Abstract
Insulin resistance is a risk factor in the development of type 2 diabetes and is a major cause of atherosclerosis. Reduction in heme oxygenase (HO-1) has been shown to exacerbate vascular dysfunction and insulin resistance in obese mice and involves a decrease in adiponectin levels. Adiponectin is released from mesenchymal stem cell (MSC)-derived adipocytes, its levels are decreased in type 2 diabetes. We hypothesized that the apoA1 mimetic peptide, L-4F, will target the expression of the HO-1-adiponectin axis and reverse adipocyte dysfunction both in vivo and in vitro. The administration of L-4F [2 mg/Kg/daily (i.p.) for 4-week to 8-week-old obese (ob) mice restored adipocyte function, increased adiponectin release (p < 0.05) and decreased the levels of IL-1 and IL-6 (p < 0.05)]. These perturbations were associated with an increase in insulin sensitivity (p < 0.01 vs. untreated ob mice) and decreased glucose levels (309 + 42 vs. 201 + 8 mg/d after L-4F treatment). Treatment of both mesenchymal stem cell (MSC)-derived adipocytes with L-4F (50 μg/ml) increased adiponectin (p < 0.05), decreased IL-1 and IL-6 (p < 0.05) levels and increased MSC-derived adipocyte cell numbers by 50% in S phase (p < 0.05). MSC-derived adipocytes treated with L-4F increased WNT10b and decreased Peg 1/Mest. Inhibition of HO activity reversed the decrease in the adipogenic response gene, Peg 1/Mest. An increase of HO-1 expression by L-4F increased insulin-receptor phosphorylation. These findings support the hypothesis that L-4F increases early adipocyte markers, HO-1-adiponectin, WNT10b and decreases Peg1/Mest, negative regulators of adipocyte differentiation.
Introduction
Insulin resistance and obesity are considered major risk factors for exacerbating the clinical consequences of diabetes, hypertension and metabolic syndrome. Insulin resistance, a result of high fat intake and inactivity leading to obesity, is a cause of vascular dysfunction.Citation1–Citation5 Increasing adipocyte size has been shown in individuals with adult-onset obesity, where adipocyte hypertrophy and hyperplasia has been reported in rodentsCitation6–Citation8 and in individuals with early-onset obesity.Citation9 Adipocyte size increase results from either the increased storage of triglycerides from dietary sources or is generated by lipogenic pathways; new adipocytes may arise via the proliferation and differentiation of both pre-adipocytes and adipoblasts to mature adipocytes.Citation10–Citation12 Small adipocytes that accumulate from small lipids differentiated from pre-adipocytes secrete the insulin-sensitizing hormone, adiponectin.Citation8,Citation13 Adiponectin is an essential growth factor and increases insulin sensitivity and vascular function and has recently been shown to be involved with aging.Citation14–Citation18 A high fat diet induced adipocyte hypertrophy (large adipocytes) which resulted in a decrease in the secretion of insulin-sensitizing hormone and an increase in insulin-resistant hormone, leading to insulin resistance in diabetes and obesityCitation19 due, in part, to a decrease in adiponectin release.Citation7,Citation18,Citation20 In cultured adipocytes, elevated levels of fatty acids and glucose increased oxidative stress resulting in the modulation of adipokines manifest by decreased adiponectin and increased IL6 and MCP-1 levels.Citation7,Citation21–Citation23
Mesenchymal stem cells (MSCs) are capable of differentiation into adipocytes, osteoblasts, myoblasts and connective tissue.Citation24,Citation25 MSCs give rise to common early precursor adipoblasts,Citation12 which develop into committed white and brown pre-adipocytes that, under appropriate stimulatory conditions, differentiate into different types of mature adipocytes.Citation11,Citation12 Adipocytes are a major organ regulating glucose homeostasis,Citation26 and adipocyte dysfunction results in the release of lower levels of adiponectin along with decreased glucose uptake, leading to insulin resistance.Citation27 MSCs express several regulatory proteins such as Wingless-type (Wnts) and β-catenin; both are essential in the regulation of the conversion of pre-adipocytes to adipocytes.Citation11,Citation28,Citation29 Wnt10b, a member of Wnt family, maintains pre-adipocytes in an undifferentiated state. Wnt10b expression is increased in pre-adipocytes and blocks adipocyte differentiation.Citation11,Citation29 Increases in Wnt/β-catenin inhibit adipogenic transcription factors CCAAT/enhancer binding protein a (C/EBPα) and peroxisome proliferator activator receptor (PPARγ) and repress adipogenesis.Citation30–Citation33 Overexpression of Wnt10b in 3T3-L1 pre-adipocytes blocks adipogenesisCitation34 and also is critical in the determination of mesodermal cell fat levels.Citation32 In contrast to Wnt, paternally expressed 1 (Peg 1)/mesoderm-specific transcript Mest,Citation35 when upregulated, results in the enlargement of adipocytes during adipose tissue expansion.Citation36 Upon accumulation of triglycerides, the levels of Peg 1/Mest gene expression, regarded as a marker of adipocyte size,Citation36 are increased and, subsequently, signal pre-adipocytes to enlarge to accommodate more triglycerides. Adipocyte enlargement is associated with an increase in the release of TNFα, IL-1, IL-6 and increased insulin resistance.Citation6–Citation8,Citation25 Induction of HO-1 has been shown to enhance cell survival and moderate the diabetic state and obesity.Citation37–Citation39 Induction of HO-1 gene expression in vivo and in cell culture is associated with an increase in pre-adipocytes, a reduction in the number of enlarged adipocytes, but an increase in small adipocyte and adiponectin levels.Citation8,Citation23,Citation25,Citation40 HO-1 is induced by a broad spectrum of inducers such as statins, curcumin and ApoA1 mimetic peptidesCitation41 and has been shown to play a crucial role in protection against oxidative insult in diabetes and cardiovascular disease.Citation41 A decrease in HO-1 expression results in increased insulin resistance and adiposity in Zucker rats and ob mice.Citation8,Citation42 It remains unclear if the endogenous expression by an inducer of HO-1 expression is beneficial or detrimental to adipocyte stem function in vivo and in vitro. Accordingly, we tested the hypothesis that the ApoA1 mimetic peptide, L-4F, mediated upregulation of HO-1 and alleviated MSC-derived adipocyte dysfunction, resulting in a reduction in the release of inflammatory cytokines and increase insulin sensitivity.
Results
Effect of L-4F on metabolic parameters, plasma adiponectin and inflammatory cytokines level.
As seen in , L-4F treatment daily for 4 weeks prevented weight gain in ob mice when compared with vehicle-treated ob mice (p < 0.01). The blood glucose levels were significantly decreased in L-4F-treated ob mice when compared with vehicle-treated ob mice (, p < 0.05). There was a significant increase in serum adiponectin levels in L-4F-treated ob mice compared with vehicle-treated animals (); adiponectin levels in ob mice were 2.3 ± 0.37 ng/ ml in vehicle-treated animals compared with 4.1 ± 0.34 ng/ml (p < 0.05) in ob mice administered L-4F for 4 weeks (p < 0.05). Ob mice administered L-4F exhibited a significant (p < 0.05) decrease in serum IL-1 levels (66 ± 25 pg/ml) when compared with vehicle-treated ob animals (141 ± 11 pg/ml) (). Similar results were observed with serum IL-6 () levels. The perturbations in serum IL-6, IL-1β levels were the reciprocal of serum adiponectin levels.
L-4F induced changes in glucose tolerance and insulin sensitivity.
Insulin sensitivity was determined in the two groups, ob treated with vehicle and ob mice treated with L-4F for 4 weeks. A rapid decrease in glucose levels following administration of insulin in ob mice treated with L-4F was evident, while vehicle-treated ob mice maintained an elevated level of glucose (). The decrease in glucose levels as a result of treatment with L-4F is reflected by improved insulin sensitivity (results not shown).
Determination of MSCs surface markers expressions and effect of L-4F on cell cycle measurement.
MSCs were obtained from ob mice bone marrow and were cultured in adipocyte-induced medium. Confirmation of the MSC phenotype was made by the presence of positive markers measured by FACS: 77% of the cells were positive for CD166, and 87.72% of the cells were positive for CD90. In addition, the absence of CD34, a hematopoietic stem cell marker, and CD45, a lymphocytic marker (), indicate that there was less than 0.2% positive staining for these negative markers, indicating that the MSCs were not contaminated with lymphocytes and hematopoietic stem cells. Cell cycle analysis revealed that 15.5% of MSCs were in the S phase when the cells were cultured in L-4F, and that L-4F increased adipocyte cell numbers by 50% in the S phase (p < 0.05) ().
Effect of L-4F on HO-1 expression and activity in MSCs.
Western blot analysis showed a significant difference (p < 0.001) in the ratio of HO-1 to actin in the MSCs cultured in adipocyte media and L-4F-treated MSCs during adipogenesis (). We then examined the basal levels of HO activity in MSC-derived adipocyte cell homogenate. As seen in , MSC-derived preadipocyte (5 d) cell homogenates display a higher activity compared with adipocytes (12 d). MSC pre-adipocytes pretreated with L-4F increased bilirubin levels compared with cell homogenates treated with vehicle solutions (p < 0.01). To ascertain the increase to activation of HO-1 gene expression and the increase in HO-1 protein, cell homogenates were incubated with SnMP, an inhibitor of HO activity.Citation43 SnMP abolished the increase in HO activity in both preadipocyte (data not shown) and adipocytes (12 d).
Effect of L-4F on Wnt10b, β-catenin and PPARγ expression.
L-4F treatment upregulated the expression of Wnt10b after 12 d of culture compared with cells treated with vehicle solutions (). Treatment with the HO inhibitor reversed the effect of L-4F on Wnt10b. Similarly, L-4F increased the levels of β-catenin (p < 0.01) (), and this effect was abolished by concurrent administration of SnMP. In addition we examined PPARγ protein expression and showed that L-4F treatment decreased PPARγ levels (p < 0.05) ().
Effect of L-4F on Peg1/Mest and IR pTyr1146 expression.
L-4F treatment inhibited Peg1/Mest protein levels after 12 d of culture compared with cells treated with vehicle solutions (), and treatment with the HO inhibitor reversed the effect of L-4F on Peg1/Mest (p < 0.01). Contrary to the above result, marked decrease in IR pTyr1146 expression was observed after 12 d of culture, compared with 5 d of culture (), and L-4F treatment significantly increased IR pTyr1146 protein levels after 12 d of culture compared with cells treated with vehicle solutions. Treatment with the HO inhibitor, reversed the effect of L-4F on IR pTyr1146 expression (; p < 0.05).
Effect of L-4F on adipocyte size.
We examined the effect of L-4F on adipocyte size at day 12 of L-4F treatment. As seen in , L-4F decreased the size of adipocytes and reduced the number of large adipocytes. This effect was negated by treatment with SnMP.
Discussion
This is the first study to demonstrate that an increase in HO-1 expression is directly involved in the regulation of adipogenic markers by increasing Wnt10b protein levels, decreasing Peg/Mest and improving insulin sensitivity. In vivoCitation7 and in vitro L-4F treatment was associated with a marked increase in HO-1 expression and HO activity. The increase of HO-1 expression was associated with an increase in insulin sensitivity as manifested by lowered blood glucose levels and a decrease in body weight. The increase in HO-1 expression appears to have an effect on adiposity. Thus, HO-1 induction by L-4F treatment is capable of reprogramming adipocytes, resulting in improved insulin sensitivity and vascular function, as evidenced by the following HO-1 overexpression, which increases HO activity, adiponectin levels and decreases glucose levels. Adiponectin is solely synthesized and released by adipocytes, although a recent report indicates that pressure of adiponectin in lung epithelial cells.Citation44 The identification of a primary role of HO-1 on adiponectin release and the role of obesity and hyperglycemia in induced adipocyte differentiation has at least two important conceptual implications. Increased HO-1 levels have anti-adipogenic potential, thus indicating a therapeutic approach by regulating the signal transduction pathways involved in preadipocyte differentiation to small mature adipocytes. There appears to be a direct link between inhibition of HO activity and the increase in adipocyte enlargement and adiposity. This highlights the importance of glucose suppression of HO-1 expression in mediating the ROS signaling pathway to increased levels of inflammatory cytokines, IL1β and IL-6. The latter are major cytokines in insulin resistance. Hyperglycemia results in a decrease in both HO-1 expression and HO activity.Citation45–Citation49 The decrease in HO-1 expression as a result of increased glucose levels, increases ROS, endothelial cell death, endothelial progenitor function and insulin resistance.Citation48,Citation50–Citation52 Increased ROS levels and decreased levels of serum adiponectin thereby contribute to the pathogenesis of insulin resistance.Citation18,Citation53 Humans and rodents deficient in either HO-1 or HO-2 develop vascular dysfunction and die prematurely.Citation54–Citation56 Indeed, HO-1 isoforms have been shown to translocate to the mitochondria in diabetesCitation57 and to play an important role in the attenuation of diabetes-mediated formation of ROS and the resultant complications. In addition to the anti-adiposity effect described above, HO-1 appears to be directly involved in resisting oxidative stress, including angiogenesis-mediated hypertension.Citation41 We have shown that HO-1 recruits EC-SOD to act as an antioxidant, dissipating H2O2,Citation48,Citation58 and to trigger an increase in adiponectin and the pAMPK-pAkt signaling pathways.
Our results support the hypothesis that the expansion of adipogenesis (as seen in the present study that increased the levels of HO-1) leads to an increased number of small size adipocytes. Small adipocytes are considered healthy, insulin-sensitive adipocyte cells that are capable of producing increased amounts of adiponectin.Citation59 Therefore, upregulation of HO-1 produced adipose remodeling and increased the number of smaller differentiated adipocytes. Thus the HO-1-mediated increase in Wnt10b resulted in either maintaining pre-adipocytes in the undifferentiated state or slowing down that process. Wnt10b is highly expressed in preadipocyte and declines rapidly after induction of adipogenic differentiation.Citation31,Citation32 Inhibition of HO activity by SnMP decreased Wnt10b and increased the number of large adipocytes. We noted that inhibition of HO activity occurred despite an increase in HO-1 mRNA and protein content, confirming previous results.Citation60 Our results are in agreement with reports that overexpression of Wnt10b in 3T3-L1 blocks adipogenesis,Citation34 and that disruption of Wnt10b signaling increases adipogenesis.Citation34 This highlights the importance of this pathway in adipogenesis (). Thus, Wnt10b may be considered an HO-1 gene target resulting in the reduction of adipogenesis and a decrease in PPARγ levels manifest by an increase in small adipocytes, release of adiponectin and a decrease in the levels of IL-1 and IL-6. Further, the increase in Wnt10b that paralleled the increase in HO-1 expression was associated with a significant reduction in Peg1/Mest. A decrease in Peg 1/Mest is beneficial in the control of adiposity, since upregulation of Peg 1/Mest occurs in obese adipose tissue in several models of obesity.Citation35 Further, overexpression of Peg1/Mest promotes adipogenesis and results in enlarged adipocytes and increased levels of inflammatory cytokines. Therefore, HO-1 may also control adiposity by its ability to decrease Peg 1/Mest. Koza et al. showed that although Peg 1/Mest levels were detectable in pre-obese animals, the levels of Peg 1/Mest was highly elevated and associated with the obese phenotype.Citation61 Wnt10b and Peg 1/Mest play a major role on the production of MSC-derived adipocytes, and their roles in adipose tissue homeostasis are well-documented.Citation32,Citation35,Citation62 Thus, we show in this report that perturbations in HO-1 expression and HO activity can affect adipose homeostasis by regulating levels of Wnt10b and Peg 1/Mest, suggesting a role for a L-4F-induced expression of HO-1 in this process. It remains to be determined if chronic L-4F treatment affects other pathways in adipose homeostasis.
In summary, HO-1 induction by L-4F effectively decreased adipose tissue in whole animals and MSCs and may offer a therapeutic approach to preventing the ravages associated with diabetes, hypertension, cardiovascular disease and metabolic syndrome. Based on the evidence that we present here, increases in HO-1 following treatment with L-4F increased the Wnt signaling pathway and decreased Peg1/Mest signaling, suggesting that the increase in HO-1 expression plays a role in the maintenance of pre-adipocytes in the undifferentiated state. In support of this conclusion, inhibition of HO activity by SnMP decreased Wnt10b, increased Peg1/Mest protein levels, increased PPARγ, increased adipocyte cell size and the release of the inflammatory cytokines IL-1 and IL-6. Our study validates a novel and clinically attractive concept that upregulation of the HO-1-adiponectin axis, using a pharmacological approach, decreases adipocyte cell dysfunction and enlargement and decreases inflammatory cytokines, leading to the restoration of insulin sensitivity and vascular function.
Materials and Methods
Animal protocols.
Male obese (ob) mice (B6V-Lep-ob/J) were purchased from Harlan at the age of 7 weeks and acclimatized for one week (8 weeks). The mice had free access to water and food and were injected at 8 weeks of age with L-4FCitation6 at a dose of 2 mg/Kg daily in 200 µl vehicle or vehicle alone (ABCT; ammonium bicarbonate buffer at pH 7.4 containing 0.01% Tween 20). L-4F and vehicle were administered intraperitoneally (i.p.) daily for 4 weeks. All animal studies were approved by the New York Medical College Animal Care and Use Committee in accordance with the National Institutes of Health Guidelines for Care and Use of Laboratory Animals.
Isolation of mice bone marrow.
The bone marrow cells were collected from the femur and tibia of ob mice. Non-adherent cells were removed on day 4, and adherent cells were further incubated in fresh IMEM, including 10% FBS (Invitrogen Corporation) and 1% antibiotic-antimycotic solution (Invitrogen). The cultured cells with spindle-like morphology after three passages were defined as mesenchymal stem cells (MSCs) and were induced to differentiate into adipocyte cells in vitro.
Detection of MSC cell markers by FACS analysis.
The MSCs presented as a homogeneous fibroblastic cell population. Flow cytometric analysis of passage 4 cells confirmed that cells were negative for CD34 and CD45, and that cells were positive for CD166 and CD90 (Thy-1).
Induction of adipocytes from bone marrow MSCs.
The MSCs were cultured in radiogenic medium, high glucose-DMEM (Invitrogen) with 10 ug/ml insulin, 0.1 mM dexamethasone, 0.2 mM indomethacin, 10% FBS and 1% antibiotic-antimycotic solution. After 50% confluence, either L-4F, 50 ug/ml or vehicle were added daily. The cell cycles were measured at day 5 after induction of adipogenesis, and the cultured cells were collected at day 10 for measurement of protein. Tin mesoporphysin (SnMP), an inhibitor of HO activity, was added to cell cultures every 2.5 d to a final concentration of 1 uM.
Cytokine measurements.
Adiponectin (high molecular weight, HMW), IL1β and IL-8 were determined in mice serum and in cell culture condition media using ELISA assay (Pierce Biotechnology Inc.), and insulin was measured using an ELISA kit (Millipore).
Insulin tolerance test.
After a 12 h fast, mice were injected intraperitoneally with glucose (2.0 g Kg body weight). Blood samples were taken at the various time points indicated in the figure legends (0–120 min), and blood glucose levels were measured. For the insulin tolerance test, mice were injected intraperitoneally with insulin (2.0 U/Kg). Blood samples were taken at the various time points indicted in the figure legends (0–90 min), and blood glucose levels were determined.
Determination of adipocyte cell size.
Adipocyte cell size was measured using Image Pro Analyzer (Media Cybernetics, Inc.) as previously described in references Citation6 and Citation25. The classification of the size of lipid droplet was as follows and is based on size by area (pixels): < 200 pixels (large lipid droplets), < 180 (small lipid droplets).
Cell preparations for western blot and HO activity.
Cultured bone marrow MSCs homogenates were frozen after inducing adipogenesis at various time points. Western blot analysis was performed on cell homogenates to measure, HO-1, Wnt10b, βcatenin, IR p-Tyr1146 and Peg1/Mest. HO activity was measured according to previously described methods in references Citation8 and Citation23, and the results were expressed as nmol bilirubin/mg cell protein/60 min.
Statistical analyses.
Statistical significance between experimental groups was determined by the Fisher method of analysis of multiple comparisons. For comparison between treatment groups, the null hypothesis was tested by both a single factor ANOVA for multiple groups and the unpaired t-test for two groups. Data are presented as means ± SE. Statistical significance was defined as p < 0.05.
Disclosure of Potential Conflicts of Interest
No potential conflicts of interest were disclosed.
Abbreviations
HO-1 | = | heme oxygenase 1 |
MSC | = | mesenchymal stem cell |
ROS | = | reactive oxygen species |
apoA-I | = | apolipoprotein A-I |
Figures and Tables
Figure 1 Effect of L-4F on body weight, blood glucose levels, adiponectin, IL-1 and IL-6 in ob mice. (A–E) respectively. (A) Body weight is shown. (B) Fasting blood glucose levels (*p < 0.001). (C) Plasma adiponectin levels (*p < 0.05). (D) Plasma IL-1 levels (*p < 0.01). (E) Plasma IL-6 levels (*p < 0.01). (F) Blood glucose levels after insulin administration (*p < 0.05). The results are mean ± SE, n = 6 in each group.
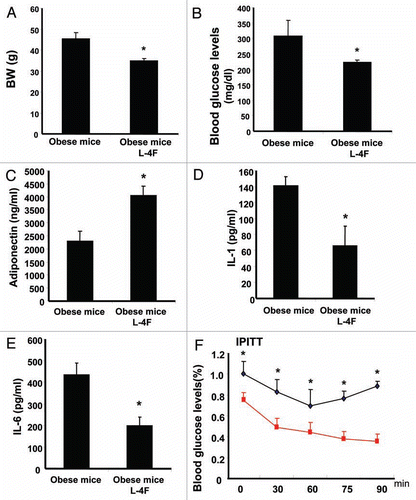
Figure 2 Determination of MSCs phenotype and cell cycle measurement. (A) Membrane antigen expressions of CD45, CD4, CD90, CD166 and on hMSCs were analyzed by FACS analysis. As positive markers CD90 (Thy-1) and CD166 (ALCAM, activated leukocyte cell adhesion molecule) were 87.72% and 77% respectively. As negative markers, CD45 (common lymphocytes antigen) and CD34, an hematopoietic stem cell marker, were shown to be expressed in less than 1% of cells. (B) Effect on cell cycle and Growth. Cells were treated Vinblastin to arrest mitosis then we measured DNA distribution by DAPI staining. Cell cycle analysis revealed that 15.5% of MSCs were in the S phase when the cells were cultured in L-4F.
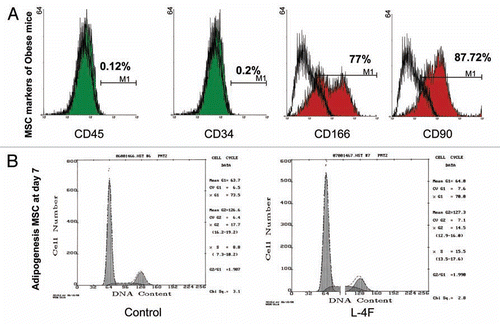
Figure 3 Effect of L-4F on HO-1 expression and activity. (A) Western blot of HO-1 proteins in MSC-derived adipocytes treated with L-4F and SnMP. Representative immunoblots are shown (n = 4). MSCs were cultured in adipogenic differentiation media and L-4F was added every 3 days. Quantitative densitometry evaluation of HO-1 and actin proteins ratio was determined. Data are expressed as means ± SD (*p < 0.05 vs. day 12). (B) Effect of L-4F on HO activity as measured by bilirubin generation. Bilirubin formed in cellular homogenates in the presence of heme and NADPH was measured (*p < 0.001 vs. day 12). Combination of L-4F with SnMP reduces HO activity (#p < 0.001 vs. day 12 + L-4F).
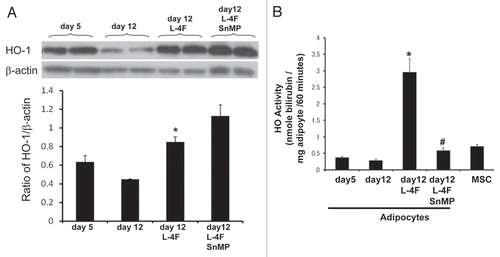
Figure 4 Effect of L-4F on Wnt10b, β-catenin and PPARγ levels. Western blot of Wnt10b, β-catenin, PPARγ and actin proteins in MSC-derived adipocytes treated with L-4F alone or in combination with SnMP. Representative immunoblots are shown (n = 4). Quantitative densitometry evaluation of Wnt10b β-catenin and actin proteins ratio was determined. Data are expressed as means ± SD (*p < 0.05 vs. day 5, #p < 0.05 vs. day 12, +p < 0.05 vs. day5 + L4F).
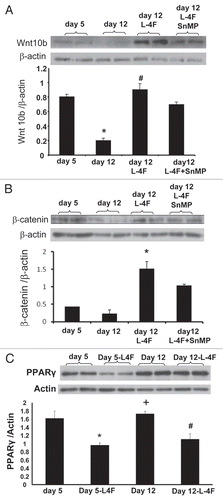
Figure 5 Effect of L-4F on Mest and IRP-Tyr1146. Western blot of Mest, IRP-Tyr1146 and actin proteins in MSC-derived adipocytes treated with L-4F alone or in combination with SnMP. Representative immunoblots are shown (n = 4). Quantitative densitometry evaluation of Mest, IRP-Tyr1146 and actin proteins ratio was determined. Data are expressed as means ± SD (*p < 0.05 vs. day 5, #p < 0.05 vs. day 12, +p < 0.05 vs. day 5 + L4F).
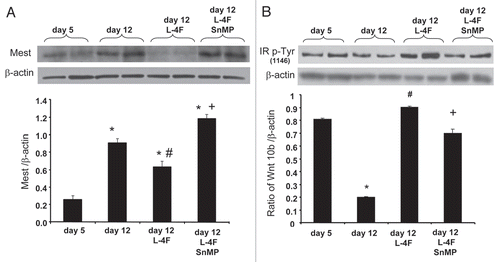
Figure 6 Effect of L-4F on adipogenesis and adipocyte size. (A) Scan of lipid size of a representative experiment. Adipogenesis was measured as the relative absorbance of Oil Red O at day 12 after inducing adipogenesis as described in Materials and Methods. (B) Measurement of diameter of lipid droplet size. Results are mean ± SD (*p < 0.05 vs. L-4F, #p < 0.05 vs. L-4F + SnMP).
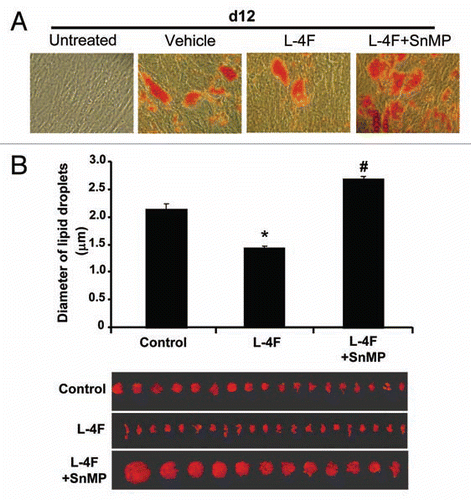
Figure 7 Hypertrophy adipocytes are associated with dysfunctional adipogenesis with resultant contributions toward metabolic imbalance. The proposed mechanism demonstrate that an upregulation of HO-1 is directly involved in the regulation of adipogenic markers by increasing Wnt10b protein levels, decreasing Peg/Mest and improving insulin sensitivity.
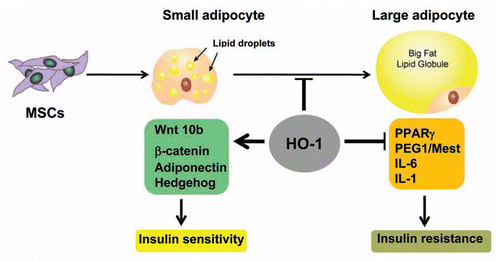
Acknowledgements
This work was supported by National Institutes of Health grants DK068134, HL55601 and HL-34300 (NGA).
References
- Schulz E, Dopheide J, Schuhmacher S, Thomas SR, Chen K, Daiber A, et al. Suppression of the JNK pathway by induction of a metabolic stress response prevents vascular injury and dysfunction. Circulation 2008; 118:1347 - 1357; PMID: 18809807
- Steinberg HO, Chaker H, Leaming R, Johnson A, Brechtel G, Baron AD. Obesity/insulin resistance is associated with endothelial dysfunction. Implications for the syndrome of insulin resistance. J Clin Invest 1996; 97:2601 - 2610; PMID: 8647954; http://dx.doi.org/10.1172/JCI118709.
- Sivitz WI, Wayson SM, Bayless ML, Sinkey CA, Haynes WG. Obesity impairs vascular relaxation in human subjects: hyperglycemia exaggerates adrenergic vasoconstriction arterial dysfunction in obesity and diabetes. J Diabetes Complications 2007; 21:149 - 157; PMID: 17493547; http://dx.doi.org/10.1016/j.jdiacomp.2005.12.003
- Hall JE. The kidney, hypertension and obesity. Hypertension 2003; 41:625 - 633; PMID: 12623970; http://dx.doi.org/10.1161/01.HYP.0000052314.95497.78
- Sodhi K, Inoue K, Gotlinger KH, Canestraro M, Vanella L, Kim DH, et al. Epoxyeicosatrienoic acid agonist rescues the metabolic syndrome phenotype of HO-2-null mice. J Pharmacol Exp Ther 2009; 331:906 - 916; PMID: 19717790; http://dx.doi.org/10.1124/jpet.109.157545
- Peterson SJ, Kim DH, Li M, Positano V, Vanella L, Rodella LF, et al. The L-4F mimetic peptide prevents insulin resistance through increased levels of HO-1, pAMPK and pAKT in obese mice. J Lipid Res 2009; 50:1293 - 1304; PMID: 19224872; http://dx.doi.org/10.1194/jlr.M800610-JLR200
- Peterson SJ, Drummond G, Kim DH, Li M, Kruger AL, Ikehara S, et al. L-4F treatment reduces adiposity, increases adiponectin levels and improves insulin sensitivity in obese mice. J Lipid Res 2008; 49:1658 - 1669; PMID: 18426778; http://dx.doi.org/10.1194/jlr.M800046-JLR200
- Li M, Kim DH, Tsenovoy PL, Peterson SJ, Rezzani R, Rodella LF, et al. Treatment of obese diabetic mice with a heme oxygenase inducer reduces visceral and subcutaneous adiposity, increases adiponectin levels, and improves insulin sensitivity and glucose tolerance. Diabetes 2008; 57:1526 - 1535; PMID: 18375438; http://dx.doi.org/10.2337/db07-1764
- Hirsch J, Batchelor B. Adipose tissue cellularity in human obesity. Clin Endocrinol Metab 1976; 5:299 - 311; PMID: 1085232; http://dx.doi.org/10.1016/S0300-595X(76)80023-0
- Roncari DA, Lau DC, Kindler S. Exaggerated replication in culture of adipocyte precursors from massively obese persons. Metabolism 1981; 30:425 - 427; PMID: 7231183; http://dx.doi.org/10.1016/0026-0495(81)90174-8
- Lefterova MI, Lazar MA. New developments in adipogenesis. Trends Endocrinol Metab 2009; 20:107 - 114; PMID: 19269847; http://dx.doi.org/10.1016/j.tem.2008.11.005
- Gesta S, Tseng YH, Kahn CR. Developmental origin of fat: tracking obesity to its source. Cell 2007; 131:242 - 256; PMID: 17956727; http://dx.doi.org/10.1016/j.cell.2007.10.004
- Naldini L, Blomer U, Gallay P, Ory D, Mulligan R, Gage FH, et al. In vivo gene delivery and stable transduction of nondividing cells by a lentiviral vector. Science 1996; 272:263 - 267; PMID: 8602510; http://dx.doi.org/10.1126/science.272.5259.263
- Lecka-Czernik B, Rosen CJ, Kawai M. Skeletal aging and the adipocyte program: New insights from an “old” molecule. Cell Cycle 2010; 9:3648 - 3654; PMID: 20890120; http://dx.doi.org/10.4161/cc.9.18.13046
- Zafren K, Feldman J, Becker RJ, Williams SR, Weiss EA, Deloughery T. D-dimer is not elevated in asymptomatic high altitude climbers after descent to 5,340?m: the Mount Everest Deep Venous Thrombosis Study (Ev-DVT). High Alt Med Biol 2011; 12:223 - 227; PMID: 21962065; http://dx.doi.org/10.1089/ham.2010.1101
- Ohashi K, Kihara S, Ouchi N, Kumada M, Fujita K, Hiuge A, et al. Adiponectin replenishment ameliorates obesity-related hypertension. Hypertension 2006; 47:1108 - 1116; PMID: 16651465; http://dx.doi.org/10.1161/01.HYP.0000222368.43759.a1
- Wang ZV, Scherer PE. Adiponectin, cardiovascular function and hypertension. Hypertension 2008; 51:8 - 14; PMID: 17998473; http://dx.doi.org/10.1161/HYPERTENSIONAHA.107.099424
- Kondo H, Shimomura I, Matsukawa Y, Kumada M, Takahashi M, Matsuda M, et al. Association of adiponectin mutation with type 2 diabetes: a candidate gene for the insulin resistance syndrome. Diabetes 2002; 51:2325 - 2328; PMID: 12086969; http://dx.doi.org/10.2337/diabetes.51.7.2325
- Kadowaki T, Hara K, Yamauchi T, Terauchi Y, Tobe K, Nagai R. Molecular mechanism of insulin resistance and obesity. Exp Biol Med (Maywood) 2003; 228:1111 - 1117; PMID: 14610248
- Kotani K, Sakane N, Saiga K, Kurozawa Y. Leptin: adiponectin ratio as an atherosclerotic index in patients with type 2 diabetes: relationship of the index to carotid intima-media thickness. Diabetologia 2005; 48:2684 - 2686; PMID: 16261311; http://dx.doi.org/10.1007/s00125-005-0015-4
- Furukawa S, Fujita T, Shimabukuro M, Iwaki M, Yamada Y, Nakajima Y, et al. Increased oxidative stress in obesity and its impact on metabolic syndrome. J Clin Invest 2004; 114:1752 - 1761; PMID: 15599400
- Choo HJ, Kim JH, Kwon OB, Lee CS, Mun JY, Han SS, et al. Mitochondria are impaired in the adipocytes of type 2 diabetic mice. Diabetologia 2006; 49:784 - 791; PMID: 16501941; http://dx.doi.org/10.1007/s00125-006-0170-2
- Vanella L, Kim DH, Sodhi K, Barbagallo I, Burgess AP, Falck JR, et al. Crosstalk between EET and HO-1 downregulates Bach1 and adipogenic marker expression in mesenchymal stem cell derived adipocytes. Prostaglandins Other Lipid Mediat 2011; 96:54 - 62; PMID: 21821145; http://dx.doi.org/10.1016/j.prostaglandins.2011.07.005
- Keating A. Mesenchymal stromal cells. Curr Opin Hematol 2006; 13:419 - 425; PMID: 17053453; http://dx.doi.org/10.1097/01.moh.0000245697.54887.6f
- Vanella L, Kim DH, Asprinio D, Peterson SJ, Barbagallo I, Vanella A, et al. HO-1 expression increases mesenchymal stem cell-derived osteoblasts but decreases adipocyte lineage. Bone 2010; 46:236 - 243; PMID: 19853072; http://dx.doi.org/10.1016/j.bone.2009.10.012
- Yu F, Takahashi T, Moriya J, Kawaura K, Yamakawa J, Kusaka K, et al. Angiotensin-II receptor antagonist alleviates non-alcoholic fatty liver in KKAy obese mice with type 2 diabetes. J Int Med Res 2006; 34:297 - 302; PMID: 16866024
- Guzik TJ, Mangalat D, Korbut R. Adipocytokines—novel link between inflammation and vascular function?. J Physiol Pharmacol 2006; 57:505 - 528; PMID: 17229978
- Chevillotte E, Giralt M, Miroux B, Ricquier D, Villarroya F. Uncoupling protein-2 controls adiponectin gene expression in adipose tissue through the modulation of reactive oxygen species production. Diabetes 2007; 56:1042 - 1050; PMID: 17395745; http://dx.doi.org/10.2337/db06-1300
- Christodoulides C, Laudes M, Cawthorn WP, Schinner S, Soos M, O'Rahilly S, et al. The Wnt antagonist Dickkopf-1 and its receptors are coordinately regulated during early human adipogenesis. J Cell Sci 2006; 119:2613 - 2620; PMID: 16763196; http://dx.doi.org/10.1242/jcs.02975
- Kawai M, Mushiake S, Bessho K, Murakami M, Namba N, Kokubu C, et al. Wnt/Lrp/beta-catenin signaling suppresses adipogenesis by inhibiting mutual activation of PPARgamma and C/EBPalpha. Biochem Biophys Res Commun 2007; 363:276 - 282; PMID: 17888405; http://dx.doi.org/10.1016/j.bbrc.2007.08.088
- Bennett CN, Ross SE, Longo KA, Bajnok L, Hemati N, Johnson KW, et al. Regulation of Wnt signaling during adipogenesis. J Biol Chem 2002; 277:30998 - 31004; PMID: 12055200; http://dx.doi.org/10.1074/jbc.M204527200
- Ross SE, Hemati N, Longo KA, Bennett CN, Lucas PC, Erickson RL, et al. Inhibition of adipogenesis by Wnt signaling. Science 2000; 289:950 - 953; PMID: 10937998; http://dx.doi.org/10.1126/science.289.5481.950
- Okamura M, Kudo H, Wakabayashi K, Tanaka T, Nonaka A, Uchida A, et al. COUP-TFII acts downstream of Wnt/beta-catenin signal to silence PPARgamma gene expression and repress adipogenesis. Proc Natl Acad Sci USA 2009; 106:5819 - 5824; PMID: 19307559; http://dx.doi.org/10.1073/pnas.0901676106
- Ahn J, Lee H, Kim S, Ha T. Curcumin-induced suppression of adipogenic differentiation is accompanied by activation of Wnt/beta-catenin signaling. Am J Physiol Cell Physiol 2010; 298:1510 - 1516; PMID: 20357182; http://dx.doi.org/10.1152/ajpcell.00369.2009
- Kamei Y, Suganami T, Kohda T, Ishino F, Yasuda K, Miura S, et al. Peg1/Mest in obese adipose tissue is expressed from the paternal allele in an isoform-specific manner. FEBS Lett 2007; 581:91 - 96; PMID: 17182038; http://dx.doi.org/10.1016/j.febslet.2006.12.002
- Takahashi M, Kamei Y, Ezaki O. Mest/Peg1 imprinted gene enlarges adipocytes and is a marker of adipocyte size. Am J Physiol Endocrinol Metab 2005; 288:117 - 124; PMID: 15353408; http://dx.doi.org/10.1152/ajpendo.00244.2004
- Li M, Peterson S, Husney D, Inaba M, Guo K, Kappas A, et al. Long-lasting expression of HO-1 delays progression of type I diabetes in NOD mice. Cell Cycle 2007; 6:567 - 571; PMID: 17299269; http://dx.doi.org/10.4161/cc.6.5.3917
- Abraham NG, Kappas A. Pharmacological and clinical aspects of heme oxygenase. Pharmacol Rev 2008; 60:79 - 127; PMID: 18323402; http://dx.doi.org/10.1124/pr.107.07104
- Cao J, Inoue K, Sodhi K, Puri N, Peterson SJ, Rezzani R, et al. High-Fat Diet Exacerbates Renal Dysfunction in SHR: Reversal by Induction of HO-1-Adiponectin Axis. Obesity (Silver Spring) 2011; In press PMID: 22193921; http://dx.doi.org/10.1038/oby.2011.365
- Sodhi K, Puri N, Inoue K, Falck JR, Schwartzman ML, Abraham NG. EET agonist prevents adiposity and vascular dysfunction in rats fed a high fat diet via a decrease in Bach 1 and an increase in HO-1 levels. Prostaglandins Other Lipid Mediat 2011; In press PMID: 22209722; http://dx.doi.org/10.1016/j.prostaglandins.2011.12.004
- Hiwasa T, Fujiki H, Sugimura T, Sakiyama S. Increase in the synthesis of a Mr. 32,000 protein in BALB/c 3T3 cells treated with tumor-promoting indole alkaloids or polyacetates. Cancer Res 1983; 43:5951 - 5955; PMID: 6416671
- Kim DH, Burgess AP, Li M, Tsenovoy PL, Addabbo F, McClung JA, et al. Heme oxygenase-mediated increases in adiponectin decrease fat content and inflammatory cytokines tumor necrosis factor-alpha and interleukin-6 in Zucker rats and reduce adipogenesis in human mesenchymal stem cells. J Pharmacol Exp Ther 2008; 325:833 - 840; PMID: 18334666; http://dx.doi.org/10.1124/jpet.107.135285
- Kappas A, Drummond GS, Munson DP, Marshall JR. Sn-Mesoporphyrin interdiction of severe hyperbilirubinemia in Jehovah's Witness newborns as an alternative to exchange transfusion. Pediatrics 2001; 108:1374 - 1377; PMID: 11731664; http://dx.doi.org/10.1542/peds.108.6.1374
- Miller M, Cho JY, Pham A, Ramsdell J, Broide DH. Adiponectin and functional adiponectin receptor 1 are expressed by airway epithelial cells in chronic obstructive pulmonary disease. J Immunol 2009; 182:684 - 691; PMID: 19109202
- Abraham NG, Kushida T, McClung J, Weiss M, Quan S, Lafaro R, et al. Heme oxygenase-1 attenuates glucose-mediated cell growth arrest and apoptosis in human microvessel endothelial cells. Circ Res 2003; 93:507 - 514; PMID: 12933701; http://dx.doi.org/10.1161/01.RES.0000091828.36599.34
- Chang SH, Barbosa-Tessmann I, Chen C, Kilberg MS, Agarwal A. Glucose deprivation induces heme oxygenase-1 gene expression by a pathway independent of the unfolded protein response. J Biol Chem 2002; 277:1933 - 1940; PMID: 11707454; http://dx.doi.org/10.1074/jbc.M108921200
- Chang SH, Garcia J, Melendez JA, Kilberg MS, Agarwal A. Haem oxygenase 1 gene induction by glucose deprivation is mediated by reactive oxygen species via the mitochondrial electron-transport chain. Biochem J 2003; 371:877 - 885; PMID: 12585963; http://dx.doi.org/10.1042/BJ20021731
- Kruger AL, Peterson S, Turkseven S, Kaminski PM, Zhang FF, Quan S, et al. D-4F induces heme oxygenase-1 and extracellular superoxide dismutase, decreases endothelial cell sloughing, and improves vascular reactivity in rat model of diabetes. Circulation 2005; 111:3126 - 3134; PMID: 15939814; http://dx.doi.org/10.1161/CIRCULATIONAHA.104.517102
- Quan S, Kaminski PM, Yang L, Morita T, Inaba M, Ikehara S, et al. Heme oxygenase-1 prevents superoxide anion-associated endothelial cell sloughing in diabetic rats. Biochem Biophys Res Commun 2004; 315:509 - 516; PMID: 14766238; http://dx.doi.org/10.1016/j.bbrc.2004.01.086
- Abraham NG, Rezzani R, Rodella L, Kruger A, Taller D, Li Volti G, et al. Overexpression of human heme oxygenase-1 attenuates endothelial cell sloughing in experimental diabetes. Am J Physiol Heart Circ Physiol 2004; 287:2468 - 2477; PMID: 15284058; http://dx.doi.org/10.1152/ajpheart.01187.2003
- Kruger AL, Peterson SJ, Schwartzman ML, Fusco H, McClung JA, Weiss M, et al. Upregulation of heme oxygenase provides vascular protection in an animal model of diabetes through its antioxidant and antiapoptotic effects. J Pharmacol Exp Ther 2006; 319:1144 - 1152; PMID: 16959961; http://dx.doi.org/10.1124/jpet.106.107482
- Sambuceti G, Morbelli S, Vanella L, Kusmic C, Marini C, Massollo M, et al. Diabetes impairs the vascular recruitment of normal stem cells by oxidant damage, reversed by increases in pAMPK, heme oxygenase-1 and adiponectin. Stem Cells 2009; 27:399 - 407; PMID: 19038792; http://dx.doi.org/10.1634/stemcells.2008-0800
- Fasshauer M, Klein J, Neumann S, Eszlinger M, Paschke R. Adiponectin gene expression is inhibited by beta-adrenergic stimulation via protein kinase A in 3T3-L1 adipocytes. FEBS Lett 2001; 507:142 - 146; PMID: 11684087; http://dx.doi.org/10.1016/S0014-5793(01)02960-X
- Yachie A, Niida Y, Wada T, Igarashi N, Kaneda H, Toma T, et al. Oxidative stress causes enhanced endothelial cell injury in human heme oxygenase-1 deficiency. J Clin Invest 1999; 103:129 - 135; PMID: 9884342; http://dx.doi.org/10.1172/JCI4165
- Kawashima A, Oda Y, Yachie A, Koizumi S, Nakanishi I. Heme oxygenase-1 deficiency: the first autopsy case. Hum Pathol 2002; 33:125 - 130; PMID: 11823983; http://dx.doi.org/10.1053/hupa.2002.30217
- Poss KD, Tonegawa S. Reduced stress defense in heme oxygenase 1-deficient cells. Proc Natl Acad Sci USA 1997; 94:10925 - 10930; PMID: 9380736; http://dx.doi.org/10.1073/pnas.94.20.10925
- Di Noia MA, Van Driesche S, Palmieri F, Yang LM, Quan S, Goodman AI, et al. Heme oxygenase-1 enhances renal mitochondrial transport carriers and cytochrome C oxidase activity in experimental diabetes. J Biol Chem 2006; 281:15687 - 15693; PMID: 16595661; http://dx.doi.org/10.1074/jbc.M510595200
- Turkseven S, Kruger A, Mingone CJ, Kaminski P, Inaba M, Rodella LF, et al. Antioxidant mechanism of heme oxygenase-1 involves an increase in superoxide dismutase and catalase in experimental diabetes. Am J Physiol Heart Circ Physiol 2005; 289:701 - 707; PMID: 15821039; http://dx.doi.org/10.1152/ajpheart.00024.2005
- Kim JY, van de Wall E, Laplante M, Azzara A, Trujillo ME, Hofmann SM, et al. Obesity-associated improvements in metabolic profile through expansion of adipose tissue. J Clin Invest 2007; 117:2621 - 2637; PMID: 17717599; http://dx.doi.org/10.1172/JCI31021
- Sardana MK, Kappas A. Dual control mechanism for heme oxygenase: tin(IV)-protoporphyrin potently inhibits enzyme activity while markedly increasing content of enzyme protein in liver. Proc Natl Acad Sci USA 1987; 84:2464 - 2468; PMID: 3470805; http://dx.doi.org/10.1073/pnas.84.8.2464
- Koza RA, Nikonova L, Hogan J, Rim JS, Mendoza T, Faulk C, et al. Changes in gene expression foreshadow diet-induced obesity in genetically identical mice. PLoS Genet 2006; 2:81; PMID: 16733553; http://dx.doi.org/10.1371/journal.pgen.0020081
- Rosen ED, MacDougald OA. Adipocyte differentiation from the inside out. Nat Rev Mol Cell Biol 2006; 7:885 - 896; PMID: 17139329; http://dx.doi.org/10.1038/nrm2066