Abstract
BubR1 is an important component of the spindle assembly checkpoint, and deregulated BubR1 functions frequently result in chromosomal instability and malignant transformation. We recently demonstrated that BubR1 was modified by sumoylation, and that lysine 250 (K250) functions as the crucial site for this modification. BubR1 sumoylation was neither required for its activation nor for binding to kinetochores. However, ectopically expressed sumoylation-deficient BubR1 mutants were retained on the kintochores even after apparent chromosome congression. The kinetochore retention of the sumoylation-deficient mutant of BubR1 caused an anaphase delay coupled with premature sister chromatid separation. Moreover, BubR1 interacted with unphosphorylated Sgo1, and its sumoylation facilitated the interaction. BubR1 sumoylation was inversely associated with its acetylation during mitotic progression. Trichostatin A, a protein deacetylase inhibitor, significantly compromised BubR1 sumoylation. Combined, these results reveal that BubR1 sumoylation plays an important role in its timely removal from the kinetochores and the checkpoint inactivation, thus allowing normal anaphase entry and chromosome segregation.
Introduction
Aneuploidy, a major manifestation of chromosomal instability, is defined as a numerical abnormality of chromosomes in diploid cells. It is highly prevalent in a variety of human malignancies. Increased chromosomal instability is the major driving force for tumor development and progression. To suppress genomic instability during cell division, eukaryotic cells have evolved important molecular mechanisms commonly referred to as checkpoints. The spindle checkpoint ensures that cells with defective mitotic spindles or a defective interaction between the spindles and kinetochores do not initiate chromosomal segregation during mitosis. It is also conceivable that the spindle checkpoint needs to be timely inactivated after the completion of bi-orientation attachment of spindles to the kinetochores of congressed chromosomes. In fact, overexpression of Mad2 also induces chromosome missegregation, anueploidy formation and malignant transformation in murine models,Citation1 suggesting that persistent activation of the checkpoint also compromises nuclear division. To date, the molecular mechanism by which the spindle checkpoint is turned off at the metaphase/anaphase transition remains unclear.
BubR1 is an evolutionarily conserved protein that functions as a key component of the spindle assembly checkpoint, playing an essential role in mitosis and meiosis.Citation2,Citation3 BubR1 interacts directly with Cdc20,Citation4 an activator of the anaphase-promoting complex/cyclosome (APC/C), thereby inhibiting the activity of APC/CCdc20. Hyper-phosphorylated BubR1 and other components of the checkpoint machinery, including Bub1, Bub3, Mad1, Mad2 and CENP-E, are associated with unattached kinetochores.Citation5–Citation7 Although BubR1 and Mad2 appear to function in the same signaling pathway after spindle checkpoint activation, BubR1 is a much more potent inhibitor of APC/C than Mad2.Citation8 BubR1 also physically interacts with CENP-E, and their interaction is affected in cells resistant to certain chemicals that affect microtubule dynamics.Citation9 Homozygous deletion of BubR1 (Bub1b) results in embryonic lethality in mice.Citation10 BubR1+/− mouse embryonic fibroblasts contain a significantly reduced amount of securin and Cdc20 and exhibit a greater level of micronuclei than wild-type cells do.Citation11 BubR1 insufficiency also causes infertility as well as phenotypes characteristic of early aging.Citation12,Citation13
Given its importance in the regulation of mitotic progression, BubR1 expression and activity are subjected to tight regulation during the cell cycle. At the protein level, BubR1 is modified by several types of post-translational modification.Citation14,Citation15 BubR1 is extensively phosphorylated on many sites.Citation15–Citation17 Plk1 plays an important role in phosphorylation of BubR1, although additional kinases including Cdk1 and Mps1 are also involved in its phosphorylation.Citation15–Citation17 Hyper-phosphorylated BubR1 as well as other components of the checkpoint machinery, including Bub1, Bub3, Mad1, Mad2 and CENP-E, are associated with unattached kinetochores and the stability of kinetochore microtubule interactions.Citation5,Citation6 A recent report shows that BubR1 is acetylated at lysine 250 during prometaphase.Citation14 This study also shows that acetylation-deficient mutant (K250R) is unstable, leading to accelerated mitotic exit.Citation14
In the current study, we describe BubR1 modification by sumoylation during late mitosis. Our functional analyses revealed that BubR1 sumoylation was not required for its binding to the kinetochores, and that K250 was a crucial site for its sumoylation. Different from the wild-type counterparts, ectopically expressed sumoylation-deficient BubR1 mutants were retained on metaphase kinetochores, causing an apparent delay in early anaphase as well as chromosome missegregation. We also observed that BubR1 sumoylation was inversely associated with its acetylation. Our study reveals sumoylation as a mechanism that inactivates BubR1-dependent spindle assembly checkpoint, thus allowing chromosome segregation and mitotic progression.
Results
BubR1 is modified by phosphorylation and acetylation.Citation14,Citation15 However, mitotic lysates contained an uncharacterized band with a slow mobility on denaturing blots that was immunoreactive to the BubR1 antibody. We named it a modified form of BubR1 (BubR1-M), as this band differed from the phosphorylated form (). The molecular weight of BubR1-M was about 170 kDa, approximately 40 kDa larger than the unphosphorylated, interphase form of BubR1. As sumoylation has been implicated as a mechanism that modifies BubR1,Citation18 we determined whether BubR1-M was derived from sumoylation. We first made a plasmid construct expressing an N-terminal fragment (610 amino acids) of BubR1 fused in-fame with both His6 and HA tags (termed His6-HA-N-Wt) (), as this fragment fully retains the checkpoint function.Citation3,Citation19 We co-transfected the plasmid construct with FLAG-tagged SUMO-1 expression construct. Immunoblotting with the anti-tag antibodies confirmed that His6-HA-BubR1 and FLAG-SUMO-1 were efficiently expressed (, input). Moreover, a specific band that migrated at about 107 kDa (arrows His6-HA-N-M) was significantly enriched by nickel resin and detected by antibodies to both FLAG-tag and HA-tag in cells transfected with His6-HA-N-Wt construct, strongly suggesting that BubR1 is SUMO-1-modified. As lysine 250 (K250) is one of the three residues that are optimal for sumoylation according to the criteria available (www.abgent.com), we made and transfected His6 and HA tagged BubR1 mutant fragment with K250 replaced with arginine (termed His6-HA-N-K250R). No significant sumoylated signals were detected, although the mutant protein was expressed at a comparable level to the wild-type counterpart (), supporting the notion that BubR1 is modified by sumoylation. The mutation analysis also indicated that BubR1 sumoylation either occurred at K250, or it was crucial for BubR1 sumoylation.
To further confirm that BubR1 is SUMO-modified and that K250 is important for BubR1 sumoylation, we made a series of plasmid constructs expressing HA-tagged full-length BubR1 as well as two corresponding mutants designated 2R and 3R by replacing either K5 and K769 or K5, K250 and K769 with arginines (). HA-tagged wild-type BubR1, BubR1–2R or BubR1-3R was transfected into HeLa cells that constitutively expressed His6-SUMO-1. Immunoblotting with the anti-HA tag antibody revealed that both mutant proteins as well as wild-type BubR1, were efficiently expressed (). Abundant BubR1-M was detected in cells transfected with wild-type HA-BubR1 and HA-BubR1–2R constructs, whereas little signal was present in cells transfected with HA-BubR1–3R, consistent with the notion that K250 is a crucial site for BubR1 sumoylation. Further supporting this, the anti-HA antibody detected BubR1-M in cells ectopically expressing HA-BubR1 and HA-BubR1–2R but not in cells expressing HA-BubR1–3R (, long exposure).
To understand the biological function of BubR1 sumoylation during the cell cycle, we first analyzed the level of BubR1 sumoylation during mitotic release in the presence of nocodazole and/or caffeine. Caffeine was used because of its ability to increase BubR1-M when cells were treated with a mitotic inducer (data not shown), although the mechanism remained unclear. At present, we cannot exclude the possibility that ATM and/ATR may play a role. A comparison with a part of mitotic markers revealed that sumoylated BubR1 was neither closely correlated with the activation status of the spindle checkpoint (phospho-Cdc27) nor with the levels of mitotic markers, including p-H3S10 and cyclin B (). The peak level of BubR1 sumoylation was instead associated with reduced levels of phospho-Cdc27 and p-H3S10 (). Given that a prolonged nocodazole treatment elicits adaptation and slippage through mitosis, these results are consistent with the notion that BubR1 sumoylation may promote mitotic exit.
To further understand the function of sumoylation in the regulation of BubR1 activity and mitotic progression, we analyzed cells ectopically expressing GFP-tagged BubR1 N-Wt or its mutant counterpart, N-K250R (), via fluorescence microscopy. Both GFP-tagged BubR1 N-Wt and N-K250R localized normally to kinetochores during prometaphase (). However, a significant fraction of metaphase-like cells (based on DAPI staining) expressing BubR1 N-K250R contained anaphase-like centromeres manifested as two aligned yet separated (“splitting”) CREST signals ( and D). Many metaphase-like cells also contained visible BubR1 N-K250R signals that co-localized with CREST (an example shown in ; arrow in upper right part). These observations suggest a case of “early anaphase delay” in which aligned sister chromatids attempt to segregate, but the segregation is unable to proceed normally due to the presence of sumoylation-deficient BubR1 on the kinetochores. Time-lapse confocal microscopy further confirmed that GFP-N-K250R, but not GFP-N-Wt, persistently localized at the congressed chromosomes, which was associated with delayed progression through mitosis (; Sup. Movies 1 and 2).
Chromosome spread analysis showed that whereas BubR1 N-Wt signals on spread chromosomes were hardly detected, BubR1 N-K250R signals on the kinetochores were heavily present and co-localized with CREST (), consistent with the notion that sumoylation-deficient BubR1 N-K250R may not be recognized for its dissociation from the kinetochores or may have a stronger binding affinity to the kinetochores than the wild-type counterpart does. Sister kinetochores were not separated in cells expressing BubR1 N-Wt; however, centromeric cohesion was disrupted in cells expressing BubR1 N-K250R, leading to premature sister chromatid separation ( and C). Intriguingly, many sister chromatids were separated at the centromeres, while some of their telomeres remained linked, resulting in the formation of a linear chromosome with two centromeres (CREST signals) (, examples shown in inlets I and II).
The above study strongly suggests that ectopically expressed BubR1 N-K250R was capable of functioning in a dominant-negative manner. To determine whether this mutant was unable to complement phenotypes such as premature sister chromatid separation caused by BubR1 deficiency,Citation20 we knocked down endogenous BubR1, but not ectopically expressed BubR1 GFPN-Wt and BubR1 GFP-N-K250R by co-transfection of siRNAs corresponding to 3′-untranslated region (3′UTR) of BubR1 transcript (). Chromosomal spread analysis revealed that GFP-N-Wt, but not GFP-N-K250R, significantly suppressed premature sister chromatid separation caused by depletion of endogenous BubR1 ( and C). Again, the functional deficiency of GFP-N-K250R mutant was associated with its significant retention at the kinetochores ().
To minimize the effect of BubR1 truncation on mitotic progression and to further confirm the role of BubR1 sumoylation in mediating mitotic progression, we expressed a GFP-tagged fulllength BubR1 mutant with K250 substituted with R (BubR1-K250R) or the wild-type counterpart (BubR1) as control (refer to for schematic presentation). Fluorescence microscopy revealed that abundant BubR1-K250R, but not BubR1, was retained at the kinetochores during metaphase (). Ectopic expression of BubR1-K250R mutant resulted in centromere splitting manifested as two lines of CREST signals in apparent metaphase chromosomes (). Further analysis via time-lapse confocal microscopy confirmed that cells expressing BubR1-K250R experienced difficulty in progressing through anaphase ().
To study whether sumoylation-deficient BubR1 was capable of rescuing major phenotypes induced as the result of depletion of endogenous BubR1, we co-transfected siRNA corresponding to BubR1 3′UTR region and GFP-BubR1 or GFP-BubR1-K250R expression construct. Ectopic expression of GFP-tagged full-length BubR1 suppressed premature separation of sister chromatids manifested as two discrete, closely aligned BubR1 foci for each sister chromatid pair; on the other hand, expressed GFP-BubR1-K250R was usually detected as a single dot correlated with prematurely separated sister chromatids (). These observations strongly suggest that that sumoylation-deficient full-length BubR1-K250R was unable to rescue phenotypes induced by depletion of endogenous BubR1.
To identify molecular components whose function might be affected by the BubR1 sumoylation status, we first examined the induction of BubR1-M after downregulation of Sgo1, CENP-E or KIF18a, because these molecules either functionally or physically interact with BubR1 during chromosome congression and/or segregation.Citation21–Citation24 Transfection of Sgo1 or CENP-E siRNA for 24 h induced both sumoylated (arrow BubR1-M) and phosphorylated (arrow p-BubR1) BubR1 (). Rounded-up cells induced by silencing KIF18a (a plus-end mitotic motor) contained some phosphorylated BubR1 but not sumoylated BubR1. BubR1 sumoylation, as well as its phosphorylation, was associated with mitotic arrest manifested as the induction of phosphorylated Eg5 and Mps1 and enhanced accumulation of KIF18a, Aurora B and Plk1 proteins (). As CENP-E physically interacts with BubR1Citation22 and is modified by sumoylation,Citation18 we examined its kinetochore localization in cells expressing BubR1 N-K250R. CENP-E staining was detected in prometaphase but not post-metaphase cells that expressed BubR1 sumoylation-resistant mutant (Fig. S1), suggesting that the phenotypes induced by the BubR1 mutant may not be due to a significant perturbation of CENP-E subcellular localization or its function.
We then determined whether BubR1 binds to Sgo1, as the latter is a protector for sister chromatid cohesion during mitosis.Citation21,Citation25–Citation27 Sgo1 IgG, but not the control IgG, brought down BubR1 from both interphase and mitotic cells (), suggesting the physical interaction between these proteins. To determine whether the physical interaction was affected by the sumoylation status of BubR1, we made and transfected plasmids expressing His6-tagged BubR1 N-Wt or His6-tagged BubR1 N-K250R () into HeLa cells for 48 h. Ectopic expression of BubR1 N-Wt or its mutant counterpart did not significantly alter phosphorylation of endogenous BubR1 or Sgo1 (). Expression of transfected BubR1 N-Wt and its mutant was confirmed by Ni-NTA resin pull-down assays. Moreover, His6-BubR1 N-Wt, but not its sumoylation-deficient mutant, was capable of coprecipitating Sgo1 (). Combined, these studies strongly suggest that BubR1 may interact preferentially with interphase, unphosphorylated Sgo1 via its N-terminal domain, and that its sumoylation may play a role in mediating the interaction.
As K250 of BubR1 was also reported to be a site of acetylation during the cell cycle,Citation14 we examined the kinetics of BubR1 acetylation and sumoylation during mitotic release. Mitotic cells collected after nocodazole treatment for 14 h were released into fresh medium. Immunoblotting revealed that BubR1 acetylation on K250 was inversely correlated with BubR1 sumoylation (). Since trichostatin A (TSA) functions as a broad spectrum inhibitor for deacetylation, we treated mitotic cells with TSA during their release. TSA was effective in our experimental system, as it significantly increased the level of acetylated histones (). Compared with the untreated control or cells treated with nocodazole alone during release, TSA significantly compromised the accumulation of sumoylated BubR1 (). The decreased level of sumoylated BubR1 was associated with increased interphase, non-phosphorylated BubR1, suggesting that TSA promotes mitotic exit. Supporting this notion, cyclin B levels were decreased at a much accelerated rate compared with the untreated control.
Discussion
Based on our results and those reported in the literature, we propose the following model to explain molecular regulation of BubR1 during mitotic progression (). BubR1 protein level and its activity are regulated by several types of posttranslational modifications, including phosphorylation and acetylation, leading to its association with sister kinetochores and the maintenance of the spindle checkpoint during early mitosis (prophase to prometaphase). After all chromosomes align at the metaphase plate, BubR1 sumoylation takes place, a modification required for its removal from the kinetochores. Inactivation of the BubR1-dependent spindle checkpoint leads to the cleavage of the cohesin complex and subsequent chromosomal segregation. Sumoylation-deficient BubR1 mutant proteins remain bound to the kinetochores, which deregulates coordinated checkpoint inactivation and anaphase entry, leading to “early anaphase delay” and chromosome missegregation.
Our current study demonstrates that BubR1 is modified via sumoylation, and that BubR1 sumoylation plays a role in regulating chromosome segregation and mitotic timing. BubR1 sumoylation is inversely correlated with its acetylation, and inhibition of acetylation by treatment with HDAC inhibitor significantly compromises BubR1 sumoylation during mitotic release ( and B). Given that lysine residues are the targets of several post-translational modifications including sumoylation and acetylation, it is conceivable that sumoylation and acetylation have opposing effects in regulating BubR1 activity through competing for overlapping target residues. In fact, we have recently observed that BubR1 sumoylation is closely associated BubR1 degradation.Citation28 Further supporting this notion, it has been shown that proteolysis is required for inactivation of the spindle assembly checkpoint.Citation29 Our molecular studies strongly suggest that K250 of BubR1 is a crucial site for sumoylation, as mutation of this residue disrupts SUMO-1 modification. A small amount of SUMO-1-modified signal is detected in cells transfected with BubR1 K250 mutants (), perhaps due to the possibility of mutation of a bona fide site, inducing spurious sumoylation at a non-canonical site at a much reduced efficiency.
A recent study reported that BubR1 is acetylated on K250, which is proposed to stabilize the checkpoint protein before anaphase entry.Citation14 This conclusion was largely based on the observations that the acetylated form of BubR1, detected by an acetylation-specific antibody, decreases during mitotic release, and that BubR1-K250R is unstable due to enhanced degradation mediated by APC/CCdc20.Citation14 However, this study and others failed to detect sumoylated BubR1. The finding that acetylated BubR1 peaks at prometaphase prompted the authors to speculate that BubR1-dependent checkpoint inactivation and BubR1 degradation at late mitosis would be attributed to or initiated at its de-acetylation. This study also reported that Myc-tagged K250R mutant was non-detectable when it was ectopically expressed.Citation14 This observation is different from ours that BubR1 K250R mutant proteins are rather stable and highly enriched at the kinetochores. It is likely that Myc antibody may have failed to detect the tagged mutant protein of BubR1 in their experimental system. Nevertheless, the phenotypes, including anaphase delay and chromosome missegregation, that are induced by BubR1 K250R mutant can be the consequence of deregulated acetylation and sumoylation.
Sumoylation is known to play an essential role in chromosome dynamics during mitosis.Citation30,Citation31 Our study reveals an unrecognized role for BubR1 in regulating chromosome dynamics during mitosis. It appears that BubR1 sumoylation status does not significantly affect chromosome condensation and congression during early mitosis. Rather, it plays a role in turning off the checkpoint signal and allowing proper chromosome segregation. The prolonged localization of SUMO-deficient mutants of BubR1 on the kinetochores may perturb metaphase and early anaphase processes, including chromosome oscillation and microtubule dynamics at the plus end. The phenotype of “early anaphase delay” caused by expression of sumoylation-deficient mutants is unlikely to be due to a dominant-negative effect of the mutant, because haplo-insufficiency of BubR1/Bub1b or weakened BubR1 function results in mitotic slippage.Citation11,Citation32
Another intriguing phenotype revealed by studying BubR1 SUMO-resistant mutants is its involvement in telomere cohesion. This is entirely unexpected, because arm cohesion is thought to be removed during early mitosis via a Plk1-dependent process.Citation33 The peculiar arrangement of two aligned CREST signals (reflecting core centromeric proteins) suggests an early anaphase status; however, chromosomes appear rather condensed and are metaphase-like ( and ). One possibility is that paired sister centromeres are stretched. Chromosome spread analyses support the notion that centromeric cohesion is either weakened or completely abolished, whereas many sister telomeres are end-to-end linked, leading to formation of “ring” chromosomes or dicentric chromosomes (). Another possibility, albeit remote, is that DNA endo-reduplication may somehow give rise to the observed patterns of chromosomes. However, depletion of Sgo1 or Bub1 can also induce the formation of abnormal chromosome patterns, with sister chromatids separated at centromeres but remained attached at one or both arms,Citation23 suggesting the existence of an unrecognized mechanism that coordinates telometric/arm and centromeric cohesion. The role of BubR1 in the maintenance of telomere cohesion is entirely unknown, as BubR1 binds primarily to unpaired kinetochores. Several recent studies support a role of spindle checkpoint proteins, including BubR1 in non-centromeric function. BubR1 and Polo that are found to decorate the so-called “tether” of acentric chromosomes play an essential role in mediating accurate segregation of sister chromatids,Citation34 suggesting that BubR1 is capable of binding to chromosomes at locations other than the centromeres. In addition, unprotected telomeres also activate the spindle checkpoint in Drosophila cells.Citation35 Furthermore, BubR1 and Mad2 colocalize with TRF1, a component of the shelterin complex at telomeres, in mouse epithelial cells with targeted expression of TRF1.Citation36 Interestingly, these cells also exhibit an increase in end-to-end chromosome fusions. TRF1 transgenic mice have shorter telomeres in the epidermis than wild-type controls,Citation36 suggesting a possibility of spindle checkpoint proteins in the regulation of telomere length and cellular aging. Consistent with this possibility, a reduction of BubR1 expression in mouse embryonic fibroblasts leads to increased senescence as well as aneuploidy, and BubR1-deficiency results in premature aging in mice, which is associated with early appearance of many progeroid features and short lifespan.Citation12 Therefore, our current study may provide a new insight into the molecular and cellular basis by which BubR1 regulates telomere integrity, cellular senescence and aging as well as chromosomal stability during cell division.
Materials and Methods
Cell culture.
HeLa and U2OS cell lines were obtained from the American Type Culture Collection. HeLa cell line constitutively expressing His6-SUMO-1 was kindly provided by Dr. Ronald Hay (University of Dundee). Cells were cultured in DMEM supplemented with 10% fetal bovine serum (FBS, Invitrogen) and antibiotics (100 µg/mL of penicillin and 50 µg/mL of streptomycin sulfate, Invitrogen) at 37°C under 5% CO2. Mitotic shake-off cells were obtained from gentle tapping of either normally growing mitotic (rounded up) cells or cells treated with nocodazole (50 ng/mL) (Sigma-Aldrich) for 14 h. Both types of shake-off cells were used for mitotic release in the presence or absence of nocodazole (or taxol) and/or caffeine (3 mM, Sigma-Aldrich) as specified in each experiment.
Antibodies.
Antibodies to HA, p-H3S10 and β-actin were purchased from Cell Signaling Technology Inc. Rabbit polyclonal antibodies for BubR1 and Sgo1 were developed in the laboratory. GFP, SUMO-1 and Cdc27 antibodies were purchased from Santa Cruz Biotechnology. Rabbit anti-ubiquitin antibodies were from Abcam. Mouse anti-FLAG antibody was purchased from Sigma-Aldrich. Human IgGs against centromere proteins (CREST) were purchased from Antibodies Inc. The antibody that was raised against acetylated K250 of BubR1 was kindly provided by Dr. Hyunsook Lee at Seoul National University.
Plasmids, mutagenesis and transfection.
The original plasmid for cloning full-length BubR1 expression plasmid or making BubR1 deletion constructs was described in reference Citation37. An N-terminal fragment (610 a.a.) of BubR1 corresponding to the caspase 3-cleaved fragmentCitation38 was cloned into a GFP- or His6-HA-expression plasmid. BubR1 mutations at lysine 5 (K5), K769 and/or K250 were performed using the QuickChange Lightning Multi Site-Directed Mutagenesis Kit (Strategene) using full-length BubR1 or the N-terminal fragment as a template. Individual mutations were confirmed by DNA sequencing. BubR1 and its truncated fragment were expressed as HA- or GFP-tagged fusion proteins. HA-UBC9 and His6-SUMO-1 plasmids were purchased from Addgene. SENP-1 and its mutant expression plasmids were kindly provided by J. Cheng.Citation39 BubR1 3′-UTR siRNA (5′-GTC TCA CAG ATT GCT GCC T-3′) and luciferase control siRNA were purchased from Dharmacon RNAi Technology. Transfection of plasmids or siRNAs was performed using Lipofectamine 2000 according to the supplier's instruction (Invitrogen).
Western blot.
SDS-PAGE was performed using the mini gel system from Bio-Rad. Proteins were transferred to PVDF membranes. After blocking with TBST containing 5% non-fat dry milk for 1 h, membranes were incubated overnight with primary antibodies, followed by incubation with horse-radish peroxidase-conjugated secondary antibodies (Cell Signaling Technology) for 1 h at room temperature. After thorough washing with TBST buffer, signals were developed with an enhanced chemiluminescent system (Pierce).
Pull-down assays.
HeLa cells transfected with various expression plasmids were lysed. Ni2+-NTA-agarose resin (Qiagen) was then added to the cell lysates and incubated with gentle agitation at 4°C overnight. The resin was successively washed at room temperature with four different buffers (washing buffer 1: 6 M guanidine-HCl, 0.1 M Na2HPO4/NaH2PO4, 0.01 M Tris/HCl, pH 8.0 plus 10 mM β-mercaptoethanol; washing buffer 2: 8 M urea, 0.1 M Na2HPO4/NaH2PO4, 0.01 M Tris/HCl, pH 8.0, 10 mM β-mercaptoethanol; washing buffer 3: 8 M urea, 0.1 M Na2HPO4/NaH2PO4, 0.01 M Tris/HCl, pH 6.3, 10 mM β-mercaptoethanol plus 0.2% Triton X-100; washing buffer 4: buffer A plus 0.1% Triton X-100). After last wash, His6-tagged products were eluted in the following buffer (200 mM imidazole, 0.15 M Tris/HCl pH 6.7, 30% glycerol, 0.72 M β-mercaptoethanol, 5% SDS) and western-blotting for BubR1, the HA tag and the FLAG tag was conducted.
Fluorescence microscopy.
Fluorescence microscopy was performed as described in our early studies in reference Citation40. Briefly, HeLa cells seeded on chamber slides were transfected with various expression constructs for 48 h. At the end of transfection, cells were fixed with 4% paraformaldehyde in PBS for 20 min at RT. After permeabilization by incubation with 0.5% Triton X-100 in PBS for 20 min, cells were incubated with 2% bovine serum albumin (BSA) in PBS for 1 h, followed by incubating overnight with antibodies to CREST and with Alex Fluor 555-conjugated goat anti-human IgG (Invitrogen) for 1 h. Cellular DNA was stained with 4′,6-diamidino-2-phenylindole (DAPI, Molecular Probe). Fluorescence signals were detected on a Leica TCS SP5 confocal microscope or on a Leica AF6000 fluorescence microscope.
To obtain mitotic chromosome spreads, cells transfected with various expression constructs were collected after Trypsin-EDTA treatment and incubated in 3.7 mM KCl at 37°C for 12 min. Cells were suspended in a fixative solution (glacial acetic acid/methanol in a ratio of 1:3) prior to spreading onto microscope slides (Fisher Scientific). Chromosome spreads were incubated overnight with antibodies to GFP and CREST. After incubation with Alex Fluor 488-conjugated goat anti-mouse IgG and Alex Fluor 555-conjugated goat anti-human IgG, cells were counterstained with DAPI. Chromosomal images were captured with a Leica TCS SP5 confocal microscope or a Leica AF6000 fluorescence microscope. For time-lapse video imaging, HeLa cells seeded onto chamber slides were transfected with various expression constructs for 24 h. The transfected cells were then cultured in a humidified chamber at 37°C in CO2-independent medium (GIBCO-BRL). Cells ectopically expressing transfected plasmids were subjected to time-lapse video imaging on a Leica TCS SP5 confocal microscope.
Statistical analysis.
Student t-test was used to evaluate the difference between two groups. A value of p < 0.05 was considered to be statistically significant.
Disclosure of Potential Conflicts of Interest
No potential conflicts of interest were disclosed.
Figures and Tables
Figure 1 BubR1 is modified by sumoylation. (A) HeLa cells were cultured in the presence or absence of nocodazole (Noc, 40 ng/mL) for 18 h. Equal amounts of cell lysates were blotted for BubR1 and β-actin. BubR1 (arrow BubR1), phosphorylated BubR1 (arrow p-BubR1) and modified BubR1 (arrow BubR1-M) are indicated. (B) Schematic presentation of various plasmid constructs expressing tagged BubR1 or its mutants used in this study. (C) HeLa cells were co-transfected with FLAG-SUMO-1 and His6-HA-N-Wt expression plasmids or with FLAG-SUMO-1 and His6-HA-N-K250R expression plasmids for 48 h. Ectopically expressed proteins were enriched by incubation with Ni-NTA resin and analyzed, along with lysate inputs, by western blotting using antibodies to both HA and FLAG tags. His6-HA-N-M denotes the sumoylated BubR1 N-terminal fragment. (D) HeLa cells constitutively expressing His6-SUMO-1 were transfected with a plasmid construct expressing HA-BubR1, HA-BubR1–2R or HA-BubR1–3R for 48 h. Equal amounts of cell lysates were then blotted for BubR1 and HA-tag. Both short and long exposures of the blot probed with the anti-HA-tag antibody are shown. Immunoblots were also stained with Ponceau S solution (Sigma) to visualize loading.
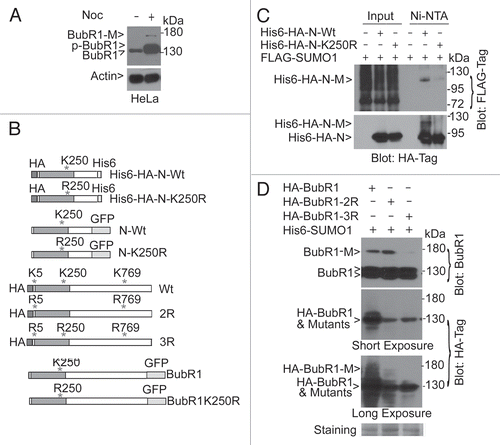
Figure 2 Expression of sumoylation-deficient mutant of BubR1 N-terminus induces early anaphase arrest. (A) HeLa cells treated with nocodazole for 14 h. Mitotic cells collected by shaking-off were re-cultured in fresh medium supplemented with or without nocodazole and/or caffeine for various times, as indicated. Equal amounts of cell lysates were blotted for BubR1, Cdc27, cyclin B, p-H3S10, histone and α-tubulin. (B) HeLa cells were transfected with a plasmid expressing either GFPtagged wild-type of N-terminal BubR1 fragment (N-Wt) or its mutant counterpart with K250 replaced with R (N-K250R). After 48 h transfection, HeLa cells were fixed and stained with antibodies to GFP and γ-tubulin. DNA was stained with DAPI. Representative prometaphase cells are shown. (C) HeLa cells transfected with a plasmid expressing N-Wt or N-K250R for 48 h were fixed and stained with antibodies to GFP and CREST. DNA was stained with DAPI. Representative metaphase (based on DNA patterns) cells are shown. Arrow denotes kinetochore N-K250R signals. (D) The percent of metaphase-like cells with split CREST signals was summarized from cells transfected with a plasmid expressing either N-Wt or N-K250R.
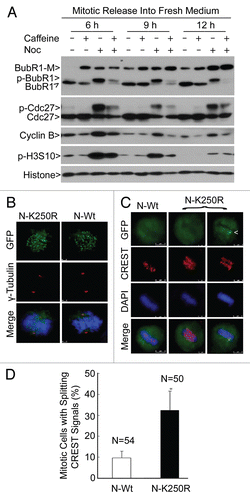
Figure 3 Sumoylation-resistant BubR1 is retained on kinetochores, inducing major defects in sister chromatid cohesion. (A) HeLa cells transfected with a plasmid expressing GFP-tagged N-Wt or N-K250R for 24 h were subjected to time-lapse confocal microscopy. Both DIC and GFP channels are shown for cells transfected with each expression plasmid. Please note the kinetochore localized GFP-N-K250R signals in GFP channel (arrow). (B) HeLa cells transfected with a plasmid expressing N-Wt or N-K250R for 48 h were subjected to chromosome spread analysis. Chromosome spreads were stained overnight with antibodies to GFP and CREST. DNA was stained with DAPI (blue in merged parts). Representative metaphase-like chromosomes are shown. Insets I and II are magnified versions of marked areas. (C) The percent of metaphaselike cells with separation of sister centromeres and/or end-to-end linkage of telomeres as shown in (A) was summarized from cells transfected with a plasmid expressing either N-Wt or N-K250R.
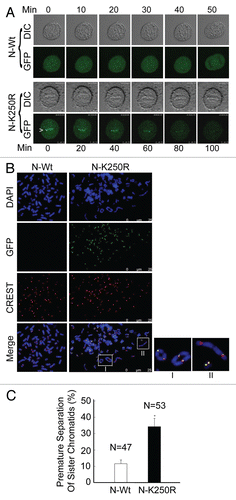
Figure 4 Sumoylation-deficient BubR1 fragment is unable to rescue premature sister chromatid segregation. (A) HeLa cells were co-transfected with BubR1 siRNAs corresponding to its 3′untranslated region (3′UTR) and a plasmid construct expressing GFP-N-Wt or GFP-N-K250R (or vector alone) for 48 h. Luciferase siRNAs were transfected as control. Equal amounts of cell lysates were blotted for BubR1 (top part), GFP (middle part) and β-actin, respectively. (B) HeLa cells were co-transfected with BubR1 siRNAs corresponding to its 3′UTR and a plasmid construct expressing GFP-N-Wt or GFP-N-K250R for 48 h. Transfected cells were subjected to chromosome spread analysis. Spread chromosomes were stained with antibodies to BubR1 (green) and Sgo1 (red). DNA was stained with DAPI (blue). Chromosome morphology was examined under a fluorescent microscope. Representative images are shown. (C) Percentage of mitotic cells with premature sister chromatid separation for each group as described in (B) was presented. Data were summarized from three independent experiments.
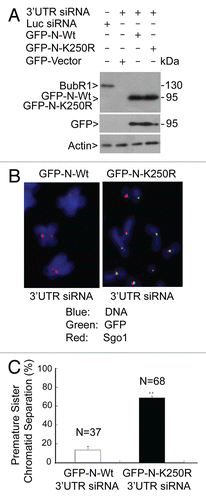
Figure 5 Sumoylation-deficient full-length BubR1 also induces mitotic defects. (A) HeLa cells seeded on chamber slides were transfected with a plasmid expressing either GFP-tagged full-length BubR1 or its mutant counterpart (BubR1 K250R). After 48 h transfection, HeLa cells were fixed and stained with antibody to GFP (green). DNA was stained with DAPI (blue). Representative metaphase or metaphase-like cells are shown. Arrow indicates enriched BubR1-K250R signals on kinetochores. (B) HeLa cells transfected with GFP-tagged full-length BubR1 or its mutant counterpart (BubR1 K250R) for 48 h were fixed and stained with the CREST antibody (Red). DNA was stained with DAPI (Blue). Representative metaphase (metaphase-like) cells are shown. (C) The duration of mitosis of each cell expressing GFP-tagged full-length BubR1 or its mutant counterpart (BubR1 K250R) was recorded by time-lapse confocal microscopy. The percentage of cells with average times (<1, 1–2.5 or >2.5 h) spent in mitosis is summarized. (D) HeLa cells were co-transfected with BubR1 siRNAs corresponding to its 3′UTR and a plasmid expressing either GFP-tagged full length BubR1 or its mutant counterpart (GFP-BubR1-K250R) for 48 h. Cells were then fixed and stained with the GFP antibody (green). DNA was stained with DAPI (blue). Representative images are shown. Please note doublet signals (for paired sister chromatids) in cells transfecfed with wild-type BubR1 vs. single signals on chromatids in cells transfected with the mutant counterpart.
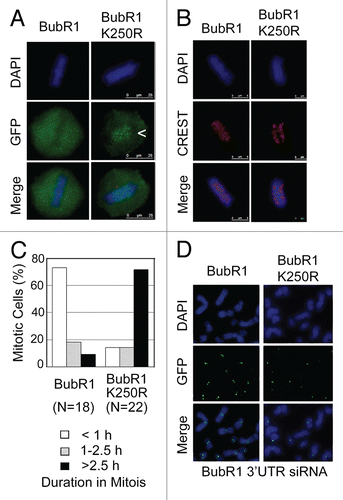
Figure 6 BubR1 interacts with Sgo1 and its interaction is sumoylation-dependent. (A) HeLa cells were transfected with Sgo1, CENP-E, KIF18a or luciferase (Luc) siRNAs for 24 h after which cells were collected and lysed. Cells transfected with KIF18a siRNA were collected as rounded-up (R after shake-off) and adherent (A after trypsin treatment) fractions. Equal amounts of cell lysates from various treatments were blotted for BubR1, Sgo1, CENP-E, KIF18a, Aurora B, Eg5, Mps1, Plk1 and Plk3. (B) HeLa cells treated with or without taxol for 24 h were collected and lysed. Equal amounts of cell lysates were immunoprecipitated with Sgo1 IgG or with control IgG. Immunoprecipitates, along cell lysate inputs, were blotted for BubR1. (B) Schematic representation of His6-tagged BubR1 N-terminal domain (610 amino acids) and its sumoylation-resistant mutant. (C) HeLa cells transfected with plasmid constructs expressing His6-N-Wt and His6-N-K250R or with the vector alone for 48 h were collected and lysed. Equal amounts of cell lysates were subjected to pull-down assays using Ni-NTA resin. Proteins specifically bound to the resin, along with lysate inputs were blotted for Sgo1 and BubR1.
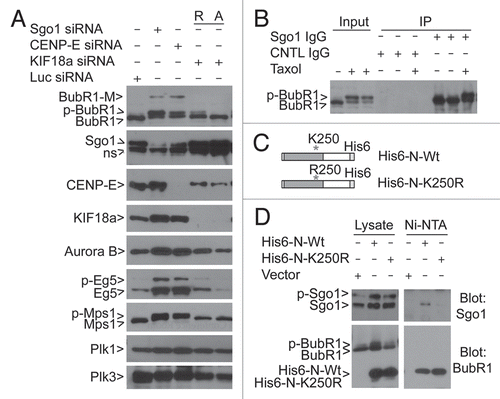
Figure 7 BubR1 sumoylation is associated with its biochemical inactivation. (A) Mitotic cells collected by shake-off after treatment with nocodazole for 14 h were released into fresh culture medium. At various times of release, cell lysates, along with interphase control ones, were blotted for BubR1 sumoylation and acetylation using an acetylation-specific antibody. Arrow Ac-BubR1 (K250) denotes K250-acetylated BubR1. (B) Mitotic cells collected by shake-off after treatment with nocodazole for 14 h were released into fresh culture medium supplemented with or without nocodazole or TSA. At various times of release as indicated, cell lysates along with interphase control ones were blotted for BubR1, cyclin B, acetylated histones and β-actin. (C) A model depicting the molecular regulation of BubR1 during mitosis. A, P and S denote acetylation, phosphorylation and sumoylation, respectively.
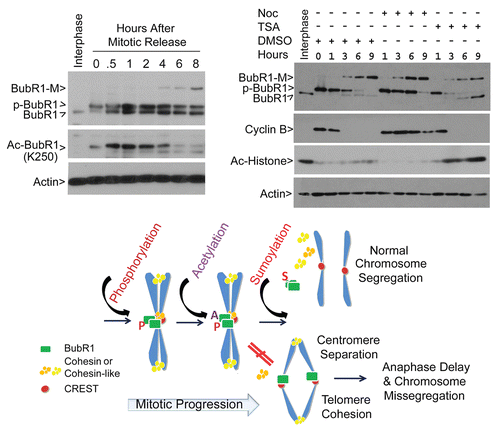
Additional material
Download Zip (5.5 MB)Acknowledgements
We thank Drs. Michele Pagano and Alexey Khodjakov for valuable discussions and suggestions. We thank Drs. Jingke Cheng and Jianxiu Yu at Shanghai Jiaotong University School of Medicine for providing us with His6-SUMO1 expression constructs and valuable discussions during the study, respectively. We thank Dr. Hyunsook Lee for BubR1 acetylation-specific antibody. This study was supported in part by US Public Service Awards to W.D. (CA090658 and CA113349) and NIEHS Center grant (ES000260).
References
- Sotillo R, Hernando E, Díaz-Rodríguez E, Teruya-Feldstein J, Cordón-Cardo C, Lowe SW, et al. Mad2 overexpression promotes aneuploidy and tumorigenesis in mice. Cancer Cell 2007; 11:9 - 23; PMID: 17189715; http://dx.doi.org/10.1016/j.ccr.2006.10.019
- Fan HY. BubR1, a spindle assembly checkpoint protein in mammalian oocyte meiosis. Cell Cycle 2010; 9:1458; PMID: 20421710; http://dx.doi.org/10.4161/cc.9.8.11405
- Harris L, Davenport J, Neale G, Goorha R. The mitotic checkpoint gene BubR1 has two distinct functions in mitosis. Exp Cell Res 2005; 308:85 - 100; PMID: 15907836; http://dx.doi.org/10.1016/j.yexcr.2005.03.036
- Wu H, Lan Z, Li W, Wu S, Weinstein J, Sakamoto KM, et al. p55CDC/hCDC20 is associated with BUBR1 and may be a downstream target of the spindle checkpoint kinase. Oncogene 2000; 19:4557 - 4562; PMID: 11030144; http://dx.doi.org/10.1038/sj.onc.1203803
- Yu H. Regulation of APC-Cdc20 by the spindle checkpoint. Curr Opin Cell Biol 2002; 14:706 - 714; PMID: 12473343; http://dx.doi.org/10.1016/S0955-0674(02)00382-4
- Yu H. Cdc20: a WD40 activator for a cell cycle degradation machine. Mol Cell 2007; 27:3 - 16; PMID: 17612486; http://dx.doi.org/10.1016/j.molcel.2007.06.009
- Logarinho E, Bousbaa H. Kinetochore-microtubule interactions “in check” by Bub1, Bub3 and BubR1: The dual task of attaching and signalling. Cell Cycle 2008; 7:1763 - 1768; PMID: 18594200; http://dx.doi.org/10.4161/cc.7.12.6180
- Fang G. Checkpoint protein BubR1 acts synergistically with Mad2 to inhibit anaphase-promoting complex. Mol Biol Cell 2002; 13:755 - 766; PMID: 11907259; http://dx.doi.org/10.1091/mbc.01-09-0437
- Yang CP, Liu L, Ikui AE, Horwitz SB. The interaction between mitotic checkpoint proteins, CENP-E and BubR1, is diminished in epothilone B-resistant A549 cells. Cell Cycle 2010; 9:1207 - 1213; PMID: 20237434; http://dx.doi.org/10.4161/cc.9.6.11122
- Wang Q, Liu T, Fang Y, Xie S, Huang X, Mahmood R, et al. BUBR1 deficiency results in abnormal megakaryopoiesis. Blood 2004; 103:1278 - 1285; PMID: 14576056; http://dx.doi.org/10.1182/blood-2003-06-2158
- Dai W, Wang Q, Liu T, Swamy M, Fang Y, Xie S, et al. Slippage of mitotic arrest and enhanced tumor development in mice with BubR1 haploinsufficiency. Cancer Res 2004; 64:440 - 445; PMID: 14744753; http://dx.doi.org/10.1158/0008-5472.CAN-03-3119
- Baker DJ, Jeganathan KB, Cameron JD, Thompson M, Juneja S, Kopecka A, et al. BubR1 insufficiency causes early onset of aging-associated phenotypes and infertility in mice. Nat Genet 2004; 36:744 - 749; PMID: 15208629; http://dx.doi.org/10.1038/ng1382
- Baker DJ, Perez-Terzic C, Jin F, Pitel K, Niederländer NJ, Jeganathan K, et al. Opposing roles for p16Ink4a and p19Arf in senescence and ageing caused by BubR1 insufficiency. Nat Cell Biol 2008; 10:825 - 836; PMID: 18516091; http://dx.doi.org/10.1038/ncb1744
- Choi E, Choe H, Min J, Choi JY, Kim J, Lee H. BubR1 acetylation at prometaphase is required for modulating APC/C activity and timing of mitosis. EMBO J 2009; 28:2077 - 2089; PMID: 19407811; http://dx.doi.org/10.1038/emboj.2009.123
- Huang H, Yen TJ. BubR1 is an effector of multiple mitotic kinases that specifies kinetochore: microtubule attachments and checkpoint. Cell Cycle 2009; 8:1164 - 1167; PMID: 19282664; http://dx.doi.org/10.4161/cc.8.8.8151
- Elowe S, Hümmer S, Uldschmid A, Li X, Nigg EA. Tension-sensitive Plk1 phosphorylation on BubR1 regulates the stability of kinetochore microtubule interactions. Genes Dev 2007; 21:2205 - 2219; PMID: 17785528; http://dx.doi.org/10.1101/gad.436007
- Huang H, Hittle J, Zappacosta F, Annan RS, Hershko A, Yen TJ. Phosphorylation sites in BubR1 that regulate kinetochore attachment, tension and mitotic exit. J Cell Biol 2008; 183:667 - 680; PMID: 19015317; http://dx.doi.org/10.1083/jcb.200805163
- Zhang XD, Goeres J, Zhang H, Yen TJ, Porter AC, Matunis MJ. SUMO-2/3 modification and binding regulate the association of CENP-E with kinetochores and progression through mitosis. Mol Cell 2008; 29:729 - 741; PMID: 18374647; http://dx.doi.org/10.1016/j.molcel.2008.01.013
- Malureanu LA, Jeganathan KB, Hamada M, Wasilewski L, Davenport J, van Deursen JM. BubR1 N terminus acts as a soluble inhibitor of cyclin B degradation by APC/C(Cdc20) in interphase. Dev Cell 2009; 16:118 - 131; PMID: 19154723; http://dx.doi.org/10.1016/j.devcel.2008.11.004
- Rao CV, Yang YM, Swamy MV, Liu T, Fang Y, Mahmood R, et al. Colonic tumorigenesis in BubR1+/−Apc−/+ compound mutant mice is linked to premature separation of sister chromatids and enhanced genomic instability. Proc Natl Acad Sci USA 2005; 102:4365 - 4370; PMID: 15767571; http://dx.doi.org/10.1073/pnas.0407822102
- Wang X, Dai W. Shugoshin, a guardian for sister chromatid segregation. Exp Cell Res 2005; 310:1 - 9; PMID: 16112668; http://dx.doi.org/10.1016/j.yexcr.2005.07.018
- Mao Y, Abrieu A, Cleveland DW. Activating and silencing the mitotic checkpoint through CENP-E-dependent activation/inactivation of BubR1. Cell 2003; 114:87 - 98; PMID: 12859900; http://dx.doi.org/10.1016/S0092-8674(03)00475-6
- Tang Z, Sun Y, Harley SE, Zou H, Yu H. Human Bub1 protects centromeric sister-chromatid cohesion through Shugoshin during mitosis. Proc Natl Acad Sci USA 2004; 101:18012 - 18017; PMID: 15604152; http://dx.doi.org/10.1073/pnas.0408600102
- Huang Y, Yao Y, Xu HZ, Wang ZG, Lu L, Dai W. Defects in chromosome congression and mitotic progression in KIF18A-deficient cells are partly mediated through impaired functions of CENP-E. Cell Cycle 2009; 8:2643 - 2649; PMID: 19625775; http://dx.doi.org/10.4161/cc.8.16.9366
- Kitajima TS, Kawashima SA, Watanabe Y. The conserved kinetochore protein shugoshin protects centromeric cohesion during meiosis. Nature 2004; 427:510 - 517; PMID: 14730319; http://dx.doi.org/10.1038/nature02312
- Wang X, Yang Y, Dai W. Differential subcellular localizations of two human Sgo1 isoforms: implications in regulation of sister chromatid cohesion and microtubule dynamics. Cell Cycle 2006; 5:635 - 640; PMID: 16582621; http://dx.doi.org/10.4161/cc.5.6.2547
- Yang Y, Wang X, Dai W. Human Sgo1 is an excellent target for induction of apoptosis of transformed cells. Cell Cycle 2006; 5:896 - 901; PMID: 16628005; http://dx.doi.org/10.4161/cc.5.8.2691
- Yang F, Hu L, Chen C, Yu J, O'Connell CB, Khodjakov A, et al. BubR1 is modified by sumoylation during mitotic progression. J Biol Chem 2011; In press PMID: 22167194
- Visconti R, Palazzo L, Grieco D. Requirement for proteolysis in spindle assembly checkpoint silencing. Cell Cycle 2010; 9:564 - 569; PMID: 20081372; http://dx.doi.org/10.4161/cc.9.3.10581
- Dawlaty MM, Malureanu L, Jeganathan KB, Kao E, Sustmann C, Tahk S, et al. Resolution of sister centromeres requires RanBP2-mediated SUMOylation of topoisomerase IIalpha. Cell 2008; 133:103 - 115; PMID: 18394993; http://dx.doi.org/10.1016/j.cell.2008.01.045
- Zhu JL, Lin SL, Li M, Ouyang YC, Hou Y, Schatten H, et al. Septin2 is modified by SUMOylation and required for chromosome congression in mouse oocytes. Cell Cycle 2010; 9:1607 - 1616; PMID: 20372094; http://dx.doi.org/10.4161/cc.9.8.11463
- Stevens FE, Beamish H, Warrener R, Gabrielli B. Histone deacetylase inhibitors induce mitotic slippage. Oncogene 2008; 27:1345 - 1354; PMID: 17828304; http://dx.doi.org/10.1038/sj.onc.1210779
- Hauf S, Roitinger E, Koch B, Dittrich CM, Mechtler K, Peters JM. Dissociation of cohesin from chromosome arms and loss of arm cohesion during early mitosis depends on phosphorylation of SA2. PLoS Biol 2005; 3:69; PMID: 15737063; http://dx.doi.org/10.1371/journal.pbio.0030069
- Royou A, Gagou ME, Karess R, Sullivan W. BubR1- and Polo-coated DNA tethers facilitate poleward segregation of acentric chromatids. Cell 2010; 140:235 - 245; PMID: 20141837; http://dx.doi.org/10.1016/j.cell.2009.12.043
- Musaro M, Ciapponi L, Fasulo B, Gatti M, Cenci G. Unprotected Drosophila melanogaster telomeres activate the spindle assembly checkpoint. Nat Genet 2008; 40:362 - 366; PMID: 18246067; http://dx.doi.org/10.1038/ng.2007.64
- Munoz P, Blanco R, de Carcer G, Schoeftner S, Benetti R, Flores JM, et al. TRF1 controls telomere length and mitotic fidelity in epithelial homeostasis. Mol Cell Biol 2009; 29:1608 - 1625; PMID: 19124610; http://dx.doi.org/10.1128/MCB.01339-08
- Li W, Lan Z, Wu H, Wu S, Meadows J, Chen J, et al. BUBR1 phosphorylation is regulated during mitotic checkpoint activation. Cell Growth Differ 1999; 10:769 - 775; PMID: 10593653
- Kim M, Murphy K, Liu F, Parker SE, Dowling ML, Baff W, et al. Caspase-mediated specific cleavage of BubR1 is a determinant of mitotic progression. Mol Cell Biol 2005; 25:9232 - 9248; PMID: 16227576; http://dx.doi.org/10.1128/MCB.25.21.9232-48.2005
- Cheng J, Kang X, Zhang S, Yeh ET. SUMO-specific protease 1 is essential for stabilization of HIF1alpha during hypoxia. Cell 2007; 131:584 - 595; PMID: 17981124; http://dx.doi.org/10.1016/j.cell.2007.08.045
- Wang X, Yang Y, Duan Q, Jiang N, Huang Y, Darzynkiewicz Z, et al. sSgo1, a major splice variant of Sgo1, functions in centriole cohesion where it is regulated by Plk1. Dev Cell 2008; 14:331 - 341; PMID: 18331714; http://dx.doi.org/10.1016/j.devcel.2007.12.007