpressed activated HIF1α or NFκB in normal stromal fibroblasts.Citation22 Notably, both HIF1α and NFκB-expressing fibroblasts undergo mitophagy, produce more lactate and stimulate the growth of human breast cancer xenografts, by up to ∼3-fold.Citation22 Similarly, a loss of caveolin-1 (Cav-1) occurs in fibroblasts undergoing oxidative stress, as it is destroyed by lysosomal degradation/autophagy.Citation23,Citation24,Citation68 In this sense, loss of stromal Cav-1 is a marker for the effects of hydrogen peroxide and oxidative stress.Citation37,Citation71,Citation72 Cav-1-deficient fibroblasts show increases in mitochondrial oxidative stress, with a shift toward aerobic glycolysis.Citation71–Citation74 In this regard, they behave like “glycolytic” myofibroblasts, which also show a loss of Cav-1 expression.Citation26,Citation75,Citation76 Most importantly, Cav-1-deficient fibroblasts promote tumor growth up to ∼4-fold, when co-injected with MDA-MB-231 triple negative human breast cancer cells.Citation77,Citation78 Cav-1-deficent fibroblasts and hydrogen peroxide treated fibroblasts also share the same proteomic profile, with the upregulation of myofibroblast markers, glycolytic enzymes and anti-oxidant proteins.Citation22,Citation74,Citation78–Citation80
Consistent with these findings, a loss of stromal Cav-1 is a powerful biomarker for a lethal tumor microenvironment, in breast and prostate cancers.Citation37,Citation81–Citation89 More specifically, in breast cancer patients, a loss of stromal Cav-1 is associated with early tumor recurrence, metastasis, tamoxifen-resistance and poor clinical outcome.Citation84 For example, in triple negative breast cancer patients, a loss of stromal Cav-1 predicts a 5-year survival of less than 10%. In the same patient cohort, high stromal Cav-1 was associated with a good prognosis, with >75% survival at 12 years post-diagnosis.Citation83
Laser-capture micro-dissection of the tumor stroma from Cav-1-negative human breast cancer patients also reveals the presence of transcriptional gene signature(s) that are consistent with the local damaging effects of chronic hydrogen peroxide production.Citation37 These gene signature(s) include aging, Alzheimer disease, DNA damage, oxidative stress, inflammation, HIF1 and NFκB-activation, as well as autophagy and aerobic glycolysis.Citation37 Thus, localized hydrogen peroxide production may underlie the prognostic value of a loss of stromal Cav-1 as a biomarker. In this context, the Alzheimer disease brain gene signature (a marker of oxidative stress) was specifically associated with breast cancer metastasis ().Citation72,Citation73
Hydrogen Peroxide and the Lactate Shuttle
As discussed above, cancer cells use hydrogen peroxide as a weapon to extract nutrients from adjacent fibroblasts, via the stromal induction of autophagy and mitophagy.Citation23,Citation24,Citation35 This results in the production of L-lactate in cancer-associated fibroblasts, via aerobic glycolysis. Then, these fibroblasts secrete L-lactate as a waste product and cancer cells use L-lactate as “fuel” to burn in their mitochondrial TCA cycle, via oxidative phosphorylation.Citation23,Citation24,Citation35 As such, there is metabolic-coupling between “glycolytic” fibroblasts and “oxidative” cancer cells.Citation90 We have previously termed this phenomenon the “reverse Warburg effect,” as aerobic glycolysis takes place in fibroblasts, rather than cancer cells.Citation74
Interestingly, the concept of a “lactate shuttle” and that glycolytic and oxidative cells share L-lactate is not new (). Lactate shuttles are now known to occur in skeletal muscle, the brain and even the female genital tract, and this is a normal physiological process. In skeletal muscle, fast-twitch fibers are glycolytic and slow-twitch fibers are oxidative.Citation91 In the brain, astrocytes take up glucose and secrete lactate that is then transferred to neurons.Citation92–Citation95 This is known as “neuron-glia metabolic coupling.”Citation92–Citation95 Finally, in the female genital tract, granulosa cells are glycolytic and produce L-lactate to “feed” the oocyte, which is oxidative and uses mitochondrial metabolism.Citation96–Citation104 Thus, this metabolic-coupling mechanism appears to be widely used by the body, to maintain proper homeostasis or energy balance.
In this context, it is believed that cancer-associated fibroblasts produce ROS (including hydrogen peroxide), and that this helps to maintain their glycolytic state.Citation23,Citation24,Citation35 Similarly, fast-twitch skeletal muscle fibers are glycolytic and are the largest producers of hydrogen peroxide, as compared with slow-twitch fibers.Citation105 Consistent with this hypothesis, MCT4, the main transporter for the extrusion of L-lactate from glycolytic cells, is induced by oxidative stress in fibroblasts.Citation38
Oxidative Stress, Metabolic-Coupling and Drug Resistance
What if acquired resistance to chemotherapeutic agents was a metabolic and stromal phenomenon? Then, we might be able to reverse drug-resistance with drug combinations that target both the tumor stroma and the epithelial cancer cells, resulting in metabolic uncoupling.
We recently tested this idea using the MCF7-fibroblast co-culture system.Citation35,Citation68 MCF7 cells are a well-established ER(+) breast cancer cell line (). As predicted, MCF7 cancer cells cultured alone were extremely sensitive to tamoxifen-induced apoptosis, which targets ER-signaling.Citation106 Remarkably, under co-culture conditions with fibroblasts, MCF7 cells became nearly completely resistant to the pro-apoptotic effects of tamoxifen.Citation106
Metabolic analysis of this phenomenon indicated that tamoxifen-resistance in co-cultured MCF7 cancer cells was due to a shift from a “glycolytic” to an “oxidative state,” with increased mitochondrial activity and decreased glucose uptake.Citation35,Citation106 Thus, we searched for a drug combination that could overcome this stromal-based tamoxifen-resistance. In this context, we observed that the addition of tamoxifen plus dasastinib was indeed sufficient to prevent stromal-based tamoxifen-resistance and restore sensitivity toward tamoxifen-induced apoptosis in cancer cells.Citation35,Citation106 Under these conditions, we observed that MCF7 cancer cells were shifted back toward the “glycolytic” state and hydrogen-peroxide production was reduced to baseline levels.Citation35,Citation106 Thus, tamoxifen plus dasatinib maintained both fibroblasts and cancer cells in a “glycolytic state,” with minimal ROS production and high-sensitivity toward apoptosis, likely due to an absence of metabolic-coupling.Citation35,Citation106 This is consistent with idea that tamoxifen plus dasatinib has a generalized anti-oxidant effect.
Interestingly, under these same coculture conditions, treatment with catalase to neurtralize hydrogen peroxide, prevented ROS production and dramatically induced apoptosis in co-cultured MCF7 cancer cells.Citation35 Thus, in co-culture, catalase has the same anti-oxidant and pro-apoptotic effects on MCF7 cancer cells, as tamoxifen plus dasatinib.Citation35,Citation106 Mechanistically, this may explain why “catalase therapy” in pre-clinical models prevents both tumor recurrence and metastasis, as it “cuts off the metabolic fuel supply.”
Clinical Utility of Hydrogen Peroxide for Cancer Diagnosis
Since cancer cells and tumors both produce large amounts of hydrogen peroxide,Citation7–Citation10 the detection of hydrogen peroxide production may be an important new approach toward cancer diagnosis and for the development of novel imaging techniques.Citation51,Citation107–Citation109
For example, cancer patients (such as those with breast and lung tumors) can be distinguished from normal controls, based on the detection of hydrogen peroxide in their exhaled breath.Citation110–Citation112 Consistent with the idea that hydrogen peroxide may also originate from the tumor stroma, patients with interstitial pulmonary fibrosis show increased levels of hydrogen peroxide in their exhaled breath.Citation113 Also, patients with pulmonary fibrosis show a loss of stromal Cav-1 expression, providing another mechanistic link.Citation114–Citation116
Finally, new imaging probes have also been invented that can detect hydrogen peroxide production in pre-clinical models, such as human tumor xenografts.Citation117 In this regard, PET imaging of human tumors, with Fluoro-2-deoxy-D-glucose (F-2-DG), may be already detecting cancer-associated fibroblasts,Citation35 that are undergoing aerobic glycolysis, due to oxidative stress induced by hydrogen peroxide production and inflammation.
In further support of this notion, PET imaging with F-2-DG can be effectively used to detect many distinct inflammatory diseases, both of infectious and non-infectious origins. These conditions include fever of unknown origin (FUO) and bacteremic foci, as well as graft rejection in liver and renal transplants.Citation118–Citation124
Summary: Hydrogen Peroxide in Tumor Initiation, Progression and Metastasis
In summary, hydrogen peroxide is a known carcinogen, and is associated with mutatgenic potential, resulting in a positive Ames test in bacteria.Citation125,Citation126 Thus, hydrogen peroxide may also induce DNA damage in normal epithelial cells.Citation126,Citation127 In fact, various known oncogenic stimuli are sufficient to drive hydrogen peroxide production, such as environmental stressors, carcinogens, radiation (including UV exposure), as well as inflammation and normal aging.Citation126–Citation137 As such, hydrogen peroxide may be directly involved in the earliest tumor initiating events.Citation126,Citation127,Citation137,Citation138 In accordance with this notion, addition of catalase to the extracellular tissue culture media is indeed sufficient to prevent or dramatically delay the onset of cell transformation and genomic instability, when normal cells are presented with an oncogenic stimulus.Citation138
Thus, we propose that carcinogenesis may be a 3-step process, related to hydrogen peroxide production, as outlined in and B. In this cascade or chain of hydrogen peroxide production, ROS and oxidative stress is passed from the oncogenic stimulus and normal epithelial cells (step 1), to the cancer cells (step 2), and then to the tumor stroma (step 3). Step 1 is hydrogen peroxide induced tumor initiation, while steps 2 and 3 are hydrogen peroxide induced tumor progression and metastasis. Hydrogen peroxide induced DNA damage in the tumor microenvironment may also account for the “field effect,” such that an entire area of tissue appears normal, but has been “cancerized” by oxidative stress and DNA damage, leading to premature aging, inflammation, cancer metabolism and metastasis.Citation23,Citation35,Citation139 Indeed, lactate production in the tumor microenvironment is sufficient to promote an ∼10-fold increase in lung metastasis.Citation70
Figures and Tables
Figure 1 Cancer cells initially produce hydrogen peroxide, which “fertilizes” the tumor microenvironment. See text for details. In this model, cancer cells initially produce and secrete hydrogen peroxide that induces oxidative stress in adjacent cancer-associated fibroblasts. Then, cancer cells mount an anti-oxidant defense by expressing key proteins, such as the peroxiredoxins and TIGAR. Oxidative stress and ROS production in cancer associated fibroblasts then “fertilizes” the tumor microenvironment via myofibroblast differentiation and DNA damage, autophagy/mitophagy, aerobic glycolysis and inflammation. Oxidative stress activates two major transcription factors in cancer-associated fibroblasts, namely HIF1α (aerobic glycolysis) and NFκB (inflammation), which both contribute to the induction of autophagy and mitophagy. ROS production in the tumor microenvironment also has a mutagenic “Bystander Effect” on cancer cells, driving their evolution toward a more aggressive phenotype, aneuploidy and genomic instability. Importantly, antioxidants that neutralize hydrogen peroxide [such as catalase and N-acetyl-cysteine (NAC)] should prevent “fertilization” of the soil.
![Figure 1 Cancer cells initially produce hydrogen peroxide, which “fertilizes” the tumor microenvironment. See text for details. In this model, cancer cells initially produce and secrete hydrogen peroxide that induces oxidative stress in adjacent cancer-associated fibroblasts. Then, cancer cells mount an anti-oxidant defense by expressing key proteins, such as the peroxiredoxins and TIGAR. Oxidative stress and ROS production in cancer associated fibroblasts then “fertilizes” the tumor microenvironment via myofibroblast differentiation and DNA damage, autophagy/mitophagy, aerobic glycolysis and inflammation. Oxidative stress activates two major transcription factors in cancer-associated fibroblasts, namely HIF1α (aerobic glycolysis) and NFκB (inflammation), which both contribute to the induction of autophagy and mitophagy. ROS production in the tumor microenvironment also has a mutagenic “Bystander Effect” on cancer cells, driving their evolution toward a more aggressive phenotype, aneuploidy and genomic instability. Importantly, antioxidants that neutralize hydrogen peroxide [such as catalase and N-acetyl-cysteine (NAC)] should prevent “fertilization” of the soil.](/cms/asset/41082e57-d8fb-452d-975a-0ecc69f56fa2/kccy_a_10916870_f0001.gif)
Figure 2 Transcriptional overlap between Alzheimer brain disease and the breast cancer tumor stroma: association with metastasis. Venn diagrams show that the gene signature for Alzheimer disease brain (1,133 gene transcripts; a signature for inflammation and oxidative stress) is most closely related to the primary tumor stroma of breast cancer patients that will undergo metastasis (1,182 gene transcripts), with a p value of nearly 10−6 (lower part). this finding is consistent with the idea that oxidative stress in the tumor stroma is associated with metastasis. Virtually identical results were also obtained with the gene signature for Cav-1-deficient stromal cells (a model for oxidative stress), which showed striking similarities toward both Alzheimer disease brain and breast cancer metastasis. reproduced with permission from.Citation72,Citation73
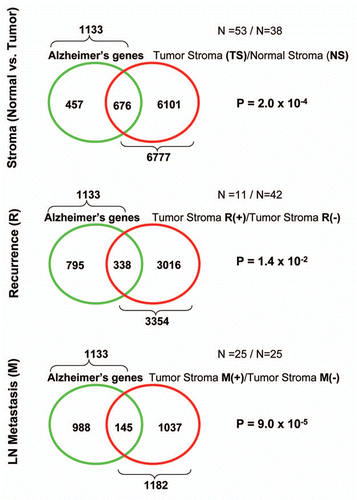
Figure 3 Energy transfer in normal metabolism and cancer: the lactate shuttle. The concept that glycolytic (green) and oxidative (red) cells can share L-lactate is shown. This is known as the “lactate shuttle,” and normally occurs in skeletal muscle, the brain and the female genital tract. In skeletal muscle, fast-twitch fibers are glycolytic and slow-twitch fibers are oxidative. In the brain, astrocytes take up glucose and secrete lactate that is then transferred to neurons. This is known as “Neuron-Glia Metabolic Coupling.” in the female genital tract, granulosa cells are glycolytic and produce L-lactate to “feed” the oocyte, which is oxidative and uses mitochondrial metabolism. Thus, this metabolic-coupling mechanism is widely used by the body, to maintain proper homeostasis or energy balance. Similarly, in human tumors, cancer-associated fibroblasts are glycolytic and cancer cells are oxidative. This is known as the “reverse Warburg effect.” Mono-carboxylate transporters (MCTs) function to shuttle the L-lactate from glycolytic cells (MCT4) to oxidative cells (MCT1/2).
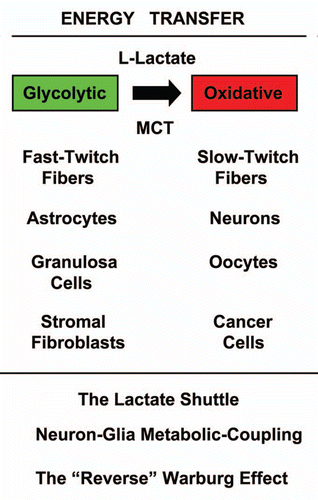
Figure 4 Understanding the stromal and metabolic basis of resistance to chemotherapy: parasitic cancer metabolism. Part 1: Fibroblasts cultured alone are oxidative, and show increased mitochondrial activity. In contrast, MCF7 cells cultured alone are glycolytic and are sensitive to tamoxifen-induced apoptosis. Part 2: However, during co-culture, cancer cells and fibroblasts undergo a complete reversal of their metabolic states. in this role reversal, MCF7 cells become oxidative and fibroblasts become glycolytic. This change is initially driven by hydrogen peroxide production in MCF7 cells, which shifts adjacent fibroblasts toward the glycolytic state, via oxidative stress. Then, under these conditions, there is a net energy transfer (in the form of nutrients, such as L-lactate; see the black arrow) from glycolytic fibroblasts to oxidative cancer cells, which confers resistance to tamoxifen-induced apoptosis. Part 3: Incubation of co-cultures with tamoxifen plus dasatinib shifts both cell types toward a glycolytic state, with decreased ROS production. As such, there is no net energy transfer. These conditions sensitize MCF7 toward apoptosis, reversing their tamoxifen-resistant phenotype. Mito, mitochondria.
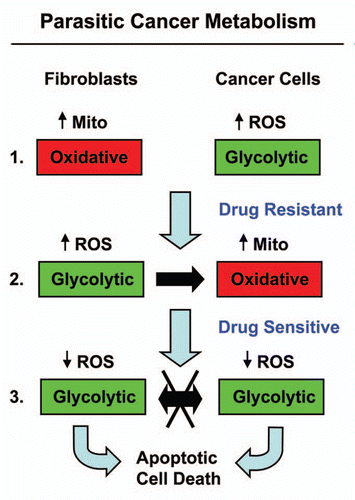
Figure 5 Three-step carcinogenesis: hydrogen peroxide in tumor initiation, progression and metastasis. (A) Tumor Initiation (step 1). Oncogenic stimuli (such as carcinogens, UV rays, inflammation and aging) initiate hydrogen peroxide production, leading to DNA damage in normal epithelial cells. Such DNA damage mimics the “wounding process,” thus driving oncogene activation, tumor suppressor inactivation and cell transformation. This leads to the formation of cancer cells, via mutagenesis and ensuing genomic instability. (B) Tumor Progression and Metastasis (steps 2 and 3). Once cancer cells are formed via oncogene activation in normal epithelial cells, then these cancer cells also begin to produce and secrete hydrogen peroxide (Step 2) to promote tumor-stroma co-evolution and metastasis (Step 3). In part a, cancer cells secrete hydrogen peroxide, which induces oxidative stress in neighboring stromal cells, such as fibroblasts. In part b, oxidative stress in fibroblasts leads to ROS production in the tumor stroma, which then further mutagenizes the cancer cells, allowing them to evolve to a more aggressive state, driving stromal lactate production and metastasis. Finally, in part c, hydrogen peroxide and rOs production could also mutagenize adjacent normal epithelial cells, further driving the formation of new cancer cells. This step, part c, may also account for the “field effect,” in which an entire area of tissue appears normal, but has been “cancerized” by hydrogen peroxide, oxidative stress and DNA damage.
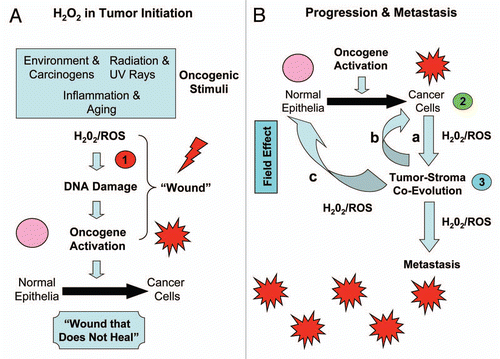
Acknowledgments
F.S. and her laboratory were supported by grants from the W.W. Smith Charitable Trust, the Breast Cancer Alliance (BCA) and a Research Scholar Grant from the American Cancer Society (ACS). M.P.L. was supported by grants from the NIH/NCI (R01-CA-080250; R01-CA-098779; R01-CA-120876; R01-AR-055660), and the Susan G. Komen Breast Cancer Foundation. R.G.P. was supported by grants from the NIH/NCI (R01-CA-70896, R01-CA-75503, R01-CA-86072 and R01-CA-107382) and the Dr. Ralph and Marian C. Falk Medical Research Trust. The Kimmel Cancer Center was supported by the NIH/NCI Cancer Center Core grant P30-CA-56036 (to R.G.P.). Funds were also contributed by the Margaret Q. Landenberger Research Foundation (to M.P.L.). This project is funded, in part, under a grant with the Pennsylvania Department of Health (to M.P.L. and F.S.). The Department specifically disclaims responsibility for any analyses, interpretations or conclusions. This work was also supported, in part, by a Centre grant in Manchester from Breakthrough Breast Cancer in the UK (to A.H.) and an Advanced ERC Grant from the European Research Council.
References
- Woo DK, Shadel GS. Mitochondrial stress signals revise an old aging theory. Cell 2011; 144:11 - 12; PMID: 21215364;; http://dx.doi.org/10.1016/j.cell.2010.12.023
- Treiber N, Maity P, Singh K, Kohn M, Keist AF, Ferchiu F, et al. Accelerated aging phenotype in mice with conditional deficiency for mitochondrial superoxide dismutase in the connective tissue. Aging Cell 2011; 10:239 - 254; PMID: 21108731; http://dx.doi.org/10.1111/j.1474-9726.2010.00658.x
- Schriner SE, Linford NJ, Martin GM, Treuting P, Ogburn CE, Emond M, et al. Extension of murine life span by overexpression of catalase targeted to mitochondria. Science 2005; 308:1909 - 1911; PMID: 15879174; http://dx.doi.org/10.1126/science.1106653
- Gil Del Valle L. Oxidative stress in aging: Theoretical outcomes and clinical evidences in humans. Biomed Pharmacother 2011; In press
- Blagosklonny MV. Increasing healthy lifespan by suppressing aging in our lifetime: preliminary proposal. Cell Cycle 2010; 9:4788 - 4794; PMID: 21150328; http://dx.doi.org/10.4161/cc.9.24.14360
- Blagosklonny MV, Campisi J, Sinclair DA. Aging: past, present and future. Aging (Albany NY) 2009; 1:1 - 5; PMID: 20157590
- Hole PS, Pearn L, Tonks AJ, James PE, Burnett AK, Darley RL, et al. Ras-induced reactive oxygen species promote growth factor-independent proliferation in human CD34+ hematopoietic progenitor cells. Blood 2010; 115:1238 - 1246; PMID: 20007804; http://dx.doi.org/10.1182/blood-2009-06-222869
- Szatrowski TP, Nathan CF. Production of large amounts of hydrogen peroxide by human tumor cells. Cancer Res 1991; 51:794 - 798; PMID: 1846317
- Burdon RH. Superoxide and hydrogen peroxide in relation to mammalian cell proliferation. Free Radic Biol Med 1995; 18:775 - 794; PMID: 7750801; http://dx.doi.org/10.1016/0891-5849(94)00198-S
- Feng Y, Santoriello C, Mione M, Hurlstone A, Martin P. Live imaging of innate immune cell sensing of transformed cells in zebrafish larvae: parallels between tumor initiation and wound inflammation. PLoS Biol 2010; 8:1000562; PMID: 21179501; http://dx.doi.org/10.1371/journal.pbio.1000562
- Alexandrova AY, Kopnin PB, Vasiliev JM, Kopnin BP. ROS upregulation mediates Ras-induced changes of cell morphology and motility. Exp Cell Res 2006; 312:2066 - 2073; PMID: 16624288; http://dx.doi.org/10.1016/j.yexcr.2006.03.004
- Martin KR, Saulnier MJ, Kari FW, Barrett JC, French JE. Timing of supplementation with the antioxidant N-acetyl-L-cysteine reduces tumor multiplicity in novel, cancer-prone p53 haploinsufficient Tg.AC (v-Ha-ras) transgenic mice but has no impact on malignant progression. Nutr Cancer 2002; 43:59 - 66; PMID: 12467136; http://dx.doi.org/10.1207/S15327914NC431_7
- Havre PA, O'Reilly S, McCormick JJ, Brash DE. Transformed and tumor-derived human cells exhibit preferential sensitivity to the thiol antioxidants, N-acetyl cysteine and penicillamine. Cancer Res 2002; 62:1443 - 1449; PMID: 11888918
- Kim JH, Park SH, Park SG, Choi JS, Xia Y, Sung JH. The Pivotal Role of Reactive Oxygen Species Generation in the Hypoxia-Induced Stimulation of Adipose-Derived Stem Cells. Stem Cells Dev 2011; In press
- Grek CL, Townsend DM, Tew KD. The impact of redox and thiol status on the bone marrow: Pharmacological intervention strategies. Pharmacol Ther 2011; 129:172 - 184; PMID: 20951732; http://dx.doi.org/10.1016/j.pharmthera.2010.09.008
- Niethammer P, Grabher C, Look AT, Mitchison TJ. A tissue-scale gradient of hydrogen peroxide mediates rapid wound detection in zebrafish. Nature 2009; 459:996 - 999; PMID: 19494811; http://dx.doi.org/10.1038/nature08119
- Toullec A, Gerald D, Despouy G, Bourachot B, Cardon M, Lefort S, et al. Oxidative stress promotes myofibroblast differentiation and tumour spreading. EMBO Mol Med 2010; 2:211 - 230; PMID: 20535745; http://dx.doi.org/10.1002/emmm.201000073
- Waghray M, Cui Z, Horowitz JC, Subramanian IM, Martinez FJ, Toews GB, et al. Hydrogen peroxide is a diffusible paracrine signal for the induction of epithelial cell death by activated myofibroblasts. FASEB J 2005; 19:854 - 856; PMID: 15857893
- Kobayashi Y, Nishikawa M, Hyoudou K, Yamashita F, Hashida M. Hydrogen peroxide-mediated nuclear factor kappaB activation in both liver and tumor cells during initial stages of hepatic metastasis. Cancer Sci 2008; 99:1546 - 1552; PMID: 18754865; http://dx.doi.org/10.1111/j.1349-7006.2008.00856.x
- Dai DF, Rabinovitch P. Mitochondrial oxidative stress mediates induction of autophagy and hypertrophy in angiotensin-II treated mouse hearts. Autophagy 2011; 7:917 - 918; PMID: 21505274
- Roszkowski K, Jozwicki W, Blaszczyk P, Mucha-Malecka A, Siomek A. Oxidative damage DNA: 8-oxoGua and 8-oxodG as molecular markers of cancer. Med Sci Monit 2011; 17:329 - 333; PMID: 21629187
- Chiavarina B, Whitaker-Menezes D, Migneco G, Martinez-Outschoorn UE, Pavlides S, Howell A, et al. HIF1alpha functions as a tumor promoter in cancer associated fibroblasts, and as a tumor suppressor in breast cancer cells: Autophagy drives compartment-specific oncogenesis. Cell Cycle 2010; 9:3534 - 3551; PMID: 20864819; http://dx.doi.org/10.4161/cc.9.17.12908
- Martinez-Outschoorn UE, Balliet RM, Rivadeneira DB, Chiavarina B, Pavlides S, Wang C, et al. Oxidative stress in cancer associated fibroblasts drives tumor-stroma co-evolution: A new paradigm for understanding tumor metabolism, the field effect and genomic instability in cancer cells. Cell Cycle 2010; 9:3256 - 3276; PMID: 20814239; http://dx.doi.org/10.4161/cc.9.16.12553
- Martinez-Outschoorn UE, Trimmer C, Lin Z, Whitaker-Menezes D, Chiavarina B, Zhou J, et al. Autophagy in cancer associated fibroblasts promotes tumor cell survival: Role of hypoxia, HIF1 induction and NFkappaB activation in the tumor stromal microenvironment. Cell Cycle 2010; 9:3515 - 3533; PMID: 20855962; http://dx.doi.org/10.4161/cc.9.17.12928
- Lisanti MP, Martinez-Outschoorn UE, Chiavarina B, Pavlides S, Whitaker-Menezes D, Tsirigos A, et al. Understanding the “lethal” drivers of tumor-stroma co-evolution: emerging role(s) for hypoxia, oxidative stress and autophagy/mitophagy in the tumor micro-environment. Cancer Biol Ther 2011; 10:537 - 542; PMID: 20861671; http://dx.doi.org/10.4161/cbt.10.6.13370
- Vincent AS, Phan TT, Mukhopadhyay A, Lim HY, Halliwell B, Wong KP. Human skin keloid fibroblasts display bioenergetics of cancer cells. J Invest Dermatol 2008; 128:702 - 709; PMID: 17943178; http://dx.doi.org/10.1038/sj.jid.5701107
- De Felice B, Garbi C, Santoriello M, Santillo A, Wilson RR. Differential apoptosis markers in human keloids and hypertrophic scars fibroblasts. Mol Cell Biochem 2009; 327:191 - 201; PMID: 19224335; http://dx.doi.org/10.1007/s11010-009-0057-x
- Ueda K, Yasuda Y, Furuya E, Oba S. Inadequate blood supply persists in keloids. Scand J Plast Reconstr Surg Hand Surg 2004; 38:267 - 271; PMID: 15513596; http://dx.doi.org/10.1080/02844310410029552
- Warburg O. On respiratory impairment in cancer cells. Science 1956; 124:269 - 270; PMID: 13351639
- Warburg O. On the origin of cancer cells. Science 1956; 123:309 - 314; PMID: 13298683; http://dx.doi.org/10.1126/science.123.3191.309
- Sieweke MH, Bissell MJ. The tumor-promoting effect of wounding: a possible role for TGFbetainduced stromal alterations. Crit Rev Oncog 1994; 5:297 - 311; PMID: 7849089
- Fidler IJ. The pathogenesis of cancer metastasis: the ‘seed and soil’ hypothesis revisited. Nat Rev Cancer 2003; 3:453 - 458; PMID: 12778135; http://dx.doi.org/10.1038/nrc1098
- Talmadge JE, Fidler IJ. AACR centennial series: the biology of cancer metastasis: historical perspective. Cancer Res 2010; 70:5649 - 5669; PMID: 20610625; http://dx.doi.org/10.1158/0008-5472.CAN-10-1040
- Fokas E, Engenhart-Cabillic R, Daniilidis K, Rose F, An HX. Metastasis: the seed and soil theory gains identity. Cancer Metastasis Rev 2007; 26:705 - 715; PMID: 17786535; http://dx.doi.org/10.1007/s10555-0079088-5
- Martinez-Outschoorn UE, Lin Z, Trimmer C, Flomenberg N, Wang C, Pavlides S, et al. Cancer cells metabolically “fertilize” the tumor microenvironment with hydrogen peroxide, driving the reverse Warburg effect: Implications for PET imaging of human tumors. Cell Cycle 2011; 10 In press
- Martinez-Outschoorn UE, Whitaker-Menezes D, Lin Z, Flomenberg N, Howell A, Pestell RG, et al. Cytokine production and inflammation drive autophagy in the tumor microenvironment: Role of stromal caveolin-1 as a key regulator. Cell Cycle 2011; 10:1784 - 1793; PMID: 21566463
- Witkiewicz AK, Kline J, Queenan M, Brody JR, Tsirigos A, Bilal E, et al. Molecular profiling of a lethal tumor microenvironment, as defined by stromal caveolin-1 status in breast cancers. Cell Cycle 2011; 10:1794 - 1809; PMID: 21521946
- Whitaker-Menezes D, Martinez-Outschoorn UE, Lin Z, Ertel A, Flomenberg N, Witkiewicz AK, et al. Evidence for a stromal-epithelial “lactate shuttle” in human tumors: MCT4 is a marker of oxidative stress in cancer-associated fibroblasts. Cell Cycle 2011; 10:1772 - 1783; PMID: 21558814
- Kasapovic J, Pejic S, Stojiljkovic V, Todorovic A, Radosevic-Jelic L, Saicic ZS, et al. Antioxidant status and lipid peroxidation in the blood of breast cancer patients of different ages after chemotherapy with 5-fluorouracil, doxorubicin and cyclophosphamide. Clin Biochem 2010; 43:1287 - 1293; PMID: 20713039; http://dx.doi.org/10.1016/j.clinbiochem.2010.08.009
- Ishii K, Zhen LX, Wang DH, Funamori Y, Ogawa K, Taketa K. Prevention of mammary tumorigenesis in acatalasemic mice by vitamin E supplementation. Jpn J Cancer Res 1996; 87:680 - 684; PMID: 8698615
- Kobayashi M, Sugiyama H, Wang DH, Toda N, Maeshima Y, Yamasaki Y, et al. Catalase deficiency renders remnant kidneys more susceptible to oxidant tissue injury and renal fibrosis in mice. Kidney Int 2005; 68:1018 - 1031; PMID: 16105032; http://dx.doi.org/10.1111/j.1523-755.2005.00494.x
- Sunami R, Sugiyama H, Wang DH, Kobayashi M, Maeshima Y, Yamasaki Y, et al. Acatalasemia sensitizes renal tubular epithelial cells to apoptosis and exacerbates renal fibrosis after unilateral ureteral obstruction. Am J Physiol Renal Physiol 2004; 286:1030 - 1038; PMID: 14722014; http://dx.doi.org/10.1152/ajprenal.00266.2003
- Fukuoka N, Sugiyama H, Inoue T, Kikumoto Y, Takiue K, Morinaga H, et al. Increased susceptibility to oxidant-mediated tissue injury and peritoneal fibrosis in acatalasemic mice. Am J Nephrol 2008; 28:661 - 668; PMID: 18337633; http://dx.doi.org/10.1159/000121357
- Odajima N, Betsuyaku T, Nagai K, Moriyama C, Wang DH, Takigawa T, et al. The role of catalase in pulmonary fibrosis. Respir Res 2010; 11:183; PMID: 21190578; http://dx.doi.org/10.1186/1465-9921-11-183
- Ahn J, Gammon MD, Santella RM, Gaudet MM, Britton JA, Teitelbaum SL, et al. Associations between breast cancer risk and the catalase genotype, fruit and vegetable consumption, and supplement use. Am J Epidemiol 2005; 162:943 - 952; PMID: 16192345; http://dx.doi.org/10.1093/aje/kwi306
- Nechuta S, Lu W, Chen Z, Zheng Y, Gu K, Cai H, et al. Vitamin supplement use during breast cancer treatment and survival: a prospective cohort study. Cancer Epidemiol Biomarkers Prev 2011; 20:262 - 271; PMID: 21177425; http://dx.doi.org/10.1158/1055-9965.EPI-101072
- Hyoudou K, Nishikawa M, Ikemura M, Kobayashi Y, Mendelsohn A, Miyazaki N, et al. Prevention of pulmonary metastasis from subcutaneous tumors by binary system-based sustained delivery of catalase. J Control Release 2009; 137:110 - 115; PMID: 19361547; http://dx.doi.org/10.1016/j.jconrel.2009.04.005
- Hyoudou K, Nishikawa M, Kobayashi Y, Umeyama Y, Yamashita F, Hashida M. PEGylated catalase prevents metastatic tumor growth aggravated by tumor removal. Free Radic Biol Med 2006; 41:1449 - 1458; PMID: 17023272; http://dx.doi.org/10.1016/j.freeradbiomed.2006.08.004
- Nishikawa M. Reactive oxygen species in tumor metastasis. Cancer Lett 2008; 266:53 - 59; PMID: 18362051; http://dx.doi.org/10.1016/j.canlet.2008.02.031
- Nishikawa M, Hashida M. Inhibition of tumour metastasis by targeted delivery of antioxidant enzymes. Expert Opin Drug Deliv 2006; 3:355 - 369; PMID: 16640496; http://dx.doi.org/10.1517/17425247.3.3.355
- Nishikawa M, Hashida M, Takakura Y. Catalase delivery for inhibiting ROS-mediated tissue injury and tumor metastasis. Adv Drug Deliv Rev 2009; 61:319 - 326; PMID: 19385054; http://dx.doi.org/10.1016/j.addr.2009.01.001
- Nishikawa M, Tamada A, Hyoudou K, Umeyama Y, Takahashi Y, Kobayashi Y, et al. Inhibition of experimental hepatic metastasis by targeted delivery of catalase in mice. Clin Exp Metastasis 2004; 21:213 - 221; PMID: 15387371; http://dx.doi.org/10.1023/B:CLIN.0000037706.13747.5e
- Nishikawa M, Tamada A, Kumai H, Yamashita F, Hashida M. Inhibition of experimental pulmonary metastasis by controlling biodistribution of catalase in mice. Int J Cancer 2002; 99:474 - 479; PMID: 11992420; http://dx.doi.org/10.1002/ijc.10387
- Goh J, Enns L, Fatemie S, Hopkins H, Morton J, Pettan-Brewer C, et al. Mitochondrial targeted catalase suppresses invasive breast cancer in mice. BMC Cancer 2011; 11:191; PMID: 21605372; http://dx.doi.org/10.1186/1471-2407-11-191
- Sotgia F, Martinez-Outschoorn UE, Lisanti MP. Mitochondrial Oxidative Stress Drives Tumor Progression and Metastasis: Should We Use Antioxidants As A Key Component of Cancer Treatment and Prevention?. BMC Med 2011; 9:62; PMID: 21605374; http://dx.doi.org/10.1186/1741-7015-9-62
- Brigelius-Flohe R, Kipp A. Glutathione peroxidases in different stages of carcinogenesis. Biochim Biophys Acta 2009; 1790:1555 - 1568
- Michielsen P, Ho E. Viral hepatitis B and hepatocellular carcinoma. Acta Gastroenterol Belg 2011; 74:48; PMID: 21563647
- Stanley M. Pathology and epidemiology of HPV infection in females. Gynecol Oncol 2010; 117:5 - 10; PMID: 20304221; http://dx.doi.org/10.1016/j.ygyno.2010.01.024
- Singhi AD, Califano J, Westra WH. High-risk human papillomavirus in nasopharyngeal carcinoma. Head Neck 2011; In press
- Yu KJ, Hsu WL, Pfeiffer RM, Chiang CJ, Wang CP, Lou PJ, et al. Prognostic utility of anti-EBV antibody testing for defining NPC risk among individuals from high-risk NPC families. Clin Cancer Res 2011; 17:1906 - 1914; PMID: 21447725; http://dx.doi.org/10.1158/1078-0432.CCR-10-1681
- Wu CY, Hu HY, Pu CY, Huang N, Shen HC, Li CP, et al. Pulmonary tuberculosis increases the risk of lung cancer: a population-based cohort study. Cancer 2011; 117:618 - 624; PMID: 20886634; http://dx.doi.org/10.1002/cncr.25616
- Handa O, Naito Y, Yoshikawa T. Redox biology and gastric carcinogenesis: the role of Helicobacter pylori. Redox Rep 2011; 16:1 - 7; PMID: 21605492; http://dx.doi.org/10.1179/174329211X12968219310756
- Qian N, Ueno T. Is dysfunction of caveolin-1 a link between systemic sclerosis and breast cancer, opening a window on both etiologies?. Arch Med Res 2010; 41:297 - 301; PMID: 20637375; http://dx.doi.org/10.1016/j.arcmed.2010.05.009
- Thorsteinsdottir S, Gudjonsson T, Nielsen OH, Vainer B, Seidelin JB. Pathogenesis and biomarkers of carcinogenesis in ulcerative colitis. Nat Rev Gastroenterol Hepatol 2011; 8:395 - 404
- Naik E, Dixit VM. Mitochondrial reactive oxygen species drive proinflammatory cytokine production. J Exp Med 2011; 208:417 - 420; PMID: 21357740; http://dx.doi.org/10.1084/jem.20110367
- Wang X, Allen TD, May RJ, Lightfoot S, Houchen CW, Huycke MM. Enterococcus faecalis induces aneuploidy and tetraploidy in colonic epithelial cells through a bystander effect. Cancer Res 2008; 68:9909 - 9917; PMID: 19047172; http://dx.doi.org/10.1158/0008-5472.CAN-08-1551
- Merliss RR. Talc-treated rice and Japanese stomach cancer. Science 1971; 173:1141 - 1142; PMID: 5098957; http://dx.doi.org/10.1126/science.173.4002.1141
- Martinez-Outschoorn UE, Pavlides S, Whitaker-Menezes D, Daumer KM, Milliman JN, Chiavarina B, et al. Tumor cells induce the cancer associated fibroblast phenotype via caveolin-1 degradation: Implications for breast cancer and DCIS therapy with autophagy inhibitors. Cell Cycle 2010; 9:2423 - 2433; PMID: 20562526; http://dx.doi.org/10.4161/cc.9.12.12048
- Martinez-Outschoorn UE, Prisco M, Ertel A, Tsirigos A, Lin Z, Pavlides S, et al. Ketones and lactate increase cancer cell “stemness,” driving recurrence, metastasis and poor clinical outcome in breast cancer: Achieving personalized medicine via Metabolo-Genomics. Cell Cycle 2011; 10:1271 - 1286; PMID: 21512313; http://dx.doi.org/10.4161/cc.10.8.15330
- Bonuccelli G, Tsirigos A, Whitaker-Menezes D, Pavlides S, Pestell RG, Chiavarina B, et al. Ketones and lactate “fuel” tumor growth and metastasis: Evidence that epithelial cancer cells use oxidative mitochondrial metabolism. Cell Cycle 2010; 9:3506 - 3514; PMID: 20818174; http://dx.doi.org/10.4161/cc.9.17.12731
- Pavlides S, Tsirigos A, Migneco G, Whitaker-Menezes D, Chiavarina B, Flomenberg N, et al. The autophagic tumor stroma model of cancer: Role of oxidative stress and ketone production in fueling tumor cell metabolism. Cell Cycle 2010; 9:3485 - 3505; PMID: 20861672; http://dx.doi.org/10.4161/cc.9.17.12721
- Pavlides S, Tsirigos A, Vera I, Flomenberg N, Frank PG, Casimiro MC, et al. Loss of stromal caveolin-1 leads to oxidative stress, mimics hypoxia and drives inflammation in the tumor microenvironment, conferring the “reverse Warburg effect”: A transcriptional informatics analysis with validation. Cell Cycle 2010; 9:2201 - 2219; PMID: 20519932; http://dx.doi.org/10.4161/cc.9.11.11848
- Pavlides S, Tsirigos A, Vera I, Flomenberg N, Frank PG, Casimiro MC, et al. Transcriptional evidence for the “Reverse Warburg Effect” in human breast cancer tumor stroma and metastasis: similarities with oxidative stress, inflammation, Alzheimer's disease and “Neuron-Glia Metabolic Coupling”. Aging (Albany NY) 2010; 2:185 - 199; PMID: 20442453
- Pavlides S, Whitaker-Menezes D, Castello-Cros R, Flomenberg N, Witkiewicz AK, Frank PG, et al. The reverse Warburg effect: aerobic glycolysis in cancer associated fibroblasts and the tumor stroma. Cell Cycle 2009; 8:3984 - 4001; PMID: 19923890; http://dx.doi.org/10.4161/cc.8.23.10238
- Migneco G, Whitaker-Menezes D, Chiavarina B, Castello-Cros R, Pavlides S, Pestell RG, et al. Glycolytic cancer associated fibroblasts promote breast cancer tumor growth, without a measurable increase in angiogenesis: Evidence for stromal-epithelial metabolic coupling. Cell Cycle 2010; 9:2412 - 2422; PMID: 20562527; http://dx.doi.org/10.4161/cc.9.12.11989
- Zhang GY, Yu Q, Cheng T, Liao T, Nie CL, Wang AY, et al. Role of caveolin-1 in the pathogenesis of tissue fibrosis by keloid-derived fibroblasts in vitro. Br J Dermatol 2011; 164:623 - 627; PMID: 21375514
- Trimmer C, Sotgia F, Whitaker-Menezes D, Balliet RM, Eaton G, Martinez-Outschoorn UE, et al. Caveolin-1 and mitochondrial SOD2 (MnSOD) function as tumor suppressors in the stromal micro-environment: a new genetically tractable model for human cancer associated fibroblasts. Cancer Biol Ther 2011; 11:383 - 394; PMID: 21150282; http://dx.doi.org/10.4161/cbt.11.4.14101
- Bonuccelli G, Whitaker-Menezes D, Castello-Cros R, Pavlides S, Pestell RG, Fatatis A, et al. The reverse Warburg effect: Glycolysis inhibitors prevent the tumor promoting effects of caveolin-1 deficient cancer associated fibroblasts. Cell Cycle 2010; 9:1960 - 1971; PMID: 20495363; http://dx.doi.org/10.4161/cc.9.10.11601
- Trimmer C, Sotgia F, Whitaker-Menezes D, Balliet R, Eaton G, Martinez-Outschoorn UE, et al. Caveolin-1 and mitochondrial SOD2 (MnSOD) function as tumor suppressors in the stromal micro-environment: A new genetically tractable model for human cancer associated fibroblasts. Cancer Biol Ther 2011; 11:383 - 394; PMID: 21150282; http://dx.doi.org/10.4161/cbt.11.4.14101
- Xie L, Pandey R, Xu B, Tsaprailis G, Chen QM. Genomic and proteomic profiling of oxidative stress response in human diploid fibroblasts. Biogerontology 2009; 10:125 - 151; PMID: 18654835; http://dx.doi.org/10.1007/s10522-008-9157-3
- Witkiewicz AK, Casimiro MC, Dasgupta A, Mercier I, Wang C, Bonuccelli G, et al. Towards a new “stromal-based” classification system for human breast cancer prognosis and therapy. Cell Cycle 2009; 8:1654 - 1658; PMID: 19448435; http://dx.doi.org/10.4161/cc.8.11.8544
- Witkiewicz AK, Dasgupta A, Nguyen KH, Liu C, Kovatich AJ, Schwartz GF, et al. Stromal caveolin-1 levels predict early DCIS progression to invasive breast cancer. Cancer Biol Ther 2009; 8:1071 - 1079; PMID: 19502809; http://dx.doi.org/10.4161/cbt.8.11.8874
- Witkiewicz AK, Dasgupta A, Sammons S, Er O, Potoczek MB, Guiles F, et al. Loss of stromal caveolin-1 expression predicts poor clinical outcome in triple negative and basal-like breast cancers. Cancer Biol Ther 2010; 10:135 - 143; PMID: 20431349; http://dx.doi.org/10.4161/cbt.10.2.11983
- Witkiewicz AK, Dasgupta A, Sotgia F, Mercier I, Pestell RG, Sabel M, et al. An absence of stromal caveolin-1 expression predicts early tumor recurrence and poor clinical outcome in human breast cancers. Am J Pathol 2009; 174:2023 - 2034; PMID: 19411448; http://dx.doi.org/10.2353/ajpath.2009.080873
- Sloan EK, Ciocca DR, Pouliot N, Natoli A, Restall C, Henderson MA, et al. Stromal cell expression of caveolin-1 predicts outcome in breast cancer. Am J Pathol 2009; 174:2035 - 2043; PMID: 19411449; http://dx.doi.org/10.2353/ajpath.2009.080924
- Koo JS, Park S, Kim SI, Lee S, Park BW. The impact of caveolin protein expression in tumor stroma on prognosis of breast cancer. Tumour Biol 2011; 32:787 - 799; PMID: 21584795; http://dx.doi.org/10.1007/s13277-011-0181-6
- Qian N, Ueno T, Kawaguchi-Sakita N, Kawashima M, Yoshida N, Mikami Y, et al. Prognostic significance of tumor/stromal caveolin-1 expression in breast cancer patients. Cancer Sci 2011; 102:1590 - 1596; PMID: 21585620; http://dx.doi.org/10.1111/j.13497006.2011.01985.x
- Ghajar CM, Meier R, Bissell MJ. Quis custodiet ipsos custodies: who watches the watchmen?. Am J Pathol 2009; 174:1996 - 1999; PMID: 19465642; http://dx.doi.org/10.2353/ajpath.2009.090363
- Di Vizio D, Morello M, Sotgia F, Pestell RG, Freeman MR, Lisanti MP. An absence of stromal caveolin-1 is associated with advanced prostate cancer, metastatic disease and epithelial Akt activation. Cell Cycle 2009; 8:2420 - 2424; PMID: 19556867; http://dx.doi.org/10.4161/cc.8.15.9116
- Martinez-Outschoorn UE, Whitaker-Menezes D, Pavlides S, Chiavarina B, Bonuccelli G, Casey T, et al. The Autophagic Tumor Stroma Model of Cancer or “Battery-Operated Tumor Growth”: A Simple Solution to the Autophagy Paradox. Cell Cycle 2010; 9:4297 - 4306; PMID: 21051947; http://dx.doi.org/10.4161/cc.9.21.13817
- Brooks GA. The lactate shuttle during exercise and recovery. Med Sci Sports Exerc 1986; 18:360 - 368; PMID: 3523107; http://dx.doi.org/10.1249/00005768198606000-00019
- Bittar PG, Charnay Y, Pellerin L, Bouras C, Magistretti PJ. Selective distribution of lactate dehydrogenase isoenzymes in neurons and astrocytes of human brain. J Cereb Blood Flow Metab 1996; 16:1079 - 1089; PMID: 8898679; http://dx.doi.org/10.1097/00004647-199611000-00001
- Laughton JD, Bittar P, Charnay Y, Pellerin L, Kovari E, Magistretti PJ, et al. Metabolic compartmentalization in the human cortex and hippocampus: evidence for a cell- and region-specific localization of lactate dehydrogenase 5 and pyruvate dehydrogenase. BMC Neurosci 2007; 8:35; PMID: 17521432; http://dx.doi.org/10.1186/1471-2202-8-35
- Magistretti PJ, Pellerin L. Cellular mechanisms of brain energy metabolism. Relevance to functional brain imaging and to neurodegenerative disorders. Ann NY Acad Sci 1996; 777:380 - 387; PMID: 8624117; http://dx.doi.org/10.1111/j.1749-6632.1996.tb34449.x
- Tsacopoulos M, Magistretti PJ. Metabolic coupling between glia and neurons. J Neurosci 1996; 16:877 - 885; PMID: 8558256
- Biggers JD, Whittingham DG, Donahue RP. The pattern of energy metabolism in the mouse oocyte and zygote. Proc Natl Acad Sci USA 1967; 58:560 - 567; PMID: 5233459; http://dx.doi.org/10.1073/pnas.58.2.560
- Brinster RL. Oxidation of pyruvate and glucose by oocytes of the mouse and rhesus monkey. J Reprod Fertil 1971; 24:187 - 191; PMID: 4994572; http://dx.doi.org/10.1530/jrf.0.0240187
- Colonna R, Cecconi S, Buccione R, Mangia F. Amino acid transport systems in growing mouse oocytes. Cell Biol Int Rep 1983; 7:1007 - 1015; PMID: 6667499; http://dx.doi.org/10.1016/0309-1651(83)90005-X
- Colonna R, Mangia F. Mechanisms of amino acid uptake in cumulus-enclosed mouse oocytes. Biol Reprod 1983; 28:797 - 803; PMID: 6860738; http://dx.doi.org/10.1095/biolreprod28.4.797
- Su YQ, Sugiura K, Eppig JJ. Mouse oocyte control of granulosa cell development and function: paracrine regulation of cumulus cell metabolism. Semin Reprod Med 2009; 27:32 - 42; PMID: 19197803; http://dx.doi.org/10.1055/s-0028-1108008
- Su YQ, Sugiura K, Wigglesworth K, O'Brien MJ, Affourtit JP, Pangas SA, et al. Oocyte regulation of metabolic cooperativity between mouse cumulus cells and oocytes: BMP15 and GDF9 control cholesterol biosynthesis in cumulus cells. Development 2008; 135:111 - 121; PMID: 18045843; http://dx.doi.org/10.1242/dev.009068
- Sugiura K, Pendola FL, Eppig JJ. Oocyte control of metabolic cooperativity between oocytes and companion granulosa cells: energy metabolism. Dev Biol 2005; 279:20 - 30; PMID: 15708555; http://dx.doi.org/10.1016/j.ydbio.2004.11.027
- Sugiura K, Su YQ, Diaz FJ, Pangas SA, Sharma S, Wigglesworth K, et al. Oocyte-derived BMP15 and FGFs cooperate to promote glycolysis in cumulus cells. Development 2007; 134:2593 - 2603; PMID: 17553902; http://dx.doi.org/10.1242/dev.006882
- Sugiura K, Su YQ, Li Q, Wigglesworth K, Matzuk MM, Eppig JJ. Fibroblast growth factors and epidermal growth factor cooperate with oocyte-derived members of the TGFbeta superfamily to regulate Spry2 mRNA levels in mouse cumulus cells. Biol Reprod 2009; 81:833 - 841; PMID: 19553596; http://dx.doi.org/10.1095/biolreprod.109.078485
- Anderson EJ, Neufer PD. Type II skeletal myofibers possess unique properties that potentiate mitochondrial H(2)O(2) generation. Am J Physiol Cell Physiol 2006; 290:844 - 851; PMID: 16251473; http://dx.doi.org/10.1152/ajpcell.00402.2005
- Martinez-Outschoorn UE, Lin Z, Ko YH, Goldberg A, Flomenberg N, Wang C, et al. Understanding the metabolic basis of drug resistance: Therapeutic induction of the Warburg effect kills cancer cells. Cell Cycle 2011; 10:2521 - 2528
- Khan N, Afaq F, Mukhtar H. Cancer chemoprevention through dietary antioxidants: progress and promise. Antioxid Redox Signal 2008; 10:475 - 510; PMID: 18154485; http://dx.doi.org/10.1089/ars.2007.1740
- López-Lázaro M. Dual role of hydrogen peroxide in cancer: possible relevance to cancer chemoprevention and therapy. Cancer Lett 2007; 252:1 - 8; PMID: 17150302; http://dx.doi.org/10.1016/j.canlet.2006.10.029
- Rui Q, Komori K, Tian Y, Liu H, Luo Y, Sakai Y. Electrochemical biosensor for the detection of H2O2 from living cancer cells based on ZnO nanosheets. Anal Chim Acta 2010; 670:57 - 62; PMID: 20685417; http://dx.doi.org/10.1016/j.aca.2010.04.065
- Stolarek RA, Potargowicz E, Seklewska E, Jakubik J, Lewandowski M, Jeziorski A, et al. Increased H2O2 level in exhaled breath condensate in primary breast cancer patients. J Cancer Res Clin Oncol 2010; 136:923 - 930; PMID: 19967414; http://dx.doi.org/10.1007/s00432-009-0734-x
- Chan HP, Tran V, Lewis C, Thomas PS. Elevated levels of oxidative stress markers in exhaled breath condensate. J Thorac Oncol 2009; 4:172 - 178; PMID: 19179892; http://dx.doi.org/10.1097/JTO.0b013e3181949eb9
- Chan HP, Lewis C, Thomas PS. Exhaled breath analysis: novel approach for early detection of lung cancer. Lung Cancer 2009; 63:164 - 168; PMID: 18599152; http://dx.doi.org/10.1016/j.lungcan.2008.05.020
- Psathakis K, Mermigkis D, Papatheodorou G, Loukides S, Panagou P, Polychronopoulos V, et al. Exhaled markers of oxidative stress in idiopathic pulmonary fibrosis. Eur J Clin Invest 2006; 36:362 - 367; PMID: 16634841; http://dx.doi.org/10.1111/j.1365-2362.2006.01636.x
- Wang XM, Zhang Y, Kim HP, Zhou Z, Feghali-Bostwick CA, Liu F, et al. Caveolin-1: a critical regulator of lung fibrosis in idiopathic pulmonary fibrosis. J Exp Med 2006; 203:2895 - 2906; PMID: 17178917; http://dx.doi.org/10.1084/jem.20061536
- Jasmin JF, Mercier I, Dupuis J, Tanowitz HB, Lisanti MP. Short-term administration of a cell-permeable caveolin-1 peptide prevents the development of monocrotaline-induced pulmonary hypertension and right ventricular hypertrophy. Circulation 2006; 114:912 - 920; PMID: 16940204; http://dx.doi.org/10.1161/CIRCULATIONAHA.106.634709
- Jasmin JF, Mercier I, Hnasko R, Cheung MW, Tanowitz HB, Dupuis J, et al. Lung remodeling and pulmonary hypertension after myocardial infarction: pathogenic role of reduced caveolin expression. Cardiovasc Res 2004; 63:747 - 755; PMID: 15306231; http://dx.doi.org/10.1016/j.cardiores.2004.05.018
- Van de Bittner GC, Dubikovskaya EA, Bertozzi CR, Chang CJ. In vivo imaging of hydrogen peroxide production in a murine tumor model with a chemoselective bioluminescent reporter. Proc Natl Acad Sci USA 2010; 107:21316 - 21321; PMID: 21115844; http://dx.doi.org/10.1073/pnas.1012864107
- Kubota K, Nakamoto Y, Tamaki N, Kanegae K, Fukuda H, Kaneda T, et al. FDG-PET for the diagnosis of fever of unknown origin: a Japanese multicenter study. Ann Nucl Med 2011; 25:355 - 364
- Vos FJ, Bleeker-Rovers CP, Sturm PD, Krabbe PF, van Dijk AP, Cuijpers ML, et al. 18F-FDG PET/CT for detection of metastatic infection in gram-positive bacteremia. J Nucl Med 2010; 51:1234 - 1240; PMID: 20660384; http://dx.doi.org/10.2967/jnumed.109.072371
- Glaudemans AW, Signore A. FDG-PET/CT in infections: the imaging method of choice?. Eur J Nucl Med Mol Imaging 2010; 37:1986 - 1991; PMID: 20700737; http://dx.doi.org/10.1007/s00259-010-1587-x
- Tsuji AB, Morita M, Li XK, Sogawa C, Sudo H, Sugyo A, et al. 18F-FDG PET for semiquantitative evaluation of acute allograft rejection and immunosuppressive therapy efficacy in rat models of liver transplantation. J Nucl Med 2009; 50:827 - 830; PMID: 19372488; http://dx.doi.org/10.2967/jnumed.108.058925
- Reuter S, Schnockel U, Edemir B, Schroter R, Kentrup D, Pavenstadt H, et al. Potential of noninvasive serial assessment of acute renal allograft rejection by 18F-FDG PET to monitor treatment efficiency. J Nucl Med 2010; 51:1644 - 1652; PMID: 20847180; http://dx.doi.org/10.2967/jnumed.110.078550
- Reuter S, Schnockel U, Schroter R, Schober O, Pavenstadt H, Schafers M, et al. Non-invasive imaging of acute renal allograft rejection in rats using small animal F-FDG-PET. PLoS ONE 2009; 4:5296; PMID: 19390685; http://dx.doi.org/10.1371/journal.pone.0005296
- Schnöckel U, Reuter S, Stegger L, Schlatter E, Schafers KP, Hermann S, et al. Dynamic 18F-fluoride small animal PET to noninvasively assess renal function in rats. Eur J Nucl Med Mol Imaging 2008; 35:2267 - 2274; PMID: 18622612; http://dx.doi.org/10.1007/s00259-008-0878-y
- Kensese SM, Smith LL. Hydrogen peroxide mutagenicity towards Salmonella typhimurium. Teratog Carcinog Mutagen 1989; 9:211 - 218; PMID: 2572065; http://dx.doi.org/10.1002/tcm.1770090404
- Naik S, Tredwin CJ, Scully C. Hydrogen peroxide tooth-whitening (bleaching): review of safety in relation to possible carcinogenesis. Oral Oncol 2006; 42:668 - 674; PMID: 16488181; http://dx.doi.org/10.1016/j.oraloncology.2005.10.020
- Nyaga SG, Jaruga P, Lohani A, Dizdaroglu M, Evans MK. Accumulation of oxidatively induced DNA damage in human breast cancer cell lines following treatment with hydrogen peroxide. Cell Cycle 2007; 6:1471 - 1477; PMID: 17568196; http://dx.doi.org/10.4161/cc.6.12.4301
- Ohshima H, Tatemichi M, Sawa T. Chemical basis of inflammation-induced carcinogenesis. Arch Biochem Biophys 2003; 417:3 - 11; PMID: 12921773; http://dx.doi.org/10.1016/S0003-9861(03)00283-2
- Roh M, van der Meer R, Abdulkadir SA. Tumorigenic polyploid cells contain elevated ROS and are selectively targeted by antioxidant treatment. J Cell Physiol 2011; In press
- Graham KA, Kulawiec M, Owens KM, Li X, Desouki MM, Chandra D, et al. NADPH oxidase 4 is an oncoprotein localized to mitochondria. Cancer Biol Ther 2010; 10:223 - 231; PMID: 20523116; http://dx.doi.org/10.4161/cbt.10.3.12207
- Du C, Gao Z, Venkatesha VA, Kalen AL, Chaudhuri L, Spitz DR, et al. Mitochondrial ROS and radiation induced transformation in mouse embryonic fibroblasts. Cancer Biol Ther 2009; 8:1962 - 1971; PMID: 19738419; http://dx.doi.org/10.4161/cbt.8.20.9648
- Uziel O, Reshef H, Ravid A, Fabian I, Halperin D, Ram R, et al. Oxidative stress causes telomere damage in Fanconi anaemia cells—a possible predisposition for malignant transformation. Br J Haematol 2008; 142:82 - 93; PMID: 18477050; http://dx.doi.org/10.1111/j.13652141.2008.07137.x
- Pennisi E. Superoxides relay Ras protein's oncogenic message. Science 1997; 275:1567 - 1568; PMID: 9072821; http://dx.doi.org/10.1126/science.275.5306.1567
- Pervaiz S, Clement MV. Superoxide anion: oncogenic reactive oxygen species?. Int J Biochem Cell Biol 2007; 39:1297 - 1304; PMID: 17531522; http://dx.doi.org/10.1016/j.biocel.2007.04.007
- Liu TY, Chen CL, Chi CW. Oxidative damage to DNA induced by areca nut extract. Mutat Res 1996; 367:25 - 31; PMID: 8596543; http://dx.doi.org/10.1016/S0165-1218(96)90018-X
- Nair UJ, Obe G, Friesen M, Goldberg MT, Bartsch H. Role of lime in the generation of reactive oxygen species from betel-quid ingredients. Environ Health Perspect 1992; 98:203 - 205; PMID: 1486850; http://dx.doi.org/10.1289/ehp.9298203
- Cerutti PA. Prooxidant states and tumor promotion. Science 1985; 227:375 - 381; PMID: 2981433; http://dx.doi.org/10.1126/science.2981433
- Jones GM, Sanford KK, Parshad R, Gantt R, Price FM, Tarone RE. Influence of added catalase on chromosome stability and neoplastic transformation of mouse cells in culture. Br J Cancer 1985; 52:583 - 590; PMID: 2415146; http://dx.doi.org/10.1038/bjc.1985.230
- Lisanti MP, Martinez-Outschoorn UE, Pavlides S, Whitaker-Menezes D, Pestell RG, Howell A, et al. Accelerated aging in the tumor microenvironment: Connecting aging, inflammation and cancer metabolism with personalized medicine. Cell Cycle 2011; 10:2059 - 2063; PMID: 21654190