Abstract
It is well established that protein kinase C (PKC) isozymes play distinctive roles in mitogenic and survival signaling as well as in cancer progression. PKCe, the product of the PRKCE gene, is up-regulated in various types of cancers including prostate, lung and breast cancer. To address a potential role for PKCs in prostate cancer progression we generated three mouse transgenic lines expressing PKCa, PKCd, or PKCe in the prostate epithelium under the control of the rat probasin (PB) promoter. Whereas PB-PKCa and PB-PKCd mice did not show any evident phenotype, PB-PKCe mice developed prostate hyperplasia as well as prostate intraepithelial neoplasia (PIN) that displayed enhanced phospho-Akt, phospho-S6, and phospho-Stat3 levels, as well as enhanced resistance to apoptotic stimuli. PKCe overexpression was insufficient to drive neoplastic changes in the mouse prostate. Notably, overexpression of PKCe by adenoviral means in normal immortalized RWPE-1 prostate cells confers a growth advantage and hyperactivation of Erk and Akt. Our results argue for a causal link between PKCe overexpression and prostate cancer development.
Introduction
The protein kinase C (PKC) isozymes are a family of serine-threonine kinases widely implicated in cancer progression. Members of the classical PKCs (cPKCs α, β and γ) and novel PKCs (nPKCs δ, ε, η and θ) are main cellular targets for the phorbol ester tumor promoters and the second messenger diacylglycerol (DAG) generated in response to the activation of growth factor receptors.Citation1,Citation2 Studies in cancer cell models have established essential roles for DAG/phorbol ester-responsive PKCs either as positive or negative regulators of proliferation and survival. While there is remarkable cell specificity in the responses triggered by activation of individual PKCs, it is well established that PKCε overexpression confers a growth advantage in a number of cellular models, and eventually transform cells to a malignant state, as described for fibroblasts and colonic epithelial cells. It is remarkable that, on the other hand, the related nPKCδ mostly conveys anti-proliferative and apoptotic signals, and suppressing PKCδ expression or activity promotes a transformed phenotype.Citation3–Citation7 cPKCs are able to exert either pro- or anti-mitogenic/tumorigenic effects.Citation1,Citation8 The diversity in PKC isozyme function has been associated with their differential ability to relocalize to distinct intracellular compartments and access specific substrates, which ultimately leads to signaling specificity.
The altered balance in the expression of PKC isozymes is a distinguished feature of cancer. One of the most notable alterations in epithelial cancers is the upregulation of PKCε, the product of the PKRCE gene. PKCε has emerged as a potential oncogene and tumor biomarker,Citation4,Citation5,Citation9–Citation11 however, little is known regarding a potential causality between its upregulation and cancer development. PKCε overexpression is a prominent feature of human prostate cancer, as determined by several studies using clinical specimens.Citation12–Citation14 Although the implications of PKCε upregulation in prostate cancer in vivo are yet to be determined, studies in cellular models have demonstrated central roles for this nPKC in prostate cancer cell survival. Our laboratory recently found that PKCε protects LNCaP prostate cancer cells against phorbol ester- and TNFα-induced apoptosis through a mechanism that involves Bad phosphorylation.Citation15 PKCε also interacts with Bax in prostate cancer cells to neutralize apoptotic signals propagated through the mitochondrial death-signaling pathway.Citation16 There is evidence that PKCε links integrin to the Akt survival pathway in recurrent prostate cancer cells.Citation17 PKCε has been also implicated in the transition to androgen independence and was found to be consistently elevated in recurrent androgen-independent tumors.Citation17,Citation18 More recently, studies identified a microRNA that exerts tumor suppressive functions in human prostate through PKCε downregulation,Citation19 arguing for a potential role for this PKC in prostate cancer development. Unlike PKCε, activation of PKCα and PKCδ positively modulates apoptotic pathways and their activation leads to programmed cell death in LNCaP cells.Citation7,Citation8,Citation20–Citation22 It is therefore likely that alterations in the balance of expression of PKC isozymes in prostate cells may lead to remarkably distinct phenotypic changes.
The aim of this study was to determine the phenotypic consequences of overexpressing PKCε in the normal prostate in vivo. To tackle this issue we generated a transgenic mouse model in which PKCε was expressed specifically in the mouse prostate under the control of a probasin (PB) promoter. Our studies revealed that targeted PKCε overexpression, but not PKCα and PKCδ overexpression, leads to the development of pre-neoplastic lesions in the prostate, therefore suggesting a potential link between PKCε and prostate cancer development.
Results
Generation of PB-PKCα, PKCδ and PKCε mice.
To study the role of PKCε overexpression in prostate tumor development, we targeted this PKC to mouse epithelial prostate cells using the androgen-responsive ARR2PB promoter.Citation23 As the roles of other DAG/phorbol ester-responsive PKCs in the prostate in vivo have not yet been determined (as well as for control of specificity), we also generated two additional prostate-specific transgenic models for PKCα and PKCδ. The cDNAs for PKCα, PKCδ or PKCε were ligated into a PB promoter vector that we previously adapted to express proteins fused to an N-terminal HA-tag (). After the DNA pronuclear microinjections, founder mice were identified by PCR genotyping using primers that amplify the rabbit beta-globin sequence. Three positive male founders were identified for PB-PKCα, two for PB-PKCδ and two for PB-PKCε. A founder was selected for each one and a line established. Protein extracts from prostates obtained from 4-month old transgenic and wild-type mice were analyzed by western blot using an anti-HA epitope antibody. HA immunoreactivity was detected in prostates from PB-PKCα, PB-PKCδ and PB-PKCε transgenic mice but not in prostates from wild-type mice. A representative experiment is shown in . Using specific anti-PKC antibodies we could observe significant overexpression of each corresponding PKC transgene, which normally ranged from 3- to 5-fold over the endogenous protein (). Using a real-time PCR specific for the rabbit beta-globin sequence present in all transgenic constructs, we developed one homozygous line (Tg/Tg) for each PKC isoform and confirmed the expression by immunohistochemistry (IHC) (). Consistent with previous studies using this PB promoter,Citation24 transgene expression for the three PKC isozymes was detected specifically in the epithelial compartment of the ventral prostate (VP), with lower expression in the dorsal prostate (DP) (data not shown).
Differential effects of PKC isozymes in the mouse prostate: only PKCε overexpression leads to a preneoplastic phenotype.
Homozygous male animals for PB-PKCα, PB-PKCδ and PB-PKCε mice were sacrificed at 12 months. Age-matched wild-type FVB mice were used as controls. All PKC transgenic mice appeared normal at birth and did not show any signs of abnormalities to adulthood. Prostates were isolated, sectioned and stained with hematoxylin and eosin (H&E) for morphologic assessment. Histological analyses revealed significant epithelial hyperplasia both in the ventral and dorsal lobes of PB-PKCε mice. In addition, a high proportion of mice developed dysplastic changes characteristic of PINs both in VP and DP. On the other hand, PB-PKCα and PB-PKCδ mice did not present any noticeable phenotypic change in the prostate. Only one mouse in each PB-PKCα and PB-PKCδ group showed hyperplasia ( and ). Additional representative low- and high-magnifications photomicrographs of H&E-stained lesions in the VP and DP are shown in . PIN lesions in VP and DP glands exhibited tufting to micropapillary pattern, and some VP glands also presented a cribriform pattern. Cells with karyomegaly and cytomegaly, nuclear atypia with apical localization and chromatin condensation were observed. The presence of one or more prominent nucleoli was also observed. Lesions were seen merely in the prostate, and no other lesions were observed in the remaining of the genito-urinary track, with the exception of mild hyperplasia in few anterior prostate lobes (data not shown). Analysis of PB-PKCε mice at different ages showed that both hyperplasia and PINs were evident at 8 months. Notably, no evidence of invasive or in situ carcinomas were seen up to 16 month of age (), although PINs in 12 months of age and older present a high degree of dysplastic cells (data not shown). BrdU incorporation index is generally very low in the prostatic epithelium, however a slight increase could be observed in VPs from 12 month old PB-PKCε mice relative to control mice (wild-type: 0.26 ± 0.10 %, PB-PKCε: 0.43 ± 0.07%, see Sup. Fig. 1). Taken together, these results suggest that overexpression of PKCε in mouse prostatic epithelial cells for several months causes preneoplastic lesions. Moreover, there is a remarkable selectivity for PKC isozymes to confer such phenotypic changes.
PIN lesions from PB-PKCε mice display hyperactivation of the Akt pathway.
The appearance of preneoplastic lesions in prostates of PB-PKCε mice suggests major dysregulations in survival signals. Studies in different cellular models identified the Akt pathway as a downstream effector of PKCε.Citation5 Hyperactivation of Akt is an established signature of prostate cancer, as revealed in mouse models of prostate cancer models as well as in clinical specimens. The activation status of Akt in hyperplasia and PIN lesions from 12 month old PB-PKCε mice was determined by IHC using an anti phospho-Ser473 (active state) Akt antibody. While total Akt staining was similar among all samples (data not shown), phospho-Akt staining was clearly elevated in hyperplasia and PINs from PB-PKCε mice. There was a significant degree of nuclear phospho-Akt staining, particularly in PIN lesions, both in VP and DP. Furthermore, PIN lesions show a significant hyperactivation of the Akt effector S6 relative to normal tissue, as determined with anti-phospho-S6 antibody ().
A physical and functional association between PKCε and Stat3 has been recently reported in human cancer. PKCε modulates the transcriptional activity of Stat3 in prostate cancer cells.Citation9,Citation14 Remarkably, when we determined the expression levels of Stat3 and its phosphorylation status in prostates from 12 month old PB-PKCε mice we observed that they were both markedly elevated compared to prostates from control mice. Total Stat3 staining in prostates from PB-PKCε mice was detected in the cytoplasm of the hyperplastic lesion in both ventral and dorsal lobes and elevated in cytoplasmic and also nuclear compartments in the PIN lesions of VP glands, whereas its expression was slightly lower in the PIN lesions of DP glands. Phosphorylated (active) Stat3-Tyr705 staining shows a clear nuclear expression and light cytoplasmic staining in hyperplasias and PINs of both VP and DP glands. Androgen receptor (AR) expression was strongly expressed in all stages in VP and DP, from normal glands to PIN lesions and no obvious changes could be detected throughout all the stages ().
PKCε overexpression confer resistance to apoptosis induced by androgen ablation.
To assess a potential role for PKCε in prostate cell survival in vivo, we investigated if PKCε overexpression confers resistance to apoptosis induced by castration. It is well established that androgen ablation leads to prostate involution characterized by an extensive apoptotic response. A general drawback of the PB-driven models is that transgene expression is reduced upon androgen ablation. Despite this limitation, it has been found that transgenes driven by the ARR2PB promoter remain expressed, although at lower levels, several days after castration (data not shown). Eight-week old PB-PKCε mice and control FVB littermates were castrated as described in Experimental Procedures, and the prostates removed after 7 or 14 days and subject to active caspase-3 staining. Notably, the apoptotic index was significantly lower in prostates from PB-PKCε mice (). These data suggest that PKCε has pro-survival activity when expressed in the mouse prostate.
PKCε confers a growth advantage to prostate epithelial cells in culture.
Next, we investigated the role of PKCε overexpression in RWPE-1 cells, a model of normal immortalized prostate epithelial cells that has been widely used to assess pro-mitogenic and transforming actions of oncogenes.Citation25–Citation27 RWPE-1 cells express very low levels of PKCε relative to prostate cancer cell lines (), which is in concordance with data in human specimens.Citation12–Citation14 To overexpress PKCε we used an adenoviral approach. RWPE-1 growing either in complete medium or in medium deficient in supplements (EGF/bovine pituitary extract) were infected with increasing MOIs (0.1–10 pfu/cell) of an AdV for PKCε (PKCε AdV), and cell number determined for 1, 2 or 3 days after infection. As shown in , infection with the PKCε AdV leads to an increase in cell number, and this effect was proportional to the MOIs. The effect was observed both in RWPE-1 cells growing in complete or incomplete medium. On the other hand, a control LacZ AdV (MOI = 10 pfu/cell) had no effect on RWPE-1 cell number. PKCε expression levels are shown in . Note that the elevation in cell number was observed at expression levels even lower than those observed in prostate cancer cells.
Finally, we examined if PKCε overexpression in RWPE-1 cells could lead to changes in the activation status of Erk and Akt. Cells growing in complete medium were infected with either PKCε AdV or LacZ AdV as described above, and phospho-Erk and phospho-Akt levels were assessed by western blot. Notably, PKCε overexpression led to a marked activation of Erk and Akt in RWPE-1 cells. The effect was proportional to the expression levels of PKCε achieved by increasing MOIs for the PKCε AdV, and was noticeable even at MOIs which confer only a slight overexpression of PKCε (). Therefore, upregulation of PKCε in prostate epithelial cells is sufficient to elicit changes in markers of mitogenesis and survival.
Discussion
PKC isozymes regulate multiple cellular processes including apoptosis, proliferation, chemoresistance, migration and invasion, and have been widely studied in the context of tumorigenesis, particularly as intracellular receptors for the phorbol ester tumor promoters. Several years of extensive research helped delineating the specific functions of individual PKCs in different cellular contexts. Members of the nPKC family play paradoxical biological roles: PKCδ signals in most cases for apoptosis or inhibition of mitogenesis whereas PKCε promotes growth advantage or can even confer in some cell lines a malignant phenotype upon overexpression.Citation2–Citation9 This divergence in PKC function is also well exemplified in mouse models of skin cancer. Targeted overxpression of PKCδ in the epidermis inhibits tumor promotion, whereas PKCε overexpression promotes the development of skin metastatic squamous cell carcinomas. On the other hand PKCα overexpression in skin does not affect tumor promotion.Citation28–Citation30 In prostate cancer models in culture, both PKCα and PKCδ mediate apoptotic responses.Citation7,Citation8,Citation20–Citation22 While the role of PKCε in prostate cancer cells was controversial, mounting evidence implicates PKCε in prostate tumorigenesis through its stimulatory effects on proliferation, survival and invasiveness.Citation14–Citation18 The recent identification of a microRNA that exerts tumor suppressive functions in human prostate through PKCε downregulationCitation19 reinforces this concept.
PKCε overexpression is a hallmark of several cancers, most notably prostate cancer. Early studies by Conford et al.Citation12 showed the upregulation of PKCε in early prostate neoplasia, a finding later confirmed by others.Citation13,Citation14 The question that remained to be addressed is whether PKCε upregulation was capable of conferring a preneoplastic or neoplastic phenotype in the prostate in vivo. The present study clearly tackled this issue and established that targeted overexpression of PKCε in the mouse prostate leads to the development of a hyperplastic state. Substantial PIN lesions, but not carcinomas, could be observed in prostates from PB-PKCε mice. Consistent with the inability of PKCα and PKCδ to activate proliferative and survival signals in prostate cells, no phenotypic changes could be observed in prostates from PB-PKCα and PB-PKCδ mice. Thus, PKCε overexpression is sufficient to initiate the development of early stage preneoplastic lesions but is incapable to lead to prostate cancer. Unlike the skin PKCε transgenic mice, PB-PKCε mice do not show carcinomas in the prostate. It is important to highlight that our prostate mouse model presents moderate overexpression, unlike the skin transgenic mice in which PKCε has been massively overexpressed.Citation29 It is conceivable that PKCε overexpression cooperates with other genetic alterations to promote prostate cancer. Interestingly, preliminary studies from our laboratory revealed that PKCε synergizes with Pten deficiency for the development of prostate cancer. Indeed, PB-PKCε/Pten+/− compound mice show a high incidence of invasive adenocarcinoma of the prostate (unpublished observations). Cooperative interactions with Pten deficiency have been previously described in prostate mouse models, such as with fibroblast growth factor 8b (FGF8b) overexpression or loss of the homeobox gene Nkx3.1, as well as in other cancer types, such as with Wnt-1 in breast cancer.Citation31–Citation33 Coincidentally, PKCε is an effector of mitogenic and tumorigenic pathways, including the Wnt and FGF receptor pathways.Citation34–Citation36
The present results also uncovered a role for PKCε in prostate cancer survival in vivo. PINs from PB-PKCε mice display hyperactivation of the Akt pathway. Moreover, we found that PKCε overexpression protects against apoptosis induced by androgen deprivation. These results support the conclusions from our recent studies in LNCaP cells showing that PKCε RNAi depletion enhances the apoptotic activity of phorbol esters and TNFα.Citation15 Together with reports in other cell types, we conclude that PKCε has a broader survival function in response to physiological and pharmacological stimuli. Indeed, PKCε protects cells against the apoptotic effect of ceramide, TRAIL and chemotherapeutic drugs such as cisplatin. Increased levels of PKCε have been associated with chemoresistance in non-small cell lung cancer (NSCLC) and ovarian cancer cells, and ectopic expression of PKCε in small cell lung cancer (SCLC) cells, which normally present low PKCε levels, confers resistance to etoposide and doxorubicin. In cellular models PKCε enhances Akt phosphorylation and activity in response to diverse stimuli, including growth factors, insulin and stress (reviewed in ref. Citation5). It has been shown that cardiac-specific overexpression of PKCε in mice leads to elevations in phospho-Ser473-Akt levels and protects against ischemia-reperfusion injury.Citation37 The mechanisms by which PKCε activates Akt in prostate cancer cells remain to be elucidated. Studies in other cellular models suggest that PKCε and Akt form a complex, and their association results in high phospho-Ser473 Akt levels. PKCε can also activate Akt by enhancing its interaction with DNA-PK.Citation5,Citation38 Another interesting observation in our study was the elevation in activated Stat3 levels in lesions from PB-PKCε mice. Stat3 has been widely implicated in apoptosis resistance of cancer cells.Citation39,Citation40 Activation of Stat3 through phosphorylation is associated with reduced patient survival and the development of hormone-refractory prostate cancer.Citation41 Moreover, there is a positive correlation between PKCε, Stat3 levels and prostate cancer progression in humans.Citation14 It remains to be determined if a positive correlation also exists between PKCε overexpression and activated Akt/Akt effectors in human prostate tumors.
We found that overexpression of PKCε in normal immortalized RWPE-1 cells leads to a proliferative response and augmented phospho-Erk levels. In support of our studies, it has been reported that the acquisition of androgen independence of LNCaP cells by PKCε overexpression is linked to the constitutive activation of Erk, cyclin D1 upregulation and Rb hyperphosphorylation.Citation18 Activation of Erk in prostate cancer cells by PKCε overexpression may conceivably proceed through the activation of Raf, as described in other cell types.Citation4,Citation5 Interestingly, oncogenic Ha-ras leads to the transcriptional activation of cyclin D1 via an Erk-PKCε-dependent manner.Citation42 As PKCε overexpression promotes the secretion of mitogenic factors,Citation43 an attractive possibility is that the activated Erk (and Akt) status may occur through an autocrine pathway.
PKCε may represent an appealing target for the treatment of prostate cancer or other cancers. PKC antagonists designed as anti-cancer drugs had only partial success possibly due to their inability to discriminate between PKC isozymes triggering disparate effects. Notably, PKCε-specific modulators have been generated for other purposes, such as inflammation, ischemia and cardiovascular diseases.Citation44–Citation46 We have recently observed that the tumorigenic activity of non-small lung cancer cells (which express very high PKCε levels) in nude mice can be impaired by continuous delivery of the selective PKCε antagonist εV1-2 (unpublished observations). In summary, our studies warrant the development of highly specific inhibitors against PKCε. Such agents could be valuable in combination therapies with inhibitors of the PI3K/Akt pathway or other survival and mitogenic pathways, and may help overcome the resistance of prostate cancer cells to chemotherapeutic agents.
Materials and Methods
Animal care.
FVB/N inbred mice (used in maintenance of transgenic lines) were acquired from Charles River Laboratories Inc., (strain code 207). Mice were housed in individually ventilated cages on autoclaved hardwood bedding in an AAALAC accredited facility at the MD Anderson Cancer Center, Science-Park-Research Division. Controlled room conditions were as follows: temperature 20–22°C, humidity 60–70% and light 14/10 h = light/dark. Commercial rodent pelleted food and autoclaved R.O. water were available ad libitum. All procedures were in compliance with the Public Health Service Guide for the Care and Use of Laboratory Animals.
Generation of PKC overexpressing transgenic mice.
To achieve prostate-specific expression of PKCα, PKCδ or PKCε, their full-length cDNAs were placed under the control of the ARR2PB promoter, which targets prostate-specific transgene expression.Citation23,Citation47 The PB vector was adapted with a cassette that includes an upstream HA tag and XhoI-MluI sites for unidirectional ligation of PKC inserts. In vitro studies have shown that this tag does not affect the basal activity or activation of PKCs (data not shown). Transgenic mice were developed by means of pronuclear microinjection of FVB/N fertilized eggs in the Transgenic Core Facility of the Department of Carcinogenesis, M.D. Anderson Cancer Center, Smithville, Texas. Expression analysis of the transgene was performed by western blot, using an anti-HA antibody (HA.11, catalog # MMS-101P) and the following specific anti-PKC antibodies: anti-PKCα (Millipore Corporation, catalog # 05-154), anti-PKCδ (Cell Signaling Technology Inc., catalog # 2058) and anti-PKCε (Santa Cruz Biotechnology Inc., catalog # sc-214). Based on expression analysis, we selected one founder per isoform to develop a transgenic line. The genotype of the animals was carried out by PCR for the detection of the reporter rabbit beta globin sequence present in the transgenic construct, as previously described in reference Citation48. The standard nomenclature for these new lines is as follows: FVB/N-Tg(Pbsn-Prkca), FVB/N-Tg(Pbsn-Prkcd) and FVB/N-Tg(Pbsn-Prkce) (referred to as PB-PKCα, PB-PKCδ and PB-PKCε, respectively). In order to develop homozygous lines (Tg/Tg), we crossed hemizygous (Tg/0) mice and genotyped the progeny using beta globin-specific quantitative real-time PCR.Citation48
Analysis of phenotype.
For each transgenic line (PB-PKCα, PB-PKCδ and PB-PKCε), we studied homozygous Tg/Tg males on a pure FVB/N background and age-matched wild-type FVB/N males at different times. Complete necropsy, macroscopic examination, tissue collection and processing were carried out as described in reference Citation49. Mice were sacrificed with CO2 and their entire genitourinary tract removed. Prostates were submitted en bloc and processed in standard formalin-fixed, paraffin-embedded sections. To avoid compromising subsequent immunohistochemical analyses, tissues were switched overnight to 70% ethanol. Formalin-fixed paraffin-embedded tissue sections were stained with H&E. Histological examination was performed by two independent pathologists and was based on the “Basic Protocols in Characterization of Prostate Lesions in GEM” from the Consensus Report from the Bar Harbor Meeting of the Mouse Models of Human Cancer Consortium Prostate Pathology Committee.Citation50
For cell proliferation, mice were injected intraperitoneally with the thymidine analog 5′-bromo-2′-deoxyuridine (BrdU) (60 µg/g; Sigma, catalog # B5002) 30 min before sacrifice. BrdU incorporation was detected by a standard 3-step immunoperoxidase detection using mouse anti-BrdU monoclonal antibody (Becton-Dickinson Immunocytometry System, catalog # 347580), biotin F(ab') rabbit anti-mouse IgG (Accurate Chemical, catalog # JZM066045) and streptavidin peroxidase (BioGenex, catalog # HK-330-9K). Diaminobenzidine (BioGenex, HK-153-5K) was used as a chromogen for visualization.
For IHC analysis on paraffin-embedded prostate tissue sections we used the following primary antibodies: phospho-Akt (Ser473) (1:50), phospho-S6 (S235/236) (1:50), Stat3 (1:50) and phospho-Stat3-Tyr705 (1:50; Cell Signaling Technologies, catalog # 9145) and anti-androgen receptor (1:200, Abcam, catalog # ab2742). Antibodies were detected with biotinylated secondary antibodies, followed by peroxidase-conjugated avidin/biotin (Vectastain ABC Kit, Vector Laboratories, Burlingame, CA) and DAB substrate (Dako, Carpinteria, CA).
Castration experiments.
Bilateral orchiectomy was performed in 8-week old FVB/N-PB-PKCε males and FVB/N wild-type controls (10 mice/group). Combined ketamine and xylazine was used as anesthetic (1.5 ml of 100 mg/ml xylazine and 10 ml of 100 mg/ml ketamine; diluted 1:4 with saline, at 0.1 ml/20 g of body weight). A small surgical incision was made in the center of the scrotum and each testicle was exposed through the surgical orifice. The ductus deferens and main arteries and veins were isolated and ligated. Subsequently, the duct and blood vessels were severed allowing the testicles and epididymis to be removed. The incision was closed, sutured and swabbed with povidone-iodine solution and post-operative procedures were applied when necessary. Prostate lobes were collected at different times after castration and subject to IHC for active caspase-3 using an anti-activated caspase-3 antibody (R&D Systems, Minneapolis, MN).
Experiments with RWPE-1 cells.
Low passage (1.5 × 104) RWPE1 cells were seeded in 12-well plates in complete K-SFM medium. After 24 h, cells were infected with either PKCε adenovirus (AdV) or LacZ AdV at different multiplicities of infection (MOI) in K-SFM medium without supplements (EGF and bovine pituitary extract). Four hours later the AdV were removed by extensive washing and cells were given either complete K-SFM medium or supplement-free K-SFM medium. Cell number was determined at 24, 48 and 72 h.
For western blot experiments, 2 × 105 cells were seeded in 6-well plates and infected with AdVs as described above. Cell extracts were prepared at different times and equal amounts of protein were subjected to SDS-polyacrylamide gel electrophoresis, transferred to PVDF membranes and immunostained with the following antibodies: anti-phospho-ERK1/2 (Cell Signaling Technology Inc., catalog # 9101), anti-total ERK1/2 (Cell Signaling Technology Inc., catalog # 9102), anti-phospho-Akt (Ser473) (Cell Signaling Technology Inc., catalog # 9271) and anti-total Akt, (Cell Signaling Technology Inc., catalog # 4691), anti-PKCε (Santa Cruz Biotechnology, catalog # sc-214) or vinculin (Sigma, catalog # V9131). Bands were visualized by using the enhanced chemiluminescence (ECL) western blotting detection system.
Figures and Tables
Figure 1 Generation of PB-PKC transgenic mice. (A) Schematic representation of transgenic constructs. The cDNAs for PKCα, PKCδ or PKCε were ligated into a PB promoter vector adapted to express proteins fused to an N-terminal HA-tag. (B) Western blot showing the expression of PKC transgenes in mouse prostates. Protein extracts from prostates obtained from 4 month-old mice were analyzed by western blot using an anti-HA epitope antibody and specific anti-PKC isozyme antibodies. (C) Prostates from homozygous PB-PKCα, PB-PKCδ and PB-PKCε, as well as control (wild-type) mice at 4 months were obtained and subjected to IHC using an anti-HA antibody. Representative HA stainings in the VP are shown.
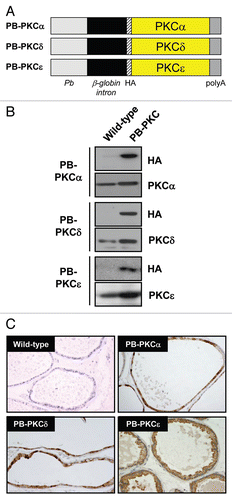
Figure 2 Phenotypes of PB-PKC transgenic mice: PKCε overexpression leads to a preneoplastic phenotype. Homozygous males for PB-PKCα, PB-PKCδ, PB-PKCε and non-transgenic FVB control mice at 12 months old were analyzed. Histological analyses revealed significant epithelial hyperplasia and PIN lesions in both VP and DP from PB-PKCε mice. Representative H&E and HA stainings are shown. All microphotographs are 20x magnification.
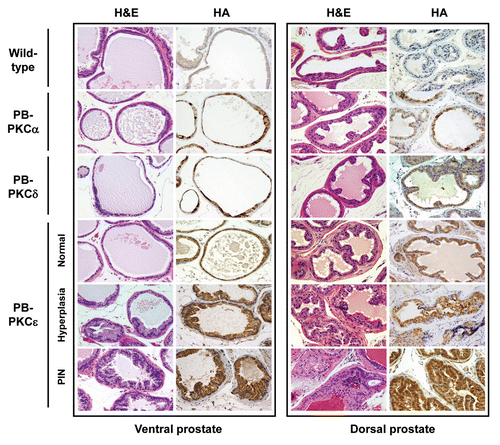
Figure 3 Representative lesions in PB-PKCε mice. Left panels. H&E stained sections of VP from PKCε mice. (A) (20x) VP showing a single stratum of luminal epithelial cells with normal lower flat and cylindrical shaped cells projecting into the gland. (B) (20x) VP showing luminal epithelial hyperplasia, few cells present nuclear atypia. (C and D) (20x) Representative examples from VP glands presenting PIN lesions with cribriform pattern (C) and a mixed of tufting with micropapillary pattern (D). Cells display from moderate to strong cellular atypia. Note also the nucleus adopting an apical localization. (E) (40x) This lesion displays a mild to moderate dysplastic luminal epithelium and the presence of nuclear atypia. Kariomegaly and kariocytomegaly are common findings. (F) (100x) Clusters of cells presenting severe dysplasia. Note the difference in the shape and size of the nucleus as well as the large number of nucleoli. Right panels. H&E stained sections of the DP from PKCε mice. (A) (20x) Normal glands showing cubical shaped cells with normal mucosal folding projected into the lumen. (B) (20x) DP showing luminal epithelial hyperplasia with increased cellular tufting but without cellular atypia. (C) (20x) A mixed hyperplasia with cellular atypia and PIN lesion with mild dysplasia. (D) (40x) Some of the PIN lesions display many small “intraluminal glands.” (E) (40x) Highly dysplastic PIN lesion with increased epithelial tufting and micropapillary pattern. (F) (100x) Higher magnification from a PIN showing dysplastic cells with enlarged nucleus presenting one or more large nucleoli.
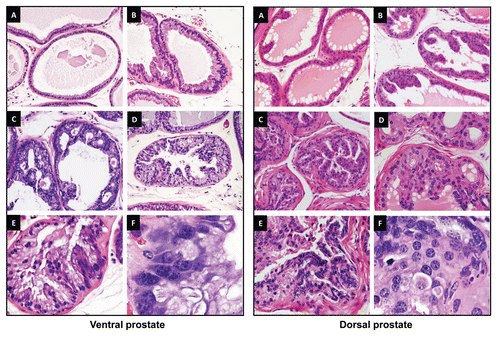
Figure 4 Immunohistochemical analysis of signaling markers in prostates from PB-PKCε mice. Paraffin-embedded prostate tissue sections from 12 month old wild-type or PB-PKCε mice were subject to IHC for the signaling markers indicated in the figure. Representative figures are shown. All microphotographs are 20x magnification.
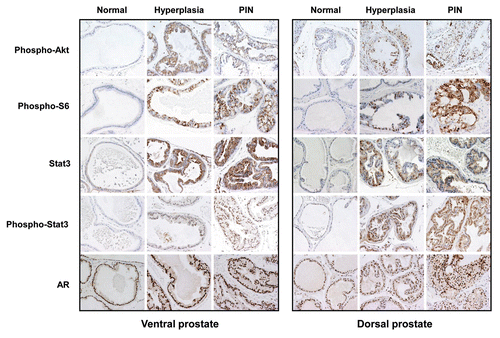
Figure 5 PKCε overexpression confers resistance to apoptosis after castration. Eight-week old mice were castrated as described in Experimental Procedures. Prostate lobes were collected 7 or 14 days later. Apoptosis was detected by IHC using an antibody specific for activated caspase-3. Data are expressed as mean ± SD. Similar results were observed in 2 independent experiments. WT, wild type; *p < 0.05, Student's t test.
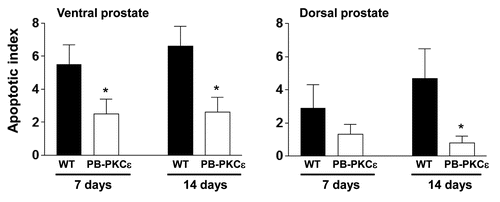
Figure 6 Overexpression of PKCε in RWPE-1 cells enhances proliferation and leads to Erk and Akt activation. (A) Comparison of the expression of PKCε between RWPE-1 cells and several prostate cancer cell lines, as determined by western blot. (B) RWPE-1 cells were infected with increasing multiplicities of infection (MOI) of PKCε AdV or LacZ AdV (control). Cell number was determined at different times after infection. Data from triplicate samples are expressed as mean ± SD (n = 3). (C) Cell extracts were prepared 72 h after infection with either PKCε AdV or LacZ AdV. Erk and Akt (total and phosphorylated) levels were determined by western blot. Similar results were observed in 3 experiments.
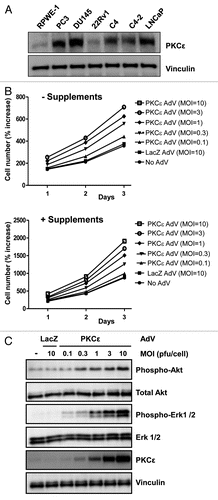
Table 1 Incidence of hyperplasia and PIN lesions in VP and DP of PB-PKC transgenic mice
Additional material
Download Zip (106.4 KB)Acknowledgements
This work was supported by grant RO1-CA89202 (NIH) to M.G.K. We wish to thank the Histology and Tissue Processing Facility Core of Science Park for the processing of samples. This study also made use of the Research Animal Support Facility-Smithville, including Genetic Services, supported by P30 CA16672-30 DHHS/NCI Cancer Center Support Grant (CCSG).
References
- Newton AC. Regulation of protein kinase C. Curr Opin Cell Biol 1997; 9:161 - 167; PMID: 9069266; http://dx.doi.org/10.1016/S0955-0674(97)80058-0
- Griner EM, Kazanietz MG. Protein kinase C and other diacylglycerol effectors in cancer. Nat Rev Cancer 2007; 7:281 - 294; PMID: 17384583; http://dx.doi.org/10.1038/nrc2110
- Mischak H, Goodnight JA, Kolch W, Martiny-Baron G, Schaechtle C, Kazanietz MG, et al. Overexpression of protein kinase C-delta and -epsilon in NIH 3T3 cells induces opposite effects on growth, morphology, anchorage dependence and tumorigenicity. J Biol Chem 1993; 268:6090 - 6096; PMID: 8454583
- Cacace AM, Ueffing M, Philipp A, Han EK, Kolch W, Weinstein IB. PKCepsilon functions as an oncogene by enhancing activation of the Raf kinase. Oncogene 1996; 13:2517 - 2526; PMID: 9000126
- Basu A, Sivaprasad U. Protein kinase Cepsilon makes the life and death decision. Cell Signal 2007; 19:1633 - 1642; PMID: 17537614; http://dx.doi.org/10.1016/j.cellsig.2007.04.008
- Lu Z, Hornia A, Jiang YW, Zang Q, Ohno S, Foster DA. Tumor promotion by depleting cells of protein kinase Cdelta. Mol Cell Biol 1997; 17:3418 - 3428; PMID: 9154841
- Tanaka Y, Gavrielides MV, Mitsuuchi Y, Fujii T, Kazanietz MG. Protein kinase C promotes apoptosis in LNCaP prostate cancer cells through activation of p38 MAPK and inhibition of the Akt survival pathway. J Biol Chem 2003; 278:33753 - 33762; PMID: 12824193; http://dx.doi.org/10.1074/jbc.M303313200
- Garcia-Bermejo ML, Leskow FC, Fujii T, Wang Q, Blumberg PM, Ohba M, et al. Diacylglycerol (DAG)-lactones, a new class of protein kinase C (PKC) agonists, induce apoptosis in LNCaP prostate cancer cells by selective activation of PKCalpha. J Biol Chem 2002; 277:645 - 655; PMID: 11584014; http://dx.doi.org/10.1074/jbc.M107639200
- Gorin MA, Pan Q. Protein kinase Cepsilon: an oncogene and emerging tumor biomarker. Mol Cancer 2009; 19:8 - 9; PMID: 19228372; http://dx.doi.org/10.1186/1476-4598-8-9
- Pan Q, Bao LW, Kleer CG, Sabel MS, Griffith KA, Teknos TN, et al. Protein kinase Cepsilon is a predictive biomarker of aggressive breast cancer and a validated target for RNA interference anticancer therapy. Cancer Research 2005; 65:8366 - 8371; PMID: 16166314; http://dx.doi.org/10.1158/0008-5472.CAN-05-0553
- Bae KM, Wang H, Jiang G, Chen MG, Lu L, Xiao L. Protein kinase Cepsilon is overexpressed in primary human non-small cell lung cancers and functionally required for proliferation of non-small cell lung cancer cells in a p21/Cip1-dependent manner. Cancer Res 2007; 67:6053 - 6063; PMID: 17616661; http://dx.doi.org/10.1158/0008-5472.CAN-06-4037
- Cornford P, Evans J, Dodson A, Parsons K, Woolfenden A, Neoptolemos J, et al. Protein kinase C isoenzyme patterns characteristically modulated in early prostate cancer. Am J Pathol 1999; 154:137 - 144; PMID: 9916928
- Koren R, Ben Meir D, Langzam L, Dekel Y, Konichezky M, Baniel J, et al. Expression of protein kinase C isoenzymes in benign hyperplasia and carcinoma of prostate. Oncol Rep 2004; 11:321 - 326; PMID: 14719062
- Aziz MH, Manoharan HT, Church DR, Dreckschmidt NE, Zhong W, Oberley TD, et al. Protein kinase Cepsilon interacts with signal transducers and activators of transcription 3 (Stat3), phosphorylates Stat3Ser727 and regulates its constitutive activation in prostate cancer. Cancer Res 2007; 67:8828 - 8838; PMID: 17875724; http://dx.doi.org/10.1158/0008-5472.CAN-06-3350
- Meshki J, Caino MC, von Burstin VA, Griner EM, Kazanietz MG. Regulation of prostate cancer cell survival by protein kinase Cepsilon involves bad phosphorylation and modulation of the TNFalpha/JNK pathway. J Biol Chem 2010; 285:26033 - 26040; PMID: 20566643; http://dx.doi.org/10.1074/jbc.M110.128371
- McJilton MA, Van Sikes C, Wescott GG, Wu D, Foreman TL, Gregory CW, et al. Protein kinase Cepsilon interacts with Bax and promotes survival of human prostate cancer cells. Oncogene 2003; 22:7958 - 7968; PMID: 12970744; http://dx.doi.org/10.1038/sj.onc.1206795
- Wu D, Thakore CU, Wescott GG, McCubrey JA, Terrian DM. Integrin signaling protein kinase Cepsilon to the protein kinase B/Akt survival pathway in recurrent prostate cancer cells. Oncogene 2004; 23:8659 - 8672; PMID: 15467757; http://dx.doi.org/10.1038/sj.onc.1207900
- Wu D, Foreman TL, Gregory CW, McJilton MA, Wescott GG, Ford OH, et al. Protein kinase cepsilon has the potential to advance the recurrence of human prostate cancer. Cancer Res 2002; 62:2423 - 2429; PMID: 11956106
- Gandellini P, Folini M, Longoni N, Pennati M, Binda M, Colecchia M, et al. Cancer Res 2009; 69:2287 - 2295; PMID: 19244118; http://dx.doi.org/10.1158/0008-5472.CAN-08-2894
- Powel CT, Brittis NJ, Stec D, Hug H, Heston WD, Fair WR. Persistent membrane translocation of protein kinase Calpha during 12-0-tetradecanoylphorbol-13-acetate-induced apoptosis of LNCaP human prostate cancer cells. Cell Growth Differ 1996; 7:419 - 428; PMID: 9052983
- Gavrielides MV, Frijhoff AF, Conti CJ, Kazanietz MG. Protein kinase C and prostate carcinogenesis: targeting the cell cycle and apoptotic mechanisms. Curr Drug Targets 2004; 5:431 - 443; PMID: 15216909
- Gonzalez-Guerrico AM, Meshki J, Xiao L, Benavides F, Conti CJ, Kazanietz MG. Molecular mechanisms of protein kinase C-induced apoptosis in prostate cancer cells. J Biochem Mol Biol 2005; 38:639 - 645; PMID: 16336777
- Zhang J, Thomas TZ, Kasper S, Matusik RJ. A small composite probasin promoter confers high levels of prostate-specific gene expression through regulation by androgens and glucocorticoids in vitro and in vivo. Endocrinology 2000; 141:4698 - 4710; PMID: 11108285; http://dx.doi.org/10.1210/en.141.12.4698
- Han G, Buchanan G, Ittmann M, Harris JM, Yu X, Demayo FJ, et al. Mutation of the androgen receptor causes oncogenic transformation of the prostate. Proc Natl Acad Sci USA 2005; 102:1151 - 1156; PMID: 15657128; http://dx.doi.org/10.1073/pnas.0408925102
- Webber MM, Quader ST, Kleinman HK, Bello-DeOcampo D, Storto PD, Bice G, et al. Human cell lines as an in vitro/in vivo model for prostate carcinogenesis and progression. Prostate 2001; 47:1 - 13; PMID: 11304724
- Thiyagarajan S, Bhatia N, Reagan-Shaw S, Cozma D, Thomas-Tikhonenko A, Ahmad N, et al. Role of GLI2 transcription factor in growth and tumorigenicity of prostate cells. Cancer Res 2007; 67:10642 - 10646; PMID: 18006803; http://dx.doi.org/10.1158/0008-5472.CAN-07-2015
- Achanzar WE, Brambila EM, Diwan BA, Webber MM, Waalkes MP. Inorganic arsenite-induced malignant transformation of human prostate epithelial cells. J Natl Cancer Inst 2002; 94:1888 - 1891; PMID: 12488483; http://dx.doi.org/10.1093/jnci/94.24.1888
- Reddig PJ, Dreckschmidt NE, Ahrens H, Simsiman R, Tseng CP, Zou J, et al. Transgenic mice overexpressing protein kinase Cdelta in the epidermis are resistant to skin tumor promotion by 12-O-tetradecanoylphorbol-13-acetate. Cancer Research 1999; 59:5710 - 5718; PMID: 10582689
- Reddig PJ, Dreckschmidt NE, Zou J, Bourguignon SE, Oberley TD, Verma AK. Transgenic mice overexpressing protein kinase Cepsilon in their epidermis exhibit reduced papilloma burden but enhanced carcinoma formation after tumor promotion. Cancer Res 2000; 60:595 - 602; PMID: 10676642
- Wang HQ, Smart RC. Overexpression of protein kinase C-alpha in the epidermis of transgenic mice results in striking alterations in phorbol ester-induced inflammation and COX-2, MIP-2 and TNFalpha expression but not tumor promotion. J Cell Sci 1999; 112:3497 - 3506; PMID: 10504298
- Zhong C, Saribekyan G, Liao CP, Cohen MB, Roy-Burman P. Cooperation between FGF8b overexpression and PTEN deficiency in prostate tumorigenesis. Cancer Res 2006; 66:2188 - 2194; PMID: 16489020; http://dx.doi.org/10.1158/0008-5472.CAN-05-3440
- Kim MJ, Cardiff RD, Desai N, Banach-Petrosky WA, Parsons R, Shen MM, et al. Cooperativity of Nkx3.1 and Pten loss of function in a mouse model of prostate carcinogenesis. Proc Natl Acad Sci USA 2002; 99:2884 - 2889; PMID: 11854455; http://dx.doi.org/10.1073/pnas.042688999
- Li Y, Podsypanina K, Liu X, Crane A, Tan LK, Parsons R, et al. Deficiency of Pten accelerates mammary oncogenesis in MMTV-Wnt-1 transgenic mice. BMC Mol Biol 2001; 2:2; PMID: 11178110; http://dx.doi.org/10.1186/1471-2199-2-2
- Doble BW, Ping P, Kardami E. The epsilon subtype of protein kinase C is required for cardiomyocyte connexin-43 phosphorylation. Circ Res 2000; 86:293 - 301; PMID: 10679481
- Barandon L, Dufourcq P, Costet P, Moreau C, Allières C, Daret D, et al. Involvement of FrzA/sFRP-1 and the Wnt/frizzled pathway in ischemic preconditioning. Circ Res 2005; 96:1299 - 1306; PMID: 15920021; http://dx.doi.org/10.1161/01.RES.0000171895.06914.2c
- Pardo OE, Wellbrock C, Khanzada UK, Aubert M, Arozarena I, Davidson S, et al. FGF-2 protects small cell lung cancer cells from apoptosis through a complex involving PKCepsilon, B-Raf and S6K2. EMBO J 2006; 25:3078 - 3088; PMID: 16810323; http://dx.doi.org/10.1038/sj.emboj.7601198
- Zhang J, Baines CP, Zong C, Cardwell EM, Wang G, Vondriska TM, et al. Functional proteomic analysis of a three-tier PKCepsilon-Akt-eNOS signaling module in cardiac protection. Am J Physiol Heart Circ Physiol 2005; 288:954 - 961; PMID: 15528226; http://dx.doi.org/10.1152/ajpheart.00756.2004
- Lu D, Huang J, Basu A. Protein kinase Cepsilon activates protein kinase B/Akt via DNA-PK to protect against tumor necrosis factor-alpha-induced cell death. J Biol Chem 2006; 281:22799 - 22807; PMID: 16785234; http://dx.doi.org/10.1074/jbc.M603390200
- Kim DJ, Chan KS, Sano S, Digiovanni J. Signal transducer and activator of transcription 3 (Stat3) in epithelial carcinogenesis. Mol Carcinog 2007; 46:725 - 731; PMID: 17610223; http://dx.doi.org/10.1002/mc.20342
- Yu H, Pardoll D, Jove R. STATs in cancer inflammation and immunity: a leading role for STAT3. Nat Rev Cancer 2009; 9:798 - 809; PMID: 19851315; http://dx.doi.org/10.1038/nrc2734
- Tam L, McGlynn LM, Traynor P, Mukherjee R, Bartlett JM, Edwards J. Expression levels of the JAK/STAT pathway in the transition from hormone-sensitive to hormone-refractory prostate cancer. Br J Cancer 2007; 97:378 - 383; PMID: 17595657; http://dx.doi.org/10.1038/sj.bjc.6603871
- Kampfer S, Windegger M, Hochholdinger F, Schwaiger W, Pestell RG, Baier G, et al. Protein Kinase C isoforms involved in the transcriptional activation of cyclin D1 by transforming Ha-Ras. J Biol Chem 2001; 276:42834 - 42842; PMID: 11551901; http://dx.doi.org/10.1074/jbc.M102047200
- Cacace AM, Ueffing M, Han EK, Marmè D, Weinstein IB. Overexpression of PKCepsilon in R6 fibroblasts causes increased production of active TGFbeta. J Cell Physiol 1998; 175:314 - 322; PMID: 9572476; http://dx.doi.org/10.1002/(SICI)1097-4652(199806)175:3<314::AID-JCP9>3.0.CO;2-R
- Koyanagi T, Noguchi K, Ootani A, Inagaki K, Robbins RC, Mochly-Rosen D. Pharmacological inhibition of epsilon PKC suppresses chronic inflammation in murine cardiac transplantation model. J Mol Cell Cardiol 2007; 43:517 - 522; PMID: 17655859; http://dx.doi.org/10.1016/j.tem.2008.10.002
- Churchill EN, Qvit N, Mochly-Rosen D. Rationally designed peptide regulators of protein kinase C. Trends Endocrinol Metab 2009; 20:25 - 33; PMID: 19056296; http://dx.doi.org/10.1016/j.tem.2008.10.002
- Newton PM, Messing RO. The substrates and binding partners of protein kinase Cepsilon. Biochem J 2010; 427:189 - 196; PMID: 20350291
- Greenberg NM, DeMayo FJ, Sheppard PC, Barrios R, Lebovitz R, Finegold M, et al. The rat probasin gene promoter directs hormonally and developmentally regulated expression of a heterologous gene specifically to the prostate in transgenic mice. Mol Endocrinol 1994; 8:230 - 239; PMID: 8170479
- Rodriguez-Puebla ML, LaCava M, Miliani De Marval PL, Jorcano JL, Richie ER, Conti CJ. Cyclin D2 overexpression in transgenic mice induces thymic and epidermal hyperplasia whereas cyclin D3 expression results only in epidermal hyperplasia. Am J Pathol 2000; 157:1039 - 1050; PMID: 10980142
- Sundberg JBD. Systematic Approach to Evaluation of Mouse Mutations 2001; Boca Raton FL CRC Press
- Shappell SB, Thomas GV, Roberts RL, Herbert R, Ittmann MM, Rubin MA, et al. Prostate pathology of genetically engineered mice: definitions and classification. The Consensus Report from the Bar Harbor meeting of the Mouse Models of Human Cancer Consortium Prostate Pathology Committee. Cancer Res 2004; 64:2270 - 2305; PMID: 15026373; http://dx.doi.org/10.1158/0008-5472.CAN-03-0946