Abstract
The three-dimensional (3D) conformation of the genome is known to be structured and to affect gene transcription, but how chromatin conformation changes in diseases such as cancer is poorly understood. Similarly, oncogenic transcription factors bind to thousands of sites in the genome without a clear transcriptional role on nearby genes. Could these factors play a non-transcriptional role in promoting tumor progression by restructuring the shape of the genome? To address this question, we recently performed unbiased high-resolution mapping of intra- and inter-chromosome interactions upon overexpression of ERG, an oncogenic transcription factor frequently overexpressed in prostate cancer as a result of a gene fusion. By integrating data from genome-wide chromosome conformation capture (Hi-C), ERG binding and gene expression, we have demonstrated that oncogenic transcription factor overexpression is associated with global, reproducible and functionally coherent changes in chromatin organization. Perhaps more importantly, we have identified novel genomic alterations associated with ERG overexpression. These results suggest a yet unappreciated role for transcription factors in promoting genomic alterations through their effect on chromatin architecture.
The three-dimensional (3D) structure of the genome is not random and is now known to play an important role in gene expression.Citation1,Citation2 Investigations into how the genome folds in 3D have been greatly facilitated by the development of chromosome conformation capture (3C). More recently 3C was combined with next generation sequencing and led to Hi-C,Citation3 a technique that can identify interactions involving nearly all possible combinations of loci (intra- and inter-chromosomally). Hi-C has now been applied to study the structure of several human and mouse cell types,Citation4,Citation5 DrosophilaCitation6 and yeastCitation7 genomes. These studies have led to important findings regarding similarities in global chromatin interaction patterns between species and the role of the 3D chromatin conformation in development. Hi-C and related techniques have not, however, been directly applied to cancer. But should 3D chromatin organization really play an important role in cancer? Several lines of evidence indicate that it does, including recent studies that have shown an association between DNA looping and genomic alterations.Citation5,Citation8,Citation9 What other roles could 3D chromatin organization play? The observation that the oncogenic activation of transcription factors capable of binding to thousands of locations in the genome is an early event in many cancer types, often without any clear transcriptional impact on nearby genes, led us to hypothesize that these factors play a non-transcriptional role in organizing the 3D genome. Specifically, we hypothesized that these factors could act to program the formation of malignancy-related transcription hubs and further genomic alterations, e.g., inversions, deletions, translocations (). Myc overexpressionCitation10 and ETS rearrangements represent two early events that involve a DNA binding protein.Citation11,Citation12 Both genes are also involved in gene fusions either in prostate cancer (ERG) or in other cancer types, e.g., Ewing sarcoma (ERGCitation13), B cell lymphoma (MYCCitation14). Both do not result in a chimeric protein, but hijack an active promoter to elevate expression of the wild-type or truncated gene to induce either a pro-invasive (ERGCitation15-Citation17), pro-proliferative (MYCCitation18) or a dedifferentiation phenotype in prostate cancer (ERG and MYC).Citation11,Citation18,Citation19
Figure 1. (A) A schematic representation of our hypothesis showing conformational changes to the topology of a chromosome in prostate epithelial cells induced by ERG resulting in the formation of an activating transcription hub involving 3 pro-invasion genes. The dotted blue line represents the basement membrane and basal cell layer of a prostate gland. (B) Shown are the reconstruction 3D models of the organization of chromosome 6 in benign prostate epithelial cells (RWPE1) in the presence (right) or absence (left) of ERG.
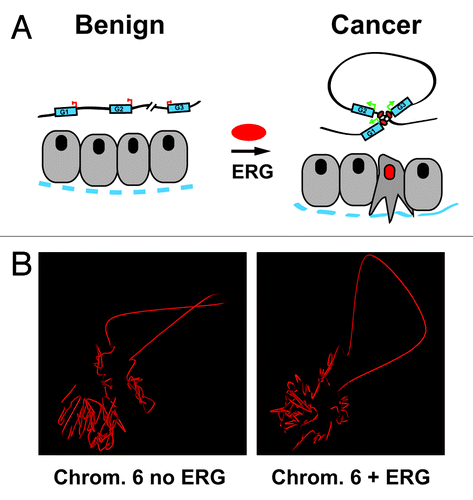
To test the hypothesis that oncogenic transcription factors play a key role in organizing chromatin architecture, we first sought to determine whether ERG overexpression is associated with global changes in chromatin structure. For this, we performed global mapping of interaction using the Hi-C technique in benign prostate epithelial cells engineered to overexpress the truncated form of ERG that is encoded by the most common ETS rearrangement in prostate cancer.Citation20 We found that ERG overexpression is associated with vast and reproducible changes in chromatin architecture, including roughly 9,000 differentially interacting intra- and inter-chromosomal interactions. Based on the Hi-C data, we also nominated hotspots of differential chromatin interaction as these loci demonstrate the most differential interactions with other loci. These hotspot loci are enriched with ERG binding sites based on genome-wide binding assays (ChIPseq) for ERG performed in the same cells. In addition, based on global transcriptome (RNaseq) data, we found that 65% of the 1,266 deregulated genes are also associated with ERG-associated interacting loci. Interestingly, this “interacting gene set” was enriched with genes that encode proteins relevant to aggressive prostate cancer, including genes associated with cell migration and invasion (e.g., FYN) and urogenital development (e.g., HOXA, B, C gene cluster members, PYGO1 and NKX3.1). This is consistent with ERG expression disrupting the normal lineage-specific prostate cell differentiation and promoting an EZH2-mediated dedifferentiation program.Citation19 Our initial Hi-C data revealed a strong inter-chromosomal interaction involving chromosomes 13 and 15 that was uniquely present in ERG-expressing cells, not in presumed genetically identical control cells. However, using spectral karyotyping, we found that the presumed interaction was in fact a novel translocation involving chromosomes 13 and 15 specifically in the ERG-overexpressing cells. Interestingly, we also found that ERG binding was significantly enriched at these loci. The novel translocation was further confirmed using multi-color FISH. Could ERG overexpression have caused this novel translocation? A number of observations indicate that this is a plausible scenario. For example, it has been shown that ERG induces DNA damage,Citation21 and that there is a positive correlation between the location of breakpoints in ETS-rearranged tumor cells and transcriptional or chromatin compartments of active genes.Citation22 The spectral karyotyping results give support to the interpretation that inter-chromosomal interactions detected by Hi-C often represent translocations, but not always, as several ERG-specific trans-interactions could not be accounted for by translocations using FISH. However, given the low resolution for detecting rearrangements using this technique, we could not entirely rule out translocations accounting for the trans-interactions involving smaller chromosomal regions that were enriched in the ERG-overexpressing cells. We anticipate that coupling future Hi-C studies with whole-genome sequencing will represent a more sensitive approach to determine structural changes. This combined approach should better resolve differences between loci proximity and genomic rearrangements. Whole-genome sequencing for Hi-C data interpretation does not necessarily need to have extremely deep coverage: low-pass DNA sequencing is being used to screen for structural variation, including chromothripsisCitation23 (and Jan Korbel, personal communication).
Genomic alterations will also need to be taken into consideration when reconstructing the 3D shape of the genome using Hi-C data. Using the Hi-C data generated as part of our study, we have performed a preliminary reconstruction of the 3D topology of human chromosome 6 in the presence and absence of ERG expression (), as the M-FISH did not indicate a translocation event. For this, we used a force-based layout algorithm. Briefly, 1 Mb blocks are considered to be nodes, and Hi-C distances are calculated between all pairs of nodes/blocks using a formula that takes into account the volume in which the chromosomes can move.Citation24 Starting from a random topology, we update node positions iteratively so as to best respect the Hi-C distances. After convergence, the reconstruction error is calculated, multiple random initializations are run; and the topology that minimizes the reconstruction error is kept. Using this approach, our reconstructed topologies of the chromosomes in ERG-overexpressing and control cells recapitulate well the Hi-C distances (< 35% error). These models are limited to representing an average 3D chromatin state and do not take into consideration the diploid nature of the genome. However, single nucleotide polymorphisms (SNPs) obtained from whole-genome sequencing may in the future help identify allele-specific interactions and resolve inter-allele contacts. In addition to sometimes complex structural variations, such as chromothripsis,Citation25 reconstructing the 3D structure of cancer genome will encounter additional challenges such as tumor heterogeneity: distinct populations of tumor cells may have different global chromatin architectures and distinct genomic rearrangements. Not accounting for these factors will greatly limit the interpretation of these types of models.
Taken together, our initial data suggests that ERG may contribute genome-wide by reorganizing the chromatin within the nucleus, facilitating both the formation of pro-malignancy transcription hubs and, perhaps more importantly, the development of genomic alterations. However, the precise nature of ERG’s causal role in these processes needs to be elucidated. Such characterization could be achieved, for example, using an inducible system in which the timing of ERG expression can be tightly controlled, and alterations in proximity and DNA binding are quantified concurrently. More work is also needed to unravel the mechanism accounting for these architectural changes. Which protein complexes are responsible for these changes? What are the nuclear domains involved? Are there any epigenetic modifications associated with these changes, and what is their functional meaning? Do other oncogenic transcription factors alter the 3D structure of the cancer genome? FLI1, for example, is in the same phylogenetic clade as ERG and is overexpressed as a fusion protein with EWS in 85% of Ewing sarcoma cases,Citation13,Citation26 suggesting that FLI1 may alter chromatin topology in a manner similar to ERG. In addition, MYC has been shown to play a non-transcription role in regulating replication, and it was found that MYC deregulation was associated with the appearance of γ-H2A.X and other DNA markers of the DNA damage response pathway.Citation27 Answering these questions will tremendously advance our understanding of how oncogenic transcription factors drive malignancy.
Acknowledgments
This work was supported by funding from the Department of Defense New Investigator Award (PC081337, D.S.R.), National Science Foundation CAREER grant (1054964, O.E.), the National Institute of Health (NCI CA125612, M.A.R.) and the Starr Cancer Consortium (M.A.R.).
References
- Osborne CS, Chakalova L, Brown KE, Carter D, Horton A, Debrand E, et al. Active genes dynamically colocalize to shared sites of ongoing transcription. Nat Genet 2004; 36:1065 - 71; http://dx.doi.org/10.1038/ng1423; PMID: 15361872
- Sexton T, Schober H, Fraser P, Gasser SM. Gene regulation through nuclear organization. Nat Struct Mol Biol 2007; 14:1049 - 55; http://dx.doi.org/10.1038/nsmb1324; PMID: 17984967
- Lieberman-Aiden E, van Berkum NL, Williams L, Imakaev M, Ragoczy T, Telling A, et al. Comprehensive mapping of long-range interactions reveals folding principles of the human genome. Science 2009; 326:289 - 93; http://dx.doi.org/10.1126/science.1181369; PMID: 19815776
- Dixon JR, Selvaraj S, Yue F, Kim A, Li Y, Shen Y, et al. Topological domains in mammalian genomes identified by analysis of chromatin interactions. Nature 2012; 485:376 - 80; http://dx.doi.org/10.1038/nature11082; PMID: 22495300
- Zhang Y, McCord RP, Ho YJ, Lajoie BR, Hildebrand DG, Simon AC, et al. Spatial organization of the mouse genome and its role in recurrent chromosomal translocations. Cell 2012; 148:908 - 21; http://dx.doi.org/10.1016/j.cell.2012.02.002; PMID: 22341456
- Sexton T, Yaffe E, Kenigsberg E, Bantignies F, Leblanc B, Hoichman M, et al. Three-dimensional folding and functional organization principles of the Drosophila genome. Cell 2012; 148:458 - 72; http://dx.doi.org/10.1016/j.cell.2012.01.010; PMID: 22265598
- Duan Z, Andronescu M, Schutz K, McIlwain S, Kim YJ, Lee C, et al. A three-dimensional model of the yeast genome. Nature 2010; 465:363 - 7; http://dx.doi.org/10.1038/nature08973; PMID: 20436457
- De S, Michor F. DNA replication timing and long-range DNA interactions predict mutational landscapes of cancer genomes. Nat Biotechnol 2011; 29:1103 - 8; http://dx.doi.org/10.1038/nbt.2030; PMID: 22101487
- Fudenberg G, Getz G, Meyerson M, Mirny LA. High order chromatin architecture shapes the landscape of chromosomal alterations in cancer. Nat Biotechnol 2011; 29:1109 - 13; http://dx.doi.org/10.1038/nbt.2049; PMID: 22101486
- Gurel B, Iwata T, Koh CM, Jenkins RB, Lan F, Van Dang C, et al. Nuclear MYC protein overexpression is an early alteration in human prostate carcinogenesis. Mod Pathol 2008; 21:1156 - 67; http://dx.doi.org/10.1038/modpathol.2008.111; PMID: 18567993
- Koh CM, Bieberich CJ, Dang CV, Nelson WG, Yegnasubramanian S, De Marzo AM. MYC and Prostate Cancer. Genes Cancer 2010; 1:617 - 28; http://dx.doi.org/10.1177/1947601910379132; PMID: 21779461
- Perner S, Mosquera JM, Demichelis F, Hofer MD, Paris PL, Simko J, et al. TMPRSS2-ERG fusion prostate cancer: an early molecular event associated with invasion. Am J Surg Pathol 2007; 31:882 - 8; http://dx.doi.org/10.1097/01.pas.0000213424.38503.aa; PMID: 17527075
- Delattre O, Zucman J, Melot T, Garau XS, Zucker JM, Lenoir GM, et al. The Ewing family of tumors--a subgroup of small-round-cell tumors defined by specific chimeric transcripts. N Engl J Med 1994; 331:294 - 9; http://dx.doi.org/10.1056/NEJM199408043310503; PMID: 8022439
- Dalla-Favera R, Bregni M, Erikson J, Patterson D, Gallo RC, Croce CM. Human c-myc onc gene is located on the region of chromosome 8 that is translocated in Burkitt lymphoma cells. Proc Natl Acad Sci U S A 1982; 79:7824 - 7; http://dx.doi.org/10.1073/pnas.79.24.7824; PMID: 6961453
- Klezovitch O, Risk M, Coleman I, Lucas JM, Null M, True LD, et al. A causal role for ERG in neoplastic transformation of prostate epithelium. Proc Natl Acad Sci U S A 2008; 105:2105 - 10; http://dx.doi.org/10.1073/pnas.0711711105; PMID: 18245377
- Rickman DS, Chen YB, Banerjee S, Pan Y, Yu J, Vuong T, et al. ERG cooperates with androgen receptor in regulating trefoil factor 3 in prostate cancer disease progression. Neoplasia 2010; 12:1031 - 40; PMID: 21170267
- Tomlins SA, Laxman B, Varambally S, Cao X, Yu J, Helgeson BE, et al. Role of the TMPRSS2-ERG gene fusion in prostate cancer. Neoplasia 2008; 10:177 - 88; http://dx.doi.org/10.1593/neo.07822; PMID: 18283340
- Koh CM, Iwata T, Zheng Q, Bethel C, Yegnasubramanian S, De Marzo AM. Myc enforces overexpression of EZH2 in early prostatic neoplasia via transcriptional and post-transcriptional mechanisms. Oncotarget 2011; 2:669 - 83; PMID: 21941025
- Yu J, Yu J, Mani RS, Cao Q, Brenner CJ, Cao X, et al. An integrated network of androgen receptor, polycomb, and TMPRSS2-ERG gene fusions in prostate cancer progression. Cancer Cell 2010; 17:443 - 54; http://dx.doi.org/10.1016/j.ccr.2010.03.018; PMID: 20478527
- Rickman DS, Soong TD, Moss B, Mosquera JM, Dlabal J, Terry S, et al. Oncogene-mediated alterations in chromatin conformation. Proc Natl Acad Sci U S A 2012; 109:9083 - 8; http://dx.doi.org/10.1073/pnas.1112570109; PMID: 22615383
- Brenner JC, Ateeq B, Li Y, Yocum AK, Cao Q, Asangani IA, et al. Mechanistic rationale for inhibition of poly(ADP-ribose) polymerase in ETS gene fusion-positive prostate cancer. Cancer Cell 2011; 19:664 - 78; http://dx.doi.org/10.1016/j.ccr.2011.04.010; PMID: 21575865
- Berger MF, Lawrence MS, Demichelis F, Drier Y, Cibulskis K, Sivachenko AY, et al. The genomic complexity of primary human prostate cancer. Nature 2011; 470:214 - 20; http://dx.doi.org/10.1038/nature09744; PMID: 21307934
- Rausch T, Jones DT, Zapatka M, Stütz AM, Zichner T, Weischenfeldt J, et al. Genome sequencing of pediatric medulloblastoma links catastrophic DNA rearrangements with TP53 mutations. Cell 2012; 148:59 - 71; http://dx.doi.org/10.1016/j.cell.2011.12.013; PMID: 22265402
- Marti-Renom MA, Mirny LA. Bridging the resolution gap in structural modeling of 3D genome organization. PLoS Comput Biol 2011; 7:e1002125; http://dx.doi.org/10.1371/journal.pcbi.1002125; PMID: 21779160
- Stephens PJ, Greenman CD, Fu B, Yang F, Bignell GR, Mudie LJ, et al. Massive genomic rearrangement acquired in a single catastrophic event during cancer development. Cell 2011; 144:27 - 40; http://dx.doi.org/10.1016/j.cell.2010.11.055; PMID: 21215367
- Hollenhorst PC, Shah AA, Hopkins C, Graves BJ. Genome-wide analyses reveal properties of redundant and specific promoter occupancy within the ETS gene family. Genes Dev 2007; 21:1882 - 94; http://dx.doi.org/10.1101/gad.1561707; PMID: 17652178
- Dominguez-Sola D, Ying CY, Grandori C, Ruggiero L, Chen B, Li M, et al. Non-transcriptional control of DNA replication by c-Myc. Nature 2007; 448:445 - 51; http://dx.doi.org/10.1038/nature05953; PMID: 17597761