Abstract
We have recently proposed a new two-compartment model for understanding the Warburg effect in tumor metabolism. In this model, glycolytic stromal cells produce mitochondrial fuels (L-lactate and ketone bodies) that are then transferred to oxidative epithelial cancer cells, driving OXPHOS and mitochondrial metabolism. Thus, stromal catabolism fuels anabolic tumor growth via energy transfer. We have termed this new cancer paradigm the “reverse Warburg effect,” because stromal cells undergo aerobic glycolysis, rather than tumor cells. To assess whether this mechanism also applies during cancer cell metastasis, we analyzed the bioenergetic status of breast cancer lymph node metastases, by employing a series of metabolic protein markers. For this purpose, we used MCT4 to identify glycolytic cells. Similarly, we used TO MM20 and COX staining as markers of mitochondrial mass and OXPHOS activity, respectively. Consistent with the “reverse Warburg effect,” our results indicate that metastatic breast cancer cells amplify oxidative mitochondrial metabolism (OXPHOS) and that adjacent stromal cells are glycolytic and lack detectable mitochondria. Glycolytic stromal cells included cancer-associated fibroblasts, adipocytes and inflammatory cells. Double labeling experiments with glycolytic (MCT4) and oxidative (TO MM20 or COX) markers directly shows that at least two different metabolic compartments co-exist, side-by-side, within primary tumors and their metastases. Since cancer-associated immune cells appeared glycolytic, this observation may also explain how inflammation literally “fuels” tumor progression and metastatic dissemination, by “feeding” mitochondrial metabolism in cancer cells. Finally, MCT4(+) and TO MM20(-) “glycolytic” cancer cells were rarely observed, indicating that the conventional “Warburg effect” does not frequently occur in cancer-positive lymph node metastases.
Introduction
Loss of stromal caveolin-1 (Cav-1) in human primary breast tumors is a powerful predictive marker of recurrence, lymph-node metastasis, tamoxifen-resistance and poor overall patient survival.Citation1,Citation2 These findings have now been independently validated in seven different countries (USA, Argentina, Australia, Korea, Japan, Egypt and the UK).Citation3–Citation7 In DCIS patients, a loss stromal Cav-1 predicts progression to invasive breast cancer.Citation8 In patients with prostate cancer and melanoma, a loss of stromal Cav-1 is specifically associated with advanced disease, progression and metastasis.Citation9,Citation10 In fact, metastatic prostate cancer cells, localized in lymph nodes and bone, are surrounded by stromal cells that lack Cav-1 protein expression.Citation9
To better understand the tight association between a loss of stromal Cav-1 expression and tumor progression and metastasis, we performed a series of OMICS analyses on human and mouse animal model systems, which were genetically engineered to lack Cav-1 expression.Citation11–Citation15 As a result of this comprehensive analysis, we discovered that a loss of stromal Cav-1 leads to a new form of two-compartment tumor metabolism, which we have termed the “reverse Warburg effect” or the “autophagic tumor stroma model of cancer.”Citation11,Citation16–Citation23
In this new model of cancer metabolism, tumor cells secrete hydrogen peroxide (H2O2) which induces oxidative stress in neighboring stromal cells.Citation17 Oxidative stress in the tumor stroma then drives the onset of autophagy and mitophagy via the activation of two key transcription factors, namely, NFκB and HIF-1α. As a consequence, catabolic stromal cells produce energy-rich mitochondrial fuels (such as L-lactate, ketone bodies, glutamine and fatty acids), which, in turn, “feed” oxidative mitochondrial metabolism (OXPHOS) in epithelial cancer cells.Citation17 In this scheme, the loss of stromal Cav-1 reflects its lysosomal degradation by autophagy. However, a loss of stromal Cav-1 also serves as a feedforward mechanism for the production of ROS and hydrogen peroxide, facilitating tumor-stroma co-evolution and genomic instability in epithelial cancer cells.Citation24–Citation27
This model is also particularly relevant to understanding the molecular basis of cancer cell metastasis.Citation18 For example, if a cancer cell can secrete hydrogen peroxide, then it can travel to any site within the body, and wherever it disseminates, it can induce oxidative stress and autophagy in normal neighboring cells, which then provides cancer cells with high-energy nutrients (such as L-lactate), without the need for the vasculature (tumor angiogenesis). In direct support of this hypothesis, L-lactate treatment fuels mitochondrial biogenesis in human breast cancer cellsCitation25,Citation26,Citation28 and increases their metastatic capacity in animal models, allowing them to colonize the lung, with a > 10-fold increase in the number of metastatic lesions.Citation29 Such a mechanism would also be critical early during tumor initiation, which is another phase of tumor development that is initially independent of a vascular blood supply, consistent with our biomarker studies in DCIS patients.Citation8
In accordance with this new “metastasis” hypothesis, several groups have shown an association between hydrogen peroxide production and metastasis.Citation30–Citation36 Similarly, we have demonstrated that when epithelial cancer cells are co-cultured with fibroblasts under low-serum conditions, the cancer cells become metabolically dependent on fibroblasts for survival.Citation27,Citation37 In fact, if hydrogen peroxide is depleted in these co-cultures by addition of catalase (a powerful antioxidant), then the cancer cells undergo apoptosis, likely due to the absence of fibroblast-derived mitochondrial fuels.Citation27,Citation37
A clear prediction of this new model for metastasis is that glycolytic fibroblasts would fuel mitochondrial metabolism in metastatic cancer cells. To test the in vivo relevance of this hypothesis in breast cancer patients, here we subjected breast cancer-positive lymph node tissue to staining with a series of markers to assess the bioenergetic state of metastatic cancer cells and their surrounding microenvironment. Our results directly show that mitochondrial mass and activity are increased in metastatic breast cancer cells. In contrast, the lymph node-associated stroma shows an absence of mitochondrial mass and activity, with the overexpression of MCT4, a known marker of oxidative stress and glycolysis. Glycolytic stromal cells included cancer-associated fibroblasts, adipocytes and inflammatory cells. In fact, MCT4 mediates the secretion of L-lactate and ketone bodies into the extracellular environment.Citation38–Citation41
Our current results provide a simple strategy for directly visualizing “epithelial-stromal metabolic coupling” or “two-compartment tumor metabolism” in a variety of human cancers and the resulting metastatic lesions or invasive tumor foci.Citation16,Citation23
Since both glycolytic and oxidative compartments co-exist side-by-side, we propose that the glycolytic compartment likely reflects the local secretion of hydrogen peroxide and stromal oxidative stress rather than tissue hypoxia. Instead, tissue hypoxia should result in glycolysis in both epithelial cancer cells and adjacent stromal cells without “organized” metabolic compartmentalization.
Consistent with our current findings, a gene signature for mitochondrial OXPHOS is transcriptionally upregulated in primary human breast cancer cells isolated by laser-capture micro-dissection, relative to adjacent tumor stroma.Citation39 Importantly, this MITO/OXPHOS gene signature is transcriptionally upregulated in human breast cancers (> 2,000 patients; with a p-value < 10-20) and is specifically associated with metastasis, especially in ER(-) breast cancer patients.Citation39
Results
Metastatic breast cancer cells show increased mitochondrial mass and activity.
To assess the bioenergetic state of metastatic human breast cancer cells, we analyzed a panel of positive lymph nodes that were surgically excised from human breast cancer patients. To determine the distribution of mitochondrial mass, we employed an antibody directed against TOMM20 (translocase of outer mitochondrial membrane 20), which serves as an established marker of mitochondrial mass and biogenesis.Citation39
directly shows that TOMM20 selectively stains metastatic breast cancer cells and is largely absent from the adjacent metastatic lymph node stroma. To ensure that the observed increase in mitochondrial mass actually reflects functional mitochondrial activity, we next performed COX (cytochrome C oxidase) activity staining, which detects the activity of complex IV, reflecting the capacity of cells to undergo mitochondrial electron transport and oxidative phosphorylation (OXPHOS).Citation42–Citation45
highlights that COX activity is largely compartmentalized within metastatic breast cancer cells and nearly absent from the stromal tissue within the lymph node. Importantly, COX activity staining was abolished by pre-treatment with a mitochondrial poison (sodium azide; a known complex IV inhibitor) (). Thus, metastatic breast cancer cells appear to have increased or amplified mitochondrial metabolism, as we have observed previously with primary human breast cancers.Citation39
Lymph node-associated stromal cells are glycolytic.
To monitor the presence of glycolytic cells within breast cancer-positive lymph nodes, we next employed MCT4. MCT4 (monocarboxylic acid transpoter 4; SLC16A3) functions to extrude L-lactate and ketone bodies from glycolytic cells, especially under conditions of oxidative stress (pseudo-hypoxia) and/or bonafide hypoxia. Thus, MCT4 is a sensitive marker of aerobic glycolysis (a.k.a., the Warburg effect).Citation38,Citation40,Citation46,Citation47
shows that the lymph node-associated stromal cells are MCT4(+), while the adjacent metastatic breast cancer cells are MCT4(-), indicating that oxidative stress (pseudo-hypoxia) is largely confined to stromal cells.
We have recently shown that upregulation of stromal MCT4 is specifically associated with a loss of stromal Cav-1 (p < 10–14) in primary human breast tumors.Citation48,Citation49 Thus, we also examined the status of stromal Cav-1 within breast cancer cell-positive lymph nodes.
Our results indicate that there is a loss of stromal Cav-1 expression, as predicted (). Importantly, a loss of stromal Cav-1 is also indicative of oxidative stress and the onset of autophagy in the stromal microenvironment.Citation11–Citation13,Citation22,Citation25,Citation26 However, the vasculature remained Cav-1-positive, as endothelial cells are resistant to oxidative stress.
Double labeling with glycolytic and mitochondrial markers allows the direct visualization of the “reverse Warburg effect” in metastatic breast cancers. To simultaneously visualize both glycolytic and oxidative metabolic compartments, breast cancer-positive lymph node tissues were subjected to double labeling, with MCT4 and COX activity staining.
indicates that MCT4 staining is predominantly localized to the cancer-associated lymph node stroma. In contrast, COX activity is strongly associated with the metastatic breast cancer cells. Thus, this approach allowed us to visualize both glycolytic and oxidative metabolic compartments within the same tissue sections. Virtually identical results were also obtained by double labeling with MCT4 and TOMM20 (). Stromal fibroblasts were consistently MCT4(+) and TOMM20(-), indicative of a glycolytic phenotype.
Importantly, lymph node-associated immune cells and adipocytes were also MCT4(+) and TOMM20(-), suggesting that these cell types are glycolytic and may also support the “reverse Warburg effect” (). Rarely, MCT4(+) and TOMM20(-) glycolytic cancer cells were also observed, indicating that the conventional “Warburg effect” does not frequently occur in cancer-positive lymph nodes (; see the black arrowhead).
Double labeling with glycolytic and mitochondrial markers allows the direct visualization of the “reverse Warburg effect” in primary tumors.
To simultaneously visualize both glycolytic and oxidative metabolic compartments, primary breast cancer tumor tissues were subjected to double labeling, with MCT4 and TOMM20.
shows that MCT4 selectively labels the tumor stroma, while TOMM20 stains the epithelial cancer cells, highlighting the co-existence of two distinct adjacent metabolic compartments: glycolytic and oxidative. Similarly, MCT4 also labeled inflammatory cells that were adjacent to TOMM20(+) tumor cells, indicating that tumor-associated immune cells may also significantly contribute to the “reverse Warburg effect” ().
Discussion
Here, we examined the bioenergetic state of metastatic breast cancer cells and their surrounding microenvironment using positive lymph node tissue as a source material. More specifically, we employed TOMM20 and COX activity staining as measures of mitochondrial mass and functional OXPHOS activity, respectively. Furthermore, we used immunostaining with MCT4 antibodies as a marker of oxidative stress and the glycolytic phenotype, a.k.a., the Warburg effect. MCT4 is a HIF1-target gene that is normally upregulated in response to hypoxia and/or oxidative stress. MCT4 functions in the efflux of monocarboxylic acids, such as L-lactate and/or ketone bodies, from cells that rely on glycolysis for energy production and suffer from mitochondrial dysfunction.Citation38,Citation40,Citation46,Citation47
In breast cancer-positive lymph node tissue, we observed that glycolytic and oxidative mitochondrial metabolism was spatially segregated and highly compartmentalized. Metastatic tumor cells were selectively labeled with both markers of mitochondrial mass (TOMM20) and OXPHOS activity (COX staining). Conversely, lymph node-associated stromal cells appeared glycolytic [MCT4(+)] and showed a near complete absence of mitochondrial markers (TOMM20 and COX activity). Double labeling experiments directly showed that these glycolytic and oxidative compartments co-exist side-by-side, ruling out the idea that the glycolytic compartment was generated via hypoxia. More likely, we propose that the glycolytic stromal compartment is generated via localized oxidative stress induced by tumor cells. These results are summarized schematically in .
Using MCF7 fibroblast co-cultures, we have previously demonstrated that MCF7 breast cancer cells secrete hydrogen peroxide,Citation25,Citation27,Citation37,Citation50 which selectively induces that upregulation of MCT4 in adjacent stromal fibroblasts due to localized oxidative stress.Citation38 In direct support of these observations, treatment with NAC (N-acetyl-cysteine), which functions as a powerful antioxidant, prevented the induction of MCT4 expression in stromal fibroblasts that were co-cultured with MCF7 cells.Citation38
MCT4 expression in cancer-associated fibroblasts has important functional consequences, allowing these stromal cells to efflux high-energy mitochondrial fuels into the extracellular microenvironment. Cancer cells can then import these extracellular mitochondrial fuels (L-lactate and ketone bodies), which are then converted to Acetyl-CoA and “burned” via the TCA cycle and OXPHOS to efficiently generate high levels of ATP. Consistent with this hypothesis, recombinant overexpression of MCT4 in stromal fibroblasts significantly protects co-cultured MCF7 cells against apoptosis.Citation38
We have recently evaluated the prognostic value of MCT4 levels in primary breast tumors using TMAs derived from a cohort of triple-negative breast cancer patients (n > 180).Citation48 Our results indicated that high stromal MCT4 levels directly correlated with a loss of stromal Cav-1, a specific marker of tumor recurrence and metastasis.Citation48 In addition, Kaplan-Meier analysis showed that high stromal MCT4 levels strictly correlated with poor overall survival (< 20% survival at 10 years post-diagnosis), while patients with an absence of stromal MCT4 had survival rates of ∼97% at up to > 20 years post-diagnosis.Citation48 Importantly, the prognostic effects of MCT4 were compartment-specific; epithelial MCT4 levels had no prognostic value.Citation48 Thus, only MCT4-mediated efflux of L-lactate and ketone bodies from stromal cells determines clinical outcome.Citation48
In support of this hypothesis, we previously treated MCF7 cells with high-energy mitochondrial fuels (L-lactate or ketones) to generate lactate- and ketone-induced gene signatures, using transcriptional gene profiling with exon arrays.Citation28 Remarkably, both the lactate- and ketone-induced epithelial gene signatures predicted recurrence, metastasis and poor overall survival in ER+/Luminal A breast cancer patients, likely due to the induction of oxidative mitochondrial metabolism in cancer cells.Citation28
Clinical implications of oxidative stress and the “reverse Warburg effect:” Importance of combining conventional chemotherapy with antioxidants.
Most cancer patients die from tumor recurrence and metastasis, not from primary tumors. Thus, great reductions in cancer-associated mortality could be achieved by the prevention of tumor recurrence and metastasis. Several groups have directly implicated the production of hydrogen peroxide in tumor recurrence and metastasis, using mouse animal models. In this context, treatment with catalase (a powerful catalytic antioxidant that detoxifies hydrogen peroxide) is sufficient to almost completely block tumor recurrence and metastasis.Citation27,Citation31–Citation37,Citation51–Citation53
So, what is the role of hydrogen peroxide in cancer cell metastasis? We have previously shown that cancer cells secrete hydrogen peroxide to induce oxidative stress in neighboring normal cells, such as stromal fibroblasts.Citation27,Citation37 Oxidative stress in cancer-associated fibroblasts, in turn, provides high-energy fuels for cancer cells to burn via oxidative mitochondrial metabolism.Citation16,Citation17 Thus, cancer cells that produce hydrogen peroxide can leave the primary tumor site, as they will extract nutrients from any adjacent cell, wherever they go, via oxidative stress and stromal autophagy. Here, we have directly visualized this metabolic coupling in both primary and metastastic breast cancers using markers of mitochondrial function, oxidative stress and glycolysis.
These findings have important clinical implications for anticancer therapy, especially using antioxidants. Consistent with this notion, breast cancer patients treated with simple antioxidants (Vitamin C and E) after conventional therapy showed dramatic reductions in both recurrence and mortality (>20%).Citation54 If breast cancer patients that had undergone radio-therapy (a treatment that generates and enhances oxidative stress) were excluded, the antioxidant-induced reductions in both recurrence and mortality approached nearly 40–50%.Citation54 Thus, we should reconsider the use of antioxidants as a critical component of anticancer therapy.Citation54
Conversely, most conventional therapies (chemo ± radiation) kill cancer cells via increased oxidative stress.Citation20 However, sub-lethal doses of conventional therapy, which fail to kill all the cancer cells, may be inducing systemic oxidative stress and the “reverse Warburg effect,” leading to tumor recurrence and/or metastasis, or even secondary malignancies, such as leukemias and lymphomas.Citation20,Citation55,Citation56 This may explain why combining antioxidants with conventional therapy effectively reduces mortality and recurrence by preventing the local and systemic induction of the “reverse Warburg effect.”Citation55,Citation56
Materials and Methods
Materials.
Commercial antibodies were as follows: anti-Cav-1 IgG [sc-894, Santa Cruz Biotech; rabbit polyclonal (pAb)]; and anti-TOMM20 IgG [cat# sc-17764, Santa Cruz Biotech; mouse monoclonal (mAb)]. Anti-MCT4 isoform-specific rabbit polyclonal antibodies (pAb) were previously generated and characterized by Dr. Nancy Philp.Citation46 Isoform-specific antibodies were produced against the 18-mer synthetic oligopeptide corresponding to the C-terminal amino acids of MCT4.Citation46 For COX activity staining, validation studies were performed with electron transport inhibitors, such as 1 mM sodium azide (Comple IV inhibitor; cat # BP922-500, Thermo-Fisher).
Immunohistochemistry.
Paraffin-embedded sections were immunostained as previously described. Briefly, sections were de-paraffinized, rehydrated and washed in PBS. Antigen retrieval was performed in 10 mM sodium citrate, pH 6.0 for 10 min using a pressure cooker. After blocking with 3% hydrogen peroxide for 10 min, sections were incubated with 10% goat serum for 1 h. Then, sections were incubated with primary antibodies overnight at 4°C. Antibody binding was detected using a biotinylated secondary (Vector Labs) followed by strepavidin-HRP (Dako). Immunoreactivity was revealed using 3,3′-diaminobenzidine.
Cytochrome C oxidase (COX)/complex IV activity attaining.
Cryostat sections (7 or 10 µm) were prepared and stored at -80°C until use. For the COX activity staining, frozen sections were brought to room temperature, washed for 5 min with 25 mM sodium phosphate buffer, pH 7.4, and then incubated for 0.5, 1 or 2 h at 37°C with the COX incubation mixture. The COX solution consisted of 10 mg Cytochrome C (cat# C7752, Sigma-Aldrich), 10 mg 3,3′-diaminobenzidine tetrahydrochloride hydrate (cat# D5637, Sigma-Aldrich) and 2 mg catalase (cat# C1345, Sigma-Aldrich) dissolved in 10 ml of 25 mM sodium phosphate buffer. The solution was filtered after preparation and the pH was adjusted to 7.2–7.4 with 1 N NaOH. Cryostat sections obtained from normal mouse skeletal muscle (hind-limb/gastrocnemius) were used as a positive control.
Double labeling with COX activity and MCT4 staining.
Cryostat sections (8 µm) of human lymph nodes containing breast carcinoma metastases were first analyzed using the COX mitochondrial assay. For double labeling, sections were brought to room temperature, washed for 10 min with 25 mM sodium phosphate buffer and then incubated for 1.5 h at 37°C with the COX incubation mixture. The reaction was stopped by washing in 25 mM sodium phosphate buffer for 5 min. Sections were then fixed with 10% formalin for 10 min at room temperature. Sections were then washed with PBS and blocked for endogenous biotin using a biotin blocking kit (Dako X0590), followed by blocking with 10% goat serum in PBS for 1 h. Rabbit anti-MCT4 antibodies were applied at a dilution of 1:200 overnight at 4°C. Antibody binding was detected using a biotinylated goat anti-rabbit IgG (Vector Labs) followed by streptavidin-alkaline phosphatase (Jackson ImmunoResearch). Immunoreactivity was then detected using the Vector Red Alkaline Phosphatase Substrate Kit I (Vector #SK-5100) and sections were counterstained with hematoxylin, dehydrated, cleared with xylene and coverslipped with permount.
Double labeling with TOMM20 and MCT4.
Paraffin sections (4 µm) of human lymph nodes containing breast carcinoma metastases were deparaffinized, rehydrated through graded ethanols and washed in PBS. Antigen retrieval was performed in 0.01 M citrate buffer, pH 6.0 for 10 min using a pressure cooker. The sections were cooled to room temperature, blocked with 3% H2O2 for 15 min and then for endogenous biotin (Dako, #X0590). Sections were then incubated with 10% goat serum in PBS for 1 h. Mouse anti-TOMM20 antibody at a 1:500 dilution (sc-17764, Santa Cruz Biotechnology) together with the rabbit anti-MCT-4 antibody (at a dilution of 1:100), were applied overnight at 4°C. Primary antibody binding was detected using anti-mouse HRP labeled polymer (Dako, #K4000) for TOMM20 and biotinylated goat anti-rabbit IgG (Vector) followed by streptavidin-alkaline phosphatase (Jackson) for MCT4. Immunoreactivity was revealed with the Vector Red Alkaline Phosphatase Substrate Kit I (for MCT4) and the Dako Liquid DAB + Substrate System (#K3468; for TOMM20). Sections were then counterstained with hematoxylin, dehydrated, cleared with xylene and coverslipped with permount.
Disclosure of Potential Conflicts of Interest
No potential conflicts of interest were disclosed.
Figures and Tables
Figure 1 Metastatic breast cancer cells have increased mitochondrial mass. Paraffin-embedded sections of human breast cancer-positive lymph nodes were immunostained with antibodies directed against TOMM20 (brown color). Slides were then counterstained with hematoxylin (blue color). Note that TOMM20 is highly expressed in metastatic breast cancer cells. Two representative images are shown. Original magnification, 40x.
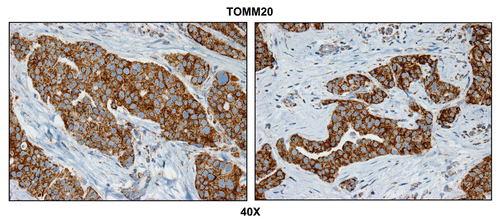
Figure 2 Metastatic breast cancer cells show increased mitochondrial activity. Frozen sections of human breast cancer-positive lymph nodes were subjected to COX activity staining (brown color). Slides were then counterstained with hematoxylin (blue color). Note that epithelial tumor cells are intensely stained, as compared with adjacent stromal cells, which show little or no COX activity. Two representative images are shown. Original magnification, 40x.
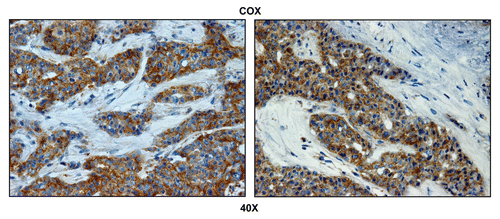
Figure 3 Mitochondrial activity staining is ablated with metabolic inhibitors. Frozen sections of human breast cancer-positive lymph nodes were subjected to COX activity staining (brown color). Slides were then counterstained with hematoxylin (blue color). Note that sodium azide (+AZ; 1 mM; a Complex IV inhibitor) effectively abolished the COX activity staining.
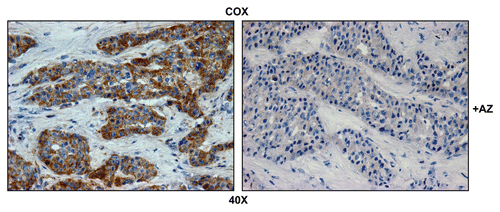
Figure 4 Lymph node associated stromal cells are glycolytic. Paraffin-embedded sections of human breast cancer-positive lymph nodes were immunostained with antibodies directed against MCT4. Slides were then counterstained with hematoxylin. Note that MCT4 is highly expressed in the stromal compartment of positive lymph nodes. Two representative images are shown. Original magnification, 40x and 60x, as indicated.
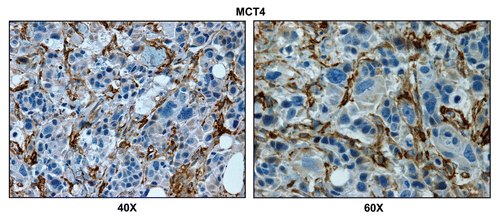
Figure 5 Cav-1 immunostaining of breast cancer-positive lymph nodes. Paraffin-embedded sections of human breast cancer-positive lymph nodes were immunostained with antibodies directed against Cav-1. Slides were then counterstained with hematoxylin. Note that Cav-1 is largely absent from the stromal compartment of positive lymph nodes, with the exception of blood vessels (indicated by red arrowheads). Two representative images are shown. Original magnification, 40x and 60x, as indicated.
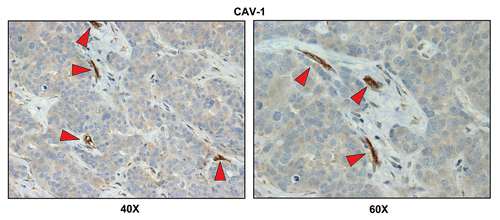
Figure 6 Visualizing the “reverse Warburg effect” by double labeling with MCT4 and COX activity staining. Frozen sections of human breast cancer-positive lymph nodes were subjected to COX activity staining (brown color) and immunostaining with MCT4 antibodies (red color). Slides were then counterstained with hematoxylin (blue color). Note that MCT4 staining is predominantly localized to the cancer-associated lymph node stroma. In contrast, COX activity is strongly associated with the metastatic breast cancer cells. Two representative images are shown. Original magnification, 40x.
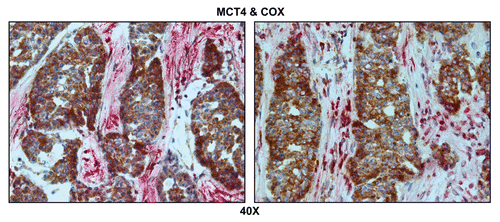
Figure 7 Visualizing the “reverse Warburg effect” by double labeling with MCT4 and TOMM20: Lymph node metastasis. Paraffin-embedded sections of human breast cancer-positive lymph nodes were immunostained with antibodies directed against MCT4 (red color) and TOMM20 (brown color). Slides were then counterstained with hematoxylin (blue color). Note that MCT4 staining is predominantly localized to the cancer-associated lymph node stroma. In contrast, TOMM20 is strongly associated with the metastatic breast cancer cells. Original magnification, 60x.
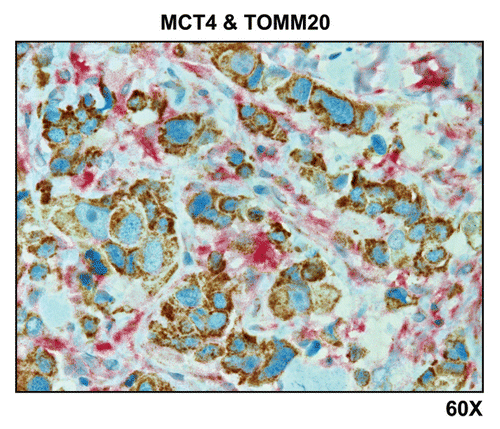
Figure 8 Lymph node-associated adipocytes and inflammatory cells are glycolytic. Paraffin-embedded sections of human breast cancer-positive lymph nodes were immunostained with antibodies directed against MCT4 (red color) and TOMM20 (brown color). Slides were then counterstained with hematoxylin (blue color). Note that lymph-node associated adipocytes (asterisks) and inflammatory cells (red arrowhead) are MCT4(+) and TOMM20(-), consistent with a glycolytic phenotype. In contrast, TOMM20 is strongly associated with the metastatic breast cancer cells. Rarely, MCT4(+) and TOMM20(-) epithelial cancer cells were also observed (black arrowhead). Original magnification, 60x.
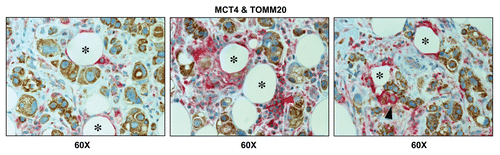
Figure 9 Visualizing the “reverse Warburg effect” by double labeling with MCT4 and TOMM20: Primary tumor tissue. Paraffin-embedded sections of human breast cancer primary tumors were immunostained with antibodies directed against MCT4 (red color) and TOMM20 (brown color). Slides were then counterstained with hematoxylin (blue color). Note that MCT4 staining is largely confined to the cancer-associated tumor stroma. In contrast, TOMM20 is specifically associated with the epithelial breast cancer cells. Two representative images are shown. Original magnification, 40x.
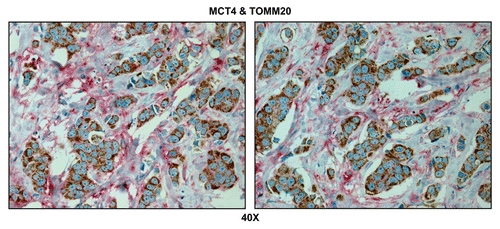
Figure 10 Tumor-associated inflammatory cells are glycolytic. Paraffin-embedded sections of human breast cancer primary tumors were immunostained with antibodies directed against MCT4 (red color) and TOMM20 (brown color). Slides were then counterstained with hematoxylin (blue color). Note that tumor-associated inflammatory cells (red arrowheads) are MCT4(+) and TOMM20(-), consistent with a glycolytic phenotype. In contrast, TOMM20 is strongly associated with the primary breast cancer cells. Two representative images are shown. Original magnification, 60x.
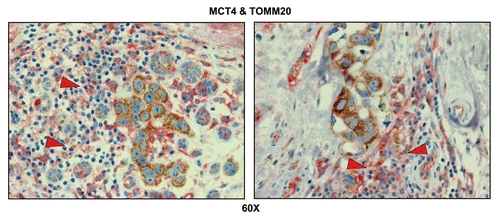
Figure 11 Mitochondrial metabolism and the “reverse Warburg effect” in cancer metastasis. Metastatic cancer cells secrete ROS, such as hydrogen peroxide, which induces oxidative stress in neighboring stromal cells. Oxidative stress, in turn, initiates the autophagic destruction of mitochondria (mitophagy), resulting in the onset of aerobic glycolysis. As a consequence, mitochondrial dysfunction in stromal cells leads to the production of high-energy mitochondrial fuels (L-lactate and ketone bodies) which are effluxed to the extracellular environment via MCT4. These fuels are then taken up by metastatic cancer cells and converted to Acetyl-CoA, which is “burned” in the TCA cycle and OXPHOS, via mitochondrial metabolism. Hence, metastatic cancer cells are mitochondria-rich and are positive for markers of mitochondrial mass (TOMM20) and OXPHOS activity (COX). Experimentally, glycolytic stromal cells included cancer-associated fibroblasts, adipocytes and inflammatory cells, which were MCT4(+) and TOMM20(-). LN, lymph node. ROS, reactive oxygen species, OXPHOS, oxidative mitochondrial metabolism.
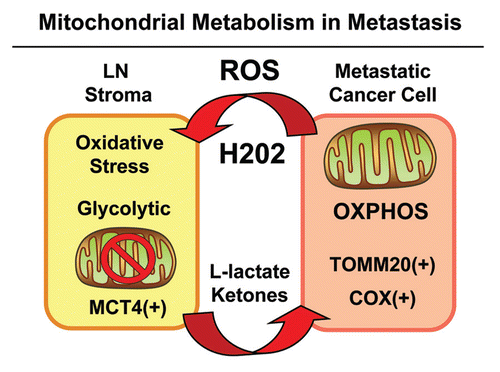
Acknowledgments
F.S. and her laboratory were supported by grants from the Breast Cancer Alliance (BCA) and the American Cancer Society (ACS). U.E.M. was supported by a Young Investigator Award from the Margaret Q. Landenberger Research Foundation. M.P.L. was supported by grants from the NIH/NCI (R01-CA-080250; R01-CA-098779; R01-CA-120876; R01-AR-055660) and the Susan G. Komen Breast Cancer Foundation. A.K.W. was supported by a Susan G. Komen Career Catalyst Grant. R.G.P. was supported by grants from the NIH/NCI (R01-CA-70896, R01-CA-75503, R01-CA-86072 and R01-CA-107382) and the Dr. Ralph and Marian C. Falk Medical Research Trust. The Kimmel Cancer Center was supported by the NIH/NCI Cancer Center Core grant P30-CA-56036 (to R.G.P.). Funds were also contributed by the Margaret Q. Landenberger Research Foundation (to M.P.L.). This project is funded, in part, under a grant with the Pennsylvania Department of Health (to M.P.L. and F.S.). The Department specifically disclaims responsibility for any analyses, interpretations or conclusions. This work was also supported, in part, by a Centre grant in Manchester from Breakthrough Breast Cancer in the UK (to A.H.) and an Advanced ERC Grant from the European Research Council.
References
- Witkiewicz AK, Dasgupta A, Sotgia F, Mercier I, Pestell RG, Sabel M, et al. An absence of stromal caveolin-1 expression predicts early tumor recurrence and poor clinical outcome in human breast cancers. Am J Pathol 2009; 174:2023 - 2034; PMID: 19411448; http://dx.doi.org/10.2353/ajpath.2009.080873
- Witkiewicz AK, Dasgupta A, Sammons S, Er O, Potoczek MB, Guiles F, et al. Loss of stromal caveolin-1 expression predicts poor clinical outcome in triple negative and basal-like breast cancers. Cancer Biol Ther 2010; 10:135 - 143; PMID: 20431349; http://dx.doi.org/10.4161/cbt.10.2.11983
- Sloan EK, Ciocca DR, Pouliot N, Natoli A, Restall C, Henderson MA, et al. Stromal cell expression of caveolin-1 predicts outcome in breast cancer. Am J Pathol 2009; 174:2035 - 2043; PMID: 19411449; http://dx.doi.org/10.2353/ajpath.2009.080924
- Koo JS, Park S, Kim SI, Lee S, Park BW. The impact of caveolin protein expression in tumor stroma on prognosis of breast cancer. Tumour Biol 2011; 32:787 - 799; PMID: 21584795; http://dx.doi.org/10.1007/s13277-011-0181-6
- Qian N, Ueno T, Kawaguchi-Sakita N, Kawashima M, Yoshida N, Mikami Y, et al. Prognostic significance of tumor/stromal caveolin-1 expression in breast cancer patients. Cancer Sci 2011; 102:1590 - 1596; PMID: 21585620; http://dx.doi.org/10.1111/j.1349-7006.2011.01985.x
- El-Gendi SM, Mostafa MF, El-Gendi AM. Stromal Caveolin-1 Expression in Breast Carcinoma. Correlation with Early Tumor Recurrence and Clinical Outcome. Pathol Oncol Res 2012; PMID: 22057638
- Simpkins S, Holliday D, Speirs V. The role of stromal caveolin-1 in breast cancer progression. NCRI Cancer Conference 2011; Absract #A222:http://www.ncri.org.uk/ncriconference/2011abstracts/abstracts/A222.html
- Witkiewicz AK, Dasgupta A, Nguyen KH, Liu C, Kovatich AJ, Schwartz GF, et al. Stromal caveolin-1 levels predict early DCIS progression to invasive breast cancer. Cancer Biol Ther 2009; 8:1071 - 1079; PMID: 19502809; http://dx.doi.org/10.4161/cbt.8.11.8874
- Di Vizio D, Morello M, Sotgia F, Pestell RG, Freeman MR, Lisanti MP. An absence of stromal caveolin-1 is associated with advanced prostate cancer, metastatic disease and epithelial Akt activation. Cell Cycle 2009; 8:2420 - 2424; PMID: 19556867; http://dx.doi.org/10.4161/cc.8.15.9116
- Wu KN, Queenan M, Brody JR, Potoczek M, Sotgia F, Lisanti MP, et al. Loss of stromal caveolin-1 expression in malignant melanoma metastases predicts poor survival. Cell Cycle 2011; 10:4250 - 4255; PMID: 22134245; http://dx.doi.org/10.4161/cc.10.24.18551
- Pavlides S, Whitaker-Menezes D, Castello-Cros R, Flomenberg N, Witkiewicz AK, Frank PG, et al. The reverse Warburg effect: aerobic glycolysis in cancer associated fibroblasts and the tumor stroma. Cell Cycle 2009; 8:3984 - 4001; PMID: 19923890; http://dx.doi.org/10.4161/cc.8.23.10238
- Pavlides S, Tsirigos A, Vera I, Flomenberg N, Frank PG, Casimiro MC, et al. Loss of stromal caveolin-1 leads to oxidative stress, mimics hypoxia and drives inflammation in the tumor microenvironment, conferring the “reverse Warburg effect”: a transcriptional informatics analysis with validation. Cell Cycle 2010; 9:2201 - 2219; PMID: 20519932; http://dx.doi.org/10.4161/cc.9.11.11848
- Pavlides S, Tsirigos A, Migneco G, Whitaker-Menezes D, Chiavarina B, Flomenberg N, et al. The autophagic tumor stroma model of cancer: Role of oxidative stress and ketone production in fueling tumor cell metabolism. Cell Cycle 2010; 9:3485 - 3505; PMID: 20861672; http://dx.doi.org/10.4161/cc.9.17.12721
- Trimmer C, Sotgia F, Whitaker-Menezes D, Balliet RM, Eaton G, Martinez-Outschoorn UE, et al. Caveolin-1 and mitochondrial SOD2 (MnSOD) function as tumor suppressors in the stromal microenvironment: a new genetically tractable model for human cancer associated fibroblasts. Cancer Biol Ther 2011; 11:383 - 394; PMID: 21150282; http://dx.doi.org/10.4161/cbt.11.4.14101
- Bonuccelli G, Whitaker-Menezes D, Castello-Cros R, Pavlides S, Pestell RG, Fatatis A, et al. The reverse Warburg effect: glycolysis inhibitors prevent the tumor promoting effects of caveolin-1 deficient cancer associated fibroblasts. Cell Cycle 2010; 9:1960 - 1971; PMID: 20495363; http://dx.doi.org/10.4161/cc.9.10.11601
- Martinez-Outschoorn UE, Pestell RG, Howell A, Tykocinski ML, Nagajyothi F, Machado FS, et al. Energy transfer in “parasitic” cancer metabolism: mitochondria are the powerhouse and Achilles' heel of tumor cells. Cell Cycle 2011; 10:4208 - 4216; PMID: 22033146; http://dx.doi.org/10.4161/cc.10.24.18487
- Martinez-Outschoorn UE, Sotgia F, Lisanti MP. Power surge: supporting cells “fuel” cancer cell mitochondria. Cell Metab 2012; 15:4 - 5; PMID: 22225869; http://dx.doi.org/10.1016/j.cmet.2011.12.011
- Martinez-Outschoorn UE, Pavlides S, Howell A, Pestell RG, Tanowitz HB, Sotgia F, et al. Stromal-epithelial metabolic coupling in cancer: integrating autophagy and metabolism in the tumor microenvironment. Int J Biochem Cell Biol 2011; 43:1045 - 1051; PMID: 21300172; http://dx.doi.org/10.1016/j.biocel.2011.01.023
- Martinez-Outschoorn UE, Whitaker-Menezes D, Pavlides S, Chiavarina B, Bonuccelli G, Casey T, et al. The autophagic tumor stroma model of cancer or “battery-operated tumor growth”: A simple solution to the autophagy paradox. Cell Cycle 2010; 9:4297 - 4306; PMID: 21051947; http://dx.doi.org/10.4161/cc.9.21.13817
- Sotgia F, Martinez-Outschoorn UE, Howell A, Pestell RG, Pavlides S, Lisanti MP. Caveolin-1 and cancer metabolism in the tumor microenvironment: markers, models and mechanisms. Annu Rev Pathol 2012; 7:423 - 467; PMID: 22077552; http://dx.doi.org/10.1146/annurev-pathol-011811-120856
- Sotgia F, Martinez-Outschoorn UE, Pavlides S, Howell A, Pestell RG, Lisanti MP. Understanding the Warburg effect and the prognostic value of stromal caveolin-1 as a marker of a lethal tumor microenvironment. Breast Cancer Res 2011; 13:213; PMID: 21867571; http://dx.doi.org/10.1186/bcr2892
- Pavlides S, Vera I, Gandara R, Sneddon S, Pestell RG, Mercier I, et al. Warburg Meets Autophagy: Cancer-Associated Fibroblasts Accelerate Tumor Growth and Metastasis via Oxidative Stress, Mitophagy and Aerobic Glycolysis. Antioxid Redox Signal 2011; PMID: 21883043; http://dx.doi.org/10.1089/ars.2011.4243
- Ertel A, Tsirigos A, Whitaker-Menezes D, Birbe RC, Pavlides S, Martinez-Outschoorn UE, et al. Is cancer a metabolic rebellion against host aging? In the quest for immortality, tumor cells try to save themselves by boosting mitochondrial metabolism. Cell Cycle 2012; 11:253 - 263; PMID: 22234241; http://dx.doi.org/10.4161/cc.11.2.19006
- Martinez-Outschoorn UE, Pavlides S, Whitaker-Menezes D, Daumer KM, Milliman JN, Chiavarina B, et al. Tumor cells induce the cancer associated fibroblast phenotype via caveolin-1 degradation: implications for breast cancer and DCIS therapy with autophagy inhibitors. Cell Cycle 2010; 9:2423 - 2433; PMID: 20562526; http://dx.doi.org/10.4161/cc.9.12.12048
- Martinez-Outschoorn UE, Balliet RM, Rivadeneira DB, Chiavarina B, Pavlides S, Wang C, et al. Oxidative stress in cancer associated fibroblasts drives tumor-stroma co-evolution: A new paradigm for understanding tumor metabolism, the field effect and genomic instability in cancer cells. Cell Cycle 2010; 9:3256 - 3276; PMID: 20814239; http://dx.doi.org/10.4161/cc.9.16.12553
- Martinez-Outschoorn UE, Trimmer C, Lin Z, Whitaker-Menezes D, Chiavarina B, Zhou J, et al. Autophagy in cancer associated fibroblasts promotes tumor cell survival: Role of hypoxia, HIF1 induction and NFκB activation in the tumor stromal microenvironment. Cell Cycle 2010; 9:3515 - 3533; PMID: 20855962; http://dx.doi.org/10.4161/cc.9.17.12928
- Martinez-Outschoorn UE, Lin Z, Trimmer C, Flomenberg N, Wang C, Pavlides S, et al. Cancer cells metabolically “fertilize” the tumor microenvironment with hydrogen peroxide, driving the Warburg effect: implications for PET imaging of human tumors. Cell Cycle 2011; 10:2504 - 2520; PMID: 21778829; http://dx.doi.org/10.4161/cc.10.15.16585
- Martinez-Outschoorn UE, Prisco M, Ertel A, Tsirigos A, Lin Z, Pavlides S, et al. Ketones and lactate increase cancer cell “stemness,” driving recurrence, metastasis and poor clinical outcome in breast cancer: achieving personalized medicine via Metabolo-Genomics. Cell Cycle 2011; 10:1271 - 1286; PMID: 21512313; http://dx.doi.org/10.4161/cc.10.8.15330
- Bonuccelli G, Tsirigos A, Whitaker-Menezes D, Pavlides S, Pestell RG, Chiavarina B, et al. Ketones and lactate “fuel” tumor growth and metastasis: Evidence that epithelial cancer cells use oxidative mitochondrial metabolism. Cell Cycle 2010; 9:3506 - 3514; PMID: 20818174; http://dx.doi.org/10.4161/cc.9.17.12731
- Szatrowski TP, Nathan CF. Production of large amounts of hydrogen peroxide by human tumor cells. Cancer Res 1991; 51:794 - 798; PMID: 1846317
- Ahn J, Gammon MD, Santella RM, Gaudet MM, Britton JA, Teitelbaum SL, et al. Associations between breast cancer risk and the catalase genotype, fruit and vegetable consumption and supplement use. Am J Epidemiol 2005; 162:943 - 952; PMID: 16192345; http://dx.doi.org/10.1093/aje/kwi306
- Goh J, Enns L, Fatemie S, Hopkins H, Morton J, Pettan-Brewer C, et al. Mitochondrial targeted catalase suppresses invasive breast cancer in mice. BMC Cancer 2011; 11:191; PMID: 21605372; http://dx.doi.org/10.1186/1471-2407-11-191
- Hyoudou K, Nishikawa M, Ikemura M, Kobayashi Y, Mendelsohn A, Miyazaki N, et al. Prevention of pulmonary metastasis from subcutaneous tumors by binary system-based sustained delivery of catalase. J Control Release 2009; 137:110 - 115; PMID: 19361547; http://dx.doi.org/10.1016/j.jconrel.2009.04.005
- Hyoudou K, Nishikawa M, Kobayashi Y, Umeyama Y, Yamashita F, Hashida M. PEGylated catalase prevents metastatic tumor growth aggravated by tumor removal. Free Radic Biol Med 2006; 41:1449 - 1458; PMID: 17023272; http://dx.doi.org/10.1016/j.freeradbiomed.2006.08.004
- Hyoudou K, Nishikawa M, Umeyama Y, Kobayashi Y, Yamashita F, Hashida M. Inhibition of metastatic tumor growth in mouse lung by repeated administration of polyethylene glycol-conjugated catalase: quantitative analysis with firefly luciferase-expressing melanoma cells. Clin Cancer Res 2004; 10:7685 - 7691; PMID: 15570002; http://dx.doi.org/10.1158/1078-0432.CCR-04-1020
- Ishii K, Zhen LX, Wang DH, Funamori Y, Ogawa K, Taketa K. Prevention of mammary tumorigenesis in acatalasemic mice by vitamin E supplementation. Jpn J Cancer Res 1996; 87:680 - 684; PMID: 8698615; http://dx.doi.org/10.1111/j.1349-7006.1996.tb00277.x
- Lisanti MP, Martinez-Outschoorn UE, Lin Z, Pavlides S, Whitaker-Menezes D, Pestell RG, et al. Hydrogen peroxide fuels aging, inflammation, cancer metabolism and metastasis: the seed and soil also needs “fertilizer”. Cell Cycle 2011; 10:2440 - 2449; PMID: 21734470; http://dx.doi.org/10.4161/cc.10.15.16870
- Whitaker-Menezes D, Martinez-Outschoorn UE, Lin Z, Ertel A, Flomenberg N, Witkiewicz AK, et al. Evidence for a stromal-epithelial “lactate shuttle” in human tumors: MCT4 is a marker of oxidative stress in cancer-associated fibroblasts. Cell Cycle 2011; 10:1772 - 1783; PMID: 21558814; http://dx.doi.org/10.4161/cc.10.11.15659
- Whitaker-Menezes D, Martinez-Outschoorn UE, Flomenberg N, Birbe RC, Witkiewicz AK, Howell A, et al. Hyperactivation of oxidative mitochondrial metabolism in epithelial cancer cells in situ: visualizing the therapeutic effects of metformin in tumor tissue. Cell Cycle 2011; 10:4047 - 4064; PMID: 22134189; http://dx.doi.org/10.4161/cc.10.23.18151
- Ullah MS, Davies AJ, Halestrap AP. The plasma membrane lactate transporter MCT4, but not MCT1, is upregulated by hypoxia through a HIF-1alpha-dependent mechanism. J Biol Chem 2006; 281:9030 - 9037; PMID: 16452478; http://dx.doi.org/10.1074/jbc.M511397200
- Bergersen LH. Is lactate food for neurons? Comparison of monocarboxylate transporter subtypes in brain and muscle. Neuroscience 2007; 145:11 - 19; PMID: 17218064; http://dx.doi.org/10.1016/j.neuroscience.2006.11.062
- Tritschler HJ, Bonilla E, Lombes A, Andreetta F, Servidei S, Schneyder B, et al. Differential diagnosis of fatal and benign cytochrome c oxidase-deficient myopathies of infancy: an immunohistochemical approach. Neurology 1991; 41:300 - 305; PMID: 1846953
- Dimauro S. A history of mitochondrial diseases. J Inherit Metab Dis 2011; 34:261 - 276; PMID: 20490929; http://dx.doi.org/10.1007/s10545-010-9082-x
- DiMauro S, Gurgel-Giannetti J. The expanding phenotype of mitochondrial myopathy. Curr Opin Neurol 2005; 18:538 - 542; PMID: 16155436; http://dx.doi.org/10.1097/01.wco.0000179761.63486.1a
- DiMauro S, Schon EA. Mitochondrial respiratory-chain diseases. N Engl J Med 2003; 348:2656 - 2668; PMID: 12826641; http://dx.doi.org/10.1056/NEJMra022567
- Gallagher SM, Castorino JJ, Wang D, Philp NJ. Monocarboxylate transporter 4 regulates maturation and trafficking of CD147 to the plasma membrane in the metastatic breast cancer cell line MDA-MB-231. Cancer Res 2007; 67:4182 - 4189; PMID: 17483329; http://dx.doi.org/10.1158/0008-5472.CAN-06-3184
- Dimmer KS, Friedrich B, Lang F, Deitmer JW, Bröer S. The low-affinity monocarboxylate transporter MCT4 is adapted to the export of lactate in highly glycolytic cells. Biochem J 2000; 350:219 - 227; PMID: 10926847; http://dx.doi.org/10.1042/0264-6021:3500219
- Witkiewicz AK, Whitaker-Menezes D, Dasgupta A, Philp NJ, Lin Z, Gandara R, et al. Using the “reverse Warburg effect” to identify high-risk breast cancer patients: Stromal MCT4 predicts poor clinical outcome in triple-negative breast cancers. Cell Cycle 2012; 11:1108 - 1117; PMID: 22313602
- Galluzzi L, Kepp O, Kroemer G. Reverse Warburg: Straight to cancer. Cell Cycle 2012; 11:1059; PMID: 22343921
- Lisanti MP, Martinez-Outschoorn UE, Pavlides S, Whitaker-Menezes D, Pestell RG, Howell A, et al. Accelerated aging in the tumor microenvironment: connecting aging, inflammation and cancer metabolism with personalized medicine. Cell Cycle 2011; 10:2059 - 2063; PMID: 21654190; http://dx.doi.org/10.4161/cc.10.13.16233
- Nishikawa M, Hashida M, Takakura Y. Catalase delivery for inhibiting ROS-mediated tissue injury and tumor metastasis. Adv Drug Deliv Rev 2009; 61:319 - 326; PMID: 19385054; http://dx.doi.org/10.1016/j.addr.2009.01.001
- Nishikawa M, Hyoudou K, Kobayashi Y, Umeyama Y, Takakura Y, Hashida M. Inhibition of metastatic tumor growth by targeted delivery of antioxidant enzymes. J Control Release 2005; 109:101 - 117; PMID: 16256238; http://dx.doi.org/10.1016/j.jconrel.2005.09.017
- Nishikawa M, Tamada A, Hyoudou K, Umeyama Y, Takahashi Y, Kobayashi Y, et al. Inhibition of experimental hepatic metastasis by targeted delivery of catalase in mice. Clin Exp Metastasis 2004; 21:213 - 221; PMID: 15387371; http://dx.doi.org/10.1023/B:CLIN.0000037706.13747.5e
- Nechuta S, Lu W, Chen Z, Zheng Y, Gu K, Cai H, et al. Vitamin supplement use during breast cancer treatment and survival: a prospective cohort study. Cancer Epidemiol Biomarkers Prev 2011; 20:262 - 271; PMID: 21177425; http://dx.doi.org/10.1158/1055-9965.EPI-10-1072
- Sotgia F, Martinez-Outschoorn UE, Lisanti MP. Mitochondrial oxidative stress drives tumor progression and metastasis: should we use antioxidants as a key component of cancer treatment and prevention?. BMC Med 2011; 9:62; PMID: 21605374; http://dx.doi.org/10.1186/1741-7015-9-62
- Balliet RM, Capparelli C, Guido C, Pestell TG, Martinez-Outschoorn UE, Lin Z, et al. Mitochondrial oxidative stress in cancer-associated fibroblasts drives lactate production, promoting breast cancer tumor growth: understanding the aging and cancer connection. Cell Cycle 2011; 10:4065 - 4073; PMID: 22129993; http://dx.doi.org/10.4161/cc.10.23.18254