Abstract
Besides its well-established role in sister chromatid cohesion, cohesin has recently emerged as major player in the organization of interphase chromatin. Such important function is related to its ability to entrap two DNA segments also in cis, thereby facilitating long-range DNA looping which is crucial for transcriptional regulation, organization of replication factories and V(D)J recombination. Vertebrate somatic cells have two different versions of cohesin, containing Smc1, Smc3, Rad21/Scc1 and either SA1 or SA2, but their functional specificity has been largely ignored. We recently generated a knockout mouse model for the gene encoding SA1, and found that this protein is essential to complete embryonic development. Cohesin-SA1 mediates cohesion at telomeres, which is required for their replication. Telomere defects in SA1- deficient cells provoke chromosome segregation errors resulting in aneuploidy despite robust centromere cohesion. This aneuploidy could explain why heterozygous animals have an earlier onset of tumorigenesis. In addition, the genome-wide distribution of cohesin changes dramatically in the absence of SA1, and the complex shows reduced accumulation at promoters and CTCF sites. As a consequence, gene expression is altered, leading to downregulation of biological processes related to a developmental disorder linked to cohesin function, the Cornelia de Lange Syndrome (CdLS). These results point out a prominent role of cohesin-SA1 in transcriptional regulation, with clear implications in the etiology of CdLS.
Two Different Cohesin Complexes in Somatic Vertebrate Cells
Sister chromatid cohesion is critical to ensure accurate chromosome segregation during cell division and faithful DNA repair by homologous recombination. Such a biological problem is successfully addressed by cohesin, a ring-shaped complex that entraps the DNA fiber and keeps the sister chromatids connected until they part to opposite spindle poles at the metaphase-to-anaphase transition.Citation1 In addition to this “canonical” role, recent studies have placed cohesin as an important player in other essential biological processes, such as DNA replication,Citation2 V(D)J recombinationCitation3 and transcriptional regulation (reviewed in ref. Citation4). In all these instances, cohesin could act by entrapping two DNA segments, either in trans to mediate cohesion, i.e., the sister chromatids, or in cis to stabilize chromatin loops ().
Figure 1. Cohesin composition and mechanism of action. (A) Cohesin (blue circle) can entrap DNA segments in trans to mediate sister chromatid cohesion (left) and in cis to form chromatin loops (right). (B) Subunit composition of cohesin and schematic representation of SA1 and SA2 showing their sequence homology.
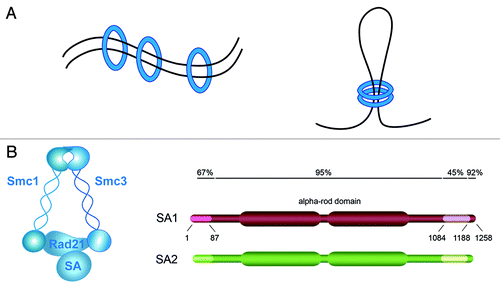
Somatic vertebrate cells have two different versions of cohesin that contain three common subunits, Smc1, Smc3 and Rad21/Scc1, and either stromal antigen 1 (SA1) or SA2, the orthologs of yeast Scc3Citation5 (). Based on the high degree of homology between the two SA proteins and their similar behavior in terms of association with chromatin during the cell cycle, it has been largely assumed that SA1 and SA2 have redundant functions. Knockdown experiments performed in HeLa cells first suggested that this was not the case by showing that cohesin-SA1 was required for telomere cohesion, while centromere cohesion was performed by cohesin-SA2.Citation6 The relative contribution of cohesin-SA1 and cohesin-SA2 to other cohesin functions has not been addressed. Importantly, a recent report described the identification of inactivating mutations in the gene encoding SA2, located in the X chromosome, in a significant number of tumors.Citation7 Loss of SA2 provoked severe cohesion defects leading to aneuploidy in human cancer cell lines. These defects could be rescued after targeted correction of SA2, while gene expression profiles of the corrected cell lines was not significantly different from their SA2-null counterparts. These results suggest that the most important function of SA2 is related to cohesion. In contrast, the characterization of SA1-knockout mice, summarized here, reveals an important contribution of SA1 not only to cohesion, but also to transcriptional regulation.Citation8,Citation9 This latter function of cohesin has become of special relevance for human disease with the discovery of heterozygous mutations in Nipbl, a factor essential for loading cohesin on chromatin, in half of the patients of Cornelia de Lange syndrome (CdLS).Citation10,Citation11 This developmental disorder affects 1:10,000–30,000 newborns and is characterized by mental retardation, reduced body size, dysmorphic face, upper limb defects and several additional organ abnormalities.Citation12 Blood cells from CdLS patients lack obvious cohesion defects, but they display altered expression patterns, featured by minor changes in hundreds of genes.Citation13 The relevance of these changes in the etiology of the disease remains unclear, and the mechanism(s) by which cohesin causes them are also unknown.
In an effort to determine the specific functions of cohesin-SA1 and cohesin‑SA2, we recently generated a knockout mouse model for SA1. Complete loss of SA1 results in embryonic lethality that starts around E11.5, although some late embryos can be obtained.Citation8 SA1 and SA2 are expressed in most adult mouse tissues, although to different extent. By means of a quantitative analysis, we have estimated that protein levels of SA2 are 3-fold higher than SA1 in MEFs.Citation8 In wild-type embryos, both SA1 and SA2 show similar distribution by immunohistochemical staining, which does not change for SA2 in the absence of SA1.Citation9 In some tissues examined, like embryonic brain, the expression of SA2 is also not affected by the lack of SA1. More importantly, the total amount of chromatin-bound cohesin is virtually the same in wild-type and SA1-null cells.Citation8 Thus, cohesin-SA1 must perform unique roles during mouse development that cannot be fulfilled by cohesin-SA2. One is related to cohesion, whereas the other affects transcription.
Cohesin-SA1, Chromosome Segregation and Cancer
SA1-null MEFs have severe proliferation defects and are aneuploid. We found that cohesin-SA1 and cohesin-SA2 mediate telomere and centromere cohesion, respectively, as proposed in human cells, whereas both contribute to cohesion along the arms (). Centromere cohesion is most critical for chromosome segregation, since it must counteract the pulling forces of microtubules during prometaphase to allow proper chromosome alignment.Citation14 What could then be the role of telomere cohesion and the reason for the aneuploidy in SA1-null cells? Telomeres pose a challenge to the replication machinery, and forks often stall in these regions.Citation15 Homologous recombination-mediated restart of stalled forks (or repair of DNA breaks generated by fork collapse) probably requires SA1-mediated cohesion so that in cells lacking SA1, telomere replication is inefficient. Incompletely replicated telomeres could be the cause of the lagging chromosomes and anaphase bridges observed in SA1-null anaphase cells, which often end in either cell death or cytokinesis failure, leading to the formation of tetraploid cells, a known pathway to aneuploidy.Citation16 Thus, the lack of cohesin-SA1 results in faulty chromosome segregation and drives aneuploidy despite robust centromere cohesion. SA1 heterozygous MEFs show also telomere replication and chromosome segregation defects, although to a lesser extent. Aneuploidy could, therefore, explain our observation that SA1 heterozygous mice show an earlier onset of spontaneous tumorigenesis when compared with their wild-type littermates and, for example, develop pancreatic cancers that are extremely infrequent in mice.Citation17 Pancreas could be more sensitive to telomere dysfunction and the genomic instability derived from it, as suggested by additional evidence.Citation18,Citation19 However, we cannot discard a contribution of dysregulated transcription to the tumorigenic effect of SA1 haploinsufficiency.
Figure 2. Division of labor between the two cohesin complexes that mediate cohesion along the chromosome. Left: Schematic representation of the distribution of cohesin complexes containing either SA1 and SA2 along the mouse chromosome. Right: Representative images of metaphase chromosomes from MEFs wild type, SA1-null, and wild type treated with SA2 siRNA stained with DAPI (top) or both with DAPI (blue) and a telomeric repeat FISH probe (red). Centromeric cohesion defects are apparent only in the absence of SA2. Aberrant telomere structures (also known as fragile telomeres) reflect defective replication and are visualized as irregularly shaped telomere signals (white arrowhead).
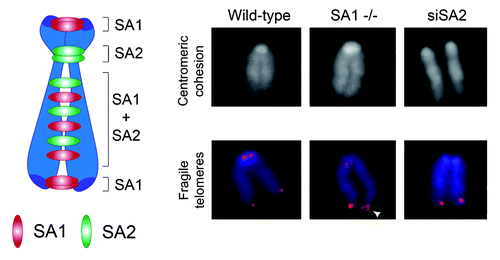
Cohesin-SA1, Gene Regulation and CdLS
ChIP sequencing analysis revealed remarkable differences in the genomic distributions of cohesin-SA1 and cohesin-SA2.Citation9 Cohesin-SA1 has greater propensity than cohesin-SA2 to localize at gene-associated regions and, in particular, to gene promoters. More striking, in the absence of SA1, cohesin is driven away from promoters and relocates to intergenic regions. The peak intensity of these new sites is clearly lower, which likely reflects a more random genomic distribution of cohesin in the different cells of the population used for the analysis.Citation20 In addition, these new positions show reduced colocalization with the chromatin insulator CTCF. In wild-type cells, 71% of the almost 24,000 sites detected with an antibody against SMC1 are also CTCF sites, confirming previous results in mouse and human cells.Citation21,Citation22 In SA1-null cells, the same antibody detects 47,000 sites, of which 46% are shared with CTCF. If we focus on the cohesin binding sites in SA1-deficient cells distinct from those bound by cohesin SA1 in wild-type cells, the overlap with CTCF drops to 19%. Thus, cohesin-SA2, the only cohesin present in SA1-null cells, lacks the ability to accumulate at promoters and CTCF sites.
The changes in cohesin distribution in SA1-null cells impact transcription, both positively and negatively. Gene ontology (GO)-defined processes related to abnormalities found in CdLS patients are downregulated in SA1-null MEFs. This is consistent with the observation of CdLS features in late SA1-null embryos, such as growth delay, skeletal and bone abnormalities and reduced body size.Citation9 A highly significant overlap was also obtained when we compared the biological processes altered in SA1-null MEFs and MEFs derived from the Nipbl heterozygous animals, a mouse model of CdLS.Citation23 In order to explore the relationship between transcriptional changes and cohesin distribution, we selected 130 genes whose expression changes in Nipbl mutant brains, with a fold change (FC) < 0.75 or > 1.25. Among these genes, 19.2% (n = 25) have SA1 binding sites at their promoters (1 kb upstream TSS) according to the ChIP data in MEFs, a percentage much higher than the one observed genome-wide (6.7%, ). Similar results were obtained when we compared the percentage of Nipbl dysregulated genes containing SMC1 at their promoters (27.7%) with the genome-wide frequency (10.3%). These data suggest that a significant proportion of the genes regulated in brains from the CdLS mouse model contain cohesin in their promoter [red (SA1) and green (SMC1) lines in the inner part of the circos diagram in ]. For some of these genes, like those encoding Protocadherins, we confirmed that cohesin-SA1 is present at their promoters also in the brain, and that reduced transcription in SA1-null embryonic brains correlates with reduced presence of cohesin.Citation9 Intriguingly, we have found high amounts of cohesin-SA1 in the promoter of Zfp608 ( and ), a zinc finger protein whose human ortholog ZNF608 has been recently proposed as a biomarker in the clinical identification and classification of CdLS cases.Citation13 Little is known about Zfp608, but a report suggests that it could act as a transcription factor regulating the expression of recombination-activating genes (RAG)1 and RAG2 expression during T-cell development.Citation24 Another transcription factor with a lot of cohesin-SA1 in its promoter is Myc (). Dowregulation of Myc in the absence of cohesin-SA1 is responsible for alterations in the transcription of its targets and likely contributes to the reduced proliferation of SA1-null cells in culture and in embryos.Citation8,Citation9
Figure 3. Transcriptional dysregulation in Nipbl +/− and SA1 −/− embryos and CdLS. (A) Percentage of genes with peaks of SA1 or SMC1 located 1kb upstream Transcription Start Site (TSS) for genes genome-wide or genes dysregulated in Nipbl +/− brains. GraphPad Prism 5 software was used to calculate two-tailed χ2 test (with Yates’ correction, ***p < 0.0001). (B) Circos diagram representing chromosomal location of Differentially Expressed Genes (FDR < 0.15) in SA1-null MEFs (black) and genes dysregulated in brains from Nipbl +/− embryos (blue). Regions shorter than 1 Mb containing two or more regulated genes are indicated by purple lines and the genes are shadowed in the same color. Binding sites at gene promoters (up to 1kb upstream the TSS) are depicted for SA1 (red) and SMC1 (green). (C) SA1 and SMC1 peaks at Zfp608 gene, a proposed biomarker of CdLS. This is one of the regions in the mouse genome with highest cohesin peak density. (D) Frequency distribution for pairs of proximal genes (< 1 Mb) in 10,000 random data sets of sample size equivalent to our experimental data set (as defined in B, n = 185). Red line highlights the frequency of pairs of proximal genes observed in the experimental data set.
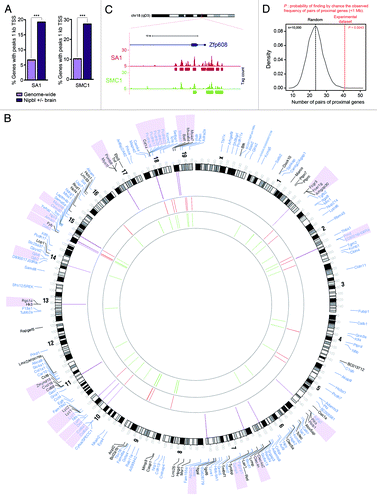
Table 1. List of gene promoters with highest density of SA1 binding sites (≥ 4 SA1 peaks in the 5 kb region upstream the TSS).
Table 2. Top five widest SA1 binding regions. All the regions correspond to gene promoters (5 kb upstream the TSS).
Importantly, presence at the promoter is not the only way in which cohesin regulates gene expression. A survey of the chromosomal location of genes whose expression is altered in either SA1-null MEFs (differentially expressed genes, FDR < 0.15, n = 55, shown in black in ) or Nipbl mutant brains (shown in blue in ) reveals that up to one fourth are located nearby (within 1 Mb region) another regulated gene (, purple shadowing and purple lines inside the circos diagram). While in random data sets, the mean of the frequency distribution is 23 pairs of proximal genes, the observed frequency in our experimental data set is 41. The probability of finding by chance such frequency of nearby genes is extremely low (p = 0.0043) (). This suggests a role for SA1 in the architectural organization of such specific loci, which likely accounts for the coordinate expression of clustered genes. This mechanism of control ensures the coordinated expression of spatially related genes and represents an efficient way to co-regulate genes involved in the same biological process.
Thus, our results indicate that cohesin-SA1 has an important role in the regulation of genes involved in CdLS pathogenesis. We speculate that a partial deficiency in Nipbl may restrict cohesin loading. While this restriction would have no major consequences for cohesion, it would significantly affect transcription of genes critical during development. Nevertheless, an involvement of cohesin functions other than gene regulation in CdLS should not be discarded yet. A better characterization of DNA replication, DNA repair and chromosome segregation must be performed in CdLS cells, both from mouse models and human patients.
Outlook
Despite the fact that cohesin was identified 15 years ago, we are still trying to understand how it works. Many aspects of its molecular mechanism of action, its cell cycle regulation or the specific functions of variant cohesin subunits and its associated factors remain a very active field of research. This knowledge will be essential to comprehend the role of somatic and germ line mutations of cohesin in human disease. In the last years, the development of chromosome conformation capture (3C)-based techniques in combination with high-throughput DNA sequencing have much contributed to analyze the organization of chromatin in its natural three-dimensional state. Such techniques are optimal tools to define the cis-regulatory networks responsible for specific transcriptional programs. It is important to highlight that transcription in mammalian cells takes place in large discrete foci, the so-called “transcription factories.” In those foci, related genes far away in the genome are organized for efficient and cooperative transcription.Citation25 We envision that the analysis of the genome-wide distribution of cohesin in different tissues and at different stages of development, together with the characterization of the spatial organization of chromatin derived from such distribution will be key to understand the basis for cell type-specific transcriptional programs. We also expect this information to provide mechanistic explanations for the transcriptional profiles found in diseases in which cohesin function is perturbed, like cancer and CdLS.
Acknowledgments
Research in our lab is supported by the Spanish Ministry of Science and Innovation through grants SAF-2010–21517 and Consolider Ingenio 2010 CSD2007–015 to A.L. and a Ramón y Cajal contract for A.C. S.R. is the recipient of a predoctoral fellowship from “La Caixa.”
References
- Nasmyth K, Haering CH. Cohesin: its roles and mechanisms. Annu Rev Genet 2009; 43:525 - 58; http://dx.doi.org/10.1146/annurev-genet-102108-134233; PMID: 19886810
- Guillou E, Ibarra A, Coulon V, Casado-Vela J, Rico D, Casal I, et al. Cohesin organizes chromatin loops at DNA replication factories. Genes Dev 2010; 24:2812 - 22; http://dx.doi.org/10.1101/gad.608210; PMID: 21159821
- Seitan VC, Krangel MS, Merkenschlager M. Cohesin, CTCF and lymphocyte antigen receptor locus rearrangement. Trends Immunol 2012; 33:153 - 9; http://dx.doi.org/10.1016/j.it.2012.02.004; PMID: 22440186
- Dorsett D. Cohesin: genomic insights into controlling gene transcription and development. Curr Opin Genet Dev 2011; 21:199 - 206; http://dx.doi.org/10.1016/j.gde.2011.01.018; PMID: 21324671
- Losada A, Yokochi T, Kobayashi R, Hirano T. Identification and characterization of SA/Scc3p subunits in the Xenopus and human cohesin complexes. J Cell Biol 2000; 150:405 - 16; http://dx.doi.org/10.1083/jcb.150.3.405; PMID: 10931856
- Canudas S, Smith S. Differential regulation of telomere and centromere cohesion by the Scc3 homologues SA1 and SA2, respectively, in human cells. J Cell Biol 2009; 187:165 - 73; http://dx.doi.org/10.1083/jcb.200903096; PMID: 19822671
- Solomon DA, Kim T, Diaz-Martinez LA, Fair J, Elkahloun AG, Harris BT, et al. Mutational inactivation of STAG2 causes aneuploidy in human cancer. Science 2011; 333:1039 - 43; http://dx.doi.org/10.1126/science.1203619; PMID: 21852505
- Remeseiro S, Cuadrado A, Carretero M, Martínez P, Drosopoulos WC, Cañamero M, et al. Cohesin-SA1 deficiency drives aneuploidy and tumorigenesis in mice due to impaired replication of telomeres. EMBO J 2012; 31:2076 - 89; http://dx.doi.org/10.1038/emboj.2012.11; PMID: 22415365
- Remeseiro S, Cuadrado A, Gómez-López G, Pisano DG, Losada A. A unique role of cohesin-SA1 in gene regulation and development. EMBO J 2012; 31:2090 - 102; http://dx.doi.org/10.1038/emboj.2012.60; PMID: 22415368
- Krantz ID, McCallum J, DeScipio C, Kaur M, Gillis LA, Yaeger D, et al. Cornelia de Lange syndrome is caused by mutations in NIPBL, the human homolog of Drosophila melanogaster Nipped-B. Nat Genet 2004; 36:631 - 5; http://dx.doi.org/10.1038/ng1364; PMID: 15146186
- Tonkin ET, Wang TJ, Lisgo S, Bamshad MJ, Strachan T. NIPBL, encoding a homolog of fungal Scc2-type sister chromatid cohesion proteins and fly Nipped-B, is mutated in Cornelia de Lange syndrome. Nat Genet 2004; 36:636 - 41; http://dx.doi.org/10.1007/s00439-004-1134-6; PMID: 15168106
- Liu J, Krantz ID. Cornelia de Lange syndrome, cohesin, and beyond. Clin Genet 2009; 76:303 - 14; http://dx.doi.org/10.1111/j.1399-0004.2009.01271.x; PMID: 19793304
- Liu J, Zhang Z, Bando M, Itoh T, Deardorff MA, Clark D, et al. Transcriptional dysregulation in NIPBL and cohesin mutant human cells. PLoS Biol 2009; 7:e1000119; http://dx.doi.org/10.1371/journal.pbio.1000119; PMID: 19468298
- Vagnarelli P, Morrison C, Dodson H, Sonoda E, Takeda S, Earnshaw WC. Analysis of Scc1-deficient cells defines a key metaphase role of vertebrate cohesin in linking sister kinetochores. EMBO Rep 2004; 5:167 - 71; http://dx.doi.org/10.1038/sj.embor.7400077; PMID: 14749720
- Sfeir A, Kosiyatrakul ST, Hockemeyer D, MacRae SL, Karlseder J, Schildkraut CL, et al. Mammalian telomeres resemble fragile sites and require TRF1 for efficient replication. Cell 2009; 138:90 - 103; http://dx.doi.org/10.1016/j.cell.2009.06.021; PMID: 19596237
- Ganem NJ, Storchova Z, Pellman D. Tetraploidy, aneuploidy and cancer. Curr Opin Genet Dev 2007; 17:157 - 62; http://dx.doi.org/10.1016/j.gde.2007.02.011; PMID: 17324569
- Gordon DJ, Resio B, Pellman D. Causes and consequences of aneuploidy in cancer. Nat Rev Genet 2012; 13:189 - 203; PMID: 22269907
- Campbell PJ, Yachida S, Mudie LJ, Stephens PJ, Pleasance ED, Stebbings LA, et al. The patterns and dynamics of genomic instability in metastatic pancreatic cancer. Nature 2010; 467:1109 - 13; http://dx.doi.org/10.1038/nature09460; PMID: 20981101
- van Heek NT, Meeker AK, Kern SE, Yeo CJ, Lillemoe KD, Cameron JL, et al. Telomere shortening is nearly universal in pancreatic intraepithelial neoplasia. Am J Pathol 2002; 161:1541 - 7; http://dx.doi.org/10.1016/S0002-9440(10)64432-X; PMID: 12414502
- Carretero M, Remeseiro S, Losada A. Cohesin ties up the genome. Curr Opin Cell Biol 2010; 22:781 - 7; http://dx.doi.org/10.1016/j.ceb.2010.07.004; PMID: 20675112
- Parelho V, Hadjur S, Spivakov M, Leleu M, Sauer S, Gregson HC, et al. Cohesins functionally associate with CTCF on mammalian chromosome arms. Cell 2008; 132:422 - 33; http://dx.doi.org/10.1016/j.cell.2008.01.011; PMID: 18237772
- Wendt KS, Yoshida K, Itoh T, Bando M, Koch B, Schirghuber E, et al. Cohesin mediates transcriptional insulation by CCCTC-binding factor. Nature 2008; 451:796 - 801; http://dx.doi.org/10.1038/nature06634; PMID: 18235444
- Kawauchi S, Calof AL, Santos R, Lopez-Burks ME, Young CM, Hoang MP, et al. Multiple organ system defects and transcriptional dysregulation in the Nipbl(+/-) mouse, a model of Cornelia de Lange Syndrome. PLoS Genet 2009; 5:e1000650; http://dx.doi.org/10.1371/journal.pgen.1000650; PMID: 19763162
- Zhang F, Thomas LR, Oltz EM, Aune TM. Control of thymocyte development and recombination-activating gene expression by the zinc finger protein Zfp608. Nat Immunol 2006; 7:1309 - 16; http://dx.doi.org/10.1038/ni1397; PMID: 17057722
- Li G, Ruan X, Auerbach RK, Sandhu KS, Zheng M, Wang P, et al. Extensive promoter-centered chromatin interactions provide a topological basis for transcription regulation. Cell 2012; 148:84 - 98; http://dx.doi.org/10.1016/j.cell.2011.12.014; PMID: 22265404