Abstract
The histone acetyltransferase coactivators CBP (CREBBP) and p300 (EP300) have more than 400 described protein interaction partners and are implicated in numerous transcriptional pathways. We have shown previously that CBP and p300 double knockout mutations in mouse embryonic fibroblasts (dKO MEFs) result in mixed effects on cAMP-inducible gene expression, with many CREB target genes requiring CBP/p300 for full expression, while others are unaffected or expressed better in their absence. Here we used CBP and p300 dKO MEFs to examine gene expression in response to four other signals: DNA damage (via p53), double-stranded RNA, serum, and retinoic acid. We found that while retinoic acid-inducible gene expression tends to be uniformly dependent on CBP/p300, dsRNA- and serum-inducible genes displayed non-uniform requirements for CBP/p300, with the dsRNA-inducible expression of Ifnb1 (interferon-β) being particularly dependent on CBP/p300. Surprisingly, the p53-dependent genes Cdkn1a (p21/CIP/WAF) and Mdm2 did not require CBP/p300 for their expression. As with cAMP-responsive CREB targets, we propose that the signal-responsive recruitment of CBP and p300 does not necessarily indicate a requirement for these coactivators at a locus. Rather, target gene context (e.g. DNA sequence) influences the extent to which transcription requires CBP/p300 versus other coactivators, which may not be HATs.
The Histone Acetyltransferases CBP and p300 are Widely Recruited Coactivators that are Required for Normal Development
CBP (Crebbp) and p300 (Ep300) are transcriptional coactivators with roles in many transcriptional programs and biological processes.Citation1–Citation3 More than 400 different proteins, including many transcription factors, have been reported to interact physically or functionally with CBP/p300.Citation4 Indeed, locating sites of CBP and p300 recruitment in the genome is used to identify gene-distal enhancers.Citation5,Citation6
CBP and p300 possess intrinsic histone/protein lysine acetyltransferase activity (HAT, also KAT or PAT), and together they form the two member KAT3 family of HATs.Citation7 With the exception of the bromodomain, CBP/p300 bear little sequence similarity to other KAT families even in the HAT domain.Citation4,Citation8 This suggests CBP and p300 should have unique functions that cannot be substituted for by other HATs. For instance, the acetylation of histone H3 lysine 18 is completely dependent on CBP/p300 in vivo.Citation9
CBP and p300 are required for normal development, as mice that are null for either gene or that are heterozygous for both CBP and p300 die as embryos.Citation10–Citation12 In addition, loss of CBP and p300 is highly deleterious to T and B lymphocytes in mice.Citation13,Citation14 To study the role of CBP and p300 in transcription, we therefore used primary mouse embryonic fibroblasts (MEFs) to conditionally knockout CBP and p300 in vitro. MEFs bearing homozygous conditional knockout alleles of both CBP and p300 were treated with Cre recombinase-expressing adenovirus in vitro, which quickly and efficiently eliminates CBP/p300 protein.Citation9 Although they cannot proliferate, the CBP/p300 double knockout (dKO) MEFs are viable for weeks, allowing interrogation of signal-dependent transcriptional requirements for CBP and p300.
Mutation of CBP and p300 Results in Non-Uniform Effects on cAMP-Dependent Transcription
Previously we reported the effects on cAMP-responsive transcription by two types of CBP and p300 mutations: point mutations in the KIX domain of CBP and p300,Citation15 and conditional knockouts ().Citation9 In both cases we found that mutation of CBP and p300 produced heterogeneous effects on the cAMP-dependent expression of CREB target genes. Mutation of the KIX domain that disrupts the main interaction of CBP/p300 with CREB reduces the expression of most CREB targets, but expression of some genes remains unaffected;Citation15 similar effects were seen in dKO MEFs () but there was an additional category of CREB targets that were more highly expressed in the absence of CBP and p300 ().Citation9
We recently showed that cAMP-responsive genes that are more dependent on CBP/p300 have a statistically significant tendency to have fewer CREB binding sites, less bound CREB and fewer recruited CREB-coactivators.Citation9 In fact, a non-HAT coactivator of CREB, CRTC2 is able to rescue some CBP/p300-dependent genes and knockdown of CRTC2 reduces the expression of CBP/p300-independent genes.Citation9 Thus, for at least some CREB target genes, non-HAT coactivators such as CRTC can buffer the loss of CBP/p300.Citation9 Together these findings led to the “coactivator-poor versus coactivator-rich” model of CBP/p300-dependence described by Kasper et al.Citation9
Is Non-uniform Dependence on CBP/p300 a Common Trait of Signal-Dependent Transcription?
To investigate how widespread unequal dependence on CBP/p300 might be, we used dKO MEFs to look at transcription in response to DNA damage, dsRNA, serum and retinoic acid. These pathways represent diverse classes of signal transductionCitation16 and have all been modeled to require CBP/p300 for the transcription of their target genes.
Expression of the Archetype p53 Target Genes p21 and Mdm2 in MEFs does not Require CBP/p300
In response to DNA damage, p53 is stabilized and activates transcriptional programs to either repair DNA or kill the damaged cell.Citation17 CBP and p300 are modeled to be essential for p53 target gene expression by acetylating p53 and by binding its transactivation domain; the latter positions CBP/p300 coactivation functions at promoters.Citation18–Citation24 CBP/p300 are also reported to impact p53 degradation through interaction with Mdm2 and via a CBP/p300 intrinsic E4 polyubiquitin ligase activity.Citation25,Citation26
We assessed the effect of CBP/p300 deletion on the classic p53 target genes, Cdkn1a (p21) and Mdm2 in response to DNA damage. Treatment with the topoisomerase II inhibitor, etoposide stabilized p53 protein in both wild type (WT) and dKO MEFs (), and resulted in a mild induction of Cdkn1a and Mdm2 mRNAs that was independent of CBP/p300 ( and C). Likewise, doxorubicin, which intercalates into DNA and stabilizes p53 (), modestly induced Cdkn1a and Mdm2 mRNAs, but the loss of CBP/p300 did not reduce basal or inducible expression ( and F). In fact, although not statistically significant, the trend was for higher expression of Cdkn1a and Mdm2 in dKO MEFs (, C, E and F). Generating triple-knockout MEFs that lack CBP, p300 and p53 (TKO MEFs) verified that the expression of Cdkn1a and Mdm2 was p53-dependent even in the absence of CBP/p300 ( and F).
A large literature models that CBP and p300 have essential roles for p53-dependent transcription, so we were surprised that Cdkn1a and Mdm2 were not repressed in dKO MEFs. Although it remains possible that other (as yet untested) p53 target genes are dependent on CBP/p300 for their expression, our data demonstrates that there is no absolute requirement for CBP and p300 in p53-dependent transcription. Furthermore, whether CBP and p300 play a major role in p53 activation and transactivation function in other cell types and cell stresses also remains to be determined. As for the trend towards enhanced expression of Cdkn1a and Mdm2 in dKO MEFs, this may be caused by the increased availability or activity of other p53-coactivators when CBP/p300 are absent. This scenario would be analogous to the increased expression of certain CREB-targets () that is dependent on CRTC. Other HATs such as PCAF and the MYST family have also been reported to acetylate p53,Citation24,Citation27 and it is possible they are critical for p53 function rather than CBP/p300. Alternatively, non-HAT coactivators of p53 may act redundantly for CBP/p300 at Cdkn1a and Mdm2.
Ifnb1 (Interferon-beta) Expression Requires CBP/p300, but TLR3/NFκB/IRF3 Target Gene Expression is not Uniformly Repressed in dKO MEFs
Treatment of cells with dsRNA mimics viral infection and results in the induction of an antiviral response mediated through Toll-like receptor 3 (TLR3) and the transcription factors NFκB and IRF3 with consequent upregulation of target genes such as Ifnb1 (IFNβ), Ccl5 (RANTES) and Cxcl10 (IP-10).Citation28 Both NFκB and IRF3 are coactivated by CBP/p300,Citation29 and the NFκB/IRF3 complex is part of the Ifnb1 enhanceosome that recruits p300 and CBP.Citation30–Citation32 The HAT coactivators PCAF/GCN5 and the chromatin remodeling complex SWI/SNF are also modeled to play a role in transcription of Ifnb1.Citation33
To determine whether dsRNA-responsive gene expression was affected in dKO MEFs, we looked at the expression of Ifnb1, Ccl5 and Cxcl10 which are all reported to be regulated by both NFκB and IRF3 in response to TLR3 signaling,Citation28 and Il6 which is also a target of both NFκB and the IRF family, although whether NFκB and IRF synergize at this promoter is less clear.Citation34–Citation36 We found that while dsRNA-inducible expression of Ifnb1, Ccl5 and Il6 was significantly decreased in dKO MEFs compared to WT MEFs () the expression of Cxcl10 was not (). For all four genes, dsRNA-dependent recruitment of CBP/p300 to the NFκB binding site (or enhanceosome site for Ifnb1,Citation30) in the promoter was verified by ChIP assay ().
One model of the Ifnb1 enhanceosome has the HATs PCAF and GCN5 being initially recruited and later replaced by CBP.Citation33 Thus, for Ifnb1, temporal constraints may restrict the ability of other coactivators to act redundantly for CBP/p300. As for other target genes, the arginine methyltransferase CARM may provide redundant coactivator function as it is required for the expression of some but not all NFκB targets in response to lipopolysaccharide.Citation37 Indeed, Cxcl10 is relatively independent of CBP/p300 in our system (), but has been shown to be strongly dependent on CARM.Citation37
Serum Inducible Expression of Some Genes is Upregulated in the Absence of CBP/p300
The transcription factor SRF (serum response factor), binds to SREs (serum response elements) in the promoters of many genes that respond to mitogenic and growth factor stimulation.Citation38,Citation39 SRF target genes are proposed to be activated via two mechanisms; one involving recruitment of both SRF and a member of the TCF (Ternary Complex Factor) family of Ets transcription factors, such as ELK1 or SAP1, and another where a myocardin-related transcription factor (MKL or MRTF) acts as a cofactor for SRF.Citation40–Citation44 While there is conflicting evidence for which mechanisms regulate individual SRF targets, it seems clear that Srf expression is more dependent on the MKL family than the TCF family, whereas Fos and Egr2 are less dependent on the MKL family, possibly because both mechanisms act redundantly at these genes.Citation41,Citation42,Citation45 CBP/p300 have been reported to be coactivators for SRF, as well as TCF and MKL family members.Citation46–Citation48
We investigated the role of CBP/p300 in serum-inducible transcription in MEFs starved overnight (0.1% serum) then stimulated for one hour with 10% serum. Many of the genes tested showed a moderate to marked increase in serum-induced gene expression in dKO compared to WT MEFs (). These hyper-induced genes included previously described SRF target genes that are dependent on either or both TCF and MKL cofactorsCitation41 (Fos, Egr2, Srf, Fosb, Egr1 and Junb, ) as well as MKL-dependent genes not previously described to be SRF targetsCitation41 (Nr4a1, Ctgf and Btg2, ). We verified by ChIP that CBP and p300 are indeed recruited to the promoters of two hyper-activated genes, Egr1 and Egr2, in response to serum (). In addition we found two MKL-dependent genes, Il6 and Ereg,Citation41 which have decreased serum-inducible gene expression in the absence of CBP/p300 ( and K). Interestingly, Areg, a CREB target that is highly dependent on CBP/p300 for its induction by cAMP () did not require CBP/p300 for serum-inducible expression ().
There are several possible explanations for the general increase in serum-responsive transcription in dKO MEFs. First, SRF regulates the transcription of its own gene, so the increased expression of Srf () in dKO MEFs may further potentiate the expression of other SRF targets. Second, some SRF targets (e.g., Egr1, Egr2 and Fos) require the coactivator Mediator for serum-responsiveness.Citation49 Perhaps Mediator is a stronger coactivator than CBP/p300 but competes with CBP/p300 for binding, such that removal of CBP/p300 increases Mediator recruitment and transactivation. Another possibility is that CBP/p300 function as repressors in certain contexts.
CREB has been reported to be a partner to SRF and the TCF family members and utilize CBP/p300 in the induction of some stress-responsive gene expression.Citation50 Indeed, many of the genes tested in and are induced by both the cAMP-agonists forskolin and IBMX (FI), and serum. Interestingly, however, we found only partial concordance for CBP/p300 dependence in response to the two stimuli. While Fos, Junb, Nr4a1 and Il6 all showed a similar requirement for CBP/p300 under both stimulatory conditions, Fosb and Btg2 were upregulated by loss of CBP/p300 when stimulated by serum, but not by FI ( and ) and Kasper et al.Citation9 In contrast, Areg and Ereg were much more dependent on CBP/p300 for FI-inducible expression ( and C),Citation9 compared to serum ( and L). The difference in the CBP/p300 dependence of these genes in response to serum and cAMP suggests either that CREB is not the primary factor dictating their expression under both conditions, or that the signal-responsive coactivation mechanisms are distinct.
Retinoic Acid Responsive Genes Require CBP/300 for Maximal Induction
The retinoic acid signaling pathway affects many biological processes.Citation51 The retinoic acid receptor (RAR) binds as a heterodimer with retinoid X receptor (RXR) to retinoic acid response elements (RARE).Citation52 Like other nuclear receptors, in the presence of ligand RAR recruits coactivators such as CBP/p300 that are thought to be important for signal transduction.Citation53,Citation54
We tested whether CBP/p300 are critical for retinoic acid-dependent transcription by treating WT and dKO MEFs for four hours with 100 µM all trans retinoic acid (RA). The RA-inducible expression of five RAR target genes (Rarb, Crabp2, Inmt, Wnt10b and Tgm2,Citation55,Citation56) was decreased in dKO compared to WT MEFs (). Since a decrease in Rarb (RARβ) gene expression could produce downstream effects on RAR-dependent gene expression, we also checked the non-RA-inducible gene Rara (RARα) and found its expression unimpaired by the loss of CBP/p300 () suggesting that the RAR machinery is not completely impacted during the short time of the assay (4 hours). Likewise, the Rarb and Crabp2 promoters showed RA-inducible recruitment of CBP/p300 by ChIP ( and B), arguing for a direct effect of CBP/p300 loss on the expression of those genes.
Our data suggests that most RA-inducible genes require CBP/p300 and is in agreement with the results of Jin et al. who also found that nuclear receptor target genes, including RA targets, require CBP/p300 for their expression in immortalized MEFs.Citation57 However, caution is called for when extrapolating the results for five genes () to all RA targets. A wider survey of RA-dependent transcription may reveal a spectrum of CBP/p300 dependence.
Recruitment of CBP/p300 does not Always Equate with Function
This study extends prior work on cAMP and CREB to other signaling pathways (dsRNA and serum), where we see a trend for loss of CBP/p300 recruitment to broadly correlate with reduced transcription. However, we also see that CBP and p300 are recruited to genes where they are not required for expression, or are even repressive ( and ).Citation9 Why might CBP/p300 recruitment occur when not needed? One possibility is that recruited coactivators like CBP/p300 have locally cryptic functions that would be revealed under different biological contexts (e.g., changes in signal strength or duration) or which have nothing to do with the transcription of the target gene. Another scenario is illustrated by analogy to a plumber (i.e., the transcription factor) who goes to different job sites (i.e., target genes) with the same set of tools (i.e., coactivators and corepressors), even though different jobs may require different subsets of those tools.
Coactivator-Poor and Coactivator-Rich Model for CBP/p300 Dependence
The gene characteristics (e.g., DNA sequence) that determine the degree to which endogenous CREB targets require CBP/p300 are not entirely known. However, our previous study showed that the number of CREB binding sites, and to a lesser extent CpG motifs, appear to contribute to CBP/p300-independence for endogenous genes but not for plasmid reporters.Citation9 The amount of CREB bound at an endogenous promoter correlated well with the amount of recruited CBP/p300 and CRTC2, which we propose provides buffering transactivation mechanisms in certain gene contexts. Thus, endogenous promoters that are CREB-rich, and hence coactivator-rich (i.e., rich for amount and diversity), tend to be more resistant to the loss of CBP/p300. Whether this “coactivator-rich/coactivator-poor” model applies to endogenous genes that respond to other CBP/p300-dependent transcription factors and signaling pathways remains to be tested, but work by Koutroubas et al. shows that increasing the enhancer strength of NFκB-responsive reporter plasmids bypasses the need for histone acetylation.Citation58 Unexpectedly, the “coactivator-rich/coactivator-poor” model predicts that CBP and p300 are more likely to be important for the expression of genes where their recruitment is weak to moderate. This has implications for interpreting genome-wide mapping studies of CBP/p300 based on ChIP-seq and ChIP-chip.
Two additional surprises were revealed by our previous dKO MEF study of cAMP-responsive transcription.Citation9 First, the local abundance of histone acetylation, as with CBP/p300 recruitment, tended to inversely correlate with dependence on CBP/p300 for transcription. Second, the non-HAT CRTC can provide redundant function for the HATs CBP and p300 in certain gene contexts. Whether similar phenomenon will be observed for other signaling pathways is unknown but the dKO MEF system will be useful for such studies. Finally, from the perspective of normal biology, it is possible that the different requirements that genes have for coactivators provides a mechanism for altering patterns of signal-responsive gene expression through the physiological- or developmental-dependent modulation of coactivator availability.
Materials and Methods
Culture and treatment of MEFs.
CBP/p300 dKO MEFs were generated as previously described in reference Citation9. Primary MEFs were transduced with adenovirus expressing cre recombinase (MOI 100) for 16 h and experiments (ChIP or RNA) were performed four days after transduction. Deletion of CBP and p300 was confirmed by semi-quantitative genomic PCR and by western blot. All experiments used primary MEFs at passages 3 to 6. For RNA experiments, treatment times and concentrations were as follows: 3 h with 100 µm etoposide (Sigma, E1383), 4 h with 0.2 µg/ml doxorubicin (Sigma D1515), 1 h with 100 µg/ml Poly I:Poly C (Sigma P0913) and 500 µg/ml DEAE dextran (Sigma D9885) followed by a wash and 3 h incubation in fresh medium, 1 h with 10% serum (Hyclone), 90 min with 10 µM forskolin plus 0.1 mM IBMX (FI) (Sigma F6886, I5879) and 4 h with 1 µM retinoic acid (Sigma R2625). For serum and FI induction, MEFs were cultured for 16 h in low serum (0.1%) medium before the experiment. MEFs for retinoic acid experiments were cultured in charcoal stripped serum and DMEM lacking phenol red.
Quantitative real-time reverse-transcriptase-linked PCR (qRT-PCR).
Measurement of gene expression normalized to Pgk1 or Gapdh mRNA was performed using qRT-PCR as previously described in reference Citation9.
Chromatin immunoprecipitation.
ChIP assays were carried out as described previously in reference Citation15. Briefly, treated MEFs were incubated for 20 min in 3% formaldehyde in PBS, the cells were lysed and the lysates sonicated at five times for 10 sec each at 15 microns in a Soniprep 150 (Sanyo). For ChIP experiments, MEFs were treated under the conditions indicated above for RNA with the following changes to time: 1 h with dsRNA, 30 min with serum and 1 h with retinoic acid.
Antibodies.
The CBP and p300 antibodies were previously reported in reference Citation9. The p53 antibody was from Santa Cruz (Cat. # SC-126X) and the actin antibody was from Sigma (Cat. # 5414).
Abbreviations
CBP | = | CREB binding protein |
CBP/p300 | = | CBP and p300 |
ChIP | = | chromatin immunoprecipitation |
CREB | = | cAMP response element binding protein |
CRTC | = | CREB regulated transcription coactivator |
dKO | = | CBP/p300 double knockout |
dsRNA | = | double stranded RNA |
FI | = | forskolin plus 3-isobutyl-1-methylxanthine |
HAT | = | histone acetyltransferase |
KIX | = | CREB-binding domain of CBP |
MEF | = | mouse embryonic fibroblast |
MKL | = | myocardin-related transcription factor |
qPCR | = | quantitative polymerase chain reaction |
qRT-PCR | = | quantitative real-time reverse transcriptase polymerase chain reaction |
RA | = | retinoic acid |
RAR | = | retinoic acid receptor |
RARE | = | retinoic acid response element |
SRE | = | serum response element |
SRF | = | serum response factor |
TCF | = | ternary complex factor |
TLR3 | = | toll-like receptor 3 |
TKO | = | CBP/p300/p53 triple knockout |
TSA | = | trichostatin A |
WT | = | wild type |
Figures and Tables
Figure 1 cAMP-inducible genes display varying requirements for CBP/p300. (A–H) qRT-PCR of RNA from WT and dKO MEFs starved overnight in low serum (0.1%) medium and treated for 90 min with 10 µM forskolin/0.1 mM IBMX (FI) or ethanol vehicle (EtOH). Normalized to expression of Pgk1 mRNA. N = 2–4; mean ± SEM.
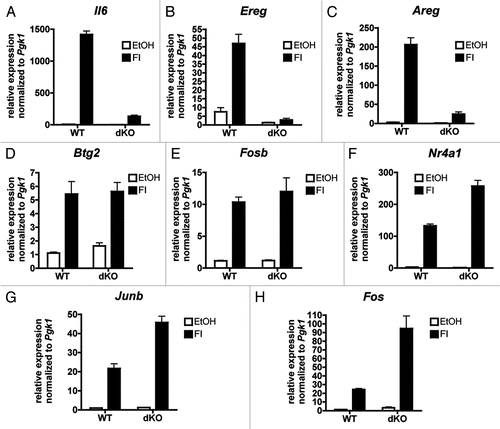
Figure 2 p53 target genes do not require CBP/p300 for expression. (A) Western blot for p53 in WT and dKO MEFs treated for 0, 1 or 3 h with 100 µm etoposide. A non-specific band serves as a loading control. (B and C) qRT-PCR for Cdkn1a and Mdm2 in RNA from WT and dKO MEFs treated for 3 h with 100 µm etoposide or DMSO vehicle (Mock). Normalized to expression of Gapdh mRNA. N = 3; mean ± SEM. (D) Western blot for p53 and Actin (loading control) in WT, dKO and TKO MEFs treated for 0 or 4 h with 0.2 µg/ml doxorubicin. (E and F) qRT-PCR for Cdkn1a and Mdm2 in RNA from WT, dKO and TKO MEFs treated for 4 h with 0.2 µg/ml doxorubicin or DMSO vehicle (Mock). Normalized to expression of Pgk1 mRNA. N = 2–5; mean ± SEM.
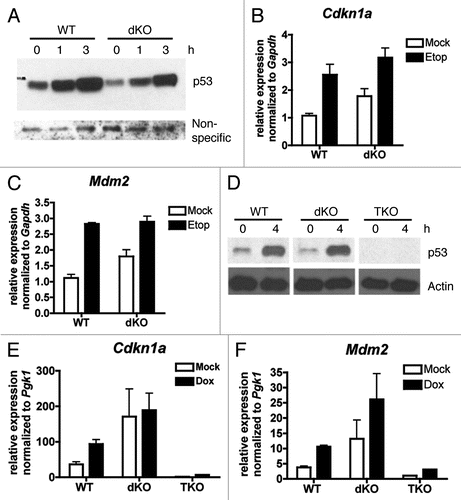
Figure 3 Not all dsRNA-induced genes require CBP/p300 for their expression. (A–D) qRT-PCR of RNA from WT and dKO MEFs treated for 1 h with 100 µg/ml Poly I:Poly C and 500 µg/ml DEAE dextran (dsRNA) or PBS (Mock), then washed and incubated in fresh medium for 3 h. For Ifnb1, RNA was DNase treated before reverse transcriptase reaction. Normalized to expression of Pgk1 mRNA. N = 2–3; mean ± SEM; p values from two-tailed unpaired t-test of dsRNA treated data points.
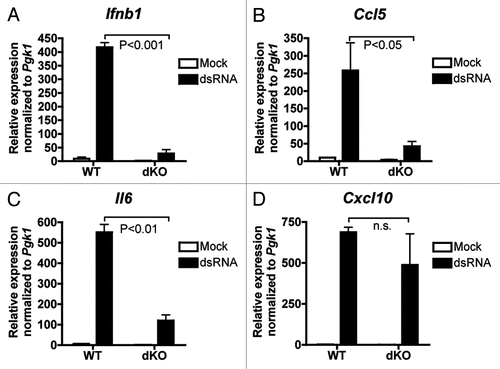
Figure 4 CBP/p300 are recruited to dsRNA-responsive gene promoters. (A–D) qPCR of chromatin from control (normal rabbit serum, NRS) or anti-CBP/p300 ChIP of WT and dKO MEFs treated for 1 h with 100 µg/ml Poly I:Poly C and 500 µg/ml DEAE dextran (dsRNA) or PBS (Mock). Primers were designed at the enhanceosome binding site (∼80 bp 5′ of TSS) in the Ifnb1 promoter and near the NFκB sites in the promoters of Ccl5, Il6 and Cxcl10. Relative ChIP signal normalized to signal from chromatin input. N = 1.
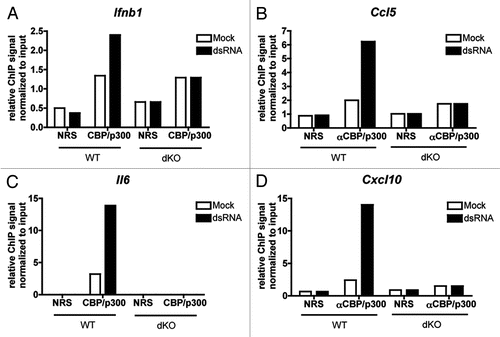
Figure 5 Loss of CBP/p300 produces different effects on different serum-inducible genes. (A–L) qRT-PCR of RNA from WT and dKO MEFs starved overnight in low serum (0.1%) medium and treated for 1 h with 10% serum or PBS (Mock). Normalized to expression of Pgk1 mRNA. N = 3; mean ± SEM.
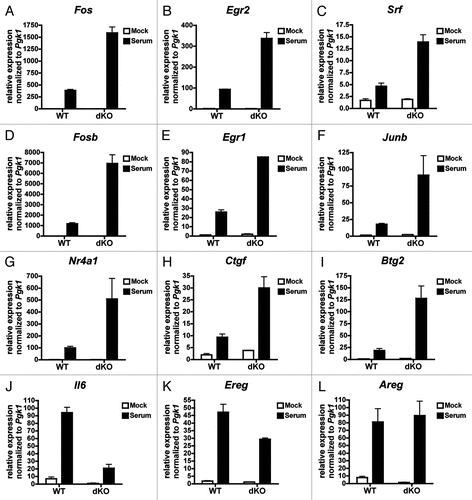
Figure 6 Serum responsive genes that do not require CBP/p300 for their expression still recruit CBP/p300. (A and B) qPCR of chromatin from control (normal rabbit serum, NRS) or anti-CBP/p300 ChIP of WT MEFs starved overnight in low serum (0.1%) medium and treated for 30 min with 10% serum or PBS (Mock). Primers designed near SREs in the indicated promoters. Relative ChIP signal normalized to signal from chromatin input. N = 1.
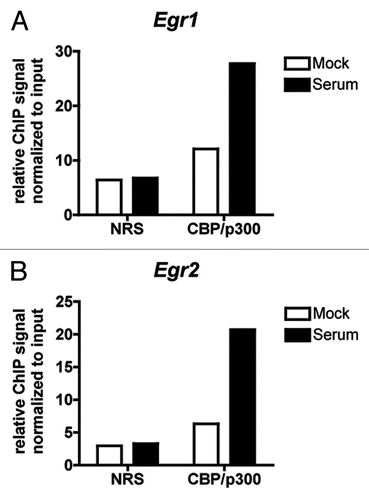
Figure 7 Expression of retinoic acid-responsive genes is CBP/p300 dependent. (A–F) qRT-PCR of RNA from WT and dKO MEFs treated for 4 h with 1 µM retinoic acid (RA) or DMSO vehicle (Mock). Normalized to expression of Pgk1 mRNA. N = 2–4; mean ± SEM.
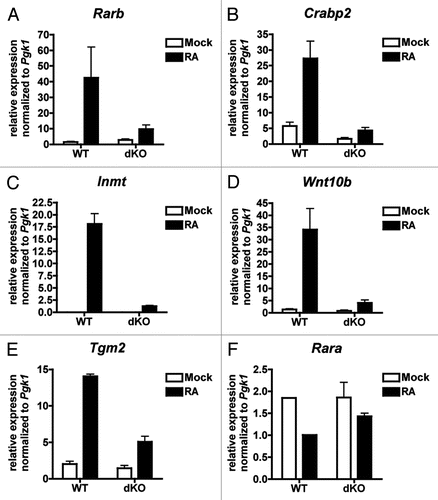
Figure 8 CBP/p300 are recruited to RAREs in response to retinoic acid. (A and B) qPCR of chromatin from control (normal rabbit serum, NRS) or anti-CBP/p300 ChIP of WT and dKO MEFs treated for 1 h with 1 µM retinoic acid (RA) or DMSO vehicle (Mock). Primers designed near RAREs in the indicated promoters. Relative ChIP signal normalized to signal from chromatin input. N = 1.
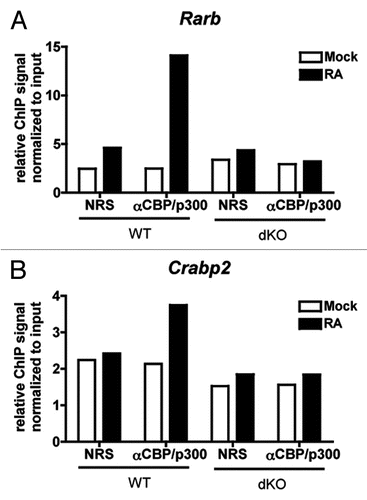
Acknowledgements
We thank Stephanie Lerach and Trushar Jeevan for excellent technical assistance. Thanks to the Vector Development and Production Core at SJCRH. The Hartwell Center at SJCRH provided oligonucleotides. This work was supported by NIH grant DE018183 (P.B.), Cancer Center (CORE) support grant P30 CA021765, and the American Lebanese Syrian Associated Charities of St. Jude Children's Research Hospital.
References
- Vo NK, Goodman RH. CBP and p300 in Transcriptional Regulation. J Biol Chem 2001; 276:13505 - 13508
- Kalkhoven E. CBP and p300: HATs for different occasions. Biochem Pharmacol 2004; 68:1145 - 1155
- Dekker FJ, Haisma HJ. Histone acetyl transferases as emerging drug targets. Drug Discov Today 2009; 14:942 - 948
- Bedford DC, Kasper LH, Fukuyama T, Brindle PK. Target gene context influences the transcriptional requirement for the KAT3 family of CBP and p300 histone acetyltransferases. Epigenetics 2010; 5:9 - 15
- Visel A, Blow MJ, Li Z, Zhang T, Akiyama JA, Holt A, et al. ChIP-seq accurately predicts tissue-specific activity of enhancers. Nature 2009; 457:854 - 858
- Kim TK, Hemberg M, Gray JM, Costa AM, Bear DM, Wu J, et al. Widespread transcription at neuronal activity-regulated enhancers. Nature 2010; 465:182 - 187
- Allis CD, Berger SL, Cote J, Dent S, Jenuwien T, Kouzarides T, et al. New nomenclature for chromatin-modifying enzymes. Cell 2007; 131:633 - 636
- Marmorstein R. Structure of histone acetyltransferases. J Mol Biol 2001; 311:433 - 444
- Kasper LH, Lerach S, Wang J, Wu S, Jeevan T, Brindle PK. CBP/p300 double null cells reveal effect of coactivator level and diversity on CREB transactivation. EMBO J 2010; 29:3660 - 3672
- Yao TP, Oh SP, Fuchs M, Zhou ND, Ch'ng LE, Newsome D, et al. Gene dosage-dependent embryonic development and proliferation defects in mice lacking the transcriptional integrator p300. Cell 1998; 93:361 - 372
- Oike Y, Takakura N, Hata A, Kaname T, Akizuki M, Yamaguchi Y, et al. Mice homozygous for a truncated form of CREB-binding protein exhibit defects in hematopoiesis and vasculo-angiogenesis. Blood 1999; 93:2771 - 2779
- Kung AL, Rebel VI, Bronson RT, Ch'ng LE, Sieff CA, Livingston DM, et al. Gene dose-dependent control of hematopoiesis and hematologic tumor suppression by CBP. Genes Dev 2000; 14:272 - 277
- Xu W, Fukuyama T, Ney PA, Wang D, Rehg J, Boyd K, et al. Global transcriptional coactivators CREB-binding protein and p300 are highly essential collectively but not individually in peripheral B cells. Blood 2006; 107:4407 - 4416
- Kasper LH, Fukuyama T, Biesen MA, Boussouar F, Tong C, de Pauw A, et al. Conditional knockout mice reveal distinct functions for the global transcriptional coactivators CBP and p300 in T-cell development. Mol Cell Biol 2006; 26:789 - 809
- Xu W, Kasper LH, Lerach S, Jeevan T, Brindle PK. Individual CREB-target genes dictate usage of distinct cAMP-responsive coactivation mechanisms. EMBO J 2007; 26:2890 - 2903
- Brivanlou AH, Darnell JE Jr. Signal transduction and the control of gene expression. Science 2002; 295:813 - 818
- Vousden KH, Prives C. Blinded by the Light: The Growing Complexity of p53. Cell 2009; 137:413 - 431
- Gu W, Roeder RG. Activation of p53 sequence-specific DNA binding by acetylation of the p53 C-terminal domain. Cell 1997; 90:595 - 606
- Gu W, Shi XL, Roeder RG. Synergistic activation of transcription by CBP and p53. Nature 1997; 387:819 - 823
- Teufel DP, Freund SM, Bycroft M, Fersht AR. Four domains of p300 each bind tightly to a sequence spanning both transactivation subdomains of p53. Proc Natl Acad Sci USA 2007; 104:7009 - 7014
- Ferreon JC, Lee CW, Arai M, Martinez-Yamout MA, Dyson HJ, Wright PE. Cooperative regulation of p53 by modulation of ternary complex formation with CBP/p300 and HDM2. Proc Natl Acad Sci USA 2009; 106:6591 - 6596
- Lee CW, Ferreon JC, Ferreon AC, Arai M, Wright PE. Graded enhancement of p53 binding to CREB-binding protein (CBP) by multisite phosphorylation. Proc Natl Acad Sci USA 2010; 107:19290 - 19295
- Lee CW, Martinez-Yamout MA, Dyson HJ, Wright PE. Structure of the p53 transactivation domain in complex with the nuclear receptor coactivator binding domain of CREB binding protein. Biochemistry 2010; 49:9964 - 9971
- Coutts AS, La Thangue NB. The p53 response: emerging levels of co-factor complexity. Biochem Biophys Res Commun 2005; 331:778 - 785
- Grossman SR, Perez M, Kung AL, Joseph M, Mansur C, Xiao ZX, et al. p300/MDM2 complexes participate in MDM2-mediated p53 degradation. Molecular Cell 1998; 2:405 - 415
- Shi D, Pop MS, Kulikov R, Love IM, Kung AL, Grossman SR. CBP and p300 are cytoplasmic E4 polyubiquitin ligases for p53. Proc Natl Acad Sci USA 2009; 106:16275 - 16280
- Sykes SM, Mellert HS, Holbert MA, Li K, Marmorstein R, Lane WS, et al. Acetylation of the p53 DNA-binding domain regulates apoptosis induction. Mol Cell 2006; 24:841 - 851
- Natoli G, De Santa F. Shaping alternative NFkappaB-dependent gene expression programs: new clues to specificity. Cell Death Differ 2006; 13:693 - 696
- Sen GC, Sarkar SN. Transcriptional signaling by double-stranded RNA: role of TLR3. Cytokine Growth Factor Rev 2005; 16:1 - 14
- Panne D, Maniatis T, Harrison SC. An atomic model of the interferon-beta enhanceosome. Cell 2007; 129:1111 - 1123
- Kim TK, Kim TH, Maniatis T. Efficient recruitment of TFIIB and CBP-RNA polymerase II holoenzyme by an interferon-beta enhanceosome in vitro. Proc Natl Acad Sci USA 1998; 95:12191 - 12196
- Merika M, Williams AJ, Chen G, Collins T, Thanos D. Recruitment of CBP/p300 by the IFNbeta enhanceosome is required for synergistic activation of transcription. Mol Cell 1998; 1:277 - 287
- Ford E, Thanos D. The transcriptional code of human IFNbeta gene expression. Biochim Biophys Acta 2010; 1799:328 - 336
- Libermann TA, Baltimore D. Activation of interleukin-6 gene expression through the NFkappaB transcription factor. Mol Cell Biol 1990; 10:2327 - 2334
- Sakaguchi S, Negishi H, Asagiri M, Nakajima C, Mizutani T, Takaoka A, et al. Essential role of IRF-3 in lipopolysaccharide-induced interferon-beta gene expression and endotoxin shock. Biochem Biophys Res Commun 2003; 306:860 - 866
- Takaoka A, Yanai H, Kondo S, Duncan G, Negishi H, Mizutani T, et al. Integral role of IRF-5 in the gene induction programme activated by Toll-like receptors. Nature 2005; 434:243 - 249
- Covic M, Hassa PO, Saccani S, Buerki C, Meier NI, Lombardi C, et al. Arginine methyltransferase CARM1 is a promoter-specific regulator of NFkappaB-dependent gene expression. EMBO J 2005; 24:85 - 96
- Arsenian S, Weinhold B, Oelgeschlager M, Ruther U, Nordheim A. Serum response factor is essential for mesoderm formation during mouse embryogenesis. EMBO J 1998; 17:6289 - 6299
- Treisman R. The serum response element. Trends Biochem Sci 1992; 17:423 - 426
- Dalton S, Treisman R. Characterization of SAP-1, a protein recruited by serum response factor to the c-fos serum response element. Cell 1992; 68:597 - 612
- Selvaraj A, Prywes R. Expression profiling of serum inducible genes identifies a subset of SRF target genes that are MKL dependent. BMC Mol Biol 2004; 5:13
- Lee SM, Vasishtha M, Prywes R. Activation and repression of cellular immediate early genes by serum response factor cofactors. J Biol Chem 2010; 285:22036 - 22049
- Posern G, Treisman R. Actin' together: serum response factor, its cofactors and the link to signal transduction. Trends Cell Biol 2006; 16:588 - 596
- Miralles F, Posern G, Zaromytidou AI, Treisman R. Actin dynamics control SRF activity by regulation of its coactivator MAL. Cell 2003; 113:329 - 342
- Gineitis D, Treisman R. Differential usage of signal transduction pathways defines two types of serum response factor target gene. J Biol Chem 2001; 276:24531 - 24539
- Ramirez S, Ait-Si-Ali S, Robin P, Trouche D, Harel-Bellan A, Ait S, Ali S. The CREB-binding protein (CBP) cooperates with the serum response factor for transactivation of the c-fos serum response element. J Biol Chem 1997; 272:31016 - 31021
- Janknecht R, Nordheim A. Regulation of the c-fos promoter by the ternary complex factor Sap-1a and its coactivator CBP. Oncogene 1996; 12:1961 - 1969
- Hanna M, Liu H, Amir J, Sun Y, Morris SW, Siddiqui MA, et al. Mechanical regulation of the proangiogenic factor CCN1/CYR61 gene requires the combined activities of MRTF-A and CREB-binding protein histone acetyltransferase. J Biol Chem 2009; 284:23125 - 23136
- Stevens JL, Cantin GT, Wang G, Shevchenko A, Berk AJ. Transcription control by E1A and MAP kinase pathway via Sur2 mediator subunit. Science 2002; 296:755 - 758
- Ravnskjaer K, Kester H, Liu Y, Zhang X, Lee D, Yates JR 3rd, et al. Cooperative interactions between CBP and TORC2 confer selectivity to CREB target gene expression. EMBO J 2007; 26:2880 - 2889
- Mark M, Ghyselinck NB, Chambon P. Function of retinoic acid receptors during embryonic development. Nucl Recept Signal 2009; 7:2
- Chambon P. A decade of molecular biology of retinoic acid receptors. FASEB J 1996; 10:940 - 954
- Chakravarti D, LaMorte VJ, Nelson MC, Nakajima T, Schulman IG, Juguilon H, et al. Role of CBP/P300 in nuclear receptor signalling. Nature 1996; 383:99 - 103
- Kamei Y, Xu L, Heinzel T, Torchia J, Kurokawa R, Gloss B, et al. A CBP integrator complex mediates transcriptional activation and AP-1 inhibition by nuclear receptors. Cell 1996; 85:403 - 414
- Balmer JE, Blomhoff R. Gene expression regulation by retinoic acid. J Lipid Res 2002; 43:1773 - 1808
- Delacroix L, Moutier E, Altobelli G, Legras S, Poch O, Choukrallah MA, et al. Cell-specific interaction of retinoic acid receptors with target genes in mouse embryonic fibroblasts and embryonic stem cells. Mol Cell Biol 2010; 30:231 - 244
- Jin Q, Yu LR, Wang L, Zhang Z, Kasper LH, Lee JE, et al. Distinct roles of GCN5/PCAF-mediated H3K9ac and CBP/p300-mediated H3K18/27ac in nuclear receptor transactivation. EMBO J 2010;
- Koutroubas G, Merika M, Thanos D. Bypassing the requirements for epigenetic modifications in gene transcription by increasing enhancer strength. Mol Cell Biol 2008; 28:926 - 938