Abstract
The 49-member human ATP binding cassette (ABC) gene family encodes 44 membrane transporters for lipids, ions, peptides or xenobiotics, four translation factors without transport activity, as they lack transmembrane domains, and one pseudogene. To understand the roles of ABC genes in pluripotency and multipotency, we performed a sensitive qRT-PCR analysis of their expression in embryonic stem cells (hESCs), bone marrow-derived mesenchymal stem cells (hMSCs) and hESC-derived hMSCs (hES-MSCs). We confirm that hES-MSCs represent an intermediate developmental stage between hESCs and hMSCs. We observed that 44 ABCs were significantly expressed in hESCs, 37 in hES-MSCs and 35 in hMSCs. These variations are mainly due to plasma membrane transporters with low but significant gene expression: 18 are expressed in hESCs compared with 16 in hES-MSCs and 8 in hMSCs, suggesting important roles in pluripotency. Several of these ABCs shared similar substrates but differ regarding gene regulation. ABCA13 and ABCB4, similarly to ABCB1, could be new markers to select primitive hMSCs with specific plasma membrane transporterlow phenotypes. ABC proteins performing basal intracellular functions, including translation factors and mitochondrial heme transporters, showed the highest constant gene expression among the three populations. Peptide transporters in the endoplasmic reticulum, Golgi and lysosome were well expressed in hESCs and slightly upregulated in hMSCs, which play important roles during the development of stem cell niches in bone marrow or meningeal tissue. These results will be useful to study specific cell cycle regulation of pluripotent stem cells or ABC dysregulation in complex pathologies, such as cancers or neurological disorders.
Introduction
There are 49 ATP binding cassette (ABC) genes in humans; these are part of a very large super-family represented in all living cells. Most ABC genes encode transmembrane transporters composed of two nucleotide binding domains (NBDs) and two transmembrane domains (TMDs), both of which are necessary for their activity.1,2 ABCD, ABCG and some ABCB genes encode half-transporters composed of only one TMD and one NBD; they must homo- or hetero-dimerize to be biologically functional. ABC functions include the active uptake or efflux of important lipids, peptides, ions or xenobiotics across the plasma membrane (PM) or intracellular membranes of the endoplasmic reticulum, Golgi, peroxisome, mitochondria and lysosome.2-5 By presenting specific lipids on the outer plasma membrane, some ABCs, especially of the ABCA subfamily, are also implicated in signaling apoptosis.6,7 Interestingly, ABCE and ABCF subfamily proteins are truncated and lack a TMD; therefore, they have no transport function but play important roles in mRNA translation.8-10 Functional connections exist between ABC expression and the pluripotency of embryonic stem cells, multipotency in early adult stem cells and cancer stem cells. Indeed, the expression of some ABC genes is under the control of pluripotency stem cell transcription factors, such as Oct-4 11,12 and SALL4.13 ABCB1 and ABCG2, which promote multidrug resistance (MDR) activity, are markers for a “side population” (SP) of pluripotent, multipotent or cancerous stem cells.14-16 We and others previously demonstrated the enrichment by cell sorting of multipotent adult stem cells, represented by a growth factor receptorlow subpopulation.17-22 We demonstrated that receptorlow stem cells respond to low cytokine concentrations for long-term self-renewal.22,23 Consequently, the differential expression of ABC genes could provide further possibilities for this approach to be utilized to enrich and analyze normal primitive or cancerous stem cells.
To investigate mechanisms underlying determination in stem cells, we previously developed and characterized an experimental system involving three cell types: pluripotent embryonic stem cells (hESCs), multipotent mesenchymal stem cells (hMSCs) and hESC-derived hMSCs (hES-MSCs). We and others selected hES-MSCs and demonstrated that they represent a more primitive population than hMSCs.24-28 hMSCs play a major role in constituting and controlling various stem cell niches in bone marrow29 or the meningeal barrier;30 in this context, it is important to note that ABC transporters control the trafficking of peptides, including pleiotropic cytokines such as interleukin-1β, that could affect stem cell self-renewal, determination or apoptosis.31,32
In this study, we used a highly sensitive qRT-PCR technique to measure and compare the expressions of all 49 human ABC genes in hESCs, hES-MSCs and hMSCs. We distinguish ABC genes displaying consistent high expression in the three populations, and those showing significant expression differences, and which may play roles during the transition from hESCs through hES-MSCs to hMSCs. These observations will be useful to better study the increasing number of pathologies in which ABC genes are mutated or their functions dysregulated.33-35
Results
Expression levels of all 49 ABC genes in hESCs, hES-MSCs and hMSCs were determined by qRT-PCR analysis. A visual overview of these data are provided in a heat-map (), which presents ABCs according to gene expression levels, together with known substrates, functions, subcellular localizations and associated diseases. summarizes the genes with high or medium (NCt < 29), low but significant (29 ≤ NCt < 35) and undetected (NCt ≥ 35) expression in each cell population. We observed that hESCs expressed a larger number (n = 44) of ABC genes compared with hES-MSCs (n = 37) and late hMSCs (n = 35). This suggests that these genes may have roles in maintaining pluripotency or in early hMSC development. This also confirms our previous results that hES-MSCs represent an intermediate developmental stage between hESCs and hMSCs.24 Due to the high sensitivity of the qRT-PCR technique, we can observe in that the genes encoding ABC plasma membrane transporters are clearly better represented in hESCs (n = 20) than in the late-hMSC population (n = 8). This is also consistent with the fact that various receptorlow subpopulations have been widely studied to select more primitive stem cells.17-22
Figure 1. Heat maps showing the expression levels of the 49 human ABC genes in hESCs, hES-MSCs and hMSCs. (A) Expression of all 49 human ABC genes in 14 samples of three stem cell types (hESCs, hES-MSCs and hMSCs). The 49 ABC genes are shown on the x-axis, ordered from high to low expression in each sub-family (ABCA to ABCG), according to the mean NCt values from the six hESC samples. The 14 samples are ordered on the y-axis with biological replicates (“a” and “b”). The color code ranges from high (NCt = 20, light green) through medium (black), to low but significant (NCt = 34.9, red) or undetectable (NCt ≥ 35, orange) gene expression. For each ABC, the structure, subcellular localization, function and substrates or association with human diseasesCitation3-Citation5,Citation12,Citation33,Citation34 are represented by symbols, as detailed in the figure. (B) Overviews of mean ABC gene expression in the three stem cell types. ABC genes are ordered from high to low expression levels according to mean NCt values. Gene expression levels are sub-divided into categories as follows: high or medium (NCt < 29), low but significant (29 ≤ NCt < 35), and undetectable (NCt ≥ 35). We can observe that the ABCs with high gene expression are those representing intracellular basal functions. Virtually all ABCs with low but significant expression are plasma membrane transporters whose expression levels vary between the three stem cell populations. Seven of these genes (marked with asterisks) are expressed exclusively in pluripotent or primitive multipotent stem cells.
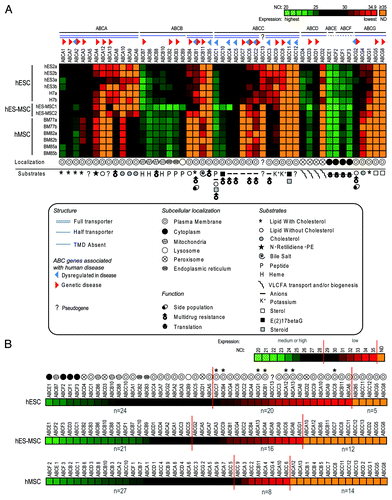
and radial plots in show that ABC gene expression in hESCs covered a wide range: 24 ABC genes were well expressed (NCt < 29); 20 had a low but significant expression (29 ≤ NCt < 35), and only five were not expressed at detectable levels (NCt ≥ 35). A quantitative and statistical comparison of the expression of all ABC genes in hESCs compared with hES-MSCs and hMSCs allowed the identification of genes without () or with significant variation ().
Figure 2. Expression of the 49 human ABC genes in hESCs. ABC genes are classified into subgroups as follows: (A) ABCA; (B) ABCB; (C) ABCC; (D) ABC-D, -E, -F and -G. Radial plots are sub-divided in areas as follows: high (NCt < 29, white), low but significant (29 ≤ NCt < 35, light-gray) and undetectable (NCt ≥ 35, gray) gene expression. Arrows and their directions indicate significant gene expression variations during development of hESCs to hES-MSCs and/or hMSCs. Information relating to structure, disease associations, subcellular localizations (bold) and substrates and specific functions (italics) derived from referencesCitation3-Citation5,Citation12,Citation33,Citation34 are detailed in the figure.
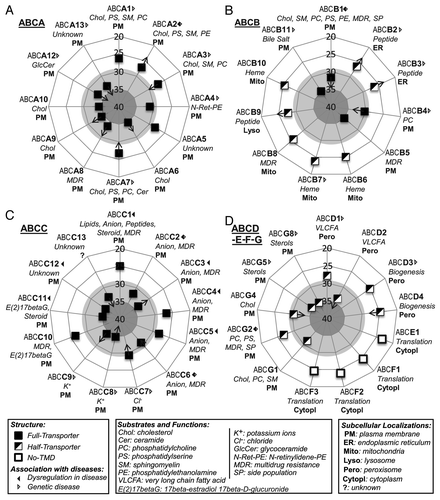
Table 1. ABC genes displaying similar expression in hESCs, hES-MSCs and hMSCs
Figure 3. Twenty-three ABC genes are significantly up- or downregulated during the development of hESCs toward hES-MSCs and hMSCs. Compared with the hESC reference group, relative quantification and standard deviation values for hES-MSCs (white) and hMSCs (hatched) were calculated as previously describedCitation24,Citation76 with the five reference genes indicated in Materials and Methods. Statistical significance (p < 0.05) is indicated by asterisks. For hESCs, mean NCt (± SD) values are shown below the x-axis. ABC genes are categorized into four sets, based on subcellular localizations and substrates: (A) intracellular transporters; (B) plasma membrane lipid transporters; (C) plasma membrane ion transporters; (D) ABCs with unknown function or substrate. Bold downward-pointing arrows (↓) indicate the abolition of gene expression (defined as NCt ≥ 35) in hES-MSCs or hMSCs. Abbreviations for substrates and specific functions (italics), and subcellular localizations (bold), are as described in .
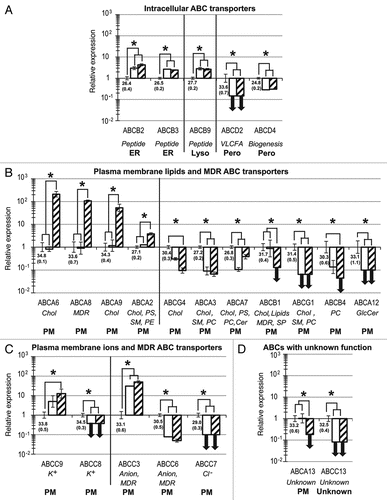
Intracellular ABC members.
ABCE1 and the ABCF subfamily members, which lack a TMD, do not act as transporters, but as important translation factors.8-10 These genes showed the highest expression levels in this study, without significant differences between hESCs, hES-MSCs or hMSCs ( and Fig. S2).
ABCB6, ABCB7 and ABCB10, which encode mitochondrial membrane heme transporters,36-38 and ABCB8, whose MDR function protects mitochondrial DNA,39 remained highly and stably expressed during mesodermal development ( and Fig. S2). Three peptide transporters: ABCB2 and ABCB3 (endoplasmic reticulum)40 and ABCB9 (lysosome),41 were well expressed () and slightly upregulated between hESCs and both mesenchymal populations (). This is consistent with the provision by hMSCs of stem cell niches (see ) for pluripotent and multipotent stem cells.29,30,42 ABC transporters in these stem cell niches could promote the traffic of peptides with pleiotropic effects on self-renewal or differentiation.31,32
Figure 3E. (E) A typical Hematopoietic Stem Cell (HSC) niche. Shown is a small HSC with a high nucleo-cytoplasmic ratio and prominent nucleoli, embedded in an adipocyte full of lipid droplets. Strong plasma membrane interdigitation is observed between the adipocyte and the HSC, suggesting possible lipid transport.
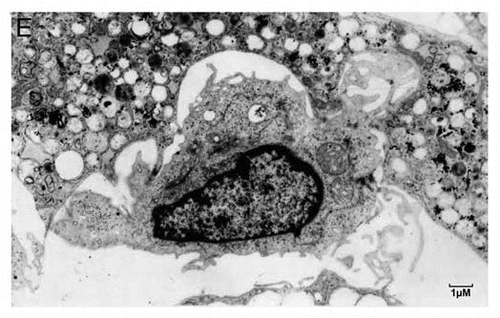
The four half-transporters of the ABCD subfamily are located in the peroxisome, being involved in energy production.43 ABCD1 and ABCD3, which are involved in neuropathologies,33 were not modulated during mesenchymal development ( and ), whereas ABCD2 and ABCD4 were downregulated ( and ).
Plasma membrane ABC transporters (PM-ABCs).
PM-ABCs with significant expression variation.
As shown in , lipid transporters with overlapping substrates, and functions often showed different gene regulation. This is particularly true for ABC cholesterol transporters, which were highly upregulated in hMSCs for ABCA6 and ABCA9 (), and slightly for ABCA2, whereas they were downregulated or even abolished for ABCG4, ABCA3, ABCA7, ABCB1 and ABCG1 (). Similarly for ABCs with MDR functions, ABCA8 was highly upregulated, while ABCB1 expression was abolished in hMSCs (). The overlapping nature of ABC substrates could partly explain why research on ABC inhibitors to study the functions of these genes or the suppression of drug resistance has proved so challenging.44
Among ABC lipid transporter genes that were up- or downregulated, most of them displayed low but significant expression levels in hESCs. Only ABCA2 and ABCA7, which have been identified as genetic risk factors in Alzheimer disease,34,45,46 and ABCA3, an important transporter mutated in neonatal surfactant deficiency disease,33,34 were well expressed by hESCs.
Compared with hESCs, ABCB1 was stably expressed in hES-MSCs but undetected in hMSCs (). ABCB1 could therefore be a useful membrane marker for the selection of more primitive hMSCs from bone marrow, similar to hES-MSCs. However, ABCB1 has already been described for its important MDR function involved in SP selection. This MDR function complicates cancer chemotherapeutic treatment but protects the brain by the blood-brain barrier. By exporting xenobiotics or small peptides, Parkinson or Alzheimer diseases could be prevented.34 ABCB4 is a specific phosphatidylcholine transporter, and its defect causes intrahepatic cholestasis.33,34 Similarly to ABCB1, ABCB4 could be a new membrane marker for the selection of primitive hMSCs with an ABCB4low phenotype.
Among the ABCC subfamily members, we observed two groups of ion transporters (ABCC8 and ABCC9, and ABCC3, ABCC6 and ABCC7) that underwent opposite changes in regulation from hESCs to early and late hMSCs (). This again stresses the fact that different transporters for similar substrates might have quite different gene regulation. ABCC7 was not expressed during mesodermal development () but during endodermal development, where its mutation causes cystic fibrosis.47,48
Stably-expressed PM-ABCs.
Some PM-ABCs maintained a stable gene expression during mesenchymal development ( and Fig. S2). Apart from ABCC1 and ABCC10, these ABC genes have a medium or low but significant expression ( and Fig. S2). They transport lipids (ABCA1, ABCA4, ABCA10, ABCC1 and ABCG2), anions (ABCC1, ABCC2, ABCC4 and ABCC5), xenobiotics (ABCC1, ABCC2, ABCC4, ABCC5, ABCC10 and ABCG2), bile salts (ABCB11) or have unknown substrates (ABCA5) ().3-5 Four ABCs (ABCC1, ABCC2, ABCC4 and ABCC5) combine MDR and anion transport activities together.
ABCs with unknown function.
ABCA5 and ABCA13 are full transporters localized on the plasma membrane, but their substrates are as yet unknown. ABCA5 remained well and stably expressed in all three cell populations ( and Fig. S2).
Expression of ABCA13 was unchanged between hESCs and hES-MSCs but was abolished in hMSCs (), making this gene a possible new candidate for the selection of primitive hMSCs, comparable to ABCB4. ABCA13 has been implicated in schizophrenia, bipolar disorder and depression,49 but initial results need to be confirmed.50
Expression of ABCC13, a pseudogene,51 was detected in hESCs, but abolished in both hES-MSCs and hMSCs (). ABCC13 gene expression has been detected in fetal liver and primitive Hematopoietic Stem Cells (HSCs),51,52 but its functions in hESCs or HSCs remain to be clarified.
Undetected ABCs.
Only five ABCs (ABCB5, ABCC11, ABCC12, ABCG5 and ABCG8) were not detected in hESCs, hES-MSCs and hMSCs ( and and ). They only appear during endoderm and ectoderm development.53-55 They transport steroids (ABCC11) or sterols (ABCG5 and ABCG8), or have MDR (ABCB5) or unknown activities (ABCC12) ().
Discussion
This study has analyzed for the first time the expression of all 49 human ABC genes in self-renewing pluripotent hESCs and in early and late multipotent mesenchymal stem cell cultures. Notwithstanding post-transcriptional and posttranslational regulation, strikingly high concordances have been described between the levels of specific mRNAs and those of the corresponding proteins within the same cell population, both for stem cells56 or adult differentiated tissues.57,58
Previous studies that selected the most primitive multipotent stem cells (such as mesenchymal, epidermal or hematopoietic primitive stem cells), which rapidly lose pluripotent, symmetric and exponential self renewal, revealed that these cells express cytokine receptors at much lower levels than later asymmetrically and non-exponentially dividing multipotent stem cells.22,59-61 PM-ABCs mediate the transport of various signaling molecules, such as peptides into and out of the cell, and modulate the lipid environment of membranes surrounding cytokine receptors, affecting their internalization and thus signaling function.2,3,62 Consistent with the expression of cytokine receptors in early stem cells and the role of PM-ABCs in cytokine signaling, in the present study we observed that it was the pluripotent hESCs that displayed the largest number of low-expressed PM-ABC transporters (). The requirement for a substantial proportion of these ABCs appears to be hESC-specific, as the expression of seven of the 18 “low-expressed” PM-ABC transporters was abolished during the mesenchymal development process. Among these genes, we identified ABCA13 and ABCB4 as well as ABCB114,63 as good candidates for new markers for the enrichment from hMSCs of more primitive stem cell populations, such as hES-MSCs. In contrast to many ABC plasma membrane transporters, intracellular ABCs that control basal functions such as translation or energy production display a much higher level of expression, which remains constant during the development from hESCs to hMSCs.
It is well established that hMSCs play important roles in forming and maintaining stem cell niches,29 for example in bone marrow (as illustrated in ). Our data on the differential expression of ABC genes between hESCs and hMSCs may help shed light on these mechanisms. Previous studies indicate that membrane lipid composition and receptor or cytokine intracellular trafficking play an important role,3,64 but more in-depth functional studies are still needed to understand the mechanisms involved.
One adult tissue derived from mesenchymal cells is the meningeal barrier of the brain. The expression of ABC transporters in this tissue is vital to maintain the equilibrium of lipids, peptides and small molecules within this compartment, and thus for normal brain function.34,65 Consistent with this, the dysregulation of several ABC genes is associated with various neurological disorders, including Parkinson and Alzheimer diseases.34,45 As the etiologies of these conditions are likely multi-factorial in nature, an understanding of the roles of ABCs will require collaborative efforts between clinicians, geneticists and cell and molecular biologists.
Materials and Methods
Cell lines and culture conditions.
hES2 and hES3 cell lines were provided by ES Cell International (ESI) and H7 by the Wisconsin Institute. They were cultivated according to supplier’s instructions or as previously described in references 24, 66 and 67.
Two different hES-MSC lines were used. The first hES-MSC1 (P51RAC), obtained from hESC H1,68 was generously provided by Dr. Emmanuel Olivier and Dr. Eric E. Bouhassira.25 The second was derived from hES2 in our laboratory as previously described in reference 24. Human bone-marrow derived hMSCs were obtained, isolated and amplified24 in accordance with international guidelines and French bioethics laws. All stem cells used for expression analysis were of a high quality, according to morphological assessment and pluripotency-marker expression.24,66,67
A long-term human Dexter-type culture providing a Hematopoietic Stem Cell (HSC) niche, used for the image in , was generated as previously described in reference 69.
Gene expression analysis.
Preparation of stem cell mRNA, cDNA and quantitative real-time PCR (qRT-PCR) were performed as previously described in reference 24. Microfluidic TaqMan® Human Endogenous Control Arrays (Applied Biosystems) and TaqMan® ABC gene Arrays were used as previously described in reference 70 and 71. Five reference genes (RPLP0, POLR2A, IPO8, PPIA, GAPDH) were chosen according to their consensus stability values (Fig. S1 and Table S1),72‑75 and their geometric mean expression levels applied to derive normalized cycle threshold (NCt) and 2-NCt values for the expression of ABC genes, as previously described in reference 24. Relative quantifications and standard deviation (SD) of gene expression were calculated using the 2-ΔΔCt method,24,76 and statistical significance tests were performed as previously described in reference 24.
Additional material
Download Zip (869.9 KB)Acknowledgements
This work was supported by the Centre National de la Recherche Scientifique (CNRS)-UPR9045 and by the FP6 European Integrated Project “Genostem” (#503161). R.B. was supported by a CNRS Fellowship (509723), and I.P. was supported by a Fellowship from Prof. R. Frydman. We thank Dr. E. Olivier and Dr. E.E. Bouhassira for supplying the P51RAC (hES-MSC1) cell line. The work in this paper benefited from the facilities of the QPCR platform of IMAGIF (Centre de Recherche de Gif, www.imagif.cnrs.fr).
Disclosure of Potential Conflicts of Interest
The authors indicate no potential conflicts of interest.
Note
Supplemental material can be found at: www.landesbioscience.com/journals/cc/article/20023
References
- Higgins CF. ABC transporters: from microorganisms to man. Annu Rev Cell Biol 1992; 8:67 - 113; http://dx.doi.org/10.1146/annurev.cb.08.110192.000435; PMID: 1282354
- Dean M, Rzhetsky A, Allikmets R. The human ATP-binding cassette (ABC) transporter superfamily. Genome Res 2001; 11:1156 - 66; http://dx.doi.org/10.1101/gr.GR-1649R; PMID: 11435397
- Quazi F, Molday RS. Lipid transport by mammalian ABC proteins. Essays Biochem 2011; 50:265 - 90; http://dx.doi.org/10.1042/bse0500265; PMID: 21967062
- Gillet JP, Efferth T, Remacle J. Chemotherapy-induced resistance by ATP-binding cassette transporter genes. Biochim Biophys Acta 2007; 1775:237 - 62; PMID: 17572300
- Slot AJ, Molinski SV, Cole SP. Mammalian multidrug-resistance proteins (MRPs). Essays Biochem 2011; 50:179 - 207; http://dx.doi.org/10.1042/bse0500179; PMID: 21967058
- Aye IL, Singh AT, Keelan JA. Transport of lipids by ABC proteins: interactions and implications for cellular toxicity, viability and function. Chem Biol Interact 2009; 180:327 - 39; http://dx.doi.org/10.1016/j.cbi.2009.04.012; PMID: 19426719
- Hamon Y, Chambenoit O, Chimini G. ABCA1 and the engulfment of apoptotic cells. Biochim Biophys Acta 2002; 1585:64 - 71; PMID: 12531538
- Kerr ID. Sequence analysis of twin ATP binding cassette proteins involved in translational control, antibiotic resistance, and ribonuclease L inhibition. Biochem Biophys Res Commun 2004; 315:166 - 73; http://dx.doi.org/10.1016/j.bbrc.2004.01.044; PMID: 15013441
- Tyzack JK, Wang X, Belsham GJ, Proud CG. ABC50 interacts with eukaryotic initiation factor 2 and associates with the ribosome in an ATP-dependent manner. J Biol Chem 2000; 275:34131 - 9; http://dx.doi.org/10.1074/jbc.M002868200; PMID: 10931828
- Chen ZQ, Dong J, Ishimura A, Daar I, Hinnebusch AG, Dean M. The essential vertebrate ABCE1 protein interacts with eukaryotic initiation factors. J Biol Chem 2006; 281:7452 - 7; http://dx.doi.org/10.1074/jbc.M510603200; PMID: 16421098
- Marques DS, Sandrini JZ, Boyle RT, Marins LF, Trindade GS. Relationships between multidrug resistance (MDR) and stem cell markers in human chronic myeloid leukemia cell lines. Leuk Res 2010; 34:757 - 62; http://dx.doi.org/10.1016/j.leukres.2009.11.004; PMID: 19969351
- Wang XQ, Ongkeko WM, Chen L, Yang ZF, Lu P, Chen KK, et al. Octamer 4 (Oct4) mediates chemotherapeutic drug resistance in liver cancer cells through a potential Oct4-AKT-ATP-binding cassette G2 pathway. Hepatology 2010; 52:528 - 39; http://dx.doi.org/10.1002/hep.23692; PMID: 20683952
- Jeong HW, Cui W, Yang Y, Lu J, He J, Li A, et al. SALL4, a stem cell factor, affects the side population by regulation of the ATP-binding cassette drug transport genes. PLoS ONE 2011; 6:e18372; http://dx.doi.org/10.1371/journal.pone.0018372; PMID: 21526180
- Goodell MA, Brose K, Paradis G, Conner AS, Mulligan RC. Isolation and functional properties of murine hematopoietic stem cells that are replicating in vivo. J Exp Med 1996; 183:1797 - 806; http://dx.doi.org/10.1084/jem.183.4.1797; PMID: 8666936
- Hirschmann-Jax C, Foster AE, Wulf GG, Nuchtern JG, Jax TW, Gobel U, et al. A distinct “side population” of cells with high drug efflux capacity in human tumor cells. Proc Natl Acad Sci USA 2004; 101:14228 - 33; http://dx.doi.org/10.1073/pnas.0400067101; PMID: 15381773
- Bunting KD. ABC transporters as phenotypic markers and functional regulators of stem cells. Stem Cells 2002; 20:11 - 20; http://dx.doi.org/10.1002/stem.200011; PMID: 11796918
- Gunji Y, Nakamura M, Osawa H, Nagayoshi K, Nakauchi H, Miura Y, et al. Human primitive hematopoietic progenitor cells are more enriched in KITlow cells than in KIThigh cells. Blood 1993; 82:3283 - 9; PMID: 7694677
- Sansilvestri P, Cardoso AA, Batard P, Panterne B, Hatzfeld A, Lim B, et al. Early CD34high cells can be separated into KIThigh cells in which transforming growth factor-beta (TGF-beta) downmodulates c-kit and KITlow cells in which anti-TGF-beta upmodulates c-kit. Blood 1995; 86:1729 - 35; PMID: 7544639
- Kawashima I, Zanjani ED, Almaida-Porada G, Flake AW, Zeng H, Ogawa M. CD34+ human marrow cells that express low levels of Kit protein are enriched for long-term marrow-engrafting cells. Blood 1996; 87:4136 - 42; PMID: 8639771
- Li A, Simmons PJ, Kaur P. Identification and isolation of candidate human keratinocyte stem cells based on cell surface phenotype. Proc Natl Acad Sci USA 1998; 95:3902 - 7; http://dx.doi.org/10.1073/pnas.95.7.3902; PMID: 9520465
- Xiao M, Oppenlander BK, Plunkett JM, Dooley DC. Expression of Flt3 and c-kit during growth and maturation of human CD34+CD38- cells. Exp Hematol 1999; 27:916 - 27; http://dx.doi.org/10.1016/S0301-472X(99)00020-X; PMID: 10340408
- Fortunel NO, Hatzfeld JA, Rosemary PA, Ferraris C, Monier MN, Haydont V, et al. Long-term expansion of human functional epidermal precursor cells: promotion of extensive amplification by low TGF-beta1 concentrations. J Cell Sci 2003; 116:4043 - 52; http://dx.doi.org/10.1242/jcs.00702; PMID: 12953061
- Fortunel NO, Hatzfeld A, Hatzfeld JA. Transforming growth factor-beta: pleiotropic role in the regulation of hematopoiesis. Blood 2000; 96:2022 - 36; PMID: 10979943
- Barbet R, Peiffer I, Hatzfeld A, Charbord P, Hatzfeld JA. Comparison of Gene Expression in Human Embryonic Stem Cells, hESC-Derived Mesenchymal Stem Cells and Human Mesenchymal Stem Cells. Stem Cells Int 2011; 2011:368192; http://dx.doi.org/10.4061/2011/368192; PMID: 21941565
- Olivier EN, Rybicki AC, Bouhassira EE. Differentiation of human embryonic stem cells into bipotent mesenchymal stem cells. Stem Cells 2006; 24:1914 - 22; http://dx.doi.org/10.1634/stemcells.2005-0648; PMID: 16644919
- Arpornmaeklong P, Brown SE, Wang Z, Krebsbach PH. Phenotypic characterization, osteoblastic differentiation, and bone regeneration capacity of human embryonic stem cell-derived mesenchymal stem cells. Stem Cells Dev 2009; 18:955 - 68; http://dx.doi.org/10.1089/scd.2008.0310; PMID: 19327009
- Gruenloh W, Kambal A, Sondergaard C, McGee J, Nacey C, Kalomoiris S, et al. Characterization and in vivo testing of mesenchymal stem cells derived from human embryonic stem cells. Tissue Eng Part A 2011; 17:1517 - 25; http://dx.doi.org/10.1089/ten.tea.2010.0460; PMID: 21275830
- Choo A, Lim SK. Derivation of mesenchymal stem cells from human embryonic stem cells. Methods Mol Biol 2011; 690:175 - 82; http://dx.doi.org/10.1007/978-1-60761-962-8_12; PMID: 21042993
- Moore MA, Sheridan AP, Allen TD, Dexter TM. Prolonged hematopoiesis in a primate bone marrow culture system: characteristics of stem cell production and the hematopoietic microenvironment. Blood 1979; 54:775 - 93; PMID: 476301
- Johansson PA, Cappello S, Götz M. Stem cells niches during development--lessons from the cerebral cortex. Curr Opin Neurobiol 2010; 20:400 - 7; http://dx.doi.org/10.1016/j.conb.2010.04.003; PMID: 20447822
- Marty V, Médina C, Combe C, Parnet P, Amédée T. ATP binding cassette transporter ABC1 is required for the release of interleukin-1beta by P2X7-stimulated and lipopolysaccharide-primed mouse Schwann cells. Glia 2005; 49:511 - 9; http://dx.doi.org/10.1002/glia.20138; PMID: 15578659
- Frye BC, Halfter S, Djudjaj S, Muehlenberg P, Weber S, Raffetseder U, et al. Y-box protein-1 is actively secreted through a non-classical pathway and acts as an extracellular mitogen. EMBO Rep 2009; 10:783 - 9; http://dx.doi.org/10.1038/embor.2009.81; PMID: 19483673
- Schuierer MM, Langmann T. Molecular diagnosis of ATP-binding cassette transporter-related diseases. Expert Rev Mol Diagn 2005; 5:755 - 67; http://dx.doi.org/10.1586/14737159.5.5.755; PMID: 16149878
- Kim WS, Weickert CS, Garner B. Role of ATP-binding cassette transporters in brain lipid transport and neurological disease. J Neurochem 2008; 104:1145 - 66; http://dx.doi.org/10.1111/j.1471-4159.2007.05099.x; PMID: 17973979
- Dean M. ABC transporters, drug resistance, and cancer stem cells. J Mammary Gland Biol Neoplasia 2009; 14:3 - 9; http://dx.doi.org/10.1007/s10911-009-9109-9; PMID: 19224345
- Mitsuhashi N, Miki T, Senbongi H, Yokoi N, Yano H, Miyazaki M, et al. MTABC3, a novel mitochondrial ATP-binding cassette protein involved in iron homeostasis. J Biol Chem 2000; 275:17536 - 40; http://dx.doi.org/10.1074/jbc.275.23.17536; PMID: 10837493
- Cavadini P, Biasiotto G, Poli M, Levi S, Verardi R, Zanella I, et al. RNA silencing of the mitochondrial ABCB7 transporter in HeLa cells causes an iron-deficient phenotype with mitochondrial iron overload. Blood 2007; 109:3552 - 9; http://dx.doi.org/10.1182/blood-2006-08-041632; PMID: 17192393
- Graf SA, Haigh SE, Corson ED, Shirihai OS. Targeting, import, and dimerization of a mammalian mitochondrial ATP binding cassette (ABC) transporter, ABCB10 (ABC-me). J Biol Chem 2004; 279:42954 - 63; http://dx.doi.org/10.1074/jbc.M405040200; PMID: 15215243
- Elliott AM, Al-Hajj MA. ABCB8 mediates doxorubicin resistance in melanoma cells by protecting the mitochondrial genome. Mol Cancer Res 2009; 7:79 - 87; http://dx.doi.org/10.1158/1541-7786.MCR-08-0235; PMID: 19147539
- Herget M, Tampé R. Intracellular peptide transporters in human--compartmentalization of the “peptidome”. Pflugers Arch 2007; 453:591 - 600; http://dx.doi.org/10.1007/s00424-006-0083-4; PMID: 16710701
- Wolters JC, Abele R, Tampé R. Selective and ATP-dependent translocation of peptides by the homodimeric ATP binding cassette transporter TAP-like (ABCB9). J Biol Chem 2005; 280:23631 - 6; http://dx.doi.org/10.1074/jbc.M503231200; PMID: 15863492
- Cabarcas SM, Mathews LA, Farrar WL. The cancer stem cell niche--there goes the neighborhood?. Int J Cancer 2011; 129:2315 - 27; http://dx.doi.org/10.1002/ijc.26312; PMID: 21792897
- Wanders RJ, Visser WF, van Roermund CW, Kemp S, Waterham HR. The peroxisomal ABC transporter family. Pflugers Arch 2007; 453:719 - 34; http://dx.doi.org/10.1007/s00424-006-0142-x; PMID: 17039367
- Wu CP, Ohnuma S, Ambudkar SV. Discovering natural product modulators to overcome multidrug resistance in cancer chemotherapy. Curr Pharm Biotechnol 2011; 12:609 - 20; http://dx.doi.org/10.2174/138920111795163887; PMID: 21118092
- Hollingworth P, Harold D, Sims R, Gerrish A, Lambert JC, Carrasquillo MM, et al, Alzheimer’s Disease Neuroimaging Initiative. CHARGE consortium; EADI1 consortium. Common variants at ABCA7, MS4A6A/MS4A4E, EPHA1, CD33 and CD2AP are associated with Alzheimer’s disease. Nat Genet 2011; 43:429 - 35; http://dx.doi.org/10.1038/ng.803; PMID: 21460840
- Macé S, Cousin E, Ricard S, Génin E, Spanakis E, Lafargue-Soubigou C, et al. ABCA2 is a strong genetic risk factor for early-onset Alzheimer’s disease. Neurobiol Dis 2005; 18:119 - 25; http://dx.doi.org/10.1016/j.nbd.2004.09.011; PMID: 15649702
- Gregory RJ, Cheng SH, Rich DP, Marshall J, Paul S, Hehir K, et al. Expression and characterization of the cystic fibrosis transmembrane conductance regulator. Nature 1990; 347:382 - 6; http://dx.doi.org/10.1038/347382a0; PMID: 1699127
- Hanrahan JW, Tabcharani JA, Becq F, Mathews CJ, Augustinas O, Jensen TJ, et al. Function and dysfunction of the CFTR chloride channel. Soc Gen Physiol Ser 1995; 50:125 - 37; PMID: 7676318
- Knight HM, Pickard BS, Maclean A, Malloy MP, Soares DC, McRae AF, et al. A cytogenetic abnormality and rare coding variants identify ABCA13 as a candidate gene in schizophrenia, bipolar disorder, and depression. Am J Hum Genet 2009; 85:833 - 46; http://dx.doi.org/10.1016/j.ajhg.2009.11.003; PMID: 19944402
- Dwyer S, Williams H, Jones I, Jones L, Walters J, Craddock N, et al. Investigation of rare non-synonymous variants at ABCA13 in schizophrenia and bipolar disorder. Mol Psychiatry 2011; 16:790 - 1; http://dx.doi.org/10.1038/mp.2011.2; PMID: 21283083
- Yabuuchi H, Takayanagi S, Yoshinaga K, Taniguchi N, Aburatani H, Ishikawa T. ABCC13, an unusual truncated ABC transporter, is highly expressed in fetal human liver. Biochem Biophys Res Commun 2002; 299:410 - 7; http://dx.doi.org/10.1016/S0006-291X(02)02658-X; PMID: 12445816
- Tang L, Bergevoet SM, Gilissen C, de Witte T, Jansen JH, van der Reijden BA, et al. Hematopoietic stem cells exhibit a specific ABC transporter gene expression profile clearly distinct from other stem cells. BMC Pharmacol 2010; 10:12; http://dx.doi.org/10.1186/1471-2210-10-12; PMID: 20836839
- Kruh GD, Guo Y, Hopper-Borge E, Belinsky MG, Chen ZS. ABCC10, ABCC11, and ABCC12. Pflugers Arch 2007; 453:675 - 84; http://dx.doi.org/10.1007/s00424-006-0114-1; PMID: 16868766
- Wittenburg H, Carey MC. Biliary cholesterol secretion by the twinned sterol half-transporters ABCG5 and ABCG8. J Clin Invest 2002; 110:605 - 9; PMID: 12208859
- Hendig D, Langmann T, Kocken S, Zarbock R, Szliska C, Schmitz G, et al. Gene expression profiling of ABC transporters in dermal fibroblasts of pseudoxanthoma elasticum patients identifies new candidates involved in PXE pathogenesis. Lab Invest 2008; 88:1303 - 15; http://dx.doi.org/10.1038/labinvest.2008.96; PMID: 18936737
- Unwin RD, Whetton AD. Systematic proteome and transcriptome analysis of stem cell populations. Cell Cycle 2006; 5:1587 - 91; http://dx.doi.org/10.4161/cc.5.15.3101; PMID: 16861929
- Yi Z, Bowen BP, Hwang H, Jenkinson CP, Coletta DK, Lefort N, et al. Global relationship between the proteome and transcriptome of human skeletal muscle. J Proteome Res 2008; 7:3230 - 41; http://dx.doi.org/10.1021/pr800064s; PMID: 18613714
- Steiling K, Kadar AY, Bergerat A, Flanigon J, Sridhar S, Shah V, et al. Comparison of proteomic and transcriptomic profiles in the bronchial airway epithelium of current and never smokers. PLoS ONE 2009; 4:e5043; http://dx.doi.org/10.1371/journal.pone.0005043; PMID: 19357784
- Spradling A, Drummond-Barbosa D, Kai T. Stem cells find their niche. Nature 2001; 414:98 - 104; http://dx.doi.org/10.1038/35102160; PMID: 11689954
- Neumüller RA, Knoblich JA. Dividing cellular asymmetry: asymmetric cell division and its implications for stem cells and cancer. Genes Dev 2009; 23:2675 - 99; http://dx.doi.org/10.1101/gad.1850809; PMID: 19952104
- Giebel B, Beckmann J. Asymmetric cell divisions of human hematopoietic stem and progenitor cells meet endosomes. Cell Cycle 2007; 6:2201 - 4; http://dx.doi.org/10.4161/cc.6.18.4658; PMID: 17671435
- Del Pozo MA. Integrin signaling and lipid rafts. Cell Cycle 2004; 3:725 - 8; http://dx.doi.org/10.4161/cc.3.6.952; PMID: 15197344
- Yamamoto A, Shofuda T, Islam MO, Nakamura Y, Yamasaki M, Okano H, et al. ABCB1 is predominantly expressed in human fetal neural stem/progenitor cells at an early development stage. J Neurosci Res 2009; 87:2615 - 23; http://dx.doi.org/10.1002/jnr.22094; PMID: 19384922
- Molday RS, Zhang K. Defective lipid transport and biosynthesis in recessive and dominant Stargardt macular degeneration. Prog Lipid Res 2010; 49:476 - 92; http://dx.doi.org/10.1016/j.plipres.2010.07.002; PMID: 20633576
- Lin T, Islam O, Heese K. ABC transporters, neural stem cells and neurogenesis--a different perspective. Cell Res 2006; 16:857 - 71; http://dx.doi.org/10.1038/sj.cr.7310107; PMID: 17088897
- Peiffer I, Barbet R, Zhou YP, Li ML, Monier MN, Hatzfeld A, et al. Use of xenofree matrices and molecularly-defined media to control human embryonic stem cell pluripotency: effect of low physiological TGF-beta concentrations. Stem Cells Dev 2008; 17:519 - 33; http://dx.doi.org/10.1089/scd.2007.0279; PMID: 18513159
- Peiffer I, Barbet R, Hatzfeld A, Li ML, Hatzfeld JA. Optimization of physiological xenofree molecularly defined media and matrices to maintain human embryonic stem cell pluripotency. Methods Mol Biol 2010; 584:97 - 108; http://dx.doi.org/10.1007/978-1-60761-369-5_5; PMID: 19907973
- Thomson JA, Itskovitz-Eldor J, Shapiro SS, Waknitz MA, Swiergiel JJ, Marshall VS, et al. Embryonic stem cell lines derived from human blastocysts. Science 1998; 282:1145 - 7; http://dx.doi.org/10.1126/science.282.5391.1145; PMID: 9804556
- Hatzfeld JA, Hatzfeld A, Maigne J. Fibrinogen and its fragment D stimulate proliferation of human hemopoietic cells in vitro. Proc Natl Acad Sci USA 1982; 79:6280 - 4; http://dx.doi.org/10.1073/pnas.79.20.6280; PMID: 6959118
- Marzac C, Garrido E, Tang R, Fava F, Hirsch P, De Benedictis C, et al. ATP Binding Cassette transporters associated with chemoresistance: transcriptional profiling in extreme cohorts and their prognostic impact in a cohort of 281 acute myeloid leukemia patients. Haematologica 2011; 96:1293 - 301; http://dx.doi.org/10.3324/haematol.2010.031823; PMID: 21606172
- Garrido E. Le transcriptome ABC dans la différenciation, la détoxication et la chimiorésistance. Available at http://www.imprimerie.polytechnique.fr/Theses/Files/Garrido.pdf, 2007.
- Pfaffl MW, Tichopad A, Prgomet C, Neuvians TP. Determination of stable housekeeping genes, differentially regulated target genes and sample integrity: BestKeeper--Excel-based tool using pair-wise correlations. Biotechnol Lett 2004; 26:509 - 15; http://dx.doi.org/10.1023/B:BILE.0000019559.84305.47; PMID: 15127793
- Silver N, Best S, Jiang J, Thein SL. Selection of housekeeping genes for gene expression studies in human reticulocytes using real-time PCR. BMC Mol Biol 2006; 7:33; http://dx.doi.org/10.1186/1471-2199-7-33; PMID: 17026756
- Vandesompele J, De Preter K, Pattyn F, Poppe B, Van Roy N, De Paepe A, et al. Accurate normalization of real-time quantitative RT-PCR data by geometric averaging of multiple internal control genes. Genome Biol 2002; 3:H0034, 11; http://dx.doi.org/10.1186/gb-2002-3-7-research0034; PMID: 12184808
- Andersen CL, Jensen JL, Ørntoft TF. Normalization of real-time quantitative reverse transcription-PCR data: a model-based variance estimation approach to identify genes suited for normalization, applied to bladder and colon cancer data sets. Cancer Res 2004; 64:5245 - 50; http://dx.doi.org/10.1158/0008-5472.CAN-04-0496; PMID: 15289330
- Willems E, Leyns L, Vandesompele J. Standardization of real-time PCR gene expression data from independent biological replicates. Anal Biochem 2008; 379:127 - 9; http://dx.doi.org/10.1016/j.ab.2008.04.036; PMID: 18485881