Abstract
In yeast cells, the vacuole divides and fuses in each round of cell cycle. While mutants defective in vacuole fusion are “wild type” for vegetative growth, most have shortened replicative lifespans under caloric restriction (CR) condition, a manipulation that extends lifespan in wild type cells. To explore whether vacuole fusion extends lifespan, we screened for genes that can complement the fusion defect of selected mutants (erg6Δ, a sterol mutant; nyv1Δ, a mutant involved in the vacuolar SNARE complex and vac8Δ, a vacuolar membrane protein mutant). This screen revealed that Osh6, a member of the oxysterol-binding protein family, can complement the vacuole fusion defect of nyv1Δ, but not erg6Δ or vac8Δ, suggesting that Osh6’s function in vacuole fusion is partly dependent on membrane ergosterol and Vac8. To measure the effect of OSH6 on lifespan, we replaced the endogenous promoter of OSH6 with a shorter version of the ERG6 promoter to obtain PERG6-OSH6. This mutant construct significantly extended the replicative lifespan in a wild type background and in a nyv1Δ mutant. Interestingly, PERG6-OSH6 cells were more sensitive to drugs that inhibit the activity of the TOR complex 1 (TORC1) than wild type cells. Moreover, a PERG6-OSH6 tor1Δ double mutant demonstrated a greatly shortened lifespan, suggesting a genetic interaction between Osh6 and Tor1. Since active TORC1 stimulates vacuole scission and CR downregulates TORC1, Osh6 may link these two pathways by adjusting vacuolar membrane organization to extend lifespan.
Keywords: :
Introduction
In yeast cells, vacuoles fuse and divide in response to environmental or intracellular cues. These fusion and scission processes quickly change the volume-to-surface ratio of vacuoles without de novo membrane biogenesis. Despite these seemingly beneficial effects, the fusion/scission process seems dispensable for the cell’s viability, at least under laboratory culture conditions; mutants blocked in vacuole fusion or scission usually are not distinguishable from wild type strains in growth or other obvious phenotypes. However, these processes change the cell’s survival capacity. Mutants with fragmented vacuoles (nyv1Δ, vac8Δ and erg6Δ) or round vacuoles (vac14Δ) have shorter replicative lifespans either when grown in normal or calorie-restricted (CR) medium, a longevity manipulation.Citation1,Citation2 The dispensability of vacuole fusion/scission for log phase cells and the involvement of vacuoles in longevity provide a unique opportunity to explore aging mechanisms, since a decrease in general fitness due to mutations in various pathways poses a challenge for dissecting longevity mechanisms with genetic approaches.
Vacuole fusion and scission processes are active research topics, as many proteins (Sec18, Sec17, Nyv1 and Vac8) and lipids (ergosterol, phosphoinositides and phosphatidic acid) are required for vacuole fusion.Citation3-Citation5 Vacuole scission studies focus mainly on the equilibrium between phosphatidylinositol-3-phosphate (PI3P) and PI(3,5)P2.Citation6-Citation8 A recent study demonstrated that the target of rapamycin complex 1 (TORC1) actively catalyzes vacuole scission.Citation5 To study the contribution of vacuole fusion/scission to longevity, we measured the replicative lifespan of selected vacuole mutants and discovered that many mutants with fragmented vacuoles (nyv1Δ, erg6Δ and ypt7Δ) have shorter lifespans on caloric restricted media than on normal media.Citation2 With multiple systems biology approaches, we predicted that nine vacuole-related genes are required for CR-mediated lifespan extension. Indeed, eight out of nine are required for longevity, and five are required for vacuole fusion under CR (Wuttke et al. manuscript submitted). While these studies show that vacuole fusion is necessary for longevity, it does not prove whether vacuole fusion is sufficient for lifespan extension in wild type cells, since many mutations resulting in fragmented vacuoles are caused by defect in other pathways.
Sterols are critical for vacuole fusion. The sterol content of the vacuolar membrane is only about 1/10 that of the plasma membrane.Citation9 In yeast cells, sterols are synthesized in the endoplasmic reticulum under aerobic conditions. Unlike the plasma membrane, the vacuolar membrane does not have lipid rafts with ordered ergosterol-sphingolipids interactions.Citation10 This raises questions as to how cells retain the sterol content of the vacuolar membrane and how cells transport sterols to the vacuolar membrane for vacuole fusion. Clues for answering these questions came from studies of a family of oxysterol binding proteins.
The yeast genome has seven oxysterol binding protein sequence homologs (OSH). While deletion of any single OSH gene does not affect the vacuolar morphology, deletion of all seven OSH genes results in highly fragmented vacuoles,Citation11 suggesting the involvement of Osh proteins in vacuole fusion/scission or some membrane trafficking pathways involving vacuoles. Deletion of OSH5 or OSH6 upregulated the mRNA levels of ERG8 or ERG9,Citation12 and overexpression of OSH6 downregulated the total cellular sterol levels.Citation13 In addition to modulating sterol synthesis, data indicate that Osh proteins can mediate non-vesicular sterol transport.Citation14 Osh proteins are proposed to locate their target membranes by binding membrane-specific phosphoinositides.Citation15 Osh6 has the potential to transport sterols to the vacuolar membrane, since it binds both PI3P and PI(3,5)P2, vacuole-specific lipids.Citation13 A recent study challenged the sterol-transporting function of Osh proteins and suggested instead that the role of Osh proteins is to reorganize plasma membrane sterols.Citation16 However, either transporting sterols to or stabilizing sterols in the vacuolar membrane support a role for Osh proteins in vacuole fusion.
In this study, we screened OSH genes for their involvement in vacuole fusion/scission and discovered that OSH6 when overexpressed can complement fragmented vacuoles in selected mutants. Consistent with the notion that vacuole fusion is sufficient to extend lifespan, a chromosomal upregulated mutant of OSH6 (PERG6‑OSH6) extended lifespan in a wild type background and in a nyv1Δ mutant. The genetic interaction between PERG6‑OSH6 and tor1Δ further revealed a potential mechanism for upregulation of OSH6 in vacuole fusion and lifespan extension.
Results
High-copy OSH6 expression complements the fragmented vacuole of nyv1Δ.
Since most mutants with fragmented vacuoles do not benefit from caloric restriction and, therefore, lifespan extension,Citation2 we reasoned that complementing the vacuole fusion defect may be a means to extend lifespan. As a first step, we overexpressed different OSH genes in mutants with fragmented vacuoles—vac8Δ, erg6Δ and nyv1Δ. Overexpression of OSH6 significantly changed the vacuolar morphology of nyv1Δ in synthetic media () but had no effect on that of vac8Δ or erg6Δ (not shown). In a nyv1Δ strain containing the vector YEp24, more than 70% of cells showed highly fragmented vacuoles (> 6 vacuolar vesicles/cell) in synthetic medium. Overexpression of OSH5 exacerbated this fragmentation. However, overexpression of OSH6 significantly decreased the fraction of cells with highly fragmented vacuoles (). The observation that OSH6 can suppress fragmented vacuoles in nyv1Δ but not erg6Δ is consistent with the notion that Osh6 promotes vacuole fusion by regulating the distribution of sterols in membranes. Thus, we focused on OSH6 in the following experiments.
Figure 1. The effect of high copy OSH6 expression in nyv1Δ on vacuolar morphology. (A) Comparison of the vacuole morphology of nyv1Δ cells with an empty vector (YEp24) and high copy OSH plasmids. OSH5ox is YEp24-OSH5 and OSH6ox is YEp24-OSH6. (B) Quantitative analyses of the vacuolar morphology of nyv1Δ cells with various plasmids. Cells were divided into three categories based on the number of vacuoles per cell. The number of cells (1 vacuole/cell, 2–5 vacuoles/cell, > 6 vacuoles/cell) were: YEp24: 3, 17, 50; OSH5ox: 0, 6, 51; OSH6ox: 37, 74, 47. Proportion tests results for the comparison of OSH6ox and YEp24 are: the fractions of cells with round vacuoles (1 vacuole/cell) and normal vacuoles (2–5 vacuoles/cell) of OSH6ox are significantly higher than those in YEp24 (p = 0.0002 for round cells and p = 0.0007 for normal cells); the fraction of highly fragmented vacuoles (> 6 vacuoles/cell) in OSH6ox (47/158) is significantly lower than the same category in YEp24 (50/70) (p < 0.0001).
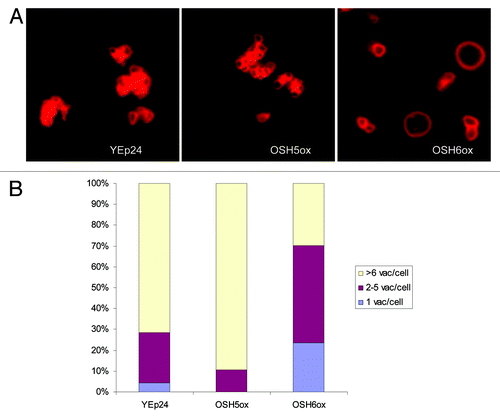
Upregulation of OSH6 extends replicative lifespan.
To generate a chromosomal version of the OSH6 upregulated mutant for lifespan analyses, we replaced the endogenous promoter of OSH6 with a shorter version of the ERG6 promoter to obtain the PERG6‑OSH6 strain (). We chose the promoter of ERG6, since the abundance of Erg6 is about 20-fold higher than that of Osh6.Citation17 To assess the effect of this genetic manipulation, we measured OSH6 mRNA abundance by real-time PCR (). However, this PERG6-OSH6 manipulation did not change the OSH6 mRNA by a factor of 2. The PERG6 promoter only increased the OSH6 mRNA level from 10 to 70% in early log phase cells (the left three bars in ). Since Osh6 interacts with Erg11,Citation18 we also compared the level of ERG11 mRNA, and found that PERG6-OSH6 consistently downregulated expression of ERG11 by an average factor of 2, suggesting that the PERG6 promoter may change the regulatory pattern of the expression of OSH6. To confirm the effect of the PERG6 manipulation directly, we replaced the OSH5 promoter with PERG6 to obtain PERG6-OSH5. The PERG6-OSH5 fusion increased the abundance of OSH5 mRNA by approximately about 100-fold. This high level indicates that replacement of the endogenous promoter with PERG6 can alter the expression pattern of the targeted gene.
Figure 2. The effect of PERG6-OSH6 on OSH6 gene expression and lifespan. (A) Stepwise illustration of the OSH6 promoter replacement by the ERG6 promoter. (a) The promoter region of ERG6 on chromosome 13. SRE, sterol regulatory element, binding sites of the activators Upc2p and Ecm22p. Vertical lines: consensus binding sites of the Mot3 repressor. (b) The ERG6 basal promoter in plasmid pRS316-ERG6. (c.) Pairing of the PCR-amplified URA3- PERG6 cassette with the promoter region of OSH6 on chromosome 11. (d) The resulting promoter region of OSH6. (B) Real-time PCR analyses of mRNA levels of indicated genes. The log2 (Y axis) value indicates the fold of change in the exponent of base 2 in comparison to the corresponding gene in the wild type. Results from three batches of log phase cells are shown. (C) The promoter of ERG6 increased the level of Osh6. Different amount of crude cell extracts of OSH6-TAP and PERG6-OSH6-TAP strains were separated by a 10% SDS-PAGE and then subject to Western analysis. (D) The survival curve of PERG6-OSH6 and PERG6-OSH5 in YEPD media. Mean lifespan, median lifespan, and sample sizes (N) are indicated in parentheses. ANOVA test showed that these three samples are all significantly different, i.e., the lifespan of wild type is significantly longer than that of PERG6-OSH5 but significantly shorter than that of PERG6-OSH6 (p < 0.0001).
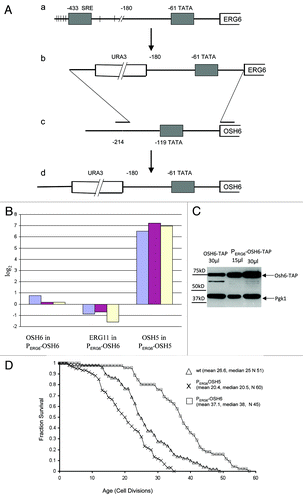
To test whether the PERG6-OSH6 fusion alters Osh6 protein levels, we constructed PERG6-OSH6 in a tandem affinity purification (TAP)-tagged OSH6-TAP strain and measured protein levels with anti-TAP antibody (). This analysis showed that PERG6-OSH6 upregulated Osh6 protein levels by about 2-fold. Since the only difference between OSH6-TAP and PERG6-OSH6-TAP strains () is the promoter region of OSH6, the observation that PERG6 did not upregulate mRNA levels by 2-fold () but increased protein levels by 2-fold () indicates that the 5' untranslated region from PERG6 may change the post-transcriptional processing pattern of OSH6 mRNA. Thus, changes in lifespan due to PERG6-OSH6 fusion () is an effect of the altered expression pattern of OSH6.
In YEPD medium, the replicative lifespan of PERG6-OSH6 (mean 37.1, median 38) was significantly longer than the lifespan of the wild type control (mean 26.6, median 25) (p < 0.0001) (). In contrast, overexpression of OSH5 (PERG6-OSH5 mean 20, median 20.5) significantly shortened replicative lifespan. We also compared the lifespan of OSH6‑TAP and PERG6‑OSH6-TAP strains, but found that both had similar lifespans (mean 22). The TAP-tag may compromise the function of Osh6 protein and mask the effect of upregulation. Another possible explanation may be differences in strain background between the TAP strain (BY 4741) and the PERG6-OSH6 strain (BY4742) used for lifespan assays. The different response of BY4742 and BY4741 to the same mutation in lifespan has been previously observed in reference Citation1. In BY4742, the observation that OSH6, but not its homolog OSH5, extended lifespan strongly suggests that Osh6 has a unique function related to lifespan extension.
The coiled-coil domain of Osh6 is dispensable for longevity.
Osh6 contains an oxysterol-binding protein related domain (ORD; amino acid 1–300) and a coiled-coil domain (CC; amino acid 301–448) ().Citation13 Coiled-coil domains are protein-protein and protein-lipid binding domains. The CC domain of Osh6 interacts with Vps4,Citation19 a protein located on late endosomes that assists in late endosome-vacuole fusion. In an attempt to locate the domain responsible for the longevity effect, we constructed a CC-deletion mutant (PERG6-OSH6Δ301–448), a C-half deletion mutant (PERG6-OSH6Δ255–448) and an ORD-CC deletion mutant (PERG6-OSH6Δ32–448) and analyzed their phenotypes. As expected, deletion of both ORD and CC (PERG6‑OSH6Δ32–448) abrogated the longevity effect. Intriguingly, deletion of the CC domain did not affect longevity, while deletion of the C-half significantly shortened the lifespan of PERG6-OSH6 (). The dispensability of the CC domain for longevity contrasts with the essential function mediated by Osh6, where both the ORD and the CC are required for the common function shared by all Osh proteins.Citation13 While both full-length Osh6 and Osh6ΔCC extended the lifespan in a wild type background when upregulated, we did not observe an obvious difference in vacuolar morphology. Thus, we constructed PERG6‑OSH6 nyv1Δ double mutants.
Figure 3. Dissection of the Osh6’s functional domains in lifespan extension. (A) An illustration of Osh6 representing the coordinates of the oxysterol-binding protein related domain (ORD) and coiled coil domain (CC). (B) Survival curves of different Osh6 mutants in PERG6-OSH6 background. Mean lifespan, median lifespan and sample size (N) of each mutant are indicated. T-test and Wilcoxon rank-sum indicated that there was no difference between PERG6-OSH6 and PERG6-OSH6Δ301–448. The lifespan of these two are significantly longer than the lifespan of PERG6-OSH6Δ255–448, which is significantly longer than that of PERG6-OSH6Δ32–448 (p < 0.0001). (D) Vacuolar morphology of nyv1Δ and PERG6-OSH6 nyv1Δ mutants in YEPD media. (D) Quantitation of cells in . The number of cells for the three categories (1 vacuole/cell, 2–5 vacuoles/cell, > 6 vacuoles/cell) were nyv1Δ: 9, 89, 41; PERG6-OSH6 nyv1Δ: 16, 91, 7; PERG6-OSH6ΔCC nyv1Δ: 81, 52, 1. E. The replicative lifespan of nyv1Δ and PERG6-OSH6 nyv1Δ mutant on YEPD.
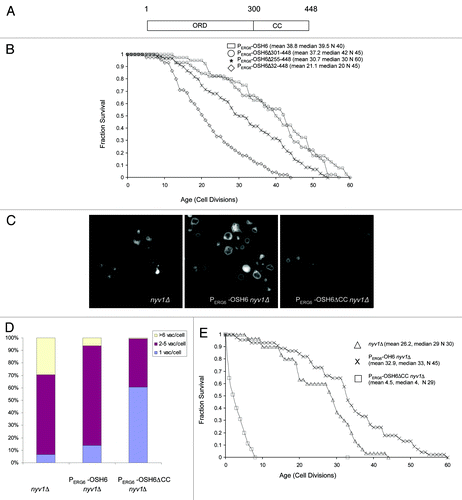
Promoting vacuole fusion by Osh6 extends lifespan.
To further test the relationship between vacuolar morphology and lifespan extension, we constructed the PERG6-OSH6 nyv1Δ double mutant. Like high-copy OSH6 (), PERG6-OSH6 promoted vacuole fusion in nyv1Δ (). In rich YEPD medium, 30% of nyv1Δ cells show highly fragmented vacuoles (). This differs from the 70% highly fragmented vacuoles observed for nyv1Δ cells in synthetic medium (), a difference due to limiting amino acids such as leucine in synthetic media.Citation20 The fraction of cells with fragmented vacuoles (> 6 vacuoles/cell) decreased from 30% in nyv1Δ strain to 6% in the PERG6-OSH6 nyv1Δ strain (p < 0.0001). Interestingly, the PERG6-OSH6ΔCC nyv1Δ double mutant had a significantly higher ratio of cells with single round vacuoles per cell than the PERG6-OSH6 nyv1Δ double mutant (p < 0.0001) or nyv1Δ mutant alone (p < 0.0001) (). Differences in vacuolar morphology also were accompanied by changes in lifespan. Similar to its effect in the wild type background ( and ), PERG6-OSH6 extended the lifespan of nyv1Δ (). The mean lifespan of PERG6-OSH6 nyv1Δ (32.9) was significantly longer than the lifespan of nyv1Δ (26.2) (p = 0.0088 by Wilcoxon text and p = 0.0098 by t-test). However, the lifespan of the PERG6-OSH6ΔCC nyv1Δ double mutant (MLS 4.5) was significantly shorter than the lifespan of nyv1Δ (p = 0.0001 by either Wilcoxon or t-test). The round vacuoles and the shortened lifespan of PERG6-OSH6ΔCC nyv1Δ suggest that vacuole fusion, to a limited extent, can extend lifespan.
PERG6-OSH6 mimics sterol-depletion from plasma membrane.
A previous report indicated that an approximate 10-fold upregulation of Osh6 decreases total cellular sterol levels.Citation13 Our analyses also showed that PERG6-OSH6 downregulated ERG11 expression (), and that the sterol-binding domain of Osh6 was critical for longevity (). We thus asked whether PERG6-OSH6 has an effect on sterol transport and metabolism. We initially tried to estimate the total plasma membrane sterol and vacuolar membrane sterols by filipin staining, but no obvious differences between wild type and the PERG6-OSH6 mutant were observed; both had concentrated filipin-staining signals on plasma membranes and some intracellular structures (most likely lipid droplets) (). These concentrated filipin staining signals made it difficult to visualize the shape of vacuoles and thus assess filipin-sterol interactions on vacuolar membranes. We subsequently measured liquid phase endocytosis by Lucifer yellow uptake, a phenotype depending on plasma membrane sterol.Citation21 The PERG6-OSH6 mutant showed a minor delay in transferring the fluorescent dye to the vacuole. In wild type cells, Lucifer yellow began to arrive at vacuoles at 15 min, which is evidenced by the bright fluorescent signal and the clear boundary of vacuoles (see the arrow in the left part of ). In PERG6-OSH6 cells, this bright fluorescent vacuole began to appear at 30 min (see the arrow in the right part of ). Quantitation corroborated a delay in uptake of Lucifer yellow by PERG6-OSH6 ().
Figure 4. Comparison of sterol-related phenotypes between wild type and PERG6-OSH6. (A) Comparison of filipin staining of wild type and PERG6-OSH6 cells. Arrows point to intracellular filipin staining structures (most likely lipid droplets). Wedges point to enriched filipin staining signal on plasma membranes. (B) Comparison of Lucifer yellow (LY) uptake by wild type and PERG6-OSH6. The uptake was stopped at indicated time points. Arrows indicate examples of fluorescent vacuoles with clear boundaries, an indication of the arrival of Lucifer yellow to the vacuole. (C) Quantitation of the cells (in panel B). The number of cells with discernible fluorescent vacuoles relative to total cells from 15 min to 45 min were 140/617, 51/95, 141/242 for wild type and 3/245, 31/59, 92/115 for PERG6-OSH6. Proportion tests showed that the fraction of LY-labeled vacuoles at 15 min for PERG6-OSH6 was significantly lower than that of the corresponding wild type (p < 0.0001). There is no difference for 30 min and 45 min between wild type and PERG6-OSH6. (D) PERG6OSH6 cells gave a similar effect as MβCD treatment on actin cables. MβCD was added to early log phase wild type cells at a final concentration of 2.5mg/ml. Cells were further incubate for 5 h before staining for actin. (E) Cells were grouped into five categories indicating F-actin structures: polarized actin (polarized), actin patches in the mother-bud neck (M-Bud neck), cells with more than three actin patches (> 3 patches), cells with actin cables stretching from the bud to the mother cell (cables), and cells without discernible actin signals (diffused). The number of cells in each category was counted and the proportions to the total number of cells were calculated (displayed as percentages on Y-axis). The sample sizes are 121, 457 and 156 for wild type, wild type + MβCD and PERG6-OSH6 respectively. Proportion test showed that the ratio of cells with more than three patches/cell in both wild type treated with MβCD (165/457) and PERG6-OSH6 (73/156) were significantly higher than that in wild type (21/121) (p < 0.0001)
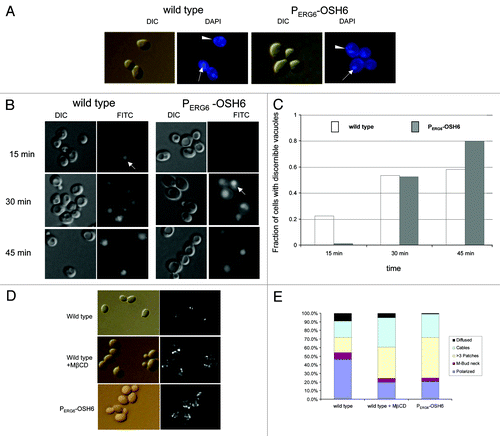
To further assess the effect of PERG6-OSH6 on plasma membrane sterol levels, we compared wild type cells treated with the sterol-depleting drug methyl-β-cyclodextrin (MβCD) and PERG6-OSH6 by actin staining (). Downregulation of ergosterol synthesis increases actin aggregation.Citation22 Treating wild type cells with 2.5 mM MβCD decreased the ratio of cells with polarized actin patches and increased the ratio of cells with more dispersed actin patches (> 3 patches/cell) on the plasma membrane (). Like MβCD treatment, PERG6-OSH6 also dispersed the actin patches; the ratio of cells with more than three actin patches per cell in PERG6-OSH6 or wild type, plus MβCD was significantly higher than the same category in wild type cells (p < 0.0001) ().
PERG6-OSH6 delays age-dependent changes in vacuolar morphology.
Our inability to identify an obvious difference between log phase wild type and PERG6-OSH6 cells may be due to a later requirement for Osh6 during cellular aging. To test this hypothesis, we compared the mRNA levels of OSH5 and OSH6 in young and mid-aged (generations 6–7) wild type and PERG6-OSH6 cells (). While there was no more than 2-fold change in mRNA levels of OSH6 either in wild type or in the PERG6-OSH6 mutant, the OSH5 mRNA level was upregulated in wild type by about 3–15-fold. This upregulation proves that our sorted cells are different from log phase cells, although presently we don’t know the mechanism of this upregulation of OSH5. Interestingly, the increase of OSH5 mRNA was blocked in PERG6-OSH6 mutant.
Figure 5. PERG6-OSH6 delays age-dependent alterations. (A) Real time PCR analyses of the indicated gene in mid-aged (generations 6–7) cells. The Y axis, log2, shows the change of mRNA levels in mid-aged cells in comparison to log phase cells in the exponent of base 2. (B) Comparison of Osh6 protein levels in log phase (young) and generations 6–7 cells (mid-aged). Varying amounts of crude cell extracts from young cells and mid-aged cells were separated by SDS-PAGE and subjected to Western analyses with anti-TAP and anti-Pgk1 antibodies. (C) Comparison of Osh6 protein levels in young and mid-aged PERG6-OSH6-TAP strain. M: molecular weight marker. (D) Comparison of Erg6 protein level in young and mid-aged cells. (E) Vacuoles of mid-aged cells. Mid-aged (generations 6–7) wild type cells (top panel) or PERG6-OSH6 cells (bottom panel) with a chromosomal version of VAC8-GFP were isolated and labeled with FM4–64. The green fluorescence (right panels) shows the localization of Vac8-GFP. Arrows indicate enrichment of Vac8 on the vacuolar membrane.
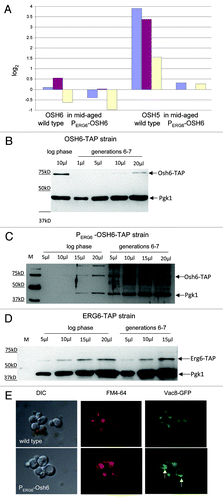
To further explore the effect of Osh6 on age-dependent changes, we compared the level of Osh6-TAP protein expressed under its endogenous promoter in young (log phase) cells and mid-aged (generations 6–7) cells () and found that total cellular Osh6 levels decreased in aged cells; the level of the Osh6-TAP signal in mid-aged cells was less than half of the Osh6-TAP signal in young cells. When OSH6-TAP was driven by the shorter promoter of ERG6 (PERG6-OSH6-TAP), the age-dependent decline of Osh6 was slowed (). For , we did a densitometry analysis of the Osh6-TAP signal and the Pgk1 signal and did not observe greater than a 2-fold difference between log phase and mid-aged PERG6-OSH6-TAP cells (data not shown). In contrast to Osh6-TAP, the Erg6-TAP signal showed no decrease at the age tested (generations 6–7) (). The lack of an age-dependent alteration of OSH6 mRNA () and the age-dependent decline of Osh6-TAP protein () suggests age-dependent post-transcriptional processing. This processing may be altered by the 5' untranslated region of the PERG6-promoter (). The decline of Osh6 protein levels with age is similar to the age-dependent decline of an established longevity protein Sir2.Citation23 Both decline at an age of about one-third of their lifespan expectancies.
Since Osh6 protein declines () and the vacuolar membrane deteriorates as judged by Vac8 localizations at or before generations 6–7,Citation2 we tested whether PERG6-OSH6 remedies the age-dependent alteration of vacuoles (). We constructed a PERG6-OSH6 mutant containing a VAC8-GFP reporter (in a LWY 7235 backgroundCitation2) and isolated mid-aged cells (generations 6–7) to analyze the enrichment of Vac8 on vacuolar membranes (). For FM4–64 labeling, mid-aged wild type cells did not show clear vacuolar vesicles as log phase cells ( and ). However, at the same age, PERG6-OSH6 cells had clear vacuolar vesicles (middle parts of ). In log phase cells, there is clear Vac8 enrichment on the vacuolar membrane ( in Tang et al.Citation24). The Vac8 enrichment in mid-aged wild type cells did not appear to be as frequent as observed for mid-aged PERG6-OSH6 cells (). Since Vac8 is required for vacuole fusion,Citation24,Citation25 and high-copy OSH6 expression cannot bypass Vac8 for vacuole fusion (data not shown), the enrichment of Vac8 suggests that PERG6-OSH6 delays the age-dependent alteration of vacuolar membranes, in part through the function of Vac8. The enrichment of Vac8 also suggests the possibility that Osh6 blocks vacuole scission. Vac8 interacts with a component of TORC1, Tco89.Citation24 TORC1 is a master regulator of cell growth and controls vacuole scission.Citation5 We thus analyzed whether there are any mechanistic links between OSH6 upregulation and TORC1 activity.
PERG6-OSH6 is sensitive to perturbations of TORC1.
Three different assays were employed to explore the relationship between Osh6 and TORC1. First, we stained the actin cables after treating cells with rapamycin, an inhibitor of TORC1. As reported in reference Citation26, rapamycin treatment disrupts actin fibers but maintains actin patches in wild type cells (the FITC image of row 2 in ). However, rapamycin treatment of PERG6-OSH6 cells disrupted both actin cables and patches; most cells did not show FITC-phalloidin staining (the FITC image of the bottom row of ). We subsequently tested whether caffeine, an inhibitor of Tor1, affects growth of PERG6-OSH6 cells. Deletion of OSH6 made cells more resistant to 0.2% caffeine, but PERG6-OSH6 made cells slightly more sensitive to caffeine than wild type cells (), suggesting that the activity of TORC1 may be in a low activity state in PERG6-OSH6 cells.
Figure 6. PERG6-OSH6 is sensitive to perturbations of TORC1 signaling. (A) Responses of actin cytoskeleton to rapamycin treatment in wild type and PERG6-OSH6 cells. For rapamycin treatment, early log phase cells were treated with 0.2 μg/ml rapamycin for 30min before staining for actin. (B) Growth of wild type and osh6 mutants on 0.2% caffeine plate. Cells were spotted on YEPD or 0.2% caffeine plate (104, 103, 102, 10 cells/spot from left to right) and incubated at 30°C for 2 d. (C) Replicative lifespan of PERG6- mutants in tor1Δ. The mean lifespan (MLS), median lifespan and sample sizes are indicated. The lifespan of PERG6-OSH6 tor1Δ is significantly shorter than the lifespan of PERG6-OSH5 tor1Δ (p = 0.0001 by either Wilcoxon or t-test). (D) A model for the relationship between Osh6 and vacuole fusion. Overexpression of Osh6 either transports sterols to the vacuolar membrane or retains sterols on the vacuolar membrane (left branch). Moreover, upregulated Osh6 enriches Vac8 and downregulates TORC1 and slows down vacuole scission (right branch). Although other processes and signals for fusion and scission are not changed, the effects of PERG6-OSH6 on sterol and TORC1 result in a pro-fusion equilibrium and extend lifespan.
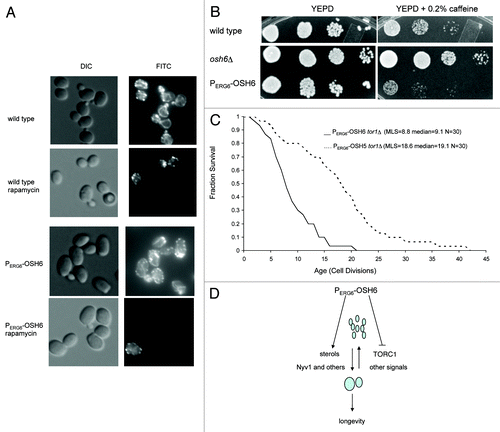
Finally, to test whether the sensitivity of PERG6-OSH6 to perturbations of TORC1 (rapamycin, caffeine) is related to aging, we constructed a PERG6-OSH6 tor1Δ double mutant (), since tor1Δ extends the lifespan in the BY4742 background.Citation1 Interestingly, this double mutant had a much shorter lifespan (MLS 8.8) than PERG6-OSH6 (MLS 37.1, ). A double mutant of PERG6-OSH5 tor1Δ had a lifespan (MLS 18.6) close to the lifespan of PERG6-OSH5 (MLS 20.4, ). The synthetic sick effect between tor1Δ and PERG6-OSH6 with respect to longevity suggests that Osh6 and Tor1 may target the same pathway to extend lifespan.
Discussion
Osh proteins are involved in multiple cellular processes, including polarized growth.Citation27 These functions are probably achieved by two categories of inter-related enzymatic activities of Osh proteins: sterol transporters or membrane sterol organizersCitation14,Citation16 and activators of phosphoinositide metabolism.Citation4 Previous studies with regard to Osh6 and results obtained from this study suggest that sterols are major targets responsible for the effect of Osh6 on vacuolar fusion and longevity.
Downregulation of ergosterol does not lead to vacuole fusion.
Downregulation of total cellular sterol by OSH6 overexpressionCitation13 mimics the effect of caloric restriction (CR), a longevity manipulation. CR downregulates the synthesis of cholesterol in mammals and ergosterol in yeast.Citation28 Vacuole fusion depends on end product ergosterol in vacuolar membranes. Depletion of sterols from isolated vacuoles prevents vacuole fusion in vitro.Citation29 Thus, downregulation of total cellular sterol levels is expected to push the vacuole fusion/scission equilibrium toward the direction of scission. Indeed, CR induces vacuole fragmentation in pfa3Δ, a mutant defective in palmitoylating the vacuolar membrane protein Vac8.Citation30 Palmitoylation of Vac8 is required for vacuole fusion.Citation25
However, we observed that high-copy OSH6 expression promoted vacuole fusion in nyv1Δ (), and PERG6-OSH6, which downregulates ERG11 mRNA levels (), also promotes vacuole fusion in nyv1Δ (). A plausible resolution of this apparent contradiction of downregulation of sterol and promotion of vacuole fusion is that Osh6 transports sterols to the vacuolar membrane or retains sterols on the vacuolar membrane. We attempted to compare the sterol content of isolated vacuoles by filipin labeling with a fluorometer and detected an approximate 20% increase in the filipin staining signal in PERG6-OSH6 vacuoles, but results were often inconsistent likely due to either technical difficulties or an intrinsic feature of sterols in the vacuolar membrane (data not shown).
Nyv1 is involved in multiple steps of vacuole fusion. One function of Nyv1 is to ensure the dedication of the V0 subunits (integral membrane proteins) of vacuolar H+-ATPase to vacuole fusion; deletion of NYV1 increases the association of V1 subunits (peripheral membrane subunits of ATPase, e.g., Vma1) with V0 subunits (Vma3) by 2-fold; an overexpression of NYV1 using the ADH1 promoter decreases the Vma1-Vma3 association by about 2-fold.Citation31 Despite this effect, the vacuolar pH of nyv1Δ in YEPD medium is in the range of wild type cells,Citation32 suggesting a normal function of the vacuolar ATPase. The vacuolar H+-ATPase activity also depends on wild type ergosterol levels in the vacuolar membrane. Treating isolated vacuoles with 8 mM MβCD (10 mg/ml) decreases the ATPase activity by more than 75%.Citation33 To extract sterols from plasma membrane, 40 mM MβCD is usually used.Citation16 The sensitivity of vacuolar membrane sterols to low doses of MβCD suggests that vacuolar membrane sterol is more unstable than plasma membrane sterols. Interestingly, extracting vacuolar sterols does not disrupt the association of V1-V0 subunits.Citation33 Thus, one function of sterols in vacuolar membrane is to maintain V1 and V0 subunits in a state where reversible association-dissociation can easily occur. Since neither nyv1Δ nor sterol depletion can totally abolish the vacuolar ATPase function, it is likely that Nyv1 and sterols compensate for the function of the other. This is likely the cause of the large round vacuoles and the shortened lifespan of PERG6-OSH6ΔCC nyv1Δ (), if we assume that Osh6 modulates sterol homeostasis in vacuolar membranes.
Vacuolar sterol is a major target of Osh6.
In addition to ergosterol, phosphoinositides are also ligands of Osh proteins.Citation13,Citation15 Like ergosterol metabolism, phosphoinositide metabolism [both PI(3,5)P2/PI3P and PI(4,5)P2/PI4P pairs] also controls vacuole fusion and scission. Blockage of the PI(3,5)P2 production (fab1Δ, vac7Δ) leads to a single large round vacuole per cell.Citation6,Citation7 It is possible that Osh6 or Osh6ΔCC titrates PI(3,5)P2 and leads to round vacuoles, but this hypothesis is not supported by the current longevity data. Deletion of VAC14 decreases PI(3,5)P2 levels to about 10% of that in wild type and leads to large round vacuoles in almost every cell.Citation7 However, the lifespan of vac14Δ (MLS 17.8) Citation1 is much longer than the lifespan of PERG6‑OSH6ΔCC nyv1Δ (). Thus, the dramatic negative effect of the ORD domain of Osh6 on nyv1Δ may be caused by other mechanisms.
Another potential link between Osh6 and PI(3,5)P2 metabolism is suggested by our unpublished observation that high-copy OSH6 expression promoted vacuole fusion in an atg18Δ strain. The mutant atg18Δ has high levels of PI(3,5)P2.Citation8 By analogy with Osh3’s activation on the phosphatase Sac1,Citation4 high-copy OSH6 expression may activate phosphoinositide phosphatases to decrease PI(3,5)P2 levels and block vacuole scission. However, this explanation seems contradictory to the longevity effect of PERG6‑OSH6. Moderately upregulated PI(3,5)P2 levels are accompanied by extended lifespan. Deletion of selected phosphoinositide phosphatases of PI(3,5)P2 (inp53Δ, MLS 32.0) extends replicative lifespan.Citation1 The lifespan of atg18Δ is not shorter than that of wild type under caloric restriction.Citation2 Thus, the effect of Osh6 in an atg18Δ strain can be explained as follows. Elevated PI(3,5)P2 levels within the vacuolar membrane attract Osh6. The enrichment of Osh6 to the vacuolar membrane either brings sterols to the vacuolar membrane or re-organizes sterols within the vacuolar membrane (the left branch of PERG6-OSH6 in ).
Downregulation of TORC1 is also a potential mechanism of the longevity effect of Osh6.
Downregulation of TORC1 is one mechanism of CR-mediated longevity.Citation1 The activity of TORC1 is dependent on vacuolar functions.Citation34 With regard to lifespan extension, the activity of Tor1 is downstream of Vac8; a vac8Δ tor1Δ double mutant has the lifespan of tor1Δ.Citation2 In this study, we found that PERG6-OSH6 enriched Vac8 on mid-aged vacuolar membrane (). If this form of Vac8 sequesters Tco89 as our yeast two-hybrid assay suggested,Citation24 Perg6-OSH6 would then be expected to downregulate TORC1. Consistent with this prediction, PERG6‑OSH6 is sensitive to perturbations of TORC1 (). The dramatic shortened lifespan of the PERG6‑OSH6 tor1Δ double mutant () suggests that downregulation of TORC1 by both PERG6-OSH6 and deletion of TOR1 leads to a state where TORC1 cannot support normal cell growth. A recent study showed that active TORC1 promotes vacuole scission.Citation5 Thus, in addition to promoting fusion, the enriched Vac8 in PERG6-OSH6 may also downregulate TORC1 activity, which, in turn, slows down the scission process (the right branch of ).
An additional link between PERG6-OSH6 and TORC1 activity is related to the function of phosphatidic acid (PA), a vacuole fusion lipid.Citation3 The ORD domain alone binds PA 8-fold greater than the full-length Osh6.Citation13 This potential Osh6-ORD-PA interaction may inhibit vacuole fusion in PERG6-OSH6ΔCC nyv1Δ; however, this may not be the case for PERG6-OSH6ΔCC in wild type cells. In mammalian cells, PA binds and activates mTORC1.Citation35 If the same phenomenon is true for yeast cells, the interaction between Osh6 and PA may sequester PA from TORC1 and make TORC1 less sensitive to activation signals. A less active TORC1 cannot promote vacuole scission.Citation5 Thus, this potential interaction of Osh6-PA would work in a fashion similar to the Vac8-Tco89 interaction with regard to TORC1 activity.
In PERG6-OSH6 cells, other vacuole mechanisms relating to fusion (response to water) and scission (response to salt) are normal. In wild type or the nyv1Δ mutant, the pro-fusion effect mediated by sterol transport or sterol organization and the anti-scission effect mediated by downregulating TORC1 result in rapid fusion after each round of vacuole scission. Since longevity manipulations such as CR generate high demand for vacuole fusion, as evidenced by the highly fragmented vacuoles of pfa3Δ in CR,Citation30 rapid vacuole fusion would allow cells to accommodate this elevated demand, which promotes longevity ().
The observations that upregulation of OSH6 promoted vacuole fusion but downregulated sterol synthesis provide a unique opportunity to explore the mechanism by which PERG6-OSH6 enhances longevity. Previously, we observed that the vacuolar membrane deteriorates before generations 6–7.Citation2 The observations that Osh6 declines with age, and that PERG6-OSH6 expression delays this decline and increases the enrichment of Vac8, a vacuolar membrane protein for vacuole fusion, support the hypothesis that promoting vacuole fusion is sufficient to extend lifespan. The phenotypic overlap between PERG6-OSH6 expression and tor1Δ (), the localization of Tor1 on the vacuolar membrane,Citation36 and the vacuole-scission activity of TORC1Citation5 all suggest that manipulating the vacuolar membrane might be a novel route to slow the TOR-driven aging process.
Materials and Methods
Strains, plasmids, media, chemicals and yeast manipulation.
The wild type strain, BY4742 (Mat α; his3Δ1; leu2Δ0; lys2Δ0; ura3Δ0) and deletion strains in this same genetic background were purchased from Invitrogen and used for lifespan analyses unless specified otherwise. The construction of mutants is described below. High-copy OSH plasmids were kindly provided by Dr. C. Beh.Citation27 Yeast media: YEPD: yeast extract (1%)-peptone (2%)-dextrose (2%). Solid media contains 2% agar. Chemicals were purchased from Sigma-Aldrich unless otherwise stated. Replicative lifespans were measured as previously described in reference Citation2.
Construction of the PERG6-OSH6 and its mutants.
The PERG6-OSH6 strain was constructed by switching the endogenous promoter of OSH6 with the promoter of ERG6 via in vivo recombination (). The promoter of ERG6 on plasmid pRS316-ERG6 Citation37 was PCR-amplified using the primer PERG6OSH6up (5'-GCT GGG AAA GGA ACG GAA CCT TTT CCT TTC CGA GTT TCG GCC AGA TTT GAT TCG GTA ATC TCC-3') and the primer PERG6OSH6down (5'-CAA CCG GTG CGA ATC AGA TCC TAC GGT CAG TTT TTT GGA GCC CAT CAT CTT ATG CTG CCT ACT-3'). This PCR-amplified fragment contained the URA3 gene followed by the basal ERG6 promoter flanked by sequences homologous to the OSH6 promoter. This fragment was transformed into BY4742 to obtain URA+ transformants. Verification of the correct insertion-replacement was confirmed with primers RCERG6up (5'-ATA GTT CGG GTG TTT T-3') and RCOSH6down (5'-CCA TTT CAT TAC GTA C-3'). A 0.5 kb PCR-amplified fragment was used as the diagnostic band for the PERG6-OSH6 replacement.
The partial OSH6 knockout mutants in PERG6-OSH6 were constructed by replacing the portion of OSH6 with the HIS3 gene amplified from pRS413. For PERG6-OSH6Δ32–448, the HIS3 marker was amplified using the primer ΔOSH6Hisup (5'‑AAA TCC AGT TTT TCA AGT AAT AAG TCG TCA CAT TCA GCA ACA AAA TAG AGA GCA GAT TCT ACT GAG AGT-3') and the primer OSH6ΔCC down (5'-ATT GTT GAC CAG GTA AAA TAG GTG CTA TTT GCA ATA TGC TTC GTA TTT CTC GTT CAG AAT GAC ACG TAT-3'). For PERG6-OSH6Δ255–448, HIS3 was amplified using the primer OSH6Δ255His-up (5'-ATG ATA ATT AAA TCT CCT AAC TTC CAA GCT GAT ATA GAG TTC AAG TAG AGA GCA GAT TGT ACT GAG AGT-3') and OSH6ΔCCdown. For PERG6-OSH6Δ301–448, HIS3 was amplified with the primer OSH6ΔCCup (5'-AAT GAC GTT ATG TAT TTA AAG GAC TTA AAG CAA CCT CGT TCT TCA TAG AGA GCA GAT TCT ACT GAG AGT-3') and OSH6ΔCCdown. The PCR-amplified knockout cassettes were transformed into PERG6-OSH6 to obtain HIS+ transformants. Verification of the correct insertion-replacement was confirmed with primers OSHdHis-up (5'-CAT ATG ATA CAT GCT CTG-3') and OSHdHisdown (5'-AAC AAG TCG TCA TCT CTT-3'). A 1.3 kb PCR-amplified fragment was used as a diagnostic band for the replacement of OSH6 portion with HIS3.
In addition to BY4742, the URA3-PERG6 cassette was also introduced into a VAC8-GFP strain (LWY 7235)Citation2 and an OSH6-TAP (tandem affinity purification-tagged) strainCitation17 to study vacuoles and protein levels in aged cells. The PERG6-OSH6 nyv1Δ and P ERG6-OSH6 tor1Δ double mutants were constructed by mating the MAT a version of PERG6-OSH6 with nyv1Δ or tor1Δ in BY4742 followed by meiosis induction and tetrad dissection with standard yeast genetics protocols.Citation2
Construction of the PERG6-OSH5 mutant.
The PERG6-OSH5 strain was constructed using the same strategy for PERG6-OSH6. The promoter of ERG6 was PCR-amplified by the primer PERG6OSH5_Up (5'-ACG CCT GTC GCT ATG TAA AAT AAT AGC AAT CGT TTG TGA TCA CCA TTT GAT TCG GTA ATC TCC-3') and the primer PERG6OSH5_down (5'-TTT CAA AAA AGA AGT CCA AGA AGA TGA GCT TGC GTG TTG AGA CAT CAT CTT ATG CTG CCT ACT-3'). Verification primers were RCERG6up (5'-ATA GTT CGG GTG TTT T-3') and RCOsh5down (5'-AGC AGT ATT GAG ATC T-3'). A 0.5 kb PCR-amplified fragment was used as the diagnostic band for the PERG6-OSH5 replacement.
Real-time PCR analyses.
A ΔΔCt method was employed to compare the relative mRNA abundance of different genes. Yeast cells were incubated in YEPD liquid media overnight. The seed cultures were then inoculated into fresh media to an initial OD600 of 0.1. Cells were grown at 30°C for 7 h. The final OD600 were 0.85–0.99. 0.5 OD600 units of cells were collected and lysed with zymolyase (Zymo Research). Total RNA was isolated using the Qiagen RNeasy® mini kit according to the manufacturer’s protocol. An aliquot of 0.5 μg of RNA sample was used for each reverse transcription with the Qiagen QuantiTech Reverse Transcription Kit. The resulting cDNA was served as template to conduct real time PCR reaction with the primers listed in and Qiagen Sybr Green qPCR master mix. PCR was performed on the Bio-Rad CFX with the following parameters: 95°C 10 min, 40 cycles of 95°C 15 sec, 56°C 30 sec and 72°C 30 sec. After obtaining the Ct (cycle number at the threshold detection value) of each gene, the relative mRNA abundance of a gene in mutant relative to that in wild type is calculated by ΔΔCt = ΔCt (Ct of interested gene - Ct of ACT1) of wild type - ΔCt (Ct of interested gene - Ct of ACT1) of mutant.
Table 1. Primers used for real-time PCR
Western blot analyses.
Detection of the TAP signal by western analyses was performed with routine protocols for yeast cell extract.Citation24 A rabbit anti-TAP monoclonal antibody (Thermo Open Biosystem, CAB1001) was used at 1:2,000 dilutions. The internal loading control was detected by mouse anti-phosphoglycerate kinase Pgk1 antibody (Invitrogen).
Fluorescent microscopy.
Filamentous actin cables were stained with FITC-phalloidin following the protocol as described in Thevissen et al.Citation22 with 5 μM FITC labeled Phalloidin (sc‑301533, Santa Cruz Biotechnology). Protocols for Filipin staining and Lucifer yellow uptake assays from Beh and RineCitation11 were used. Filipin-labeled cells were visualized under the UV channel of fluorescence microscope. Lucifer yellow-labeled cells were visualized under the FITC channel of the fluorescence microscope. Vacuole morphology was analyzed using FM4–64 as described previously in reference Citation2.
Isolation of mid-aged cells and their analyses.
Mid-aged cells were isolated as described in reference Citation2. Briefly, one OD600 unit of early log phase cells were labeled with biotin and inoculated into 100 ml of fresh YEPD. Cells were then incubated for 12 h reaching log phase (OD600 was between 0.9–1.5). By calculation, the biotin-labeled cells had replicated approximately seven generations. An aliquot of cells were saved as the log phase control. The remaining cells were bound by streptavidin-iron beads and sorted by magnet. The log phase (young) cells and the sorted (mid-aged) cells were subjected to different assays. To analyze the mRNA abundance, total RNA was isolated from both young and mid-aged samples. The change of mRNA in mid-aged cells was measured by ΔΔCt = ΔCt (Ct of interested gene - Ct of ACT1) of log phase cells - ΔCt (Ct of interested gene - Ct of ACT1) of mid-aged cells.
Statistical analyses.
Aging assay data were analyzed using normality tests and plots to assess whether the distribution of the lifespan fitted a normal distribution. For normal distributed samples, a t-test was used. For non-normal distribution samples, the nonparametric test Wilcoxon rank-sum was used. For comparison of vacuolar morphology and actin staining, proportion tests were conducted to assess whether the differences were significant. All the analyses were performed using the Statistical Analysis Software (SAS®).
Acknowledgements
We thank Drs. Christopher Beh for the pCB (OSHox) plasmids, and Gary Eitzen for the pRS316-ERG6 plasmid. We thank Dr. Lois Weisman and Emily Kauffman for TAP strains and the YEp24 plasmid. We thank Drs. Kuo-kuang Wen and Peter Rubenstein for letting us using their fluorescent microscope. We thank Drs. Yao Liu and Nawab Ali in assisting the real-time PCR analyses. The authors are grateful for the two anonymous reviewers for suggestions on experiments with the PERG6-OSH6 nyv1Δ double mutant. This work is partially supported by the American Heart Association (09BGIA2290189) to F.T.
Disclosure of Potential Conflicts of Interest
No potential conflicts of interest were disclosed.
References
- Kaeberlein M, Powers RW 3rd, Steffen KK, Westman EA, Hu D, Dang N, et al. Regulation of yeast replicative lifespan by TOR and Sch9 in response to nutrients. Science 2005; 310:1193 - 6; http://dx.doi.org/10.1126/science.1115535; PMID: 16293764
- Tang F, Watkins JW, Bermudez M, Gray R, Gaban A, Portie K, et al. A life-span extending form of autophagy employs the vacuole-vacuole fusion machinery. Autophagy 2008; 4:874 - 86; PMID: 18690010
- Wickner W. Membrane fusion: five lipids, four SNAREs, three chaperones, two nucleotides, and a Rab, all dancing in a ring on yeast vacuoles. Annu Rev Cell Dev Biol 2010; 26:115 - 36; http://dx.doi.org/10.1146/annurev-cellbio-100109-104131; PMID: 20521906
- Stefan CJ, Manford AG, Baird D, Yamada-Hanff J, Mao Y, Emr SD. Osh proteins regulate phosphoinositide metabolism at ER-plasma membrane contact sites. Cell 2011; 144:389 - 401; http://dx.doi.org/10.1016/j.cell.2010.12.034; PMID: 21295699
- Michaillat L, Baars TL, Mayer A. Cell-free reconstitution of vacuole membrane fragmentation reveals regulation of vacuole size and number by TORC1. Mol Biol Cell 2012; 23:881 - 95; http://dx.doi.org/10.1091/mbc.E11-08-0703; PMID: 22238359
- Gary JD, Wurmser AE, Bonangelino CJ, Weisman LS, Emr SD. Fab1p is essential for PtdIns(3)P 5-kinase activity and the maintenance of vacuolar size and membrane homeostasis. J Cell Biol 1998; 143:65 - 79; http://dx.doi.org/10.1083/jcb.143.1.65; PMID: 9763421
- Duex JE, Tang F, Weisman LS. The Vac14p-Fig4p complex acts independently of Vac7p and couples PI3,5P2 synthesis and turnover. J Cell Biol 2006; 172:693 - 704; http://dx.doi.org/10.1083/jcb.200512105; PMID: 16492811
- Efe JA, Botelho RJ, Emr SD. Atg18 regulates organelle morphology and Fab1 kinase activity independent of its membrane recruitment by phosphatidylinositol 3,5-bisphosphate. Mol Biol Cell 2007; 18:4232 - 44; http://dx.doi.org/10.1091/mbc.E07-04-0301; PMID: 17699591
- Zinser E, Paltauf F, Daum G. Sterol composition of yeast organelle membranes and subcellular distribution of enzymes involved in sterol metabolism. J Bacteriol 1993; 175:2853 - 8; PMID: 8491706
- Bagnat M, Keränen S, Shevchenko A, Shevchenko A, Simons K. Lipid rafts function in biosynthetic delivery of proteins to the cell surface in yeast. Proc Natl Acad Sci U S A 2000; 97:3254 - 9; http://dx.doi.org/10.1073/pnas.060034697; PMID: 10716729
- Beh CT, Rine J. A role for yeast oxysterol-binding protein homologs in endocytosis and in the maintenance of intracellular sterol-lipid distribution. J Cell Sci 2004; 117:2983 - 96; http://dx.doi.org/10.1242/jcs.01157; PMID: 15173322
- Beh CT, Cool L, Phillips J, Rine J. Overlapping functions of the yeast oxysterol-binding protein homologues. Genetics 2001; 157:1117 - 40; PMID: 11238399
- Wang P, Duan W, Munn AL, Yang H. Molecular characterization of Osh6p, an oxysterol binding protein homolog in the yeast Saccharomyces cerevisiae. FEBS J 2005; 272:4703 - 15; http://dx.doi.org/10.1111/j.1742-4658.2005.04886.x; PMID: 16156791
- Raychaudhuri S, Im YJ, Hurley JH, Prinz WA. Nonvesicular sterol movement from plasma membrane to ER requires oxysterol-binding protein-related proteins and phosphoinositides. J Cell Biol 2006; 173:107 - 19; http://dx.doi.org/10.1083/jcb.200510084; PMID: 16585271
- Schulz TA, Prinz WA. Sterol transport in yeast and the oxysterol binding protein homologue (OSH) family. Biochim Biophys Acta 2007; 1771:769 - 80; PMID: 17434796
- Georgiev AG, Sullivan DP, Kersting MC, Dittman JS, Beh CT, Menon AK. Osh proteins regulate membrane sterol organization but are not required for sterol movement between the ER and PM. Traffic 2011; 12:1341 - 55; http://dx.doi.org/10.1111/j.1600-0854.2011.01234.x; PMID: 21689253
- Ghaemmaghami S, Huh WK, Bower K, Howson RW, Belle A, Dephoure N, et al. Global analysis of protein expression in yeast. Nature 2003; 425:737 - 41; http://dx.doi.org/10.1038/nature02046; PMID: 14562106
- Tarassov K, Messier V, Landry CR, Radinovic S, Serna Molina MM, Shames I, et al. An in vivo map of the yeast protein interactome. Science 2008; 320:1465 - 70; http://dx.doi.org/10.1126/science.1153878; PMID: 18467557
- Wang P, Zhang Y, Li H, Chieu HK, Munn AL, Yang H. AAA ATPases regulate membrane association of yeast oxysterol binding proteins and sterol metabolism. EMBO J 2005; 24:2989 - 99; http://dx.doi.org/10.1038/sj.emboj.7600764; PMID: 16096648
- Cakar ZP, Sauer U, Bailey JE, Müller M, Stolz M, Wallimann T, et al. Vacuolar morphology and cell cycle distribution are modified by leucine limitation in auxotrophic Saccharomyces cerevisiae. Biol Cell 2000; 92:629 - 37; http://dx.doi.org/10.1016/S0248-4900(01)01111-X; PMID: 11374441
- Munn AL, Heese-Peck A, Stevenson BJ, Pichler H, Riezman H. Specific sterols required for the internalization step of endocytosis in yeast. Mol Biol Cell 1999; 10:3943 - 57; PMID: 10564282
- Thevissen K, Ayscough KR, Aerts AM, Du W, De Brucker K, Meert EM, et al. Miconazole induces changes in actin cytoskeleton prior to reactive oxygen species induction in yeast. J Biol Chem 2007; 282:21592 - 7; http://dx.doi.org/10.1074/jbc.M608505200; PMID: 17553796
- Lindstrom DL, Leverich CK, Henderson KA, Gottschling DE. Replicative age induces mitotic recombination in the ribosomal RNA gene cluster of Saccharomyces cerevisiae. PLoS Genet 2011; 7:e1002015; http://dx.doi.org/10.1371/journal.pgen.1002015; PMID: 21436897
- Tang F, Peng Y, Nau JJ. Kauffman, E. J. and L. S. Weisman. Vac8p, an armadillo repeat protein, co-ordinates vacuole inheritance with multiple vacuolar processes. Traffic 2006; 7:1368 - 77; http://dx.doi.org/10.1111/j.1600-0854.2006.00458.x; PMID: 16824055
- Peng Y, Tang F, Weisman LS. Palmitoylation plays a role in targeting Vac8p to specific membrane subdomains. Traffic 2006; 7:1378 - 87; http://dx.doi.org/10.1111/j.1600-0854.2006.00472.x; PMID: 16978392
- Aronova S, Wedaman K, Anderson S, Yates J 3rd, Powers T. Probing the membrane environment of the TOR kinases reveals functional interactions between TORC1, actin, and membrane trafficking in Saccharomyces cerevisiae. Mol Biol Cell 2007; 18:2779 - 94; http://dx.doi.org/10.1091/mbc.E07-03-0274; PMID: 17507646
- Kozminski KG, Alfaro G, Dighe S, Beh CT. Homologues of oxysterol-binding proteins affect Cdc42p- and Rho1p-mediated cell polarization in Saccharomyces cerevisiae. Traffic 2006; 7:1224 - 42; http://dx.doi.org/10.1111/j.1600-0854.2006.00467.x; PMID: 17004323
- Plank M, Wuttke D, van Dam S, Clarke SA, de Magalhães JP. A meta-analysis of caloric restriction gene expression profiles to infer common signatures and regulatory mechanisms. Mol Biosyst 2012; 8:1339 - 49; http://dx.doi.org/10.1039/c2mb05255e; PMID: 22327899
- te Welscher YM, Jones L, van Leeuwen MR, Dijksterhuis J, de Kruijff B, Eitzen G, et al. Natamycin inhibits vacuole fusion at the priming phase via a specific interaction with ergosterol. Antimicrob Agents Chemother 2010; 54:2618 - 25; http://dx.doi.org/10.1128/AAC.01794-09; PMID: 20385867
- Smotrys JE, Schoenfish MJ, Stutz MA, Linder ME. The vacuolar DHHC-CRD protein Pfa3p is a protein acyltransferase for Vac8p. J Cell Biol 2005; 170:1091 - 9; http://dx.doi.org/10.1083/jcb.200507048; PMID: 16186255
- Strasser B, Iwaszkiewicz J, Michielin O, Mayer A. The V-ATPase proteolipid cylinder promotes the lipid-mixing stage of SNARE-dependent fusion of yeast vacuoles. EMBO J 2011; 30:4126 - 41; http://dx.doi.org/10.1038/emboj.2011.335; PMID: 21934648
- Brett CL, Kallay L, Hua Z, Green R, Chyou A, Zhang Y, et al. Genome-wide analysis reveals the vacuolar pH-stat of Saccharomyces cerevisiae. PLoS ONE 2011; 6:e17619; http://dx.doi.org/10.1371/journal.pone.0017619; PMID: 21423800
- Zhang YQ, Gamarra S, Garcia-Effron G, Park S, Perlin DS, Rao R. Requirement for ergosterol in V-ATPase function underlies antifungal activity of azole drugs. PLoS Pathog 2010; 6:e1000939; http://dx.doi.org/10.1371/journal.ppat.1000939; PMID: 20532216
- Zurita-Martinez SA, Puria R, Pan X, Boeke JD, Cardenas ME. Efficient Tor signaling requires a functional class C Vps protein complex in Saccharomyces cerevisiae. Genetics 2007; 176:2139 - 50; http://dx.doi.org/10.1534/genetics.107.072835; PMID: 17565946
- Fang Y, Vilella-Bach M, Bachmann R, Flanigan A, Chen J. Phosphatidic acid-mediated mitogenic activation of mTOR signaling. Science 2001; 294:1942 - 5; http://dx.doi.org/10.1126/science.1066015; PMID: 11729323
- Sturgill TW, Cohen A, Diefenbacher M, Trautwein M, Martin DE, Hall MN. TOR1 and TOR2 have distinct locations in live cells. Eukaryot Cell 2008; 7:1819 - 30; http://dx.doi.org/10.1128/EC.00088-08; PMID: 18723607
- Tedrick K, Trischuk T, Lehner R, Eitzen G. Enhanced membrane fusion in sterol-enriched vacuoles bypasses the Vrp1p requirement. Mol Biol Cell 2004; 15:4609 - 21; http://dx.doi.org/10.1091/mbc.E04-03-0194; PMID: 15254266