Abstract
Although adult mammals are unable to significantly regenerate their heart, this is not the case for a number of other vertebrate species. In particular, zebrafish are able to fully regenerate their heart following amputation of up to 20% of the ventricle. Soon after amputation, cardiomyocytes dedifferentiate and proliferate to regenerate the missing tissue. More recently, identical results have also been obtained in neonatal mice. Ventricular amputation of neonates leads to a robust regenerative response driven by the proliferation of existing cardiomyocytes in a similar manner to zebrafish. However, this ability is progressively lost during the first week of birth. The fact that adult zebrafish retain the capacity to regenerate their heart suggests that they either possess a unique regenerative mechanism, or that adult mammals lose/ inhibit this process. p38α ΜAPK has previously been shown to negatively regulate the proliferation of adult mammalian cardiomyocytes. We sought to determine whether a similar mechanism exists in adult zebrafish, and whether this needs to be overcome to allow regeneration to proceed. To determine whether p38α ΜAPK also regulates zebrafish cardiomyocytes in a similar manner, we generated conditional transgenic zebrafish in which either dominant-negative or active p38α ΜAPK are specifically expressed in cardiomyocytes. We found that active p38α ΜAPK but not dominantnegative p38α ΜAPK blocks proliferation of adult zebrafish cardiomyocytes and, consequently, heart regeneration as well. It appears that adult zebrafish cardiomyocytes share many characteristics with adult mammalian cardiomyocytes, including p38α MAPK-mediated cell cycle inhibition. These findings raise the possibility that zebrafish-like heart regeneration could be achieved in adult mammals.
Introduction
Adult zebrafish cardiomyocytes possess the ability to proliferate in vivo which permits them to regenerate the heart following substantial amounts of damage.Citation1-Citation3 However, the same is not true for adult mammalian cardiomyocytes and hence the inability of adult mammals to mount any significant regenerative response following cardiac injury. Nevertheless adult mammalian cardiomyocytes can be induced to proliferate in vitro which indicates that the necessary cellular machinery is present.Citation4 This could mean that adult mammalian cardiomyocytes are not receiving the necessary proliferative signals or, that these are present but proliferation is being inhibited. Indeed it is possible to induce adult mammalian cardiomyocytes to proliferate by either stimulating the cell cycleCitation5,Citation6 or by overriding inhibitory signals.Citation7-Citation9 Because adult zebrafish obviously possess the necessary proliferative signals we sought to determine whether they are also regulated by known inhibitory mechanisms identified in mammals and hence begin to elucidate the difference in proliferative potential between adult mammalian and adult zebrafish cardiomyocytes. In mammals, p38α ΜΑPK activity is inversely correlated with heart growth. During fetal development, when cardiomyocytes proliferate readily, p38α ΜΑPK activity is low, however shortly after birth p38α ΜΑPK activity increases and is maintained throughout adult life.Citation7 Inducing p38α ΜΑPK activity in fetal cardiomyocytes either in vitro or in vivo results in a substantial reduction of proliferation and consequently cardiogenesis is perturbed.Citation7,Citation10 To determine whether p38α ΜΑPK also regulates zebrafish cardiomyocytes in a similar manner, we generated conditional transgenic zebrafish in which either dominant negative(dn)p38α ΜΑPK (tg:cmlc2:LrL:dn p38α ΜΑPK) or a constitutively active (ca)MKK6 (the upstream activator of p38α ΜΑPK and a well-established technique for inducing and maintaining high levels of active p38α ΜΑPKCitation11-Citation13) (tg:cmlc2:LrL:caMKK6) are specifically expressed in cardiomyocytes following tamoxifen (4-HT)/Cre mediated recombination, both lines were created on our previously published tg-cmlc2a-Ert2-Cre-Ert2/tg-cmlc2a-LnL-GFP background,Citation14 allowing us to monitor Cre activity, via GFP expression, and to concomitantly lineage trace recombined cardiomyocytes.
Results
Cardiomyocyte specific expression of caMKK6 disrupts zebrafish cardiogenesis
Previous results in mammals indicate that active p38α ΜΑPK inhibits the proliferation of fetal cardiomyocytes.Citation7 To determine if this is also the case in zebrafish we induced expression of either dnp38α ΜΑPK or caMKK6 specifically in cardiomyocytes during embryogenesis. Transgenic (tg:cmlc2:LrL:dn p38α ΜΑPK) or (tg:cmlc2:LrL:caMKK6) zebrafish embryos were treated at 24hpf with 4-HT then allowed to develop for a further 48hrs. In situ hybridization using anti-sense dnp38α ΜΑPK or caMKK6 RNA probes revealed robust expression of both transgenes specifically in the heart of developing zebrafish embryos obtained from their respective transgenic lines, no expression was observed in wild-type embryos (Fig. One A,D and G). During zebrafish embryogenesis the heart undergoes a series of distinct morphological changes. By 24hpf the heart tube has been established, then during the next 24hrs it performs an S-shaped loop bringing the prospective atria and ventricle adjacent to one another.Citation15 We could not detect any noticeable morphological defects in embryos expressing dnp38α ΜΑPK compared with wild-type embryos (), similar to results obtained from conditional, cardiomyocyte specific, p38α ΜΑPK knockout mice.Citation16 However, embryos expressing caMKK6 exhibited severely disrupted cardiogenesis characterized by a failure of the heart tube to loop by 3dpf (). Despite the severity of this phenotype we could not detect any obvious cytotoxic effects, such as heart edema, produced by expressing caMKK6. To determine whether cardiomyocyte proliferation had been affected by expression of caMKK6, we BrdU labeled developing embryos between 24hpf and 48hpf. Previous research has established that cardiomyocyte proliferation does occur during this developmental time window, albeit at a relatively low rate.Citation17 We were able to detect BrdU labeling co-localized with RFP-positive cardiomyocytes in both wild-type and dnp38α ΜΑPK embryos, however this was not the case in caMKK6 embryos which show virtually no co-localization of BrdU with cardiomyocytes (, insets). These results indicate that caMKK6 inhibits cardiomyocyte proliferation during zebrafish cardiogenesis and also that the small amount of proliferation that normally occurs in wild-type embryos is required for cardiac looping.
Figure 1. Cardiomyocyte specific expression of caMKK6 disrupts zebrafish cardiogenesis. In situ hybridization for either caMKK6 or dnp38α MAPK in, wild-type 3dpf embryos (A), dnp38α MAPK expressing embryos (D) or caMKK6 expressing embryos (G), the black circle indicates approximately where the heart is. Morphological analysis of cardiogenesis, (cardiomyocytes are expressing GFP under the control of the cmlc2a promoter) in wild-type 3dpf embryos (B), dnp38α MAPK expressing embryos (E) or caMKK6 expressing embryos (H). BrdU colocalization with cardiomyocytes (BrdU-green, cardiomyocytes-red) in wild-type 3dpf embryos (C), dnp38α MAPK expressing embryos (F) or caMKK6 expressing embryos (I), insets show a graphical representation of BrdU/cardiomyocyte colocalization.
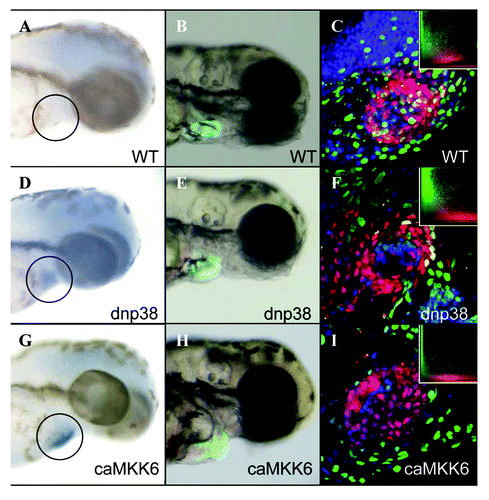
Mosaic caMMK6 expressing cardiomyocytes do not contribute to the regenerative process
Although adult mammalian cardiomyocytes are unable to proliferate, a concomitant inhibition of p38α ΜΑPK in conjunction with FGF stimulation is sufficient to induce cardiomyocyte proliferation both in vitro and in vivo.Citation7 We next sought to determine whether expression of caMKK6 would inhibit the extensive proliferation of cardiomyocytes which occurs during zebrafish heart regeneration.Citation1,Citation14 To achieve this we utilized a transgenic line in which the Cre transgene has been partially silenced, resulting in random mosaic expression of Cre throughout the heart. Consequently, treatment with 4-HT induces Cre mediated recombination only in a subset of cardiomyocytes which begin to express GFP (−D). This allowed us to express caMKK6 in a random subpopulation of cardiomyocytes and then determine whether these cells proliferate and contribute to the regenerative process (). At 14 d post amputation (dpa) we found substantial regeneration had occurred with new myocardial tissue clearly labeled for α-sarcomeric actin (). Within the same heart, only a random subpopulation of cardiomyocytes express caMKK6 (), however, no caMKK6 expressing cardiomyocytes are present in the newly regenerated tissue (−H). BrdU labeling also clearly delineates the regenerated tissue with the most abundant BrdU labeling clearly overlapping the newly formed myocardium (). At 30dpa, heart regeneration is almost complete and again we found that the newly regenerated tissue was completely lacking any cardiomyocytes expressing caMKK6 (−L). To determine whether any of the caMKK6 cardiomyocytes had proliferated, we first counted the number of BrdU-positive nuclei per section then subsequently determined what proportion of these were cardiomyocytes. In control 14dpa hearts we found that 52.1% of the total amount of counted cells labeled with BrdU (6887) were cardiomyocytes (3591). However, although considerable proliferation occurs in regenerating hearts mosaically expressing caMKK6, we could not detect any BrdU labeling in cardiomyocytes which expressed caMKK6 (BrdU-positive nuclei counted = 4657, BrdU-positive/ caMKK6-positive nuclei = 0). These results indicate that cardiomyocytes expressing caMKK6 are unable to proliferate and do not contribute to the regenerative process.
Figure 2. Mosaic caMMK6 expressing cardiomyocytes do not contribute to the regenerative process. An adult zebrafish heart immunolabelled for α sarcomeric actin (yellow) (A), GFP(caMKK6)(green) (B), merged image showing α sarcomeric actin (yellow), GFP(caMKK6)(green) and BrdU (red) (C). In situ hybridization for caMKK6 (blue) in a similar mosaic adult zebrafish heart (D). A 14dpa regenerating heart (white dashed line indicates the plane of amputation) immunolabelled for α sarcomeric actin (yellow) (E), GFP(caMKK6)(green) (F), GFP(caMKK6)(green) and BrdU (red) (G) and a merged image showing α sarcomeric actin (yellow), GFP(caMKK6)(green), BrdU (red) and DAPI (blue) (H). A 30dpa regenerating heart (white dashed line indicates the plane of amputation) immunolabelled for α sarcomeric actin (yellow) (I), GFP(caMKK6)(green) (J), GFP(caMKK6)(green) and BrdU (red) (K) and a merged image showing α sarcomeric actin (yellow), GFP(caMKK6)(green), BrdU (red) and DAPI (blue) (L).
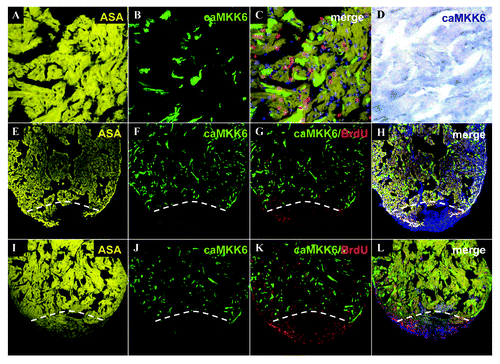
Expression of caMKK6 in all cardiomyocytes effectively blocks zebrafish heart regeneration
Although we could not observe any proliferation of caMKK6 expressing cardiomyocytes, this does not necessarily mean that caMKK6 is completely blocking this process. Another possible explanation is that cardiomyocytes not expressing caMKK6 preferentially proliferate rather than the caMKK6 expressing cells. To determine to what extent expression of caMKK6 blocks cardiomyocyte proliferation we performed identical amputation experiments on transgenic zebrafish which express caMKK6 in all cardiomyocytes using a Cre transgenic line which is not silenced. As we have previously shown, at 14dpa a substantial amount of myocardial regeneration normally occurs (). We could not detect any observable differences in regenerative capacity between controls hearts and those expressing dnp38α ΜΑPK (−H). However, in hearts expressing caMKK6 in all cardiomyocytes, regeneration, if not completely blocked, was severely inhibited (n = 8). Few if any cardiomyocytes had been produced below the plane of amputation and in a significant number of hearts (n = 3/8) a large clot remained next to the plane of amputation, something we have never observed in wild-type regenerating hearts at this stage (). Similarly, at 30dpa when regeneration is virtually complete in wild-type hearts () this process has been severely inhibited in zebrafish expressing caMKK6 (n = 10) (). These results indicate that the expression of caMKK6 effectively blocks myocardial regeneration in zebrafish. Furthermore, we could not detect any obvious GFP-negative myocardium (−L) indicating that if stem/progenitor cells reside in the zebrafish heart then they are unable to significantly regenerate the myocardium when cardiomyocyte proliferation is blocked.
Figure 3. Expression of caMKK6 in all cardiomyocytes effectively blocks zebrafish heart regeneration. A 14dpa wild-type regenerating heart immunolabeled for GFP (green), BrdU (red) and DAPI (blue), the white dashed line indicates the plane of amputation (A). The same heart at higher magnification (B). A 30dpa wild-type regenerating heart immunolabeled for GFP (green), BrdU (red) and DAPI (blue), the white dashed line indicates the plane of amputation (C). The same heart at higher magnification (D). A 14dpa dnp38α MAPK regenerating heart immunolabeled for GFP (green), BrdU (red) and DAPI (blue), the white dashed line indicates the plane of amputation (E). The same heart at higher magnification (F). A 30dpa dnp38α MAPK regenerating heart immunolabeled for GFP (green), BrdU (red) and DAPI (blue), the white dashed line indicates the plane of amputation (G). The same heart at higher magnification (H). A 14dpa caMKK6 regenerating heart immunolabeled for GFP (green), BrdU (red) and DAPI (blue), the white dashed line indicates the plane of amputation (I). The same heart at higher magnification (J). A 30dpa caMKK6 regenerating heart immunolabeled for GFP (green), BrdU (red) and DAPI (blue), the white dashed line indicates the plane of amputation (K). The same heart at higher magnification (L).
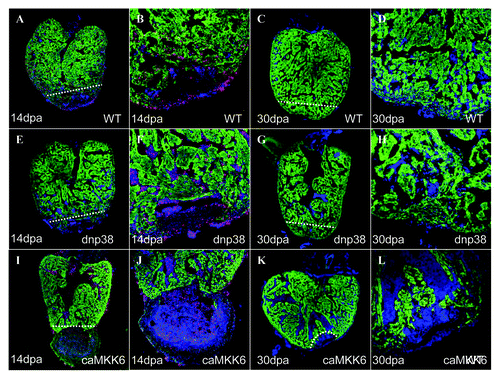
Adult zebrafish cardiomyocytes express active p38α MAPK which is switched off upon entry into mitosis
Although active p38α ΜΑPK naturally inhibits the proliferation of adult mammalian cardiomyocytes, one possible explanation for our observed results is that this mechanism does not exist in wild-type zebrafish, which would go some way to explaining why zebrafish are capable of extensive heart regeneration and mammals are not. To determine whether or not this is the case, we first examined if active p38α ΜΑPK is present in cultured adult zebrafish cardiomyocytes. Using a specific active p38α ΜΑPK antibody that had been verified via western blot, we found that all adult zebrafish cardiomyocytes contain active p38α ΜΑPK in their nucleus (). To determine whether active p38α ΜΑPK needs to be switched off during cardiomyocyte proliferation we first analyzed BrdU labeled cardiomyocytes. Although the majority of BrdU labeled cardiomyocytes retain a clear active p38α ΜΑPK signal, we were able to find individuals in which the signal was absent indicating that active p38α ΜΑPK may need to be switched off at a specific point during the cell cycle (−D). p38α ΜΑPK is known to induce arrest at various points in the cell cycle including the G2/M phase.Citation18 To establish if p38α ΜΑPK activity must be switched off in order for cardiomyocytes to enter mitosis, we immunolabelled them with a phospho histone H3 (PHH3) antibody that is only present during the M phase of the cell cycle.Citation19 We found numerous cardiomyocytes labeled with PHH3, however, the active p38α ΜΑPK signal was completely absent from these cells (51 cells PHH3-positive/ active p38α ΜΑPK-negative) (−H). We next sought to determine if this was also the case in vivo during heart regeneration. Adult zebrafish hearts were amputated and allowed to regenerate for 10 d, these were subsequently analyzed via immunohistochemistry using PHH3 and active p38α ΜΑPK antibodies. We were unable to detect any PHH3 signal co-localized with active p38α ΜΑPK(n = 10 PHH3-positive cardiomyocytes analyzed from 2 different hearts) (). This shows that in order for adult zebrafish cardiomyocytes to enter into mitosis they must first switch off p38α ΜΑPK activity.
Figure 4. Adult zebrafish cardiomyocytes express active p38α MAPK which is switched off upon entry into mitosis. Cultured adult zebrafish cardiomyocytes were immunolabeled for α sarcomeric actin (yellow) (A), phospho- p38α MAPK (green) (B), BrdU (red) (C) and a merged image with α sarcomeric actin (yellow), phospho- p38α MAPK (green), BrdU (red) and DAPI (blue) (D), the white arrow indicates the BrdU-positive cardiomyocyte in (A-D). Cultured adult zebrafish cardiomyocytes were immunolabeled for α sarcomeric actin (yellow) (E), phospho- p38α MAPK (green) (F), phospho histone H3 (PHH3) (red) (G) and a merged image with α sarcomeric actin (yellow), phospho- p38α MAPK (green), BrdU (red) and DAPI (blue) (H), the white arrow indicates the PHH3-positive cardiomyocytes in (E-H). A 10dpa cmlc2a-GFP regenerating heart immunolabeled for GFP (green) (I), phospho- p38α MAPK (magenta)(J), and phospho histone H3 (PHH3) (red) (K) and a merged image with GFP (green), phospho- p38α MAPK (magenta), phospho histone H3 (PHH3) (red) and DAPI (blue) (L), the white arrow indicates the PHH3-positive cardiomyocytes in (I-L).
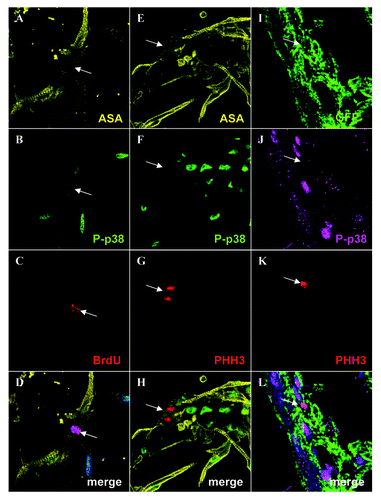
Discussion
Here we have shown that adult zebrafish and mammalian cardiomyocytes share a common molecular mechanism which regulates their proliferation. Our findings indicate that active p38α ΜΑPK negatively regulates the proliferation of adult zebrafish cardiomycoytes in a similar manner to previous reports in mammals.Citation7 p38α ΜΑPK activity is inversely correlated with heart growth in mammals, low during hyperplastic fetal stages and high in adults.Citation7 Consequently, inducing p38α ΜΑPK activity in mammalian fetal cardiomyocytes blocks proliferation.Citation7 Similarly in embryonic zebrafish, induction of p38α ΜΑPK activity blocks cardiomyocyte proliferation resulting in severely disrupted cardiogenesis. Conversely, expression of dnp38α ΜΑPK has no observable effects during embryonic cardiogenesis. These findings mirror previous reports in mammals and indicate that during zebrafish embryonic development p38α ΜΑPK activity must be switched off (or be absent) for normal cardiomyocyte proliferation to occur. During heart regeneration in adult zebrafish, we found that induction of p38α ΜΑPK activity effectively blocks this process, however, inhibiting p38α ΜΑPK activity had no observable effects. This highlights the fact that p38α ΜΑPK activity must be switched off in order for adult zebrafish cardiomyocytes to proliferate and ultimately regenerate the heart. Conversely, expression of dnp38α ΜΑPK does not lead to unrestricted proliferation as one might have expected, and indicates that other mechanisms are also involved in regulating cardiomyocyte proliferation. Interestingly, we also found that when cardiomyocyte proliferation is blocked, this essentially also blocks myocardial regeneration. It would appear then, that there is no back up mechanism for heart regeneration in zebrafish. If stem/progenitor cells are present in the zebrafish heart then they are incapable of producing any substantial regenerative response when cardiomyocyte proliferation is blocked. We also found that active p38α ΜΑPK is present in adult zebrafish cardiomyocytes both in vitro and in vivo and only disappears when the cells enter mitosis. This suggests that p38α ΜΑPK seems to be involved in entry into the M phase of the cell cycle. In adult mammals, active p38α ΜΑPK is known to induce arrest at various points of the cell cycle including the G2/M checkpoint.Citation18 It appears then that active p38α ΜΑPK arrests both adult mammalian and zebrafish cardiomyocytes in the cell cycle and once this inhibition is released, either pharmacologically in mammals or naturally in zebrafish, then cardiomyocytes are able to enter mitosis and ultimately proliferate. Our findings indicate that adult zebrafish and mammalian cardiomyocytes share a common p38α ΜΑPK mediated molecular mechanism which regulates their proliferation. Although adult mammalian cardiomyocytes do not naturally proliferate, numerous reports have shown that they can be induced to achieve this, which indicates that the proliferative machinery is present in these cells.Citation4 While one could argue that mammals lack some extrinsic signal(s) necessary for inducing heart regeneration, results from neonatal mice indicate that these are present early in life.Citation20 If adult mammals lose/inhibit heart regeneration then understanding how adult zebrafish regenerate their heart will hopefully, one day, allow us to reactivate this latent ability in adult mammals.
Materials and Methods
Constructs and zebrafish lines
All constructs and transgenic lines were generated using the Tol2 Kit as described.Citation14,Citation21 For the tg:cmlc2:LrL:dnp38α ΜΑPK construct the 5′ entry clone cmlc2a contained a 1kb PCR fragment of the cmcl2a promoter,Citation22 the middle entry clone contained a floxed RFP stop cassette amplified from pBOB-LRL-CBReGFPpA (a kind gift from Geoff Whal) and the 3′ entry clone contained murine dominant negative p38α ΜΑPK (86.2% protein homology to zebrafish p38α ΜΑPK) (a kind gift from Roger Davis). The tg:cmlc2:LrL:caMKK6 was identical except the 3′ entry clone contained human constitutively active MKK6 (86.9% protein homology to zebrafish MKK6) (a kind gift from Roger Davis).
Cre/tamoxifen induction and heart amputation
Transgenes were expressed by inducing Cre mediated recombination with tamoxifen as described.Citation14 All amputations were performed as described.Citation14
BrdU labeling
BrdU treatment was performed essentially as described.Citation17,Citation23 Metamorph® software (Molecular Devices) was used to count BrdU labeled cardiomyocytes.
Cardiomyocyte isolation
Fish were sacrificed in tricaine. Hearts were collected and put into PBS with penicillin and streptomycin and 10U/ml heparin. The outflow tracts were then removed and ventricles and atriums were opened to get rid of the blood. They were then washed three times in perfusion buffer [PBS, 10mM HEPES, 30mM taurine, 5,5mM glucose and 10mM 2,3-butanedione monoxime (BDM)] and placed into digestion buffer [perfusion buffer plus 12,5μM calcium chloride and collagenase, II and IV, 5 mg each (Gibco)] to digest for 2 h at 32°C in a thermomixer at 800rpm. Next, an equal volume of stop buffer 1 (perfusion buffer plus 12,5μM calcium chloride and 10%FBS) was added and cells were mechanically separated. Undigested material was left to sediment and cells suspended in the supernatant were pelleted by centrifugation at 250 g for 5 min. The cells were then resuspended in stop buffer 2 (perfusion buffer plus 12,5μM and 5%FBS) and calcium reintroduction was performed by gradually raising the concentration to 62, 112, 212, 500 and 1000μM respectively. Cells were then pelleted again and resuspended in plating medium (MEM, 5%FBS, 10mM BDM, 2mM Glutamax, 100U/ml penicillin and 100μg/ml streptomycin). Cell preparations were plated onto collagen type I (BD Biosciences, ref. 354236) treated chamber slides (Nunc, ref. 177445), and allowed to attach overnight. Immunofluorescence was performed the following day.
Immunohistochemistry
Immunohistochemistry was performed on 10 μm cryo-sections as previously described.Citation1 The The antibodies used in this manuscript are anti-GFP (AVES GFP-1020), anti-BrdU (Accuratechemical OBT0030), anti-phopsho histone H3 (Upstate 06–570), anti-PCNA (Sigma P8825), anti-α sarcomeric actin (Sigma A2172), anti-RFP (Abcam ab34771), anti-Tropomyosin (Sigma T2780), anti-p-p38 (Thr180/Tyr182) (Cell Signaling #4511), anti-Mef2c (Abcam ab79436).
In situ hybridization
In situ hybridization was performed as described previously.Citation23 The dnp38α ΜΑPK probe was generated by subcloning dominant negative dnp38α ΜΑPK into the Hind111 and BamH1 sites of pBSK- . The caMKK6 probe was generated by subcloning constitutively active MKK6 into the Hind111 and Xba1 sites of pBSK- .
Confocal microscopy
Confocal microscopy was performed using a Leica SP5. For co-localization analysis the formula used to calculate the axial resolution is as follows.
Dz = √((l*n/NA2) + (AU*n*√2*1,22*l/NA2)2)
Emission (l) = 500 nm
Refractive index 1.518
NA = 1,4
Airy Units: 1
This results in a section thickness of z = 0,773μm
Acknowledgements
Work in the laboratory of J.C.I.B. was supported by grants from MICINN, Fundacion Cellex, IPSEN Foundation, The Leona M. and Harry B. Helmsley Charitable Trust, The G. Harold and Leila Y. Mathers Charitable Foundation and Sanofi-Aventis.
Disclosure of Potential Conflicts of Interest
No potential conflicts of interest were disclosed.
References
- Poss KD, Wilson LG, Keating MT. Heart regeneration in zebrafish. Science 2002; 298:2188 - 90; http://dx.doi.org/10.1126/science.1077857; PMID: 12481136
- Wang J, Panáková D, Kikuchi K, Holdway JE, Gemberling M, Burris JS, et al. The regenerative capacity of zebrafish reverses cardiac failure caused by genetic cardiomyocyte depletion. Development 2011; 138:3421 - 30; http://dx.doi.org/10.1242/dev.068601; PMID: 21752928
- González-Rosa JM, Martín V, Peralta M, Torres M, Mercader N. Extensive scar formation and regression during heart regeneration after cryoinjury in zebrafish. Development 2011; 138:1663 - 74; http://dx.doi.org/10.1242/dev.060897; PMID: 21429987
- Bicknell KA, Coxon CH, Brooks G. Can the cardiomyocyte cell cycle be reprogrammed?. J Mol Cell Cardiol 2007; 42:706 - 21; http://dx.doi.org/10.1016/j.yjmcc.2007.01.006; PMID: 17362983
- Bicknell KA, Coxon CH, Brooks G. Forced expression of the cyclin B1-CDC2 complex induces proliferation in adult rat cardiomyocytes. Biochem J 2004; 382:411 - 6; http://dx.doi.org/10.1042/BJ20031481; PMID: 15253691
- Tamamori-Adachi M, Ito H, Sumrejkanchanakij P, Adachi S, Hiroe M, Shimizu M, et al. Critical role of cyclin D1 nuclear import in cardiomyocyte proliferation. Circ Res 2003; 92:e12 - 9; http://dx.doi.org/10.1161/01.RES.0000049105.15329.1C; PMID: 12522130
- Engel FB, Schebesta M, Duong MT, Lu G, Ren S, Madwed JB, et al. p38 MAP kinase inhibition enables proliferation of adult mammalian cardiomyocytes. Genes Dev 2005; 19:1175 - 87; http://dx.doi.org/10.1101/gad.1306705; PMID: 15870258
- Di Stefano V, Giacca M, Capogrossi MC, Crescenzi M, Martelli F. Knockdown of cyclin-dependent kinase inhibitors induces cardiomyocyte re-entry in the cell cycle. J Biol Chem 2011; 286:8644 - 54; http://dx.doi.org/10.1074/jbc.M110.184549; PMID: 21209082
- Porrello ER, Johnson BA, Aurora AB, Simpson E, Nam YJ, Matkovich SJ, et al. MiR-15 family regulates postnatal mitotic arrest of cardiomyocytes. Circ Res 2011; 109:670 - 9; http://dx.doi.org/10.1161/CIRCRESAHA.111.248880; PMID: 21778430
- Liao P, Georgakopoulos D, Kovacs A, Zheng M, Lerner D, Pu H, et al. The in vivo role of p38 MAP kinases in cardiac remodeling and restrictive cardiomyopathy. Proc Natl Acad Sci USA 2001; 98:12283 - 8; http://dx.doi.org/10.1073/pnas.211086598; PMID: 11593045
- Raingeaud J, Whitmarsh AJ, Barrett T, Dérijard B, Davis RJ. MKK3- and MKK6-regulated gene expression is mediated by the p38 mitogen-activated protein kinase signal transduction pathway. Mol Cell Biol 1996; 16:1247 - 55; PMID: 8622669
- Thornton TM, Pedraza-Alva G, Deng B, Wood CD, Aronshtam A, Clements JL, et al. Phosphorylation by p38 MAPK as an alternative pathway for GSK3beta inactivation. Science 2008; 320:667 - 70; http://dx.doi.org/10.1126/science.1156037; PMID: 18451303
- Zhang R, Murakami S, Coustry F, Wang Y, de Crombrugghe B. Constitutive activation of MKK6 in chondrocytes of transgenic mice inhibits proliferation and delays endochondral bone formation. Proc Natl Acad Sci USA 2006; 103:365 - 70; http://dx.doi.org/10.1073/pnas.0507979103; PMID: 16387856
- Jopling C, Sleep E, Raya M, Martí M, Raya A, Izpisúa Belmonte JC. Zebrafish heart regeneration occurs by cardiomyocyte dedifferentiation and proliferation. Nature 2010; 464:606 - 9; http://dx.doi.org/10.1038/nature08899; PMID: 20336145
- Glickman NS, Yelon D. Cardiac development in zebrafish: coordination of form and function. Semin Cell Dev Biol 2002; 13:507 - 13; http://dx.doi.org/10.1016/S1084952102001040; PMID: 12468254
- Nishida K, Yamaguchi O, Hirotani S, Hikoso S, Higuchi Y, Watanabe T, et al. p38alpha mitogen-activated protein kinase plays a critical role in cardiomyocyte survival but not in cardiac hypertrophic growth in response to pressure overload. Mol Cell Biol 2004; 24:10611 - 20; http://dx.doi.org/10.1128/MCB.24.24.10611-10620.2004; PMID: 15572667
- de Pater E, Clijsters L, Marques SR, Lin YF, Garavito-Aguilar ZV, Yelon D, et al. Distinct phases of cardiomyocyte differentiation regulate growth of the zebrafish heart. Development 2009; 136:1633 - 41; http://dx.doi.org/10.1242/dev.030924; PMID: 19395641
- Thornton TM, Rincon M. Non-classical p38 map kinase functions: cell cycle checkpoints and survival. Int J Biol Sci 2009; 5:44 - 51; http://dx.doi.org/10.7150/ijbs.5.44; PMID: 19159010
- Gurley LR, D’Anna JA, Barham SS, Deaven LL, Tobey RA. Histone phosphorylation and chromatin structure during mitosis in Chinese hamster cells. Eur J Biochem 1978; 84:1 - 15; http://dx.doi.org/10.1111/j.1432-1033.1978.tb12135.x; PMID: 206429
- Porrello ER, Mahmoud AI, Simpson E, Hill JA, Richardson JA, Olson EN, et al. Transient regenerative potential of the neonatal mouse heart. Science 2011; 331:1078 - 80; http://dx.doi.org/10.1126/science.1200708; PMID: 21350179
- Kwan KM, Fujimoto E, Grabher C, Mangum BD, Hardy ME, Campbell DS, et al. The Tol2kit: a multisite gateway-based construction kit for Tol2 transposon transgenesis constructs. Dev Dyn 2007; 236:3088 - 99; http://dx.doi.org/10.1002/dvdy.21343; PMID: 17937395
- Huang CJ, Tu CT, Hsiao CD, Hsieh FJ, Tsai HJ. Germ-line transmission of a myocardium-specific GFP transgene reveals critical regulatory elements in the cardiac myosin light chain 2 promoter of zebrafish. Dev Dyn 2003; 228:30 - 40; http://dx.doi.org/10.1002/dvdy.10356; PMID: 12950077
- Raya A, Koth CM, Büscher D, Kawakami Y, Itoh T, Raya RM, et al. Activation of Notch signaling pathway precedes heart regeneration in zebrafish. Proc Natl Acad Sci USA 2003; 100:Suppl 1 11889 - 95; http://dx.doi.org/10.1073/pnas.1834204100; PMID: 12909711