Abstract
In the presence of double-stranded DNA breaks (DSBs), the activation of ATR is achieved by the ability of ATM to phosphorylate TopBP1 on serine 1131, which leads to an enhancement of the interaction between ATR and TopBP1. In Xenopus egg extracts, the Mre11-Rad50-Nbs1 (MRN) complex is additionally required to bridge ATM and TopBP1 together. In this report, we show that CtIP, which is recruited to DSB-containing chromatin, interacts with both TopBP1 and Nbs1 in a damage-dependent manner. An N-terminal region containing the first two BRCT repeats of TopBP1 is essential for the interaction with CtIP. Furthermore, two distinct regions in the N-terminus of CtIP participate in establishing the association between CtIP and TopBP1. The first region includes two adjacent putative ATM/ATR phosphorylation sites on serines 273 and 275. Secondly, binding is diminished when an MRN-binding region spanning residues 25–48 is deleted, indicative of a role for the MRN complex in mediating this interaction. This was further evidenced by a decrease in the interaction between CtIP and TopBP1 in Nbs1-depleted extracts and a reciprocal decrease in the binding of Nbs1 to TopBP1 in the absence of CtIP, suggestive of the formation of a complex containing CtIP, TopBP1, and the MRN complex. When CtIP is immunodepleted from egg extracts, the activation of the response to DSBs is compromised and the levels of ATR, TopBP1, and Nbs1 on damaged chromatin are reduced. Thus, CtIP interacts with TopBP1 in a damage-stimulated, MRN-dependent manner during the activation of ATR in response to DSBs.
Introduction
Eukaryotic cells have evolved a series of intricately choreographed processes to ensure that their DNA is swiftly repaired if harmed by the continuous assault of metabolic byproducts and exogenous insults.Citation1 A failure in repair is usually associated with multiple pathological disorders, including cancer.Citation2 Genomic safeguarding is achieved by the activation of checkpoints that delay cell cycle progression while damage is being repaired.Citation3,Citation4 Members of the phosphoinositide-kinase related family of protein kinases (PIKKs), ATM and ATR, function as the principal regulators of the response to damaged DNA.Citation5–Citation7 ATM specifically responds to double-stranded DNA breaks (DSBs), a very deleterious form of damage.Citation8 On the other hand, ATR is activated in response to an array of DNA lesions, including DSBs and has been largely characterized as the main apical kinase in the response to stalled replication forks.Citation7
The activation of ATR is a highly regulated process that is partly modulated by its interaction with other proteins. Among these factors is the ATR-Interacting Protein (ATRIP) that is crucial for the checkpoint regulatory functions of ATR.Citation9,Citation10 ATRIP is required to establish a critical association between ATR and the BRCA1 C-terminus (BRCT) repeat-containing protein, TopBP1, which is essential for the activation of the ATR-ATRIP kinase complex.Citation11 In Xenopus egg extracts and in human cells, the exogenous introduction of a TopBP1 fragment that interacts with the ATR-ATRIP complex, the ATR-Activating Domain (AAD), triggers an increase in the kinase activity of ATR towards a number of substrates, including Chk1, the main downstream effector kinase of the DNA replication checkpoint.Citation12 The activation of ATR-ATRIP by TopBP1 is further regulated by differentially specified interactions determined by the nature of the initial source of damage.Citation13–Citation16 In response to DSBs, the TopBP1-mediated activation of ATR is achieved through the phosphorylation of TopBP1 by ATM.Citation15,Citation17 ATM phosphorylates TopBP1 on serine 1131 (S1131), a modification that leads to the strengthening of the interaction between ATR and TopBP1 and thereby enhances the activity of ATR. The activation of ATR in response to DSBs is additionally regulated by the capacity of TopBP1 to interact with the Mre11-Rad50-Nbs1 (MRN) complex.Citation16 The MRN complex is one of the first factors to be localized to DSBs and, together with ATM, is responsible for the generation of stretches of single-stranded DNA (ssDNA) that are subsequently coated by replication protein A (RPA).Citation18,Citation19 The production of ssDNA (RPA-ssDNA) is crucial for proper localization of the ATR-ATRIP complex to sites of damage and therefore another important determinant of ATR activity.Citation10,Citation19–Citation21
Among the agents that along with ATM and the MRN complex are required for the formation of the checkpoint-stimulating RPA-ssDNA is CtIP.Citation21–Citation26 CtIP was initially identified in yeast two-hybrid screens as an interacting protein of the transcriptional co-repressor CtBP (CtBP-Interacting Protein).Citation27,Citation28 Subsequently, it has been described as an ATM substrate that is recruited to DSBs to mediate the activation of repair by homologous recombination (HR).Citation24,Citation29–Citation35 In fission yeast and in higher eukaryotes, the recruitment of Ctp1 and CtIP, respectively, to DSBs is partly mediated by the activity of the MRN complex.Citation23,Citation26,Citation36 CtIP has been shown to physically interact with all the components of the MRN complex through multiple points of contact and the absence of either CtIP or Mre11 decreases the frequency of HR repair to levels similar to those achieved by the depletion of Rad51, a critical regulator of the HR pathway.Citation23,Citation24,Citation36,Citation37 Likewise, fission yeast cells lacking Ctp1 displayed defects in HR similar to those observed in nbs1Δ and mre11Δ mutant strains. In addition, ctp1Δ strains were more sensitive to DNA-damaging agents because of inefficient formation of RPA-ssDNA adjacent to breaks.Citation23 Similar results were observed in human cells, where knockdown of CtIP by siRNA resulted in a reduction of RPA accumulation in irradiated regions and diminished activation of ATR.Citation24
The parallels exhibited by CtIP and TopBP1 in mediating the activation of ATR by regulating the formation of RPA-ssDNA in an ATM- and MRN-dependent fashion prompted us to explore the possibility of an interaction between these two checkpoint proteins. Using Xenopus egg extracts, we were successful in characterizing a damage-stimulated interaction between CtIP and TopBP1 that requires the first two BRCT repeats of TopBP1. The adjacent ATM/ATR consensus phosphorylation sites S273/S275 in CtIP are also required for this interaction and it is further stimulated by the presence of Nbs1, which also interacts with CtIP in a damage-dependent manner. The absence of CtIP in egg extracts compromises the activation of ATR in response to DSBs by reducing the level of TopBP1 and Nbs1 on damaged chromatin, suggesting that CtIP collaborates with TopBP1 and the MRN complex to stimulate the activity of ATR in response to DSBs.
Results
CtIP associates with chromatin containing double-stranded DNA breaks (DSBs) in Xenopus egg extracts.
The Xenopus egg extract system has been a valuable resource for the molecular characterization of the pathways that mediate the response to stalled replication forks. The tools generated to study the activation of these checkpoint pathways have recently been utilized in the dissection of the response to double-stranded DNA breaks (DSBs).Citation15,Citation16,Citation26 In these studies, DSBs were generated by supplementing egg extracts with the restriction endonuclease EcoRI. Treatment of extracts with EcoRI typically results in a relatively modest activation of the downstream effector kinase of the DNA damage response pathway, Chk1, in comparison to the stronger activation caused by the DNA polymerase inhibitor aphidicolin (, compare lanes 2 and 3). To generate a more robust response to DSBs, we used the endonuclease PflMI. This enzyme, which produces non-complementary DNA ends, is known to provoke a very robust Chk1 response (, lane 4). Treatment with PflMI also caused a dramatic increase in the nuclear accumulation of RPA, while only causing a modest increase in the phosphorylation of Chk2, the downstream effector of ATM-specific responses to damage (, lane 8).
With this system, we set out to characterize the involvement of CtIP in the DSB response. Upon treatment with PflMI, a considerable decrease in the mobility of CtIP was observed (). A similarly modified form of CtIP arises in response to ionizing radiation (IR) and topoisomerase inhibitors in human cells.Citation24,Citation29 Nbs1, a member of the MRN complex involved in the early stages of DNA damage sensing and TopBP1, a crucial regulator of the activation of ATR, both displayed similar changes in mobility in response to PflMI. In extracts treated with the aphidicolin, we could observe a modest increase in the accumulation of CtIP in nuclear fractions but no discernible change in mobility. The replication initiation factor Cdc45, on the other hand, displayed significant nuclear accumulation in response to replication blocks triggered by aphidicolin but no changes upon treatment with PflMI in comparison with untreated controls.
To further characterize the behavior of CtIP in the response to DSBs, we examined its recruitment to chromatin in response to damage (). In the absence of damage or in the presence of replication blocks, CtIP is only marginally loaded onto chromatin. Conversely, we could observe a substantial accumulation of CtIP on chromatin treated with PflMI. The chromatin-bound CtIP also displayed the decreased mobility shift seen in nuclear fractions. PflMI treatment also triggered an increase in the recruitment of TopBP1, Nbs1 and the ssDNA-binding protein RPA onto damaged chromatin. Increased binding of a plethora of replication and checkpoint factors in response to damage is regulated by ATM and ATR. Inhibition of these kinases with caffeine disrupts checkpoint signaling and thus leads to a failure to activate the downstream effector kinase Chk1.Citation38 Interestingly, however, certain proteins involved in the regulation of Chk1 activation and other checkpoint functions are seen to increase dramatically on damaged chromatin in the presence of caffeine.Citation39 To assess if CtIP falls within this class of proteins, we looked at the DSB-induced chromatin binding of CtIP in the presence of caffeine (). We observed that the levels of CtIP on PflMI-treated chromatin are further increased in extracts supplemented with caffeine. In fact, treatment with caffeine alone or along with aphidicolin leads to a subtle increase of CtIP binding onto chromatin, implying a strong correlation between the checkpoint functions of ATM and ATR and the chromatin binding of CtIP. Taken together, our results indicate that a modified form of CtIP is loaded onto chromatin containing DSBs and that this recruitment is sensitive to the presence of a functional checkpoint apparatus.
CtIP interacts with TopBP1 and Nbs1 in Xenopus egg extracts.
Activation of checkpoint signaling in response to DSBs is mainly regulated by the activity of ATM. The initial activation of ATM in turn leads to an increase in ATR activity.Citation21 In egg extracts, the transition from the initiating function of ATM to the subsequent activation of ATR is in part achieved by the ATM-dependent phosphorylation of TopBP1.Citation15 TopBP1 molecules that have been phosphorylated on S1131 interact more strongly with ATR. This interaction is a crucial step in the initiation of ATR signaling in response to damage.Citation15,Citation40 In U2OS cells, CtIP has also been shown to be involved in the recruitment to sites of irradiation as well as in its activation.Citation24 The involvement of both TopBP1 and CtIP in the activation of ATR in response to DSBs prompted us to investigate if these proteins displayed any functional interactions in Xenopus egg extracts. This was indeed the case, as TopBP1 immunoprecipitates (IPs) from interphase extracts supplemented with the checkpoint-stimulating synthetic oligonucleotides dA70-dT70 (hereafter termed pA-pT) contained CtIP (). Likewise, the reciprocal interaction was observed, as TopBP1 was present in CtIP immunoprecipitates from extracts containing pA-pT (). To further validate the interaction between CtIP and TopBP1, a recombinant version of CtIP (CtIP-FLAG) was added to interphase extracts and subsequently recovered and analyzed for the presence of TopBP1. When CtIP-FLAG was recovered from egg extracts, TopBP1 was present specifically when these pull-downs were carried out in the presence a checkpoint-stimulating signal (). In addition, the damage-stimulated interactions between CtIP and TopBP1 were also noticeable in reciprocal IPs of these proteins from nuclear lysates of extracts treated with PflMI (), although the effect was more pronounced in anti-TopBP1 IPs.
Previous work from our laboratory has established that the MRN complex, specifically Nbs1, is a critical mediator of the TopBP1-dependent activation of ATR in response to DSBs.Citation16 In light of these findings, we investigated the ability of Xenopus CtIP to interact with Nbs1 in the presence of damage. To this end, Nbs1 was immunoprecipitated from egg extracts in the presence and absence of pA-pT. Indeed, CtIP was present in Nbs1 IPs from extracts that contained pA-pT ().
The BRCT I–II region of TopBP1 is necessary and sufficient for association with CtIP.
Having determined that CtIP and TopBP1 associate in a checkpoint-induced manner, we investigated which region of TopBP1 is responsible for this interaction. CtIP interacts with the breast cancer tumor suppressor BRCA1 through its BRCT domains in a highly regulated manner to modulate the response to DSBs and the activation of repair pathways.Citation29–Citation33,Citation35,Citation41,Citation42 TopBP1 contains 8 BRCT domains distributed throughout the length of the protein (). To assess the necessity of specific BRCT domains of TopBP1 in mediating the interaction with CtIP, we used a series of deletion mutants of recombinant full-length TopBP1 (HF-TopBP1) lacking one or more BRCT repeats.Citation13,Citation15 These FLAG-tagged mutants were added to extracts in the absence and presence of pA-pT oligomers and subsequently retrieved for analysis (data not shown). Using this approach, we found that the mutant version of TopBP1 lacking its first two BRCT repeats (ΔI–II) fails to interact with CtIP (). The region comprising BRCT repeats I–II is also responsible for the association of TopBP1 with the Nbs1 subunit of the MRN complex (Mre11-Rad50-Nbs1), also a key regulator of the response to DSBs.Citation16 The interaction between TopBP1 and Nbs1 is compromised when the phosphate-binding pocket within the first BRCT repeat of TopBP1 is disrupted by mutating two adjacent lysines, K154 and K155, to alanine and methionine, respectively (designated as the KKAM mutant).Citation16 To assess the effects of these mutations on the association between TopBP1 and CtIP, we incubated HF-TopBP1-KKAM in egg extracts and carried out the pull-downs described above. The KKAM version of TopBP1 displayed a decrease in binding to CtIP, as opposed to a full abrogation of the interaction as seen with Nbs1 ().
To characterize further the requirement of the BRCT I–II region for the CtIP-TopBP1 interaction, we utilized a FLAG-tagged fragment of TopBP1 that only contains the first two of the BRCT repeats (HF-BRCT I–II). This fragment strongly interacted with CtIP in a checkpoint-regulated manner (). As was the case with the full-length protein, the binding of CtIP was decreased when the KKAM mutation was introduced into HF-BRCT I–II.
The requirement of BRCT domains to mediate the interactions between TopBP1 and CtIP prompted us to explore the possibility of the binding between CtIP and Nbs1 being regulated in a similar fashion. In addition, the damage-stimulated interaction between TopBP1 and Nbs1 is mediated by tandem BRCT repeats in Nbs1.Citation16 To assess if the BRCT domains of Nbs1 are required for its interaction with CtIP, we made use of a mutant Nbs1 protein (Nbs1-2A), which fails to interact with TopBP1 in the presence of damage because of mutations introduced into its BRCT-containing region (T156 and K158 to alanine).Citation16 In the presence of damage, wild-type (WT) Nbs1-FLAG could pull-down CtIP, whereas Nbs1-2A pull-downs did not yield any detectable CtIP (), indicative of the necessity of the BRCT repeat-containing region of Nbs1 to achieve the association with CtIP.
In order to define more precisely the contribution of Nbs1 in mediating the interaction between CtIP and TopBP1, we carried out HF-TopBP1 pull-downs in extracts from which we had immunodepleted Nbs1 (). In the absence of Nbs1, the amount of CtIP that interacts with TopBP1 in response to pA-pT is reduced akin to the decreased binding observed with KKAM mutants (). Interestingly, in extracts where CtIP had been removed by immunodepletion, the degree to which Nbs1 interacts with TopBP1 in response to damage is also reduced. The compromised association between Nbs1 and TopBP1 in the absence of CtIP was also observed when recombinant Nbs1 (Nbs1-FLAG) was added to CtIP-depleted extracts ().
Taken together, these results indicate that the first two BRCT repeats of TopBP1 are required for association with CtIP. Likewise, the interaction between Nbs1 and CtIP requires the tandem BRCT repeats of Nbs1. Additionally, Nbs1 and CtIP reciprocally mediate their association with TopBP1 in response to damage, which suggests the existence of a larger complex that would minimally be composed of CtIP, TopBP1 and presumably the whole MRN heteromultimer. It should be noted that we do not know at this time whether binding between TopBP1 and CtIP and between TopBP1 and Nbs1 is direct. Thus, additional proteins could help to mediate these interactions.
Two distinct regions in the N-terminus of CtIP mediate association with TopBP1.
We next performed a structure-function analysis of CtIP to determine the region within CtIP necessary for interacting with TopBP1. For these studies, we generated GST fusions containing N-terminal (residues 1–349) and C-terminal (residues 304–856) regions from CtIP. The GST-CtIP fragments were incubated in egg extracts containing HF-TopBP1. Upon retrieval of HF-TopBP1 with anti-FLAG antibody beads, we could readily observe that in the presence of pA-pT, the N-terminal, but not the C-terminal, fragment of CtIP could bind efficiently to beads containing HF-TopBP1 (). While this manuscript was in preparation, You and colleaguesCitation26 characterized a region of Xenopus CtIP spanning residues 479–584, termed the damage-recruitment (DR) motif, which is sufficient for recruitment to EcoRI-treated chromatin in egg extracts. Based on our studies, this region appears not to be required for the association of CtIP with TopBP1.
Next, to more precisely define the region required for this interaction, we tested the capacity of smaller N-terminal fragments to bind TopBP1 (Sup. Fig. 1A). Among the generated fragments, the one containing residues 1–359 maintains the capacity to robustly bind TopBP1, whereas the 1–298 fragment is not as efficient in binding while still displaying the large damage-dependent decrease in mobility characteristic of the other binding competent peptides. Moreover, binding is further compromised but not completely abolished in a smaller 1–247 fragment. Notably, this fragment also fails to shift in response to pA-pT. These studies loosely define a region between residues 247–359 that is modified in response to pA-pT and is involved in the damage-dependent interaction between CtIP and TopBP1, with an additional contribution from another region that further strengthens the interaction.
The first two BRCT repeats of TopBP1 are required for the interaction with CtIP (). Previous work has demonstrated that tandem BRCT repeats form a phosphopeptide-binding pocket to mediate their interactions.Citation43,Citation44 Therefore, we looked for potential phosphorylation sites within amino acids 247–359 of CtIP that might be involved in the establishment of the association with TopBP1. This region contains a sequence that is homologous to the known CDK phosphorylation site (SPVF) at S327 in human CtIP (Sup. Fig. S1C). Phosphorylation of this residue by CDK mediates the functionally important interaction with BRCA1.Citation31,Citation32,Citation35 However, mutation of this critical residue to alanine did not affect the capacity of CtIP to bind TopBP1 in egg extracts (Sup. Fig. S1B). Another candidate within this region is the well-conserved ATM/ATR phosphorylation consensus sequence (S/T-Q) at serine 275 of the Xenopus protein (Sup. Fig. 1D). Because this site in the Xenopus protein lies directly downstream of another SQ site (containing S273), we mutated both serine 273 and 275 to alanine within the context of both full-length CtIP and the GST-CtIP (1–349) fragment (to create CtIP-2AQ mutant versions of these polypeptides) and then performed binding assays to measure the contribution of these sites in mediating the interaction with TopBP1 ( and C). We observed that the binding of 2AQ mutant forms of both full-length CtIP and the 1–349 fragment was reduced in comparison with their respective wild-type polypeptides (see lane 10 in both B and C of ).
The N-terminal region of human CtIP has been shown to be involved in regulating the response to DSBs by interacting with components of the MRN complex.Citation36 Correspondingly, in our experiments in Xenopus, GST-CtIP (1–349) was efficiently pulled-down by Nbs1 in the presence of damage (, bottom part). In the human CtIP protein, it is specifically residues 22–45 that mediate the interaction with the MRN complex and are required for proper localization of CtIP to sites of damage and activation of the G2/M checkpoint in response to γ-irradiation in HeLa cells.Citation36 The corresponding residues (25–48 in Xenopus), which are highly conserved among higher eukaryotes (Sup. Fig. 1E), were deleted from Xenopus CtIP to assess the extent to which this MRN binding region is involved in mediating the interaction with TopBP1. Consistent with the decrease in the strength of the CtIP-TopBP1 interaction in Nbs1-depleted extracts (), the degree of binding of Δ25–48 mutants of full-length CtIP and GST-CtIP (1–349) to TopBP1 was significantly lower than that of the corresponding wild-type forms (lane 15 in and C). Moreover, in mutant versions lacking the MRN-binding domain in combination with the mutated SQ sites (Δ25–48-2AQ), there appeared to be an additive effect, with the interaction being compromised further than for the Δ25–48 and 2AQ mutants alone (lane 20 in and C).
In the pull-down experiments using radiolabeled proteins described above, extracts were supplemented with the potent phosphatase inhibitor tautomycin to stabilize the checkpoint-stimulated phosphorylation events triggered by pA-pT.Citation38 In the absence of tautomycin, we could detect essentially no association of TopBP1 and Nbs1. On the other hand, we could still observe significant, albeit reduced, binding of CtIP to TopBP1 under these conditions (, lane 4). Utilizing this system, we examined the effect of caffeine on the Nbs1-independent interaction between TopBP1 and CtIP. We could readily observe that, in the absence of tautomycin, the interaction between CtIP and TopBP1 was abolished when the activities of ATM and ATR were inhibited by caffeine (, lane 5).
In summary, as represented in , two distinct regions within the N-terminus of CtIP are required for the association with TopBP1. The first is the region that mediates the binding to the MRN complex at residues 25–48; the second comprises a larger region from amino acids 247 to 359. This second area harbors a conserved putative ATM/ATR phosphorylation at S275, which when mutated, along with another adjacent SQ site, to a non-phosphorylatable form (2AQ), partially compromises the interaction.
CtIP mediates damage-dependent accumulation of ATR, TopBP1 and Nbs1 on chromatin in response to DSBs in Xenopus egg extracts.
CtIP is required for the recruitment of ATR to laser-induced DSBs in human cellsCitation24 and to EcoRI-treated chromatin in Xenopus egg extracts.Citation26 As the recruitment of ATR to DSBs is a function of TopBP1 activity, and in light of the interaction described above, we examined how the depletion of CtIP affected loading of TopBP1 onto chromatin in response to DSBs. To assess the effect of the absence of CtIP, we generated an antibody directed against a C-terminal region of CtIP (amino acids 628–856) and used it to successfully immunodeplete CtIP from egg extracts ().
We proceeded to determine the effect of depleting CtIP on the activation of the checkpoint response to DSBs. Towards this end, we examined the effect of PflMI treatment on the nuclear accumulation of checkpoint factors and the activation of Chk1 in CtIP-depleted extracts. There is ample evidence to support the role of CtIP in DNA end resection, which ultimately leads to the accumulation of RPA.Citation23,Citation24,Citation26 Consistent with these observations, we could note a decrease in the PflMI-induced nuclear accumulation of RPA in CtIP-depleted extracts in comparison with mock-depleted extracts (). The loss of resection and ssDNA generation likely to be occurring in the absence of CtIP also leads to compromised activation of Chk1, along with a mild decrease in the levels of ATM and ATR. Moreover, the levels of TopBP1 in nuclear fractions were dramatically reduced in the absence of CtIP. Depletion of CtIP removed a small amount of TopBP1 from interphase extracts, possibly due to their association (). However, the decrease in nuclear accumulation of TopBP1 in the absence of CtIP is much more pronounced. Moreover, in the absence of damage, the nuclear accumulation of TopBP1 in CtIP-depleted extracts is only slightly affected. Likewise, the nuclear accumulation of Nbs1 in response to DSBs is also severely compromised in the absence of CtIP ().
To probe further the effects of the absence of CtIP on the accumulation of TopBP1 and Nbs1, we isolated PflMI-treated chromatin from depleted extracts and examined the chromatin binding of TopBP1 and other checkpoint factors. Consistent with the observations in nuclear fractions, TopBP1 levels were markedly diminished, with only a minimal amount of protein associating with DSB-containing chromatin in the absence of CtIP (). Furthermore, the critical ATM phosphorylation of the ATR-Activating Domain (AAD) of TopBP1 on S1131 that is required for proper activation of ATR in response to DSBs was severely compromised. Moreover, the levels of ATR on damaged chromatin were noticeably reduced and a decrease in the chromatin accumulation of RPA was observed. Conversely, the levels of ATM, even though reduced in nuclear fractions, did not decrease substantially on chromatin in the absence of CtIP. In contrast to a previous report,Citation26 we observed that the levels of Nbs1 on chromatin were significantly reduced in CtIP-depleted extracts. The levels of Nbs1 in egg extracts, on the other hand were not reduced, discounting the possibility of co-depletion (). Taken together, our data strongly suggest that CtIP is required for the increase in nuclear accumulation and chromatin association of TopBP1 and Nbs1 in response to damage, important steps in the activation of ATR in response to DSBs.
Discussion
ATR is activated in response to a wide range of DNA structures ranging from DSBs and base adducts to the intermediates that arise upon stalling of replication forks. These observations led to the model that these different structures generate a common intermediate that triggers the activation of ATR.Citation7 Various lines of evidence have suggested that this structure contains RPA-coated single-stranded DNA (RPA-ssDNA).Citation10,Citation19–Citation21 A protein that is critical in the generation of RPA-ssDNA, particularly in response to DSBs, is CtIP.Citation23,Citation24,Citation26 Another integral component of the activation of ATR is TopBP1, which is sufficient to stimulate the in vitro kinase activity of ATR towards many of its substrates, even in the absence of DNA.Citation12,Citation45 In this report, we have explored the possibility of an interaction between CtIP and TopBP1, two critical components for ATR activation, in Xenopus egg extracts. We have found that CtIP and TopBP1 interact in a regulated manner in response to checkpoint activation by synthetic oligonucleotides (pA-pT) and DSBs generated by restriction endonuclease.
To understand the nature of this interaction, we utilized a series of TopBP1 deletion mutants lacking one or more of its BRCT domains, which have been shown to be involved in mediating damage-stimulated interactions with other checkpoint proteins.Citation13,Citation16 With this approach, we determined that the region of TopBP1 containing BRCT domains I and II is necessary and sufficient to mediate the interaction with CtIP. These same domains required for the interaction between TopBP1 and Nbs1. Further analysis revealed that a version of TopBP1 with mutations in the BRCT I–II region that abolish the interaction with Nbs1, TopBP1-KKAM, partially compromises the capacity of CtIP to bind TopBP1. Moreover, depletion of Nbs1 from egg extracts also reduced the interaction to a similar extent. It has previously been demonstrated by work from our laboratory that the presence of Nbs1 is critical for another key aspect of the function of TopBP1, namely, its ability to bridge the interaction between ATM and TopBP1. In the absence of Nbs1, TopBP1 cannot interact with ATM, which hinders the ATM-dependent activation of ATR in response to DSBs.Citation16 With our current work, we have further shown that CtIP, which also interacts with Nbs1 in a damage-stimulated manner, participates in the establishment of this interaction, as indicated by a decrease in the levels of Nbs1 in TopBP1 pull-downs from CtIP-depleted extracts.
The MRN complex mediates the recruitment of CtIP to sites of damage to resect DSBs and give rise to RPA-ssDNA.Citation23,Citation24,Citation26,Citation36 Recently, Chen and colleaguesCitation36 have characterized a region in the N-terminus of human CtIP (residues 22–45) that is critical for targeting CtIP to DNA breaks. This region is capable of interacting with the MRN complex, although it is not unique since the C-terminus also has the capacity to bind MRN components. Our structure/function analysis of Xenopus CtIP reveals that the N-terminal and not the C-terminal region, is required for the interaction with TopBP1. Furthermore, recombinant TopBP1 shows reduced but not abolished binding to mutant forms of CtIP lacking the homologous N-terminal region required for MRN binding (Δ25–48). This outcome is not surprising in light of the observations that there is significant binding of CtIP to TopBP1 in Nbs1-depleted extracts and that CtIP can still associate to some extent with the TopBP1-KKAM mutant. Overall, these results indicate that the regulated interaction between TopBP1 and CtIP in response to damage is partially but not fully mediated by the presence of the MRN complex, suggesting the formation of a larger damage-stimulated complex that would at least be composed of the MRN complex, CtIP and TopBP1.
BRCT repeats mainly interact with phosphorylated substrates.Citation31,Citation43,Citation44 Phosphorylation of CtIP on serine 327 (S327) of the human protein mediates the formation of a CtIP-BRCA1 complex that is involved in regulating the delay in cell cycle progression and Chk1 activation in irradiated HeLa cells.Citation31 Subsequent work in avian DT40 cells further highlights the importance of this phosphorylation event, where complementation of CtIP null cells with CtIP-S327A leads to a decrease in the generation of ssDNA and defects in homologous recombination (HR).Citation35 We explored the possibility of the corresponding Xenopus site being required for the interaction with TopBP1. Mutation of serine 328 to a non-phosphorylatable alanine (S328A) had no effect on the interaction. This result is consistent with the notion that even though the phosphorylation of S327 in the human and chicken CtIP is required for checkpoint activation, this phosphorylation event is not induced by DNA damage.Citation31 By contrast, as we have shown here, the TopBP1-CtIP interaction is strongly stimulated the presence of damage.
To further explore the events that are required for the interaction between TopBP1 and CtIP, we looked at other potential phosphorylation sites within the region that was determined to be involved in the interaction (residues 247–359). Since the interaction occurs in response to damage, we turned our attention to potential ATM/ATR sites. A couple of S/T-Q sites in CtIP have been demonstrated to be phosphorylation sites for ATM.Citation29 Both of these sites lie in the C-terminal region of CtIP, which is not required for the TopBP1 interaction. Sequence alignments with other vertebrate homologues pointed towards two directly adjacent ATM/ATR consensus SQ sites within the 247–359 region, the second of which, S275, is highly conserved. When both of these serines were mutated to alanine within full-length CtIP, the binding of the resulting 2AQ mutant to TopBP1 was lower relative to the wild-type protein. Binding was further reduced when these mutations were combined with a deletion (Δ25–48) that affects binding to MRN. In addition, under conditions where TopBP1 does not interact with Nbs1, the interaction between TopBP1 and CtIP is sensitive to caffeine. When viewed together, our results present a picture where the presence of the MRN complex and the checkpoint-stimulated activity of PIKKs comprise two separate modes by which the interaction between CtIP and TopBP1 is regulated.
While this manuscript was in preparation, You and colleaguesCitation26 published an article characterizing Xenopus CtIP. Consistent with their results, we observed that a highly modified form of CtIP binds to chromatin containing DSBs in egg extracts. In addition, in the presence of replication blocks, even though nuclear accumulation of CtIP is evident, its electrophoretic mobility is not significantly affected and it does not bind well to chromatin. Interestingly, we observed that when caffeine was added to inhibit the activity of ATM and ATR, the levels of CtIP on chromatin increased even in the absence of damage or replication blockages. Furthermore, a massive accumulation was observed when caffeine was added in combination with PflMI, mirroring the behavior displayed by other checkpoint factors, including TopBP1 and RPA. These data strongly suggest that CtIP plays a key role in the response to DSBs in egg extracts.
Consistent with what has been found in mammalian cells,Citation24 we have observed that activation of Chk1 in egg extracts in response to DSBs is defective in the absence of CtIP. Also, as You et al.Citation26 demonstrated with EcoRI treatment, we found that the nuclear and chromatin levels of RPA substantially decreased in response to PflMI in CtIP-depleted extracts. Likewise, the levels of ATR but not ATM on chromatin are reduced in the absence of CtIP. These observations are concordant with the model presented by Shiotani and ZouCitation21 of the involvement of CtIP in the biphasic response to DSBs. Using human cell extracts containing defined DNA structures, they could observe ATM activation in the presence of structures with double-stranded ends and short single-stranded overhangs (SSOs). As the length of the overhangs increases or when linearized plasmids incubated with nucleases are used as templates, the activity of ATM is reduced whereas the activity of ATR increases. In HeLa cells treated with ionizing radiation (IR), this ATM to ATR switch, gauged by Chk1 and Chk2 phosphorylation, was enhanced by the overexpression of CtIP and Exo1, an alternative nuclease with known roles in DSB resection.Citation46–Citation48 Under these conditions of overexpression of CtIP and Exo1, the inactivation of ATM and activation of ATR, respectively, occurred at earlier time points, suggesting that the switch from ATM to ATR signaling is stimulated by the progressive resection of ends by nucleolytic activities.
Our work adds a key mechanistic insight into the impact of the lack of resection by CtIP in the transition from ATM to ATR signaling. In the absence of CtIP, the amounts of TopBP1 in the nucleus and on chromatin are drastically reduced. Concomitantly, phosphorylation of S1131 of TopBP1 by ATM is abrogated. Furthermore, the nuclear and chromatin levels of Nbs1 were significantly reduced in the absence of CtIP. This observation differs from what was reported by You and colleagues.Citation26 The reason for the discrepancy is not clear, but one difference between the experiments is that PflMI triggers a stronger checkpoint response than EcoRI and thus the nuclear and chromatin accumulation of checkpoint proteins is greater after treatment with PflMI (see ). Overall, our results suggest that CtIP plays a key role in the recruitment of TopBP1 to chromatin and the ensuing, activating phosphorylation of TopBP1 on S1131. This sequence of events appears to be critical for the activation of ATR in response to DSBs.
In summary, we have described a damage-stimulated association between CtIP and TopBP1 that requires S273/S275 of CtIP and is further enhanced by presence of the MRN complex. In the absence of CtIP, the recruitment of both TopBP1 and Nbs1 to damaged chromatin is reduced thereby compromising the checkpoint response to DSBs. These observations strongly suggest that CtIP, TopBP1 and the MRN complex collaborate to trigger the transition from the initial ATM response to the subsequent ATR activity in response to DSBs.
Materials and Methods
Preparation of egg extracts.
Xenopus egg extracts were prepared as described in reference Citation49. To elicit a checkpoint response, extracts were treated with 50 µg/ml of annealed oligonucleotides poly(dA)70-poly (dT)70 (pA-pT) in the presence of 3 µM tautomycin as described in reference Citation38. To prepare extracts containing chromatin, extracts were supplemented with 3,000 demembranated Xenopus sperm nuclei/µl. Replication blocks were induced by the addition of aphidicolin at 50 µg/ml. Double-stranded DNA breaks were created by the addition of either the restriction endonuclease PflMI (final concentration of 0.1 U/µl) or EcoRI (0.05 U/µl). When noted, caffeine was added at a final concentration of 5 mM. NuclearCitation50 and chromatin fractionsCitation51 were isolated according to previously described methods. For isolation of fractions from extracts containing caffeine, all buffers were supplemented with 5 mM caffeine.
Production of recombinant proteins.
A cDNA clone encoding a full-length version of Xenopus CtIP was isolated from a Xenopus oocyte library by PCR based on an expressed sequence tag with significant homology to human CtIP. A PCR-generated DNA fragment encoding Xenopus CtIP was cloned into a pFast-Bac-FLAG vector to express proteins with a FLAG tag at the C-terminal end. Recombinant baculoviruses were generated with the Bac-to-Bac system (Invitrogen). Glutathione S-transferase (GST) fusion proteins containing fragments of CtIP (amino acids 1–349 and 304–856) were produced in Escherichia coli BL21 CodonPlus RIL cells and purified as described in reference Citation12. Recombinant, full-length HF-TopBP1 with both hemagglutinin and His6 tags at the N-terminal end and a FLAG tag at the C-terminal end was produced in baculovirus-infected Sf9 cells. Full-length wild-type (WT), ΔI–II and BRCT I–II versions of TopBP1 were generated as described in reference 16. Point mutations were produced using the QuikChange Kit (Stratagene).
Antibodies.
A DNA fragment encoding amino acids 628–856 of Xenopus CtIP was generated by PCR and cloned into a pET-His6 expression vector. The His6-CtIP(628–856) protein was expressed in Escherichia coli, isolated with nickel agarose, and used for production of rabbit antibodies at a commercial facility. Affinity-purified antibodies against Xenopus versions of ATM, ATR, TopBP1, Nbs1, RPA70, Cdc45 and Orc2 were described previously in references Citation12, Citation16, Citation39 and Citation52. Anti-human Mcm2 (BM28), anti-FLAG and control rabbit antibodies (IgG fraction) were purchased from Cell Signaling Technology, Sigma and Zymed Laboratories, respectively.
Production of 35S-labeled proteins.
35S-labeled proteins were synthesized in vitro using the TnT System (Promega). For production of 35S-labeled full-length (amino acids 1–856) and truncated forms of CtIP (amino acids 1–430, 1–359, 1–298 1–247 and 415–856), PCR-generated DNA fragments encoding these regions were cloned into pBluescript and the resulting plasmids were used as templates in the TnT system. Mutant versions of the proteins were produced using QuikChange Kit (Stratagene). 35S-Chk1 was generated as described in reference Citation40.
Pull-downs of recombinant TopBP1 and Nbs1 from Xenopus egg extracts.
Recombinant TopBP1 (WT, KKAM, ΔI–II, BRCT I–II and BRCT I–II-KKAM) (0.5 µg) and Nbs1 (1 µg) bound to anti-FLAG antibody beads were incubated in egg extracts (100 µl) containing 100 µg/ml cycloheximide in the absence or presence of 50 µg/ml pA-pT. When noted, caffeine was added at a final concentration of 5 mM. The beads were isolated by centrifugation and washed three times in CHAPS buffer (10 mM HEPES-KOH, pH 7.5, 0.1% CHAPS, 150 mM NaCl, 2.5 mM EGTA, 20 mM β-glycerolphosphate) and twice with HEPES-buffered saline (HBS) (10 mM HEPES-KOH, pH 7.5, 150 mM NaCl). Bound proteins were subjected to SDS-PAGE and immunoblotting.
Immunoprecipitations.
For immunoprecipitations, interphase extracts (100 µl) were incubated under constant agitation for 45 min at 4°C with Affiprep-protein A beads (Bio-Rad) coated with anti-TopBP1 (3 µg), anti-CtIP (3 µg), anti-Nbs1 (3 µg) or control IgG antibodies (3 µg). The beads were isolated by centrifugation and washed three times in CHAPS buffer and twice with HBS. Bound proteins were subjected to SDS-PAGE and immunoblotting. For nuclear immunoprecipitates, nuclei isolated from extracts (250 µl) were subsequently lysed by the addition of half the volume of the original extract in CHAPS buffer and incubation at 4°C with rotation for 30 min. Lysates were centrifuged for 10 min at 14,000x g. An equal volume of 20 mM HEPES-KOH (pH 7.5) was added to the supernatant. Diluted lysates were incubated with protein A beads coated with anti-TopBP1 (3 µg), anti-CtIP (3 µg) or control antibodies (3 µg) under constant agitation for 45 min at 4°C. Beads were washed 3 times in CHAPS buffer and twice in HBS before addition of 2X-SDS sample buffer for SDS-PAGE and immunoblotting analysis.
Immunodepletions.
For immunodepletion of CtIP, interphase extracts (100 µl) were incubated at 4°C for 60 min with 25 µg anti-CtIP antibodies bound to 20 µl of protein A beads. Equal amounts of rabbit IgG antibodies were used as a control. After incubation, beads were recovered by gentle centrifugation and the supernatants were treated for a second round of depletion. Resulting supernatants were then used in the specified experiments. Nbs1 was immunodepleted as described in reference Citation16.
Abbreviations
DSB | = | double-stranded DNA break |
ATM | = | ataxia-telangiectasia mutated |
ATR | = | ATM and Rad3-related |
TopBP1 | = | topoisomerase IIβ-binding protein |
Nbs1 | = | nijmegen breakage syndrome 1 |
CtIP | = | CtBP interacting protein |
BRCT | = | BRCA1 C-terminus |
ssDNA | = | single-stranded DNA |
RPA | = | replication protein A |
RPA-ssDNA | = | RPA coated single-stranded DNA |
GST | = | glutathione S-transferase |
Figures and Tables
Figure 1 CtIP associates with chromatin containing DSBs. (A) Egg extracts containing 35S-Chk1 (lanes 1–4) or 35S-Chk2 (lanes 5–8) and demembranated sperm nuclei were incubated with no checkpoint inducer (lanes 1 and 5) or sperm nuclei treated with 50 µg/ml aphidicolin (lanes 2 and 6), 0.05 U/µl of EcoRI (lanes 3 and 7) or 0.1 U/µl of PflMI (lanes 4 and 8). Nuclear fractions were isolated and analyzed by phosphorimaging and immunoblotting with anti-RPA70 antibodies. Unshifted and shifted forms of Chk1 are indicated with a dash and arrow, respectively. (B) Egg extracts containing sperm nuclei were incubated without checkpoint inducer (lane 1), 50 µg/ml aphidicolin (lane 2) or 0.1 U/µl PflMI (lane 3). Nuclear fractions were subjected to SDS-PAGE and immunoblotted with anti-TopBP1, anti-CtIP, anti-Nbs1, anti-Cdc45 and anti-Mcm2 antibodies. (C) Egg extracts lacking (lane 2) or containing sperm nuclei (lanes 3–5) were incubated without checkpoint inducer (lane 3), 50 µg/ml aphidicolin (lane 4) or 0.1 U/µl PflMI (lane 5). Chromatin fractions were subjected to SDS-PAGE and immunoblotted with anti-TopBP1, anti-CtIP, anti-Nbs1, anti-RPA70 and anti-Mcm2 antibodies. One microliter of extract was loaded in lane 1 as a loading control. (D) Chromatin fractions were isolated from egg extracts lacking (lane 2) or containing sperm nuclei treated as above in (C) (lanes 3–5) or in the presence of 5 mM caffeine (lanes 6–8). One microliter of extract was loaded in lane 1 as a loading control.
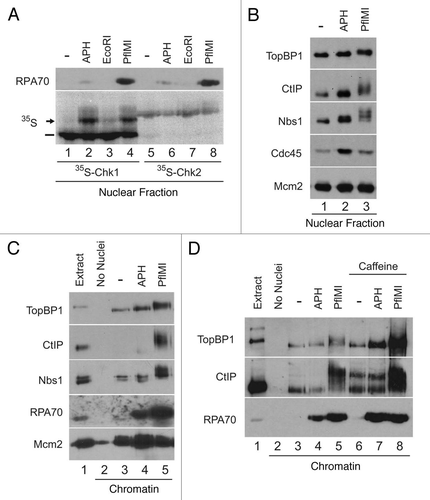
Figure 2 CtIP interacts in a regulated manner with TopBP1 and Nbs1 in Xenopus egg extracts. (A) Control IgG (lane 1) and anti-TopBP1 immunoprecipitates (IP) from interphase extracts incubated in the absence (lane 2) or presence of pA-pT (lane 3) were immunoblotted for CtIP. (B) Control IgG (lane 1) and anti-CtIP IPs from interphase extracts incubated in the absence (lane 2) or presence of pA-pT (lane 3) were immunoblotted for TopBP1. (C) Anti-FLAG antibody beads containing no recombinant protein (lanes 1 and 2) or CtIP-FLAG (lanes 3 and 4) were incubated in egg extracts in the absence or presence of pA-pT. Beads were retrieved and immunoblotted with anti-TopBP1 antibodies. (D) Control IgG (lanes 1 and 2), anti-CtIP (lanes 3 and 4) and anti-TopBP1 (lanes 5 and 6) IPs from nuclear fractions isolated from interphase extracts supplemented with demembranated sperm nuclei in the absence or presence of 0.1 U/µl of PflMI. Isolated nuclear fractions were subjected to SDS-PAGE and immunoblotted with anti-TopBP1 and anti-CtIP antibodies. (E) Control IgG (lane 1) and anti-Nbs1 IPs from interphase extracts incubated in the absence (lane 2) or presence of pA-pT (lane 3) were immunoblotted for CtIP.
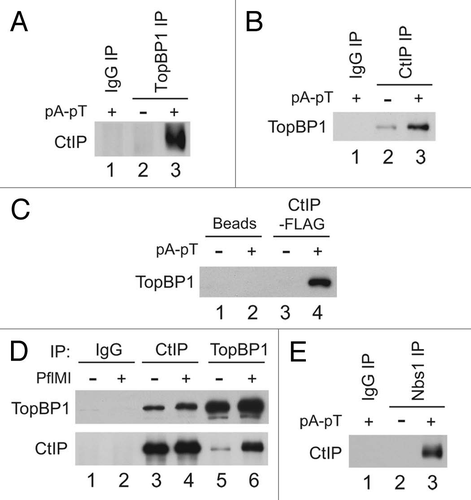
Figure 3 The BRCT I–II region of TopBP1 is necessary and sufficient for association with CtIP. (A) TopBP1 domain architecture. Roman numerals denote BRCT domains of TopBP1. Full-length HF-TopBP1 (WT) and versions of TopBP1 lacking residues 96–282 (ΔI–II) or 360–1485 (BRCT I–II) were produced in Sf9 cells. Location of residues mutated in KKAM mutants is specified in schematic. (B) Anti-FLAG antibody beads containing no recombinant protein (lanes 1 and 2) or the indicated versions of HF-TopBP1 (lanes 3–8) were incubated in egg extracts in the absence or presence of pA-pT. Beads were retrieved and immunoblotted with anti-CtIP, anti-Nbs1 and anti-FLAG antibodies. (C) Anti-FLAG antibody beads containing no recombinant protein (lanes 1 and 2) or wild-type (lanes 3 and 4) and KKAM (lanes 5 and 6) versions of HF-TopBP1-BRCT I–II were incubated in egg extracts in the absence or presence of pA-pT. Beads were retrieved and immunoblotted with anti-CtIP, anti-Nbs1 and anti-FLAG antibodies. (D) Anti-FLAG antibody beads containing no recombinant protein (lanes 1 and 2) or wild-type (lanes 3 and 4) and 2A (lanes 5 and 6) versions of Nbs1-FLAG were incubated in egg extracts in the absence or presence of pA-pT. Beads were retrieved and immunoblotted with anti-CtIP antibodies. (E) Left Part: Representative samples of extracts that were left undepleted (lane 1), mock-depleted with control IgG antibodies (lane 2), depleted with anti-CtIP antibodies (lane 3) or depleted with anti-Nbs1 antibodies (lane 4). Extracts were immunoblotted with anti-CtIP, anti-Nbs1 and anti-RPA70 antibodies. Right Part: Anti-FLAG antibody beads containing no recombinant protein (lanes 1 and 2) or wild-type HF-TopBP1 (lanes 3–8) were incubated in the absence or presence of pA-pT in egg extracts that were either mock-depleted with control IgG antibodies (lanes 3 and 4), depleted with anti-CtIP antibodies (lanes 5 and 6) or depleted with anti-Nbs1 antibodies (lanes 7 and 8). Beads were retrieved and immunoblotted with anti-CtIP, anti-Nbs1 and anti-FLAG antibodies. In the CtIP immunoblot, the band in lanes 3, 5 and 7 represents a cross-reacting band, not CtIP signal, as it is present even in CtIP-depleted extracts. (F) Left Part: Representative samples of extracts that were mock-depleted with control IgG antibodies (lane 1) or depleted with anti-CtIP antibodies (lane 2). Extracts were immunoblotted with anti-CtIP antibodies. Right Part: Anti-FLAG antibody beads containing Nbs1-FLAG were incubated in the absence or presence of pA-pT in egg extracts that were either mock-depleted with control IgG antibodies (lanes 1 and 2) or depleted with anti-CtIP antibodies (lanes 3 and 4). Beads were retrieved and immunoblotted with anti-TopBP1 and anti-CtIP antibodies.
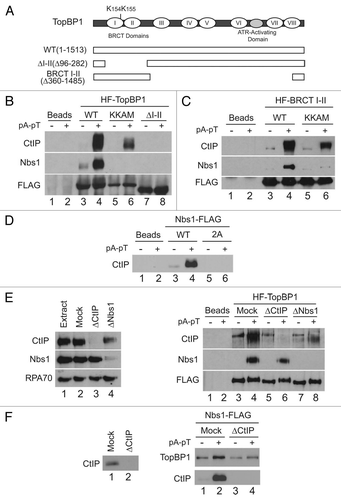
Figure 4 Two distinct regions in the N-terminus of CtIP mediate association with TopBP1. (A) Anti-FLAG antibody beads containing HF-TopBP1 or Nbs1-FLAG were incubated with GST-CtIP (1–349) (lanes 1 and 2) or GST-CtIP(304–856) (lanes 3 and 4) in the absence (lanes 1 and 3) or presence of pA-pT (lanes 2 and 4). Beads were isolated and immunoblotted with anti-GST antibodies. The top part shows in the inputs for the GST fragments. The middle and bottom parts show the TopBP1 and Nbs1 pull-downs, respectively. (B) Anti-FLAG antibody beads containing no recombinant protein (lanes 3, 8, 13 and 18) or wild-type HF-TopBP1 (lanes 1, 2, 4–7, 9–12, 14–17, 19 and 20) were incubated in egg extracts containing full-length 35S-CtIP wild-type (WT) (lanes 1–5), 2AQ (lanes 6–10), Δ25–48 (lanes 11–15) and Δ25–48-2AQ (lanes 16–20) in the absence or presence of pA-pT. The beads were retrieved and bound 35S-labeled proteins were detected by SDS-PAGE and phosphorimaging. (C) Anti-FLAG antibody beads containing no recombinant protein (lanes 3, 8, 13 and 18) or wild-type HF-TopBP1 (lanes 1, 2, 4–7, 9–12, 14–17, 19 and 20) were incubated in egg extracts containing GST-CtIP(1–349) wild-type (WT) (lanes 1–5), 2AQ (lanes 6–10), Δ25–48 (lanes 11–15) and Δ25–48-2AQ (lanes 16–20) in the absence or presence of pA-pT. Beads were isolated and immunoblotted with anti-GST antibodies. (D) Anti-FLAG antibody beads containing no recombinant protein (lanes 1 and 2) or wild-type HF-TopBP1 (lanes 3–8) were incubated in egg extracts in the absence (lanes 3–5) or presence (lanes 6–8) of tautomycin. Checkpoint activation was induced by the addition of pA-pT (lanes 4, 5, 7 and 8) and PIKK activity was inhibited by supplementing extracts with 5 mM caffeine (lanes 5 and 8). Beads were retrieved and immunoblotted with anti-CtIP, anti-Nbs1 and anti-FLAG antibodies. One microliter of extract was loaded in lane 9 as a loading control. (E) Summary of the capacity of indicated forms of CtIP to interact with TopBP1.
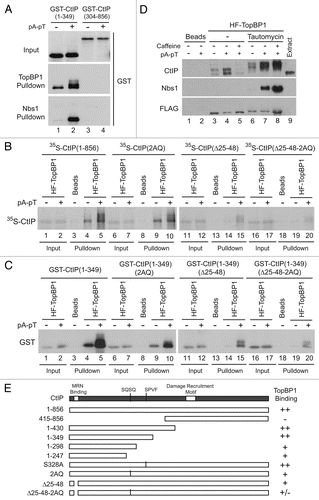
Figure 5 CtIP mediates damage-dependent nuclear accumulation of TopBP1 in response to DSBs in Xenopus egg extracts. (A) Representative samples of extracts that were either left undepleted (lane 1), mock-depleted with control IgG antibodies (lane 2) or depleted with anti-CtIP antibodies (lane 3). Extracts were immunoblotted with anti-ATM, anti-ATR, anti-TopBP1, anti-CtIP, anti-Nbs1 and anti-RPA70 antibodies. (B) Mock-depleted (lanes 1 and 2) and CtIP-depleted egg extracts (lanes 3 and 4) containing 35S-Chk1 were incubated with sperm nuclei in the absence (lanes 1 and 3) or in the presence of PflMI (lanes 2 and 4). Nuclear fractions were isolated and subjected to SDS-PAGE and phosphorimaging to detect radiolabeled Chk1 and also immunoblotted with anti-ATM, anti-ATR, anti-TopBP1, anti-CtIP, anti-Nbs1 and anti-RPA70 antibodies. Unshifted and shifted forms of Chk1 are indicated with a dash and arrow, respectively. (C) Mock-depleted (lanes 1 and 2) and CtIP-depleted (lanes 3 and 4) extracts containing sperm nuclei were incubated in the absence (lanes 1 and 3) or presence (lanes 2 and 4) of PflMI. Chromatin fractions were isolated, subjected to SDS-PAGE and immunoblotted with anti-ATM, anti-ATR, anti-TopBP1, anti-phospho[S-1131]TopBP1, anti-CtIP, anti-Nbs1, anti-RPA70 and anti-Orc2 antibodies. ATM is denoted with an arrow, whereas residual ATR signal from previous blotting is marked with an asterisk.
![Figure 5 CtIP mediates damage-dependent nuclear accumulation of TopBP1 in response to DSBs in Xenopus egg extracts. (A) Representative samples of extracts that were either left undepleted (lane 1), mock-depleted with control IgG antibodies (lane 2) or depleted with anti-CtIP antibodies (lane 3). Extracts were immunoblotted with anti-ATM, anti-ATR, anti-TopBP1, anti-CtIP, anti-Nbs1 and anti-RPA70 antibodies. (B) Mock-depleted (lanes 1 and 2) and CtIP-depleted egg extracts (lanes 3 and 4) containing 35S-Chk1 were incubated with sperm nuclei in the absence (lanes 1 and 3) or in the presence of PflMI (lanes 2 and 4). Nuclear fractions were isolated and subjected to SDS-PAGE and phosphorimaging to detect radiolabeled Chk1 and also immunoblotted with anti-ATM, anti-ATR, anti-TopBP1, anti-CtIP, anti-Nbs1 and anti-RPA70 antibodies. Unshifted and shifted forms of Chk1 are indicated with a dash and arrow, respectively. (C) Mock-depleted (lanes 1 and 2) and CtIP-depleted (lanes 3 and 4) extracts containing sperm nuclei were incubated in the absence (lanes 1 and 3) or presence (lanes 2 and 4) of PflMI. Chromatin fractions were isolated, subjected to SDS-PAGE and immunoblotted with anti-ATM, anti-ATR, anti-TopBP1, anti-phospho[S-1131]TopBP1, anti-CtIP, anti-Nbs1, anti-RPA70 and anti-Orc2 antibodies. ATM is denoted with an arrow, whereas residual ATR signal from previous blotting is marked with an asterisk.](/cms/asset/c39aee9e-f353-428c-bebe-71b3682b4971/kccy_a_10914711_f0005.gif)
Additional material
Download Zip (845.6 KB)Acknowledgements
We deeply appreciate all the members of our laboratory for their insightful discussions and comments on the manuscript and the data. This work was supported by NIH grants GM043974 and GM070891 and an Ellison Senior Scholar in Aging award to W.G.D. H.Y.Y. was supported by Samsung Biomedical Research Institute grant #SBRI O-A9-001-2 and by the Mid-career Researcher Program (2009-0085548) through an NRF grant funded by the MEST of Korea.
References
- Kastan MB, Bartek J. Cell cycle checkpoints and cancer. Nature 2004; 432:316 - 323
- Negrini S, Gorgoulis VG, Halazonetis TD. Genomic instability: an evolving hallmark of cancer. Nat Rev Mol Cell Biol 2010; 11:220 - 228
- Nyberg KA, Michelson RJ, Putnam CW, Weinert TA. Toward maintaining the genome: DNA damage and replication checkpoints. Annu Rev Genet 2002; 36:617 - 656
- Sancar A, Lindsey-Boltz LA, Unsal-Kacmaz K, Linn S. Molecular mechanisms of mammalian DNA repair and the DNA damage checkpoints. Annu Rev Biochem 2004; 73:39 - 85
- Abraham RT. Cell cycle checkpoint signaling through the ATM and ATR kinases. Genes Dev 2001; 15:2177 - 2196
- Lavin MF. Ataxia-telangiectasia: From a rare disorder to a paradigm for cell signalling and cancer. Nat Rev Mol Cell Biol 2008; 9:759 - 769
- Cimprich KA, Cortez D. ATR: An essential regulator of genome integrity. Nat Rev Mol Cell Biol 2008; 9:616 - 627
- Halazonetis TD, Gorgoulis VG, Bartek J. An oncogene-induced DNA damage model for cancer development. Science 2008; 319:1352 - 1355
- Cortez D, Guntuku S, Qin J, Elledge SJ. ATR and ATRIP: Partners in checkpoint signaling. Science 2001; 294:1713 - 1716
- Zou L, Elledge SJ. Sensing DNA damage through ATRIP recognition of RPA-ssDNA complexes. Science 2003; 300:1542 - 1548
- Mordes DA, Glick GG, Zhao R, Cortez D. TopBP1 activates ATR through ATRIP and a PIKK regulatory domain. Genes Dev 2008; 22:1478 - 1489
- Kumagai A, Lee J, Yoo HY, Dunphy WG. TopBP1 activates the ATR-ATRIP complex. Cell 2006; 124:943 - 955
- Lee J, Kumagai A, Dunphy WG. The Rad9-Hus1-Rad1 checkpoint clamp regulates interaction of TopBP1 with ATR. J Biol Chem 2007; 282:28036 - 28044
- Lee J, Dunphy WG. Rad17 plays a central role in establishment of the interaction between TopBP1 and the Rad9-Hus1-Rad1 complex at stalled replication forks. Mol Biol Cell 2010; 21:926 - 935
- Yoo HY, Kumagai A, Shevchenko A, Shevchenko A, Dunphy WG. Ataxia-telangiectasia mutated (ATM)-dependent activation of ATR occurs through phosphorylation of TopBP1 by ATM. J Biol Chem 2007; 282:17501 - 17506
- Yoo HY, Kumagai A, Shevchenko A, Shevchenko A, Dunphy WG. The Mre11-Rad50-Nbs1 complex mediates activation of TopBP1 by ATM. Mol Biol Cell 2009; 20:2351 - 2360
- Hashimoto Y, Tsujimura T, Sugino A, Takisawa H. The phosphorylated C-terminal domain of Xenopus Cut5 directly mediates ATR-dependent activation of Chk1. Genes Cells 2006; 11:993 - 1007
- Uziel T, Lerenthal Y, Moyal L, Andegeko Y, Mittelman L, Shiloh Y. Requirement of the MRN complex for ATM activation by DNA damage. EMBO J 2003; 22:5612 - 5621
- Jazayeri A, Falck J, Lukas C, Bartek J, Smith GC, Lukas J, et al. ATM- and cell cycle-dependent regulation of ATR in response to DNA double-strand breaks. Nat Cell Biol 2006; 8:37 - 45
- Byun TS, Pacek M, Yee MC, Walter JC, Cimprich KA. Functional uncoupling of MCM helicase and DNA polymerase activities activates the ATR-dependent checkpoint. Genes Dev 2005; 19:1040 - 1052
- Shiotani B, Zou L. Single-stranded DNA orchestrates an ATM-to-ATR switch at DNA breaks. Mol Cell 2009; 33:547 - 558
- Lengsfeld BM, Rattray AJ, Bhaskara V, Ghirlando R, Paull TT. Sae2 is an endonuclease that processes hairpin DNA cooperatively with the Mre11/Rad50/Xrs2 complex. Mol Cell 2007; 28:638 - 651
- Limbo O, Chahwan C, Yamada Y, de Bruin RA, Wittenberg C, Russell P. Ctp1 is a cell cycle-regulated protein that functions with Mre11 complex to control double-strand break repair by homologous recombination. Mol Cell 2007; 28:134 - 146
- Sartori AA, Lukas C, Coates J, Mistrik M, Fu S, Bartek J, et al. Human CtIP promotes DNA end resection. Nature 2007; 450:509 - 514
- Mimitou EP, Symington LS. DNA end resection: many nucleases make light work. DNA Repair (Amst) 2009; 8:983 - 995
- You Z, Shi LZ, Zhu Q, Wu P, Zhang YW, Basilio A, et al. CtIP links DNA double-strand break sensing to resection. Mol Cell 2009; 36:954 - 969
- Fusco C, Reymond A, Zervos AS. Molecular cloning and characterization of a novel retinoblastoma-binding protein. Genomics 1998; 51:351 - 358
- Schaeper U, Subramanian T, Lim L, Boyd JM, Chinnadurai G. Interaction between a cellular protein that binds to the C-terminal region of adenovirus E1A (CtBP) and a novel cellular protein is disrupted by E1A through a conserved PLDLS motif. J Biol Chem 1998; 273:8549 - 8552
- Li S, Ting NS, Zheng L, Chen PL, Ziv Y, Shiloh Y, et al. Functional link of BRCA1 and ataxia telangiectasia gene product in DNA damage response. Nature 2000; 406:210 - 215
- Wu-Baer F, Baer R. Effect of DNA damage on a BRCA1 complex. Nature 2001; 414:36
- Yu X, Chen J. DNA damage-induced cell cycle checkpoint control requires CtIP, a phosphorylation-dependent binding partner of BRCA1 C-terminal domains. Mol Cell Biol 2004; 24:9478 - 9486
- Yu X, Fu S, Lai M, Baer R, Chen J. BRCA1 ubiquitinates its phosphorylation-dependent binding partner CtIP. Genes Dev 2006; 20:1721 - 1726
- Chen L, Nievera CJ, Lee AY, Wu X. Cell cycledependent complex formation of BRCA1.CtIP.MRN is important for DNA double-strand break repair. J Biol Chem 2008; 283:7713 - 7720
- Hartsuiker E, Neale MJ, Carr AM. Distinct requirements for the Rad32(Mre11) nuclease and Ctp1(CtIP) in the removal of covalently bound topoisomerase I and II from DNA. Mol Cell 2009; 33:117 - 123
- Yun MH, Hiom K. CtIP-BRCA1 modulates the choice of DNA double-strand-break repair pathway throughout the cell cycle. Nature 2009; 459:460 - 463
- Yuan J, Chen J. N terminus of CtIP is critical for homologous recombination-mediated double-strand break repair. J Biol Chem 2009; 284:31746 - 31752
- Takeda S, Nakamura K, Taniguchi Y, Paull TT. Ctp1/CtIP and the MRN complex collaborate in the initial steps of homologous recombination. Mol Cell 2007; 28:351 - 352
- Kumagai A, Dunphy WG. Claspin, a novel protein required for the activation of Chk1 during a DNA replication checkpoint response in Xenopus egg extracts. Mol Cell 2000; 6:839 - 849
- Lee J, Kumagai A, Dunphy WG. Claspin, a Chk1-regulatory protein, monitors DNA replication on chromatin independently of RPA, ATR and Rad17. Mol Cell 2003; 11:329 - 340
- Kumagai A, Guo Z, Emami KH, Wang SX, Dunphy WG. The Xenopus Chk1 protein kinase mediates a caffeine-sensitive pathway of checkpoint control in cell-free extracts. J Cell Biol 1998; 142:1559 - 1569
- Yu X, Wu LC, Bowcock AM, Aronheim A, Baer R. The C-terminal (BRCT) domains of BRCA1 interact in vivo with CtIP, a protein implicated in the CtBP pathway of transcriptional repression. J Biol Chem 1998; 273:25388 - 25392
- Nakamura K, Kogame T, Oshiumi H, Shinohara A, Sumitomo Y, Agama K, et al. Collaborative action of Brca1 and CtIP in elimination of covalent modifications from double-strand breaks to facilitate subsequent break repair. PLoS Genet 2010; 6:1000828
- Caldecott KW. Cell signaling. The BRCT domain: signaling with friends?. Science 2003; 302:579 - 580
- Rodriguez M, Yu X, Chen J, Songyang Z. Phosphopeptide binding specificities of BRCA1 COOH-terminal (BRCT) domains. J Biol Chem 2003; 278:52914 - 52918
- Toledo LI, Murga M, Gutierrez-Martinez P, Soria R, Fernandez-Capetillo O. ATR signaling can drive cells into senescence in the absence of DNA breaks. Genes Dev 2008; 22:297 - 302
- Mimitou EP, Symington LS. Sae2, Exo1 and Sgs1 collaborate in DNA double-strand break processing. Nature 2008; 455:770 - 774
- Zhu Z, Chung WH, Shim EY, Lee SE, Ira G. Sgs1 helicase and two nucleases Dna2 and Exo1 resect DNA double-strand break ends. Cell 2008; 134:981 - 994
- Farah JA, Cromie GA, Smith GR. Ctp1 and Exonuclease 1, alternative nucleases regulated by the MRN complex, are required for efficient meiotic recombination. Proc Natl Acad Sci USA 2009; 106:9356 - 9361
- Murray AW. Cell cycle extracts. Methods Cell Biol 1991; 36:581 - 605
- Yoo HY, Kumagai A, Shevchenko A, Shevchenko A, Dunphy WG. Adaptation of a DNA replication checkpoint response depends upon inactivation of Claspin by the Polo-like kinase. Cell 2004; 117:575 - 588
- Yanow SK, Gold DA, Yoo HY, Dunphy WG. Xenopus Drf1, a regulator of Cdc7, displays checkpoint-dependent accumulation on chromatin during an S-phase arrest. J Biol Chem 2003; 278:41083 - 41092
- Yoo HY, Shevchenko A, Shevchenko A, Dunphy WG. Mcm2 is a direct substrate of ATM and ATR during DNA damage and DNA replication checkpoint responses. J Biol Chem 2004; 279:53353 - 53364