Abstract
Skp2, a F-box protein that determines the substrate specificity for SCF ubiquitin ligase, has recently been demonstrated to be degraded by Cdh1/APC in response to TGFβ signaling. The TGFβ-induced Skp2 proteolysis results in the stabilization of p27 that is necessary to facilitate TGFβ cytostatic effect. Previous observation from immunocytochemistry indicates that Cdh1 principally localizes in the nucleus while Skp2 mainly localizes in the cytosol, which leaves us a puzzle on how Skp2 is recognized and then ubiquitylated by Cdh1/APC in response to TGFβ stimulation. Here, we report that Skp2 is rapidly translocated from the cytosol to the nucleus upon the cellular stimulation with TGFβ. Using a combinatorial approach of immunocytochemistry, biochemical-fraction-coupled immunoprecipitation, mutagenesis as well as protein degradation assay, we have demonstrated that the TGFβ-induced Skp2 nucleus translocation is critical for TGFβ cytostatic effect that allows physical interaction between Cdh1 and Skp2 and in turn facilitates the Skp2 ubquitylation by Cdh1/APC. Disruption of nuclear localization motifs on Skp2 stabilizes Skp2 in the presence of TGF-β signaling, which attenuates TGFβ-induced p27 accumulation and antagonizes TGFβ-induced growth inhibition. Our finding reveals a cellular mechanism that facilitates Skp2 ubiquitylation by Cdh1/APC in response to TGFβ.
Introduction
Transforming Growth Factor β (TGFβ) signaling regulates a variety of cellular processes, including cell proliferation, differentiation, apoptosis and fate specification during embryogenesis. Transduction of the complex signaling starts on the cell surface where TGFβ binding induces the formation of type I and II receptor complex. Type II receptor phosphorylates the GS domain of Type I receptor, resulting in kinase activation. Type I receptor then propagates the signal through phosphorylation of Smad2 or Smad3 (receptor-regulated Smad, R-Smad) on the carboxy-terminal SXS motif. Upon phosphorylation, Smad2 or Smad3 releases from SARA (Smad anchor for receptor activation), forms oligomeric complex with Smad4 (co-mediator Smad, Co-Smad) and translocates to the nucleus where they regulate target gene transcription in collaboration with DNA-binding co-factors such as forkhead family member FOXH1, co-activators such as p300 or co-repressors such as Ski.Citation1,Citation2
TGFβ signaling plays a critical role in tumorigenesis of several types of epithelia, paradoxically switching from a role as a tumor suppressor to a promoter of invasiveness and metastasis during tumor progression.Citation3 The cytostatic effect of TGFβ in early stages of tumorigenesis primarily involves activated transcription of the cyclin-dependent kinase inhibitors (CDKI) p15,Citation4 p21,Citation5 and p57,Citation6 and repressed transcription of the growth promoting transcription factors c-Myc and Id. Repression of c-Myc and Id facilitates the induction of p15 and p21, which is a critical prerequisite for the execution of the full cytostatic response of epithelial cells to TGFβ.Citation7–Citation11 Increased expression of p15 facilitates its binding to CDK4 and CDK6 and displaces p27 from these kinases to cyclin E-CDK2, causing CDK2 inhibition. Concomitantly, p21 is induced and binds to CDK2 as well, ensuring a maximal suppression of CDK2 activity and cell cycle arrest at G1/S transition.Citation12 In addition to transcriptional control, the proteolytic regulation of CDKIs is also involved in cell cycle arrest, as mediated by TGFβ. Our recent studies demonstrate that p27, a short-lived regulatory protein, is stabilized in response to TGFβ stimulation through Skp2SCF degradation targeted by Cdh1/APC.Citation13 Since p27 is constitutively degraded by Skp2SCF, the stabilization of p27 by TGFβ facilitates its complete and successful redistribution from CDK4 and CDK6 to cyclin E-CDK to achieve arrest of cell growth.
Although our findings indicate that TGFβ-induced Skp2 degradation is governed by Cdh1/APC, the mechanism by which APC is regulated in response to TGFβ signaling and how Skp2 is ubiquitylated for destruction by Cdh1/APC still remain unclear. Result from our recent immunocytochemistry provided us an unexpected indication that Skp2 resides mainly in the cytosol of Mv1Lu mink epithelial cells in the absence of TGFβ. Given the notion that Cdh1 is principally located in the nucleus, we face a puzzle on how Cdh1/APC and Skp2 communicate with each other given that they are in different cellular compartments not to permit recognition and ubiquitylation of Skp2 by its E3 ligase Cdh1/APC. Using a combinatorial approach encompassing cell biology and biochemistry, we have revealed that Skp2 cellular localization is quite dynamic in response to TGFβ signaling, while Cdh1 localization is relatively stable. Our present results show, upon stimulation with TGFβ, Skp2 is rapidly translocated from the cytosol to the nucleus. The measurement of TGFβ-induced nuclear localization of Skp2 by immunocytochemistry and biochemical cellular fractionation further suggests that Skp2 translocation is a critical condition precedent for the ubiquitin protein ligase Cdh1/APC to physically interact with its substrate Skp2. Characterization of Skp2 translocation by altering its NLS (nuclear localization signal) results in the stabilization of Skp2 in the presence of TGFβ signaling, which consequentially results in a failure to accumulate p27 that is necessary for TGFβ-induced growth inhibition. This present work fills a knowledge gap on how Skp2 is regulated by Cdh1/APC in response to TGFβ for growth inhibition, which further advances our understanding of the molecular basis of TGFβ signaling pathway.
Results
Targeted degradation of Skp2 by Cdh1/APC is involved in TGFβ-induced growth inhibition.
Recent studies have demonstrated that TGFβ signaling pathway is tightly regulated by the UPS (ubiquitin-proteasome system),Citation14 where UPS targets various components of the TGFβ pathway including cytoplasmic second messengers, transmembrane bound receptors and accumulated nuclear proteins.Citation15–Citation17 Our endeavor to search for TGFβ-induced fast turnover proteins led us to identify Skp2 as a rapidly degraded protein in response to TGFβ signaling. As shown in , Skp2 is rapidly degraded in response to TGFβ stimulation, which in turn results in accumulation of p27. The half-life of Skp2 in response to TGFβ signaling is approximately 60 minutes (). Furthermore, Skp2 degradation is blocked by incubating cells with 50 µM of a proteasome inhibitor, MG-132 (). To exclude the possibility that the observed change in Skp2 protein levels is due to altered transcriptional regulation, we measured Skp2 mRNA levels after stimulation with TGFβ by RT-PCR. As shown in , Skp2 mRNA remains constant, suggesting that the drop in Skp2 protein levels is due to protein degradation.
Previous study indicated that Skp2 protein abundance is regulated by Cdh1/APC during cell cycle.Citation18,Citation19 We previously demonstrated that Cdh1/APC activity is enhanced in response to TGFβ stimulation.Citation20 Thus, we tested the possibility that Cdh1/APC is a putative E3 ligase that governs the TGFβ-induced Skp2 degradation. As shown in and E, depletion of Cdh1 by RNA interference in Mv1Lu results in significant attenuation of Skp2 degradation in response to TGFβ, while Skp2 is drastically destroyed in response to TGFβ in the wild-type cells.
To evaluate the biological significance of Skp2 degradation upon TGFβ stimulation, we constructed a stable Skp2 with deletion of its destruction box (ubiquitin-targeting-degron) and further engineered a Mv1Lu cell that stably expresses stabilized Skp2 (D box deletion) (). As shown in and G, failure of Skp2 proteolysis in response to TGFβ stimulation significantly antagonizes TGFβ-induced growth inhibition. Taken all together, our results suggest an important role for Skp2 proteolysis by Cdh1/APC in TGFβ-mediated growth inhibition.
Nuclear localization of Skp2 induced by TGFβ promotes its recognition and degradation by Cdh1/APC.
Previous work suggested that the cellular presence of Cdh1 is in the nucleus.Citation21,Citation22 Localization of Skp2 has been variable depending on cell type.Citation23–Citation27 To assess the localization of Cdh1 and Skp2 in Mv1Lu cell, we performed immunocytochemistry in the presence and absence of TGFβ. Localization of Cdh1 was measured by using monoclonal antibody against Cdh1 and FITC conjugated anti-mouse secondary antibody. As show in , expression of Cdh1 is localized in nucleus, which is consistent with previous observation. To test the localization of Skp2, we used rabbit antibody against Skp2 coupled with Texas Red conjugated secondary antibody. As shown in , the majority of Skp2 in the absence of TGFβ is localized in the cytosol, which is consistent with known previous Skp2 localization in prostate, melanoma, colon, lymphoma and breast cancer tissues.Citation23–Citation27 To examine the potential effect of TGFβ stimulation on cellular translocation of Cdh1 and Skp2, a similar study was performed as described in the above. As shown in , TGFβ stimulation results in significant translocation of Skp2 from the cytosol to the nucleus, while no obvious redistribution of Cdh1 was observed.Citation28 The finding of TGFβ-induced Skp2 nuclear translocation provides us an important clue to explain the possible mechanism of how Skp2 is recognized and catalyzed by Cdh1/APC for ubiquitylation and degradation in response to TGFβ signaling.
To biochemically confirm the observation from our immunostaining, we measured the interaction between Cdh1 and Skp2 in the absence and presence of TGFβ by immunoprecipitation. As shown in , coimunoprecipitation of Cdh1 and Skp2 were detected in nuclear fraction after stimulation with TGFβ. In contrast, no obvious interaction of Cdh1 and Skp2 was observed by co-IP in the cytosol in both absence and presence of TGFβ.
To determine where Cdh1 interacts with Skp2 and catalyzes the ubiquitylation of Skp2, we performed an ubiquitylation assay by using either cytosolic or nuclear fraction. Skp2 was pulled down with antibody against Skp2. The ubiquitin conjugated Skp2 was measured by immunoblotting of Skp2 IP complex with antibody against ubiquitin.Citation13,Citation29 As shown in , Skp2 ubiquitin conjugates were significantly detected in the nuclear fraction after stimulation with TGFβ, while no obvious Skp2 ubiquitin conjugates were visualized in the cytosol fraction in both absence and presence of TGFβ. Taken together, the above results demonstrated Skp2 localization is tightly regulated in response TGFβ, where TGFβ-induced Skp2 nuclear translocation permits recognition of Skp2 by Cdh1/APC and its ubiquitylation by Cdh1/APC.
Identification of nuclear localization signal (NLS) responding to Skp2 translocation in response to TGFβ.
To elucidate the mechanism by which Skp2 translocates into the nucleus in response to TGFβ, we analyzed the Skp2 sequence for a nuclear localization signal (NLS). The search revealed a conserved NLS motif () (cubic.bioc.columbia.edu/newwebsite/services/predict NLS/), a sequence necessary and sufficient for nuclear import of other host proteins.Citation30,Citation31 To test whether the putative NLS in Skp2 mediates its nuclear transport, we deleted the NLS. HA-tagged wild-type Skp2 or NLS mutant Skp2 was transfected into Mv1Lu cells and extracts were prepared from cells exposed (or not exposed) to TGFβ. The fractionated cytosol and nuclear preparations were further examined by immunoblotting. Consistent with the data from the immuno-staining assay, the majority of Skp2 was observed in the cytosol in the absence of TGFβ but redistributed to the nucleus in response to TGFβ stimulation (). As predicted, deletion of the NLS on Skp2 completely blocked TGFβ-induced Skp2 nuclear translocation ( and D). To ask whether nuclear translocation is coupled to Skp2 degradation, we measured the change in Skp2 levels in response to TGFβ. As shown in and F, deletion of the NLS significantly attenuated the Skp2 degradation in response to TGFβ. These results suggest that the NLS in Skp2 is required for TGFβ-induced Skp2 translocation and destruction.
Impaired Skp2 translocation inhibits its degradation and antagonizes TGFβ-induced growth inhibition.
Impaired Skp2 translocation could disturb the recognition and ubiquitylation of Skp2 by Cdh1/APC and cause the stabilization of Skp2 and proteolysis of p27. Failure to degrade Skp2 and the resulting stabilization of p27 in response to TGFβ should antagonize the TGFβ response. To test this hypothesis, wild-type Skp2 or NLS-deleted Skp2 was introduced stably into Mv1Lu cells by retroviral infection ().Citation13,Citation32 Subsequently, the pools of infected cells were measured for their ability to respond to TGFβ-induced growth inhibition.Citation13 As shown in and B, stably expressed Skp2 degraded in response to TGFβ stimulation while NLS-deleted Skp2 was quite stable. Growth inhibition analysis showed that expression of wild-type Skp2 only moderately blocked the ability of cells to undergo TGFβ-induced cell cycle arrest. The reason for this may be because the wild-type Skp2 was unstable in response to TGFβ stimulation and thus failed to accumulate (). In contrast, NLS-deleted Skp2 markedly attenuated TGFβ-induced growth inhibition (). Therefore, failure of Skp2 translocation in response to TGFβ impairs the TGFβ-induced growth inhibitory effect. These results support the hypothesis that TGFβ-induced Skp2 translocation facilitates its degradation by Cdh1/APC, which helps to maintain p27 protein levels to inhibit cyclin E/CDK2.
Discussion
Previous studies from us and others have implicated a role for Cdh1/APC in TGFβ signaling pathway.Citation20,Citation33 Elevated activity for Cdh1/APC was measured in the presence of TGFβ. The TGFβ-induced activation of APC was thought to remove SnoN, a transcriptional co-suppressor, for the induction of TGFβ-responsive genes and to degrade Skp2 to stabilize p27 for TGFβ cytostatic effect.Citation13,Citation32,Citation34 However, how Cdh1/APC targets Skp2 for degradation remains a puzzle since both components are localized in different cellular compartments in the testing model cell line-Mv1Lu. In the present work, finding that Skp2 translocates to the nucleus in response to TGFβ and the further identification of Skp2's nuclear translocation motifs have unveiled the mystery of how Skp2 is recognized for ubiquitylation by Cdh1/APC in the presence of TGFβ signaling. This work elucidates the mechanism by which Skp2 is regulated by Cdh1/APC in TGFβ signaling.
Skp2 was first identified as a cyclinA-CDK2 S-phase kinase-associated proteinCitation35 and subsequently characterized as a rate-limiting component of the SCF machinery that ubiquitylates p27 for degradation, which in turn releases cyclin E-CDK2 and promotes entry into S phase.Citation36,Citation37 p27 functions as a tumor suppressor and its inactivation predisposes mice to tumorigenesis. It has been proposed that Skp2 confers oncogenic function through accelerated degradation of p27 and other tumor suppressors, such as CDKIs p21 and p57; RASSF1 (Ras association domain family 1); and RBL2 (retinoblastoma-like, also known as p130).Citation38 Indeed, increased levels of Skp2 proteins are often observed in many human cancers where Skp2 overexpression is usually inversely correlated with reduced p27 expression, and positively correlated with tumor malignancy and poor prognosis in terms of cancer treatment.Citation39,Citation40 Mechanistic studies show that, in addition to gene amplification and mRNA accumulation, deregulated proteolysis is also involved in elevated Skp2 expression in human cancers. We and other groups have demonstrated that in normal cell cycle, Cdh1/APC targets Skp2 for degradation, thus preventing premature entry into S phase.Citation18,Citation19 Our recent work further reveals that the reduced expression of Cdh1 and inverse elevation of Skp2 are evident in various human cancers. Moreover, the deregulated Cdh1/Skp2/p27 cascade is implicated in the development of breast and colorectal cancer.Citation41–Citation43 The tumor suppressor potential of Cdh1 is further illustrated in knockout mice. Cdh1 heterozygous mice show increased susceptibility to spontaneous tumors.Citation44 Given the pivotal role of TGFβ signaling in tumorigenesis and the notion that Cdh1/APC mediates TGFβ-induced cell growth inhibition as demonstrated here and by other studies,Citation13,Citation34 abrogation of Cdh1/APC function in TGFβ signaling could be one mechanism for the initiation of tumor formation.
Besides aberrant Cdh1 activity, Skp2 cytoplasmic localization might also be another mechanism for the abnormal accumulation of Skp2 protein in human cancers, which allows Skp2 to escape from Cdh1-mediated degradation. Cytoplasmic Skp2 has been observed in many clinical tumor samples and correlated with aggressive malignancy. Recent mechanistic studies suggest that Akt-dependent phosphorylation of Skp2 at Ser 72 is responsible for Skp2 cytoplasmic translocation.Citation27,Citation28 In this study, both immunofluorescence staining and compartmental immunoprecipitation assay shows that Skp2 resides mainly in the cytosol of Mv1Lu mink lung epithelial cells, and translocates to nucleus in response to TGFβ signaling. Identification and characterization of translocation motifs on Skp2 advanced the knowledge about the Cdh1-Skp2-p27-cyclin E/CDC2 axis in TGFβ signaling. Nevertheless, the mechanism of how Skp2 translocation is orchestrated in response to TGFβ is still unknown. Given the report of Skp2 regulation by Akt, it would be interesting to address the possible connection between Akt and the TGFβ-induced localization of Skp2.Citation27,Citation28 It would be helpful to explore how whether TGFβ signaling alters the activity of Akt to control Skp2 cytosol-nucleus trafficking. Ubiquitylation assay indicated that in the presence of TGFβ signaling, Skp2 translocates to the nucleus and is ubiquitylated by Cdh1/APC for degradation. Deletion of the nuclear localization signal (NLS) sequence impairs Skp2 nuclear translocation and inhibits its degradation, which in turn antagonizes growth inhibition by TGFβ. This work implicates that subcellular distribution of Skp2 is an important cellular marker that reflects the status of cell proliferation. Regulation of Skp2 translocation could be a valuable therapeutic strategy to control cell growth. Moreover, cytoplasmic Skp2 has been suggested to promote cell migration and thus has a potential function in tumor metastasis.Citation27 Given the critical role of TGFβ in epithelial-mesenchymal transition (EMT), which correlates to increased invasiveness and metastasis, it would be important to investigate the possible link between TGFβ-induced translocation and degradation of Skp2 and cell motility in the context of TGFβ signaling.
Materials and Methods
Plasmids and constructs.
Skp2ΔNLS (nuclear localization signal deleted Skp2) was constructed by PCR using following primers and then cloned into pCS2-HA or pREX-IRES-CD2, a mammalian expression vector:
5′-AAA ATC GAT ATG CAC GTA TTT AAA ACT CCC GGG CC-3′
5′-AAA GTC TTT GTC ACT CCC TTT GGG GCT CTC CGG GTG GCC CAG GTT-3′
5′-AAA GGG AGT GAC AAA GAC TTT GTG-3′
5′-TTG GCG CGC CTA GAC AAC TGG GCT TTT GCA GTG TC-3′.
pREX-IRES-CD2 is a gift from Dr. Xuedong Liu (University of Colorado-Boulder). pREX-HA-Skp2-IRES-CD2 and pREX-HA-Skp2ΔNLS-IRES-CD2 were generated by PCR using following primers:
5′-AAA AGT CGA CCC ACA GGA AGC ACC TCC AGG AG-3′
5′-TTG CGG CCG CTC ATA GAC AAC TGG GCT TTT G-3′.
Antibodies and immunoprecipitation.
Western blot analysis was performed using the anti-Skp2 (Santa Cruz, sc-7164), anti-p27 (Santa Cruz, sc-776), anti-Cdh1 (Abcam, DCS-266), anti-PCNA (Santa Cruz, sc-56), anti-HA (Santa Cruz, sc-7392), anti-Myc (Santa Cruz, sc-789), anti-Flag (Sigma, F3165), anti-SnoN (Cascade BioScience, ABM-3002), Ubiquitin (BD bioscience, 550944) and HRP-conjugated goat-anti-mouse (Promega, W4021) or anti-rabbit secondary antibody (Promega, W4011) with ECL detection kit (Amersham, RPN2106). Semi-quantification of data was performed using NIH image.
For immunoprecipitation assay, cell lysate was incubated with anti-Cdh1 antibody overnight at 4° on a rotator, following by addition of UltraLink Immobilized Protein A/G (Pierce, 53133). IP-complex was resolved by SDS-PAGE. Western blotting was conducted by using antibody against Skp2.
Growth inhibition assay.
For growth inhibition assay, 5 × 103 Mv1Lu cells were incubated with various concentrations of TGFβ1 (R&D, 40-B) for 3–4 days. The growth of cells was determined by counting compared with that of unstimulated cells.Citation13
Immunofluorescence microscopy.
Cells were washed and fixed in 4% paraformaldehyde solution for 10 min at room temperature and permeabilized with 0.1% Triton X-100 in PBS. Cells with and without stimulation by TGFβ1 were incubated with a rabbit anti-Skp2 and a mouse anti-Cdh1 antibodies followed by incubating with FITC conjugated anti-mouse (Jackson Lab, 115-095-146) and Texas Red conjugated anti-rabbit (Jackson Lab, 111-075-045) secondary antibodies.
Abbreviation
Skp2 | = | S-phase kinase-associated protein 2 |
TGFβ | = | transforming growth factor β |
SCF | = | SKP1-CUL1-F-box protein |
APC | = | anaphase promoting complex |
R-smad | = | receptor-regulated smad |
SARA | = | smad anchor for receptor activation |
Co-Smad | = | co-mediator smad |
CDKI | = | cyclin-dependent kinase inhibitors |
Figures and Tables
Figure 1 Targeting Skp2 by Cdh1/APC for destruction is involved in TGFβ-mediated growth inhibition. (A) Skp2 protein levels drop in response to TGFβ stimulation. Skp2 and actin (control) mRNA levels were monitored by RT-PCR analysis. (B and C) Skp2 protein chase analysis in response to TGFβ stimulation. Mink lung epithelia cells (Mv1Lu) cells were treated with 20 µM cycloheximide. Skp2 protein turnover was measured by immunoblotting. The half-life of Skp2 in response to TGFβ signaling is about 60 minutes while half-life for SnoN is about 30 minutes. p27 protein levels gradually increase while Skp2 protein levels decrease. Mv1Lu were treated with TGFβ (100 pM). Skp2 degradation is further blocked by proteasomal inhibitor, MG-132 (50 µM). Protein levels were measured by immunoblotting. Equal amounts of total protein were subjected to immunoblot analysis, as evidenced by the equal concentration of tubulin. (D) TGFβ-induced Skp2 degradation is mediated via Cdh1/APC. Depletion of Cdh1 blocks Skp2 degradation in response to TGFβ stimulation, which in turn results in lower levels of p27. (E) Summary of TGFβ-induced alteration of Skp2 and p27 protein levels in wild-type and Cdh1 knockdown cells. (F) Disruption of destruction box in Skp2 stabilizes Skp2 protein in the presence of TGFβ. Upper part shows construction of retroviral vector harboring wild-type or non-degradable Skp2. (G) Stabilization of Skp2 antagonizes TGFβ-induced growth inhibition. Mv1Lu cells stably expressing Skp2 or Skp2Δdb respectively were incubated for 4 days with various concentration of TGFβ1 as indicated. The growth of cells was quantified by cell counting and compared with the growth of unstimulated cells.
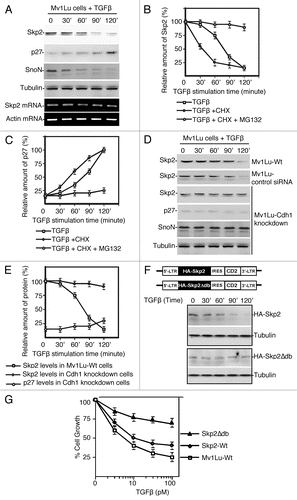
Figure 2 Skp2 translocation facilitates recognition of Skp2 by Cdh1/APC that ensures the TGFβ-induced Skp2 degradation. (A) TGFβ-induced translocation of Skp2 generates compartmental interaction for Skp2 and Cdh1. In the absence of TGFβ, Cdh1 is mainly localized in the nucleus, while Skp2 is principally expressed in the cytosol in Mv1Lu cells indicating by immuno-staining using antibodies against Cdh1 and Skp2. In the presence of TGFβ, Skp2 are translocated into the nucleus and therefore meet with Cdh1, measuring between 30–60 minutes after stimulation with TGFβ. (B) Time-dependent interaction between Skp2 and Cdh1 in response to TGFβ signaling. Mv1Lu cell were treated with TGFβ and collected at various time points. Cytosol and nuclear lysates were prepared for immunoprecipitation. Cdh1 IP complexes from cytosol and nucleus were immunoblotted with antibody against Skp2. Transient interaction of Cdh1 and Skp2 was only observed in the nucleus but not in the cytosol. (C) Skp2 forms ubiquitin-conjugates in the nucleus in response to TGFβ signaling. Skp2 ubiquitin-conjugates were pulled down by immunoprecipitation followed by immunoblotting using antibody against ubiquitin.
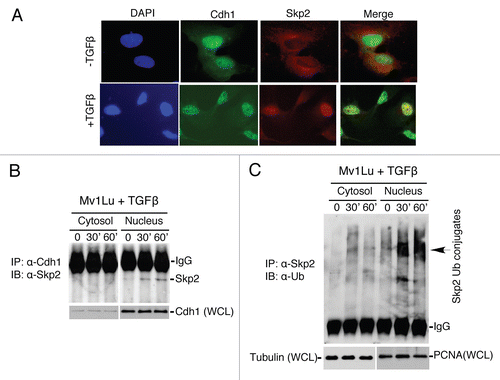
Figure 3 Identification of a nuclear localization signal (NLS) in Skp2 that facilitates Skp2 translocation and degradation in response to TGFβ signaling. (A) Skp2 contains a conserved nuclear localization signal (NLS) motif. The alignment was performed using the CLUSTA W method. (B) Translocation of endogenous Skp2 in response to TGFβ signaling. Cells were collected 30 minutes after the stimulation with TGFβ. (C) Translocation of HA tagged Skp2 in response to TGFβ signaling. (D) Disruption of NLS in Skp2 abrogates Skp2 translocation in response to TGFβ signaling.
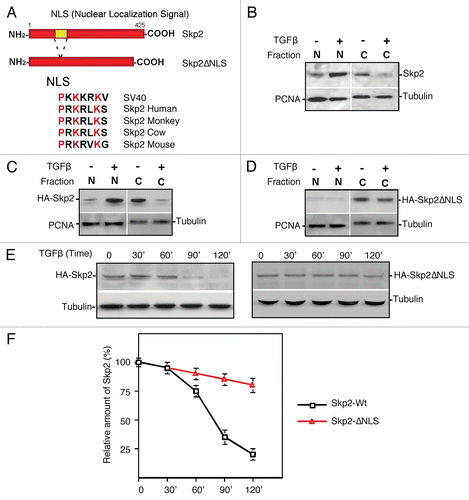
Figure 4 Disruption of Skp2 translocation attenuates its degradation in response to TGFβ, which in turn antagonizes TGFβ-induced growth inhibition. (A) Deletion of NLS in Skp2 impairs Skp2 degradation and p27 accumulation induced by TGFβ. Upper part indicates the retro-viral expression vector of wild-type and NLS Skp2 mutant. (B) Summary of protein degradation for wild-type and NLS Skp2 mutant in response to TGFβ signaling. (C) Impaired Skp2 translocation leads to attenuation of TGFβ-induced growth inhibition. Mv1Lu cells stably expressing Skp2 or Skp2DNLS respectively were incubated for four days with various concentration of TGFβ1 as indicated. The growth of cells was quantified by cell counting and compared with the growth of unstimulated cells.
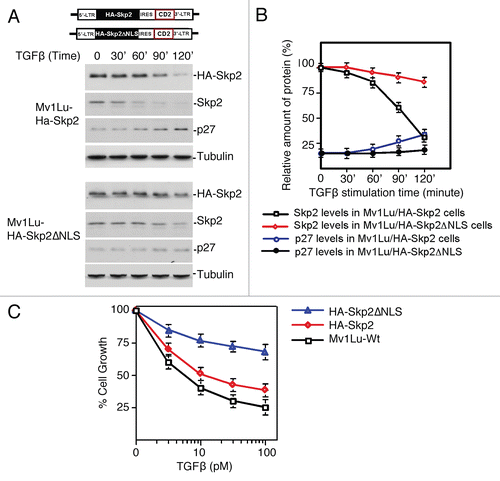
Additional material
Download Zip (502.2 KB)Acknowledgements
We thank Drs. X. Liu, W. Malcolm, W. Kaelin, A. Weissman and D. Zhang for cDNA clones. We are grateful to members of our laboratory for critical reading of the manuscript. This work is supported by NIH grants CA115943. Y. Wan is a scholar of American Cancer Society and V Cancer Research Foundation.
References
- Shi Y, Massague J. Mechanisms of TGFbeta signaling from cell membrane to the nucleus. Cell 2003; 113:685 - 700
- Siegel PM, Massague J. Cytostatic and apoptotic actions of TGFbeta in homeostasis and cancer. Nat Rev Cancer 2003; 3:807 - 821
- Massague J. TGFbeta in Cancer. Cell 2008; 134:215 - 230
- Hannon GJ, Beach D. p15INK4B is a potential effector of TGFbeta-induced cell cycle arrest. Nature 1994; 371:257 - 261
- Datto MB, Li Y, Panus JF, Howe DJ, Xiong Y, Wang XF. Transforming growth factor beta induces the cyclin-dependent kinase inhibitor p21 through a p53-independent mechanism. Proc Natl Acad Sci USA 1995; 92:5545 - 5549
- Scandura JM, Boccuni P, Massague J, Nimer SD. Transforming growth factor beta-induced cell cycle arrest of human hematopoietic cells requires p57KIP2 upregulation. Proc Natl Acad Sci USA 2004; 101:15231 - 15236
- Pardali K, Kowanetz M, Heldin CH, Moustakas A. Smad pathway-specific transcriptional regulation of the cell cycle inhibitor p21(WAF1/Cip1). J Cell Physiol 2005; 204:260 - 272
- Kowanetz M, Valcourt U, Bergstrom R, Heldin CH, Moustakas A. Id2 and Id3 define the potency of cell proliferation and differentiation responses to transforming growth factor beta and bone morphogenetic protein. Mol Cell Biol 2004; 24:4241 - 4254
- Seoane J, Le HV, Massague J. Myc suppression of the p21(Cip1) Cdk inhibitor influences the outcome of the p53 response to DNA damage. Nature 2002; 419:729 - 734
- Seoane J, Pouponnot C, Staller P, Schader M, Eilers M, Massague J. TGFbeta influences Myc, Miz-1 and Smad to control the CDK inhibitor p15INK4b. Nat Cell Biol 2001; 3:400 - 408
- Staller P, Peukert K, Kiermaier A, Seoane J, Lukas J, Karsunky H, et al. Repression of p15INK4b expression by Myc through association with Miz-1. Nat Cell Biol 2001; 3:392 - 399
- Reynisdottir I, Polyak K, Iavarone A, Massague J. Kip/Cip and Ink4 Cdk inhibitors cooperate to induce cell cycle arrest in response to TGFbeta. Genes Dev 1995; 9:1831 - 1845
- Liu W, Wu G, Li W, Lobur D, Wan Y. Cdh1-anaphase-promoting complex targets Skp2 for destruction in transforming growth factor beta-induced growth inhibition. Mol Cell Biol 2007; 27:2967 - 2979
- Datto M, Wang XF. Ubiquitin-mediated degradation a mechanism for fine-tuning TGFbeta signaling. Cell 2005; 121:2 - 4
- Wrighton KH, Feng XH. To (TGF)beta or not to (TGF)beta: fine-tuning of Smad signaling via post-translational modifications. Cell Signal 2008; 20:1579 - 1591
- Lonn P, Moren A, Raja E, Dahl M, Moustakas A. Regulating the stability of TGFbeta receptors and Smads. Cell Res 2009; 19:21 - 35
- Liang M, Liang YY, Wrighton K, Ungermannova D, Wang XP, Brunicardi FC, et al. Ubiquitination and proteolysis of cancer-derived Smad4 mutants by SCFSkp2. Mol Cell Biol 2004; 24:7524 - 7537
- Bashir T, Dorrello NV, Amador V, Guardavaccaro D, Pagano M. Control of the SCF(Skp2-Cks1) ubiquitin ligase by the APC/C(Cdh1) ubiquitin ligase. Nature 2004; 428:190 - 193
- Wei W, Ayad NG, Wan Y, Zhang GJ, Kirschner MW, Kaelin WG Jr. Degradation of the SCF component Skp2 in cell cycle phase G1 by the anaphase-promoting complex. Nature 2004; 428:194 - 198
- Wan Y, Liu X, Kirschner MW. The anaphase-promoting complex mediates TGFbeta signaling by targeting SnoN for destruction. Mol Cell 2001; 8:1027 - 1039
- Zhou Y, Ching YP, Chun AC, Jin DY. Nuclear localization of the cell cycle regulator CDH1 and its regulation by phosphorylation. J Biol Chem 2003; 278:12530 - 12536
- Jaquenoud M, van Drogen F, Peter M. Cell cycle-dependent nuclear export of Cdh1p may contribute to the inactivation of APC/C(Cdh1). EMBO J 2002; 21:6515 - 6526
- Drobnjak M, Melamed J, Taneja S, Melzer K, Wieczorek R, Levinson B, et al. Altered expression of p27 and Skp2 proteins in prostate cancer of African-American patients. Clin Cancer Res 2003; 9:2613 - 2619
- Li Q, Murphy M, Ross J, Sheehan C, Carlson JA. Skp2 and p27kip1 expression in melanocytic nevi and melanoma: an inverse relationship. J Cutan Pathol 2004; 31:633 - 642
- Lim MS, Adamson A, Lin Z, Perez-Ordonez B, Jordan RC, Tripp S, et al. Expression of Skp2, a p27(Kip1) ubiquitin ligase, in malignant lymphoma: correlation with p27(Kip1) and proliferation index. Blood 2002; 100:2950 - 2956
- Radke S, Pirkmaier A, Germain D. Differential expression of the F-box proteins Skp2 and Skp2B in breast cancer. Oncogene 2005; 24:3448 - 3458
- Lin HK, Wang G, Chen Z, Teruya-Feldstein J, Liu Y, Chan CH, et al. Phosphorylation-dependent regulation of cytosolic localization and oncogenic function of Skp2 by Akt/PKB. Nat Cell Biol 2009; 11:420 - 432
- Gao D, Inuzuka H, Tseng A, Chin RY, Toker A, Wei W. Phosphorylation by Akt1 promotes cytoplasmic localization of Skp2 and impairs APCCdh1-mediated Skp2 destruction. Nat Cell Biol 2009; 11:397 - 408
- Bloom J, Pagano M. Experimental tests to definitively determine ubiquitylation of a substrate. Methods Enzymol 2005; 399:249 - 266
- Jans DA, Xiao CY, Lam MH. Nuclear targeting signal recognition: a key control point in nuclear transport?. Bioessays 2000; 22:532 - 544
- Mattaj IW, Englmeier L. Nucleocytoplasmic transport: the soluble phase. Annu Rev Biochem 1998; 67:265 - 306
- Sun Y, Liu X, Ng-Eaton E, Lodish HF, Weinberg RA. SnoN and Ski protooncoproteins are rapidly degraded in response to transforming growth factor beta signaling. Proc Natl Acad Sci USA 1999; 96:12442 - 12447
- Stroschein SL, Bonni S, Wrana JL, Luo K. Smad3 recruits the anaphase-promoting complex for ubiquitination and degradation of SnoN. Genes Dev 2001; 15:2822 - 2836
- Wang W, Ungermannova D, Jin J, Harper JW, Liu X. Negative regulation of SCFSkp2 ubiquitin ligase by TGFbeta signaling. Oncogene 2004; 23:1064 - 1075
- Zhang H, Kobayashi R, Galaktionov K, Beach D. p19Skp1 and p45Skp2 are essential elements of the cyclin A-CDK2 S phase kinase. Cell 1995; 82:915 - 925
- Carrano AC, Eytan E, Hershko A, Pagano M. SKP2 is required for ubiquitin-mediated degradation of the CDK inhibitor p27. Nat Cell Biol 1999; 1:193 - 199
- Tsvetkov LM, Yeh KH, Lee SJ, Sun H, Zhang H. p27(Kip1) ubiquitination and degradation is regulated by the SCF(Skp2) complex through phosphorylated Thr187 in p27. Curr Biol 1999; 9:661 - 664
- Frescas D, Pagano M. Deregulated proteolysis by the F-box proteins SKP2 and beta-TrCP: tipping the scales of cancer. Nat Rev Cancer 2008; 8:438 - 449
- Signoretti S, Di Marcotullio L, Richardson A, Ramaswamy S, Isaac B, Rue M, et al. Oncogenic role of the ubiquitin ligase subunit Skp2 in human breast cancer. J Clin Invest 2002; 110:633 - 641
- Hershko DD. Oncogenic properties and prognostic implications of the ubiquitin ligase Skp2 in cancer. Cancer 2008; 112:1415 - 1424
- Fujita T, Liu W, Doihara H, Date H, Wan Y. Dissection of the APCCdh1-Skp2 cascade in breast cancer. Clin Cancer Res 2008; 14:1966 - 1975
- Fujita T, Liu W, Doihara H, Wan Y. Regulation of Skp2-p27 axis by the Cdh1/anaphase-promoting complex pathway in colorectal tumorigenesis. Am J Pathol 2008; 173:217 - 228
- Fujita T, Liu W, Doihara H, Wan Y. An in vivo study of Cdh1/APC in breast cancer formation. Int J Cancer 2009; 125:826 - 836
- Garcia-Higuera I, Manchado E, Dubus P, Canamero M, Mendez J, Moreno S, et al. Genomic stability and tumour suppression by the APC/C cofactor Cdh1. Nat Cell Biol 2008; 10:802 - 811