Abstract
Embryonic stem cells (ESC) are able to self-renew and to differentiate into any cell type. To escape error transmission to future cell progeny, ESC require robust mechanisms to ensure genomic stability. It was stated that stress defense of mouse and human ESC against oxidative stress and irradiation is superior compared with differentiated cells. Here, we investigated heat shock response of human ESC (hESC) and their differentiated progeny. Fibroblast-like cells were generated by spontaneous hESC differentiation via embryoid bodies. Like normal human diploid fibroblasts, these cells have a finite lifespan in culture, undergo replicative senescence and die. We found that sublethal heat shock affected survival of both cell types, but in hESC it induced apoptosis, whereas in differentiated cells it produced cell cycle arrest and premature senescence phenotype. Heat shock survived hESC and differentiated cells restored the properties of initial cells. Heated hESC progeny exhibited pluripotent markers and the capacity to differentiate into the cells of three germ layers. Fibroblast-like cells resisted heat shock, proliferated for a limited number of passages and entered replicative senescence as unheated parental cells. Taken together, these results show for the first time that both hESC and their differentiated derivatives are sensitive to heat shock, but the mechanisms of their stress response are different: hESC undergo apoptosis, whereas differentiated cells under the same conditions exhibit stress-induced premature senescence (SIPS) phenotype. Both cell types that survived sublethal heat shock sustain parental cell properties.
Introduction
At early stages of embryogenesis the cells are pluripotent and ensure the development of all cell diversity in the adult organism. They must have mechanisms, probably more precise than in other somatic cells, to maintain the genome integrity, because errors may be inherited by developing cells. It was observed that a common feature of early human embryos at morula and blastocyst stages are scatted cells dying by apoptosis.Citation1 It implies that an approach to escape damage transmission to the next cell generation is elimination of abnormal cells.
Human embryonic stem cells (hESC) are established from puripotent inner mass cells of blastocyte stage embryos. ESC sustain in vitro pluripotent properties and, therefore, also should have mechanisms to maintain genomic stability. hESC are immortal cells capable for unlimited proliferation. A number of studies were focused on human ESC genetic stability during long-term cultivation. Although many cell lines maintain normal diploid karyotype during prolonged cultivation, hESC lines with chromosomal rearrangements detected even by conventional karyotypic methods are also described.Citation2
Not much is known about ESC strategies for genetic stability. Several mechanisms are proposed regarding how ESC may avoid errors that may be transmitted to their progeny. It was proposed that ESC are hypersensitive to environmental challenges, and damaged cells are eliminated by apoptosis.Citation3 There are limited and controversial data on hESC sensitivity to genotoxic agents compared with differentiated cells. Thus, hESC displayed higher resistance to mytomycin C, a potent DNA cross-linker, manifested as less frequency of mitomycin C induced chromosome aberrations than in fetal lung fibroblasts.Citation4 According to an MTT test, viability of ESC treated with low doses of hydrogen peroxide was higher than viability of dermal fibroblasts exposed to equal oxidative stress.Citation5 However, most data suggest that hESC are very prone to apoptosis induced by DNA-damaged agents: etoposide, a topoisomerase II inhibitor,Citation6,Citation7 UV-exposureCitation8 (Luo, 2012) and γ-irradiation.Citation9 Paradoxically, but in spite of hESC high susceptibility to genotoxic agents, DNA repair system in hESC is more efficient relative to non-pluripotent cells. hESC readily repair DNA damages generated from H2O2, UV and ionizing radiation.Citation8,Citation10
Another possible mechanism that contributes to preservation of hESC genomic integrity is their more efficient stress defense. It was stated first for mouse embryonic stem cellsCitation11 and later confirmed for human ESC and induced pluripotent cells,Citation12,Citation13 that stress defense of ESC is superior to that of differentiated cells. The authors examined radiation-induced and oxidative stress and expression of genes implicated in stress defense. They demonstrated that most genes involved in DNA repair and protection from reactive oxygen species are downregulated during ESC differentiation. Among stress defense genes downregulated during ESC differentiation, the authors indicated genes responsible for heat shock proteins (Hsp). It is well known that heat shock proteins are associated with cell stress response. Although, initially their modified expression was registered in cells under elevated temperature, later it became obvious that Hsp synthesis may be also induced by various chemical agents, UV-irradiation, inflammation, etc.Citation14 These proteins are molecular chaperones whose general function is to assist proper protein conformation and assembly. The key players in heat-stress response are the proteins of Hsp70 family. There are two major isoforms of Hsp70 proteins: constitutive (Hsc70) and inducible (Hsp70) ones. Inducible Hsp70 may be constitutively expressed in some cells, mostly in tumor cells, but its expression is drastically elevated in cells that underwent stress. Hsp70 protects cells from adverse consequences of stress not only because of its chaperone activity, but also due to its anti-apoptotic function.Citation14 To our knowledge, there is no data on hESC response to heat-stress. Taking into account the reported hsp70 downregulation during differentiation,Citation13 it was interesting to compare heat-shock response of hESC and their differentiated progeny.
The aim of the work was to investigate heat-shock response of hESC and their fibroblast-like derivates. As little is known about the properties of hESC that survived any damaging insult, more specifically whether their pluiripotency is maintained or lost, we monitored the parental properties in heat-shock survived cells. Our results show that under equal sublethal heat shock conditions, hESC died by apoptosis, whereas their fibroblast-like progeny exhibited premature cell senescence phenotype. hESC and fibroblast-like cells that survived heat shock sustained the properties of parental cells: survived hESC maintained pluripotency; fibroblast-like cells remained diploid and underwent replicative senescence.
Results
Cells
Human embryonic stem cells hESC C910 have typical hESC properties: cell renewal, diploid karyotype, expressed pluripotent markers, capacity for differentiation into three germ layer cells and produced teratoma. Fibroblast-like cells are spontaneously differentiated cells from hESC C910 embryoid bodies. Like normal human fibroblasts, these cells have diploid chromosome number and a finite lifespan in culture. They can be serially propagated in culture for about 30–35 population doublings (PD), then ceased to divide and slowly died. During early passages, the cells are actively proliferate but then enter the state of replicative senescence. Senescent cells undergo irreversible cell cycle arrest and display altered morphology (become enlarged and flattened) and senescence-associate β-galactosidase activity (SA-β-Gal) (). Experiments have been performed on the cells within 10–14 PD (passages 5–7).
Expression of heat shock proteins in human embryonic stem cells and their differentiated derivates
Proteins of HSP70 family are key players in cellular response to heat and other stresses. There are two isoforms of Hsp70 protein: inducible (Hsp70) and constitutive (Hsc70). We examined expression and localization of these isoforms in undifferentiated and differentiated hESC C910 cells under normal conditions and after heat shock (). Slight difference in expression of constitutive Hsc70 was observed between these cells: the level of its expression was slightly higher in undifferentiated hESC C910 cells than in the differentiated progeny. Heat shock insignificantly increased its content in both cell types; Hsc70 induction was more evident in undifferentiated cells (). Expression pattern of Hsp70 was different. Differentiated cells are distinguished from parental ESC by higher level of basal Hsp70 expression (). It is known that inducible Hsp70 isoform may be constitutively expressed. Heat shock enhanced Hsp70 expression in both cell types (). Maximal induction of Hsp70 in undifferentiated cells was observed under mild heat shock (45°C, 10 min) conditions. Severe heat shock (45°C, 30 min) slightly inhibited Hsp70 induction in embryonic stem cells but not in their differentiated counterparts (). Immunofluorescence assay of permeabilized cells showed that the pattern of Hsc70 localization in these cells was common to that observed in other somatic cells (). Both constitutive Hsc70 and inducible Hsp70 proteins were slightly discernible in unheated cells. After heat shock, Hsp70 translocate into nuclei. Nuclear localization of inducible Hsp70 in hESC was the most apparent under mild heat shock conditions. Severe heat shock dramatically reduced the number of Hsp70-positive cells in cultures of undifferentiated cells but not in cultures of their differentiated derivates (). Since the proteins of HSP70 family are found on the surface of some cells, including human ESC,Citation15 we examined possible expression of Hsp70 and Hsc70 proteins on the surface of hESC and their differentiated derivates. Experiments were performed on living cells. We revealed that undifferentiated but not differentiated hESC C910 cells express Hsc70 on their surface (). Antibodies to Hsp70 we employed did not reveal the presence of inducible Hsp70 isoform on the surface either undifferentiated or differentiated cells.
Figure 2. Expression and localization of Hsp70 and Hsc70 proteins in hESCC910 and their differentiated progeny. hESC and differentiated fibroblast-like cells were subjected to mild (45°C, 10 min) or severe (45°C, 30 min) heat-shock, recovered for 3 h under normal conditions and assayed by immunoblot-blot (A), RT-PCR (B) and immunofluorescence. (C and D). (C) Antibodies to Hsc70 visualize Hsc70 protein on the surface of hESC 910 but not the differentiated derivates. Living cells were first treated with antibodies to Hsc70, than fixed and treated with secondary antibodies. (D) Cells were fixed, permeabilized and then treated with antibodies to Hsp70. Heat shock induced Hsp70 translocation into the nucleus in both cell types. Note the reduced number of Hsp70-positive cells in hESC 910 culture after severe heat shock.
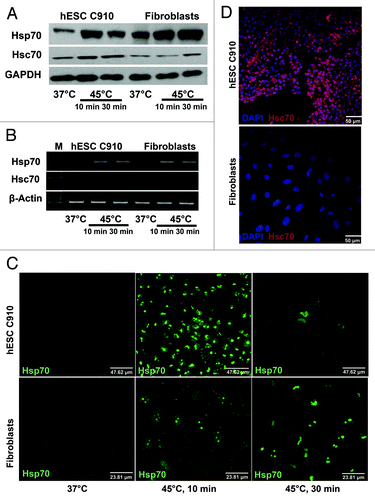
Heat-shock induced apoptosis in HESC but not in their differentiated derivates
Mild heat-shock (45°C, 10 min) did not affect the cell viability measured by annexin V/PI staining of both undifferentiated and differentiated cells. Severe heat shock (45°C, 30 min) reduced the number of viable hESC C910 cells from 88% to 57.5%; viability of differentiated fibroblast-like cells under severe heat shock conditions remained close to the unheated cells (). Most of hESC C910 cells subjected to the severe heat shock died by apoptosis. Apoptotic death was quantified by flow cytometry and counting cells with nuclear fragmentation (). Notably, intact hESC C910 cultures exhibit a relatively high rate of annexin V-positive cells and cells with fragmented nuclei (about 10%). Many researchers note that the high level of spontaneous apoptosis is typical for human ESC cultures.Citation16 Of interest, mouse ESC under standard culture conditions do not display a high rate of spontaneous apoptosis.Citation17 Severe heat shock increased the number of apoptotic embryonic stem cells by more than twice (about 30%) but did not alter the number of apoptotic cells in cultures of differentiated cells. As caspases, in particular caspase 3, are involved in the regulation and implementation of the apoptotic program we examined expression of caspase 3 in intact and heated undifferentiated and differentiated cells and found that hESC C910 cells had relatively high level of caspase 3 expression, which was enhanced after heat shock. Caspase 3 expression was hardly detectable in both unheated and heated differentiated cells ().
Figure 3. Heat shock induced apoptosis in hESC 910 but not in differentiated fibroblastic progeny. The cells were subjected to mild (45°C, 10 min) or severe (45°C, 30 min) heat-shock and recovered for 3 h under normal conditions. (A) RT-PCR, heat-shock enhanced expression of caspase 3 only in hESC 910 cells. (B) Apoptosis was measured by counting cells with fragmented nuclei. Cells were stained with DAPI. (C) Apoptosis was assayed by annexin V/PI staining and flow cytometry.
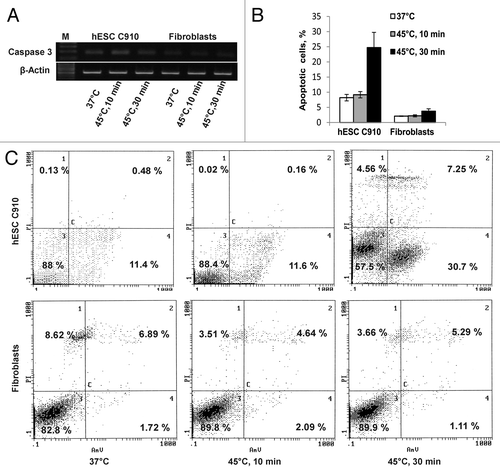
Heat-shock induced cell cycle arrest and SIPS phenotype of differentiated progeny but not HESC
Cell number in fibroblast-like cultures exposed to the heat shock (45°C, 30 min) and returned to the normal conditions was counted during 72 h. Growth curves of intact and heated differentiated cells are presented in . It is seen that cell number in control cultures increased about three times, whereas heated cells did not divide. Cell cycle analysis by flow cytometry shows that these cells are arrested in G1 and G2/M phases (). Proliferation status of these cells was also verified by staining with antibodies to proliferation marker Ki-67. demonstrates that in 3 days after severe heat shock, most fibroblast-like cells are Ki-67-negative, i.e., ceased to divide. Cell cycle arrest provoked by various stress factors in normal fibroblasts is a hallmark of the phenomenon called stress-induced premature senescence (SIPS). Other SIPS markers are altered сell morphology and expression of SA-β-Gal. Twenty-four hours after heat shock, enlarged and flattened SA β-gal-positive cells were observed in cultures of fibroblast-like cells. Their number steadily increased and in 3 d the number of SA β-gal-positive cells was about 60% ().
Figure 4. Heat shock induced cell cycle arrest and SIPS phenotype in differentiated progeny. The fibroblast-like cells were heated at 45°C for 30 min, returned under normal culture conditions at 37°C and assayed 24, 48 and 72 h after heat shock. (A) Growth curve of heated and unheated cells. (B) Analysis of cell cycle distribution by flow cytometry. (C) Staining pattern of Ki-67 expression as a time-course after heat shock. Note that only single Ki-67-positive cells are visible 72 h after the heat shock. (D) SA-β-Gal staining at various time intervals after heat shock. Note that 72 h after heat shock most cells are SA-β-Gal-positive. (E) Quantitative assay of SA-β-Gal-positive cells in fibroblast-like cultures 72 h after heat shock.
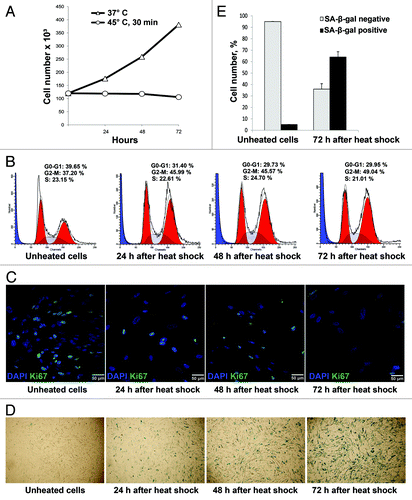
No apparent perturbation in the cell cycle progression was observed in undifferentiated hESC C910 cultures exposed severe heat shock (45°C, 30 min) and recovered for 24 h. Cells that survived heat shock continue to proliferate as the parental cells (). We also did not observe SA β-gal-positive cells in cultures of heat-shocked hESC C910 cells (). Thus, differentiated cells unlike undifferentiated ESC respond to the heat shock by inhibition of proliferation and display premature cell senescence phenotype.
Figure 5. Heat shock did not induce hESC910 cell senescence. hESC 910 cells were heated at 45°C for 30 min and returned under normal conditions at 37°C for 24 h. Control and heated cells were assayed for cell cycle distribution and stained for expression of SA-β-Gal (A). Heat shock does not cause cell cycle arrest of hESC C910 cells. (B) Only single morphologically modified, probably, differentiated cells are SA-β-Gal-positive.
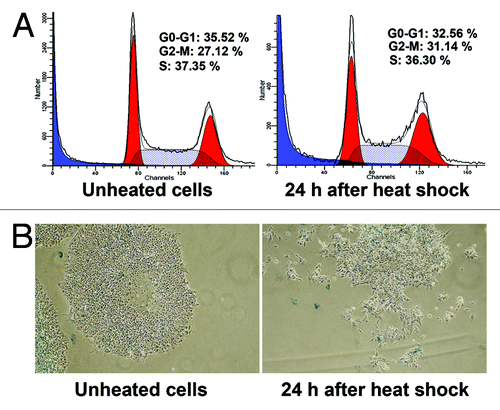
Cell properties of heat shock survived fractions of embryonic stem cells and their differentiated derivates
Heat-shock conditions we employed (45°C, 30 min) are sublethal as only a part of population responded by apoptosis in ESC culture or SIPS in differentiated fibroblasts. To investigate whether the stress had irreversible impact on proliferation of differentiated cells 4 d after the heat shock, the cells were plated into new culture dishes. Some cells adhered to culture vessels. Fibroblast-like cells survived heat shock, restored normal morphology, resumed proliferation and continued to divide as not-heated cells. With increasing PD number, the recovered cell populations exhibited enhanced number of cells with senescence phenotype and, at last, ceased growth-reaching replicative senescence stage after about 30–35 PD (17–19 passages) as their parental cells did (). Thus, the fraction of fibroblast-like cells that survived the heat shock retain the properties of initial cells, at least, morphology and lifespan limit.
Figure 6. Differentiated hESC derivates that survived sublethal heat shock exposure undergo replicative senescence as their parental cells. The fibroblast-like cells were exposed to heat shock (45°C, 30 min) at passage 7; survived cells were assayed for SA-β-Gal activity at passage 3 (7+3) and 12 (7+12).
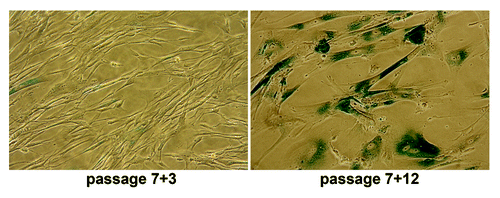
We also addressed the fate of undifferentiated hESC C910 cells that survived the heat shock. Twenty-four hours after the heat-shock, the cells were harvested and seeded under routine conditions on the feeder layer. Survived cells generated colonies with usual morphology. The colonies were passaged mechanically as initial cells. At passages 3–5 we tested these cells for the expression of pluripotent markers. demonstrates that survived human embryonic cells have typical morphology and expressed common pluripotent markers: Oct. 4, SSEA4, ABCG2 and alkaline phosphatase (AP). To verify whether survived cells are pluripotent, we generated EB from these cells and then allow them spontaneously differentiate. shows that the progeny of survived cells retain the capacity for differentiation into three germ layers. Thus, human embryonic stem cells that survived heat-shock retain the key properties of embryonic stem cells: self-renewal, expression of pluripotent markers and pluripotency.
Figure 7. Survived fraction of hESC910 exposed to sublethal heat shock sustains pluripotency. hESC 910 cells were heated at 45°C for 30 min and returned under normal cell culture conditions. Survived cells were assayed for pluripotent markers and capacity for differentiation. (A) Expression of pluripotent markers: immunocytochemical assay and analysis of alkaline phosphatase activity. (B) Survived cells are able to differentiate into three-germ layer cells: ectoderm (ІІІβ-tubulin), mesoderm (vimentin, muscle α-actinin) and endoderm (α-fetoprotein).
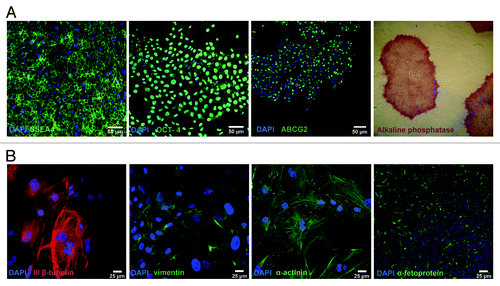
Discussion
It is generally accepted that hESC derived from pluripotent cells of early embryos should employ mechanisms, which guarantee their genome integrity. Multiple mechanisms of hESC genetic stability are suggested and discussed.Citation8,Citation18 It was postulated that ESC exhibit higher stress tolerance than differentiated cells against oxidative and UV-induced DNA damage. Differentiation was accompanied by downregulation of antioxidant genes and genes involved in DNA repair.Citation12,Citation13 However, hESC response to other stress triggers which induce DNA damage was unknown. In this work, we examined the heat-shock response of hESC and their differentiated derivates.
Although hyperthermia appears to not have a single intracellular target indirectly, it produces DNA damage and induces apoptosis.Citation19 Key players in cell defense from heat-shock are heat-shock proteins. An argument in favor of higher stress resistance of ESCCitation11,Citation20 was downregulation of HSPA1B (Hsp70) protein, an inducible isoform of major heat-shock protein, in differentiated cells. If so, and taking into account that heat shock proteins are critical in protection from cell injury, it was possible to suppose that hESC would be more resistant to hyperthermia than their differentiated progeny. However, the results we obtained were opposite to those expected. Unlike previous reports on downregulation of Hsp70 during ESC differentiation in our experiments, its content was lower in hESC than in differentiated progeny. Upregulation of Hsp70 was also observed in hESC induced to differentiation by histone deacetylase inhibitors.Citation21
hESC and differentiated derivates were slightly different in Hsc70 (constitutive isoform) expression: it was a little higher in hESC. Moreover, we detected Hsc70 on the surface of ESC but not on their differentiated progeny. These observations agree with previously reported data on the presence of HSPA8 (Hsc70 constitutive isoform) on the surface of human ESC. It was even proposed to consider it as a putative cell-surface marker for undifferentiated hESC.Citation15 Biological significance of surface Hsp70 expression in pluripotent cells is not clear. It is possible to assume that it plays an important role in fertilization and early development of mammalian embryos. The guess is based on experiments on increased apoptosis and reduced number of embryos reaching the blastocyte stage if antibodies to Hsp70 were present in culture medium for the development of bovine embryos in vitro.Citation22 High level of antibodies to Hsp70 in early pregnancy correlated with increased birth defects.Citation23 There are numerous reports on Hsp70 expression on the surface of cancer cells. Hsp70 surface expression may be regarded as another feature shared by ESC and cancer cells. However, tumor cells express on their surface inducible rather than constitutive Hsp70 isoform.Citation24
Expression of heat-shock proteins is induced by stressful stimuli. A major stressful factor that regulates expression of HSP70 family proteins is hyperthermia. Cell response on elevated temperature is a function of temperature and time. It is commonly accepted that the level of Hsp70 is generally correlates with cell resistance to hyperthermia. There a few data on hESC sensitivity to heat-shock. Cell viability measured by MTT test dropped only by about 40% after prolonged (4 h) hESC heating at 43°C.Citation25 In other words, hESC are rather heat-resistant. In our experiments, hESC heated at 45°C for 10 min remained viable (mild heat shock); the cell exposure to the same temperature but for 30 min reduced ESC viability by 30–35% (severe heat shock) due to apoptosis. Neither apoptosis nor a decline in viability measured by the cell membrane integrity was observed in the differentiated derivates under severe heat shock. hESC counterparts used in this work are fibroblast-like cells sharing the major properties with normal human fibroblasts described in literature. Human fibroblasts under normal culture can divide a limited number of times (Hayflick limit) and then enter a state of replicative senescence (RS). hESC differentiated fibroblasts-like cells are diploid and underwent RS during long-term cultivation. Hallmarks of senescence include a large flat morphology, senescence-associated β-galactosidase (SA-β-gal) staining, cellular over-activation and hyper-function, feedback signal resistance and loss of replication potential.Citation26 Growth arrest may by evoked in human fibroblasts just within a few days, the phenomenon known as stress-induced premature senescence (SIPS). Different stress factors are able to elicit SIPS: ethanol, ionizing radiations, H2O2.Citation27 Higher doses of these stressors may induce apoptosis. Major SIPS biomarkers are growth arrest, altered cell morphology, SA β-gal activity and modified gene expression. In our experiments hESC differentiated cells after the heat stress exhibited SIPS phenotype. Heat shock blocked their cell cycle progression, and in 3 d after the heat shock, most cells were Ki-67-negative, whereas the number of enlarged, flattened and SA-β-gal-positive cells in culture increased to 60%. Thus, equal sublethal heat-shock conditions induced apoptosis in hESC and premature senescence phenotype of their fibroblast-like progeny. Although heat shock triggered apoptosis in various cells, we did not find in literature any records on heat-induced premature cell senescence. Nevertheless, it is well documented that chaperons, Hsp70 in particular, are fundamentally involved in apoptosis and senescence processes.Citation28 Hsp70 induction is altered in human fibroblasts during their replicative senescence.Citation29 Its depletion may induce senescence in cancer cells but does not affect proliferation of untransformed cells.Citation30 Expression of Hsp70 was diminished in CHO-K1 mutant cells underwent rapid senescence by high NaCl.Citation31 In our experiments, heat shock, as in many other cells, drastically modified Hsp70 and slightly affected Hsc70 expression in both hESC and their differentiated derivates. Mild heat shock increased Hsp70 quantity in both cell types. However, under severe heat shock, Hsp70 content reduced in hESC but continued to increase in differentiated cells. Heat shock insignificantly increased Hsc70 content in both cell types; it induction was more evident in undifferentiated cells. At this moment it is difficult to say whether the differences in expression and induction of the heat shock proteins and various heat shock response of hESC and their differentiated derivates (apoptosis SIPS, respectively) are causally related.
Whatever are the mechanisms hESC employ for the defense from injury, the fact is that these cells are very vulnerable to insults. Thereby, the main concern is the fate of survived cells: whether their pluripotency is compromised or not. Many papers describe ESC response to damage, but only a few papers appeared quite recently are devoted to the stress survived ESC progeny. It was found that mouse ESC exposed to H2O2 underwent apoptosis. The cells survived sublethal H2O2 exposure displayed proliferation activity comparable with control cells and expressed pluripotent markers.Citation32 Similar results were reported for mouse ESC survived γ-ray exposure.Citation33 hESC after irradiation in doses known to induce apoptosis of these cells are able to generate teratomas and express pluripotent genes.Citation34,Citation35 In our experiments hESC exposed to sublethal heat shock easily underwent apoptosis, but survived cells rapidly restore their initial properties: morphology, high proliferation activity, pluripotent properties (expression of pluripotent markers, generation of embryoid bodies and differentiation into three germ layers). Differentiated fibroblast-like cells that survived heat shock also resumed proliferation and underwent RS simultaneously with their parental cells, i.e., restore initial cell properties. As fibroblasts are used as model system in studies of stem cells,Citation36 this observation may be important in light of increasing interest to stress protection mechanisms in adult stem cells.Citation37 It has recently be reported that mesenchymal stem cells isolated from bone marrow and periodontal ligament irradiated up to 20 Gy underwent premature senescence,Citation38 whereas both mouse and human ESC are very sensitive to irradiation, and the dose of less than 1 Gy increased the apoptosis frequency more than twice.Citation35 It seems highly probable that damaged plutipotent cells are destined to die by apoptosis. This is beneficial for embryogenesis, while SIPS of pluripotent cells will be a disaster for the embryo development. It was speculated that apoptosis and premature senescence are two main stress-activated outcomes for cells with irreparable damages.Citation39 How the challenged cells choose between these pathways is not clear. However, it seems highly probable that SIPS is the prohibited pathway for insulted pluripotent cells.
In conclusion, our results show that both hESC and their differentiated progeny are sensitive to hyperthermia, but their heat shock response is different. Under equal sublethal heat shock conditions, human hESC underwent apoptosis, whereas differentiated counterparts displayed the SIPS hallmarks: growth arrest, enlarged and flattened cell morphology and SA-β-gal activity. Heat shock survived hESC sustain the self-renewal and pluripotency (express pluripotent markers, able to form embryoid bodies and differentiate into three germ layers). These observations, together with literature data, demonstrate that genotoxic stress induces in hESC apoptosis but not premature senescence. hESC, like cancer cells, are immortal but unlike tumor cells, they have mechanisms to avoid induced senescence. Elucidation of these mechanisms is very important to understand how hESC and, maybe, pluritipotent cells of early embryos secure their genome integrity to prevent transmission of mutations and other damages to the cell progeny.
Materials and Methods
Cell culture
Human embryonic cell line (hESC) C910Citation40 was maintained in mTeSR1 medium (Stem Cell Technol) on Petri dishes covered with mitomycin-treated human endometrial cells as feeder layer and passaged mechanically. For heat-shock experiments, cells were grown on Matrigel matrix (BD Biosciences), which sustains their pluripotent potential, at least for several passages.
Differentiated progeny
hESC C910 cells were spontaneously differentiated through embryoid bodies (EB) formation. hESC colonies were cut in pieces and plated into non-adhesive plastic dishes in the growth medium composed of 80% knockout DMEM, 20% knockout serum replacement), 1% non-essential amino acids, 0.1 mM β-mercaptoethanol, antibiotics (ICN). Within 7–10 d cystic EB were formed. EB were transferred into tissue culture dishes and cultivated in DMEM/F12 medium with 10% fetal calf serum (Gibco). Under these conditions, EB differentiated into various cell types but prevailed fibroblast-like cells. Cells were subcultured once a week at the split ratio 1:3. After 2–3 passages, the culture was composed of morphologically homogenous fibroblast-like cells. Cytogenetical analysis was performed by the routine method. Briefly, cells were accumulated in metaphase with 0.01–0.02 μg/ml demecolcine, treated with 0.56% KCl as hypotonic solution and stained with Giemsa dye for G-banding. Metaphases were analyzed under the light microscope (Carl Zeiss).
Heat-shock conditions
hESCC910 cells and fibroblast-like cells were seeded in 3 cm Petri dishes and in subconfluent density were exposed to heat shock. Plates sealed with parafilm were heated at 45°C for 10 min (mild heat shock) or 30 min (severe heat shock) in a water bath and then were recovered at 37°C in CO2 incubator for various times.
Assessment of cell viability and apoptosis
Cell viability was estimated by annexin V/propidium iodide staining according to the routine procedure. Briefly, heated and unheated cells were harvested by trypsin/EDTA in suspension, incubated with annexin V-FITC (BD PharMingen) and 10 µg/ml propidium iodide for 10 min and analyzed on Coulter EPICS XL Flow Cytometer (Backman Coulter). Apoptosis was also quantities by nuclear fragmentation. Cells fixed with 4% formalin for 15 min were stained with 1 μg/ml DAPI for 20 min and washed. The number of cells showing nuclear fragmentation was determined microscopically. Not less than 500 randomly selected cells were counted. Data are means ± s.d. of three independent experiments.
Cell cycle analysis
Heated and unheated embryonic stem cells or their differentiated derivates were harvested with trypsin/EDTA and processed for the cell cycle analysis. Cell suspension was treated with 200 μg/ml saponin (Fluka) for 30 min at room temperature, then with 50 μg/ml PI and 250 μg/ml RNase A for 30 min at 37°C. Cell cycle analysis was performed with Coulter EPICS XL Flow Cytometer (Beckman Coulter) and WinList and ModFit LT software (Verity Software House).
Immunofluorescence and alkaline phosphatase assay
Cells grown on coverslips were fixed with 4% formalin in PBS for 15 min, permeabilized with 0.1% Triton X-100, incubated with 1% bovine serum albumin for 30 min to block non-specific binding, treated with primary antibodies for 45 min, washed with PBS/0.1% Tween-20, treated with secondary antibodies for 45 min, washed with PBS/0.1% Tween-20, counterstained with 1 μg/ml DAPI and mounted in media with 2% propyl gallate. The procedures were performed at room temperature. Localization of surface antigens was performed on living cells fixed after the binding with primary antibodies. Next steps were the same as for other antigens. Primary antibodies: mouse monoclonal to Hsp70,Citation24 Ki-67 (Abcam), oct4 (Santa Cruz), ssea-4 (Chemicon), ABCG2 (Abcam), muscle α-actinin (Sigma), vimentin (Chemicon), α-fetoprotein (Sigma), rabbit monoclonal to hsc70 (Abcam) and rabbit polyclonal β-tubulin ІІІ (Sigma). Secondary antibodies: DylightTM-488-conjugated goat anti-mouse (Jackson ImmunoResearch), Cy3-conjugated sheep anti-rabbit (Sigma) and Cy2-conjugated goat anti-rabbit (Jackson ImmunoResearch). Images have been obtained with laser-scanning confocal microscope Lieca TCS SP5.
Alkaline phosphatase expression was assayed with Leukocyte Alkaline Phosphatase Kit (Sigma) according to the manufacturer’s instructions under the light microscope.
Immunoblot analysis
Whole cell lysates prepared in the lysis buffer [50 mM TRIS-HCl, pH 7.4, 1% Triton X-100, 10% glycerol, 150 mM NaCl, 1 mM EDTA, 1 mM NaF, 1 mM Na3VO4, 1 mM PMSF and protease inhibitor cocktail (Sigma)] were mixed electrophoresis sample buffer. Equal amounts of protein were separated by SDS-PAGE electrophoresis and transferred to PVDF membranes (Millipore). Membranes were blocked, incubated with primary antibodies, washed and incubated with secondary antibodies. Primary antibodies: mouse monoclonal to Hsp70Citation24 (Lasunskaia et al., 1997) and rabbit monoclonal to hsc70 (Abcam). Secondary antibodies: horse-raddish-conjugated goat anti-rabbit (Cell Signaling) and horse-raddish-conjugated goat anti-mouse (Sigma). Proteins were visualized with SuperSignal chemiluminescence reagent kit (Pierce).
RT-PCR assay
To analyze gene expression, total RNA from differentiated and undifferentiated hESC C910 cells was isolated with RNesy Micro Kit (Qiagen) according to the manufacturer’s instructions. cDNA synthesis was performed with 1 μg of total RNA using RevertAid H Minus First Strand cDNA Synthesis Kit (Fermentas) according to the manufacturer’s instructions. Specific genes were amplified by Taq DNA polymerase (Fermentas) with Cyclo Temp amplificator. The program was as follows: hot start-denaturation at 93°C for 3 min, primer annealing at 51–70°C for 2 min, elongation at 72°C for one min 30 sec. Then, 25–27 cycles of denaturation at 93°C for 45 sec, primer annealing at 51–70°C for one min, elongation at 72°C for 1 min 30 sec, final elongation at 72°C for 10 min. Primers: hsp 70, sense 5′ atgcggccaagaaccaggtg 3′, antisense 5′ gcgctgcgagtcgttgaagt 3′, annealing temperature 61° (307 bp); hsc 70, sense 5′ atccccaagattcagaagct 3′, antisense 5′ ttgatgaggacagtcatga 3′, annealing at 63° (218 bp); caspase 3, sense 5′ agccatggtgaagaagga 3′, antisense 5′ cactgtctgtctcaatgc 3′, annealing temperature 51°C (171 bp) β-actin gene was used for RNA quantitative control and DNA contamination monitoring: sense primer 5′gccgagcgggaaatcgtgcgt 3′, antisense 5′ cggtggacgatggaggggccg 3′, annealing temperature 70°C (507 bp). The electrophoresis of amplified products was performed in 2% agarose gel with TAE buffer and ethidium bromide. 100 kb DNA ladder (Fermentas) was used as molecular weight markers. Amplified products were visualized in UV light (302 nm) with Transilluminator and registered with a digital Canon camera.
SA-β-gal activity
Cells expressing senescent-associated β-galactosidase were detected with senescence β-galactosidase staining kit (Cell Signaling Technology). Their number was quantitated by counting X-gal-positive cells among not less 500 cells.
Acknowledgments
The work was supported by Russian Foundation for Basic Research (project 11–04–12077) and Program of Russian Academy of Sciences “Molecular and Cellular Biology.” The authors are grateful to N. Aksenov, O.Lublinkaya and A. Shatrova for the advices in experiments on the cell cycle analysis and B. Margulis and I. Guzova for fruitful discussions and valuable comments.
Disclosure of Potential Conflicts of Interest
No potential conflicts of interest were disclosed.
References
- Hardy K. Apoptosis in the human embryo. Rev Reprod 1999; 4:125 - 34; http://dx.doi.org/10.1530/ror.0.0040125; PMID: 10521149
- Maitra A, Arking DE, Shivapurkar N, Ikeda M, Stastny V, Kassauei K, et al. Genomic alterations in cultured human embryonic stem cells. Nat Genet 2005; 37:1099 - 103; http://dx.doi.org/10.1038/ng1631; PMID: 16142235
- Stambrook PJ, Tichy ED. Preservation of genomic integrity in mouse embryonic stem cells. Adv Exp Med Biol 2010; 695:59 - 75; http://dx.doi.org/10.1007/978-1-4419-7037-4_5; PMID: 21222199
- Vinoth KJ, Heng BC, Poonepalli A, Banerjee B, Balakrishnan L, Lu K, et al. Human embryonic stem cells may display higher resistance to genotoxic stress as compared to primary explanted somatic cells. Stem Cells Dev 2008; 17:599 - 607; http://dx.doi.org/10.1089/scd.2007.0088; PMID: 18498216
- George S, Heng BC, Vinoth KJ, Kishen A, Cao T. Comparison of the response of human embryonic stem cells and their differentiated progenies to oxidative stress. Photomed Laser Surg 2009; 27:669 - 74; http://dx.doi.org/10.1089/pho.2008.2354; PMID: 19530910
- Grandela C, Pera MF, Grimmond SM, Kolle G, Wolvetang EJ. p53 is required for etoposide-induced apoptosis of human embryonic stem cells. Stem Cell Res (Amst) 2007; 1:116 - 28; http://dx.doi.org/10.1016/j.scr.2007.10.003; PMID: 19383392
- Velichko AK, Lagarkova MA, Philonenko ES, Kiselev SL, Kantidze OL, Razin SV. Sensitivity of human embryonic and induced pluripotent stem cells to a topoisomerase II poison etoposide. Cell Cycle 2011; 10:2035 - 7; http://dx.doi.org/10.4161/cc.10.12.16006; PMID: 21673500
- Luo LZ, Gopalakrishna-Pillai S, Nay SL, Park S-W, Bates SE, Zeng X, et al. DNA repair in human pluripotent stem cells is distinct from that in non-pluripotent human cells. PLoS ONE 2012; 7:e30541; http://dx.doi.org/10.1371/journal.pone.0030541; PMID: 22412831
- Filion TM, Qiao M, Ghule PN, Mandeville M, van Wijnen AJ, Stein JL, et al. Survival responses of human embryonic stem cells to DNA damage. J Cell Physiol 2009; 220:586 - 92; http://dx.doi.org/10.1002/jcp.21735; PMID: 19373864
- Maynard S, Swistowska AM, Lee JW, Liu Y, Liu ST, Da Cruz AB, et al. Human embryonic stem cells have enhanced repair of multiple forms of DNA damage. Stem Cells 2008; 26:2266 - 74; http://dx.doi.org/10.1634/stemcells.2007-1041; PMID: 18566332
- Saretzki G, Armstrong L, Leake A, Lako M, von Zglinicki T. Stress defense in murine embryonic stem cells is superior to that of various differentiated murine cells. Stem Cells 2004; 22:962 - 71; http://dx.doi.org/10.1634/stemcells.22-6-962; PMID: 15536187
- Saretzki G, Walter T, Atkinson S, Passos JF, Bareth B, Keith WN, et al. Downregulation of multiple stress defense mechanisms during differentiation of human embryonic stem cells. Stem Cells 2008; 26:455 - 64; http://dx.doi.org/10.1634/stemcells.2007-0628; PMID: 18055443
- Armstrong L, Tilgner K, Saretzki G, Atkinson SP, Stojkovic M, Moreno R, et al. Human induced pluripotent stem cell lines show stress defense mechanisms and mitochondrial regulation similar to those of human embryonic stem cells. Stem Cells 2010; 28:661 - 73; http://dx.doi.org/10.1002/stem.307; PMID: 20073085
- Beere HM. “The stress of dying”: the role of heat shock proteins in the regulation of apoptosis. J Cell Sci 2004; 117:2641 - 51; http://dx.doi.org/10.1242/jcs.01284; PMID: 15169835
- Son YS, Park JH, Kang YK, Park JS, Choi HS, Lim JY, et al. Heat shock 70-kDa protein 8 isoform 1 is expressed on the surface of human embryonic stem cells and downregulated upon differentiation. Stem Cells 2005; 23:1502 - 13; http://dx.doi.org/10.1634/stemcells.2004-0307; PMID: 16100000
- Qin H, Yu T, Qing T, Liu Y, Zhao Y, Cai J, et al. Regulation of apoptosis and differentiation by p53 in human embryonic stem cells. J Biol Chem 2007; 282:5842 - 52; http://dx.doi.org/10.1074/jbc.M610464200; PMID: 17179143
- Chuykin IA, Lianguzova MS, Pospelova TV, Pospelov VA. Activation of DNA damage response signaling in mouse embryonic stem cells. Cell Cycle 2008; 7:2922 - 8; http://dx.doi.org/10.4161/cc.7.18.6699; PMID: 18787397
- Tichy ED. Mechanisms maintaining genomic integrity in embryonic stem cells and induced pluripotent stem cells. Exp Biol Med (Maywood) 2011; 236:987 - 96; http://dx.doi.org/10.1258/ebm.2011.011107; PMID: 21768163
- Tomita M. Involvement of DNA-PK and ATM in radiation- and heat-induced DNA damage recognition and apoptotic cell death. J Radiat Res (Tokyo) 2010; 51:493 - 501; http://dx.doi.org/10.1269/jrr.10039; PMID: 20814172
- Prinsloo E, Setati MM, Longshaw VM, Blatch GL. Chaperoning stem cells: a role for heat shock proteins in the modulation of stem cell self-renewal and differentiation?. Bioessays 2009; 31:370 - 7; http://dx.doi.org/10.1002/bies.200800158; PMID: 19274656
- Park JA, Kim YE, Seok HJ, Park WY, Kwon HJ, Lee Y. Differentiation and upregulation of heat shock protein 70 induced by a subset of histone deacetylase inhibitors in mouse and human embryonic stem cells. BMB Rep 2011; 44:176 - 81; http://dx.doi.org/10.5483/BMBRep.2011.44.3.176; PMID: 21429295
- Matwee C, Kamaruddin M, Betts DH, Basrur PK, King WA. The effects of antibodies to heat shock protein 70 in fertilization and embryo development. Mol Hum Reprod 2001; 7:829 - 37; http://dx.doi.org/10.1093/molehr/7.9.829; PMID: 11517289
- Child DF, Hudson PR, Hunter-Lavin C, Mukhergee S, China S, Williams CP, et al. Birth defects and anti-heat shock protein 70 antibodies in early pregnancy. Cell Stress Chaperones 2006; 11:101 - 5; http://dx.doi.org/10.1379/CSC-130R1.1; PMID: 16572734
- Lasunskaia EB, Fridlianskaia I, Arnholdt AV, Kanashiro M, Guzhova I, Margulis B. Sub-lethal heat shock induces plasma membrane translocation of 70-kDa heat shock protein in viable, but not in apoptotic, U-937 leukaemia cells. APMIS 2010; 118:179 - 87; http://dx.doi.org/10.1111/j.1600-0463.2009.02576.x; PMID: 20132183
- Heng BC, Vinoth KJ, Lu K, Deng X, Ge Z, Bay BH, et al. Prolonged exposure of human embryonic stem cells to heat shock induces necrotic cell death. Biocell 2007; 31:405 - 10
- Blagosklonny MV. Cell cycle arrest is not yet senescence, which is not just cell cycle arrest: terminology for TOR-driven aging. Aging (Albany NY) 2012; 4:159 - 65; PMID: 22394614
- Toussaint O, Medrano EE, von Zglinicki T. Cellular and molecular mechanisms of stress-induced premature senescence (SIPS) of human diploid fibroblasts and melanocytes. Exp Gerontol 2000; 35:927 - 45; http://dx.doi.org/10.1016/S0531-5565(00)00180-7; PMID: 11121681
- Sõti C, Sreedhar AS, Csermely P. Apoptosis, necrosis and cellular senescence: chaperone occupancy as a potential switch. Aging Cell 2003; 2:39 - 45; http://dx.doi.org/10.1046/j.1474-9728.2003.00031.x; PMID: 12882333
- Gutsmann-Conrad A, Heydari AR, You S, Richardson A. The expression of heat shock protein 70 decreases with cellular senescence in vitro and in cells derived from young and old human subjects. Exp Cell Res 1998; 241:404 - 13; http://dx.doi.org/10.1006/excr.1998.4069; PMID: 9637782
- Sherman M. Major heat shock protein Hsp72 controls oncogene-induced senescence. Ann N Y Acad Sci 2010; 1197:152 - 7; http://dx.doi.org/10.1111/j.1749-6632.2010.05196.x; PMID: 20536844
- Dmitrieva NI, Chen HT, Nussenzweig A, Burg MB. Knockout of Ku86 accelerates cellular senescence induced by high NaCl. Aging (Albany NY) 2009; 1:245 - 53; PMID: 19946467
- Guo YL, Chakraborty S, Rajan SS, Wang R, Huang F. Effects of oxidative stress on mouse embryonic stem cell proliferation, apoptosis, senescence, and self-renewal. Stem Cells Dev 2010; 19:1321 - 31; http://dx.doi.org/10.1089/scd.2009.0313; PMID: 20092403
- Rebuzzini P, Pignalosa D, Mazzini G, Di Liberto R, Coppola A, Terranova N, et al. Mouse embryonic stem cells that survive γ-rays exposure maintain pluripotent differentiation potential and genome stability. J Cell Physiol 2012; 227:1242 - 9; http://dx.doi.org/10.1002/jcp.22908; PMID: 21732352
- Sokolov MV, Panyutin IV, Onyshchenko MI, Panyutin IG, Neumann RD. Expression of pluripotency-associated genes in the surviving fraction of cultured human embryonic stem cells is not significantly affected by ionizing radiation. Gene 2010; 455:8 - 15; http://dx.doi.org/10.1016/j.gene.2010.01.006; PMID: 20123005
- Wilson KD, Sun N, Huang M, Zhang WY, Lee AS, Li Z, et al. Effects of ionizing radiation on self-renewal and pluripotency of human embryonic stem cells. Cancer Res 2010; 70:5539 - 48; http://dx.doi.org/10.1158/0008-5472.CAN-09-4238; PMID: 20530673
- Cmielová J, Havelek R, Jiroutová A, Kohlerová R, Seifrtová M, Muthná D, et al. DNA damage caused by ionizing radiation in embryonic diploid fibroblasts WI-38 induces both apoptosis and senescence. Physiol Res 2011; 60:667 - 77; PMID: 21574764
- Tower J.. Stress and stem cells. WIREs Dev Biol 2012; http://dx.doi.org/10.1002/wdev.56
- Cmielova J, Havelek R, Soukup T, Jiroutová A, Visek B, Suchánek J, et al. Gamma radiation induces senescence in human adult mesenchymal stem cells from bone marrow and periodontal ligaments. Int J Radiat Biol 2012; 88:393 - 404; http://dx.doi.org/10.3109/09553002.2012.666001; PMID: 22348537
- Chen J, Goligorsky MS. Premature senescence of endothelial cells: Methusaleh’s dilemma. Am J Physiol Heart Circ Physiol 2006; 290:H1729 - 39; http://dx.doi.org/10.1152/ajpheart.01103.2005; PMID: 16603702
- Kozhukharova IV, Fridlianskaia II, Kovaleva ZV, Pugovkina NA, Alekseenko LL, Zenin VV, et al. Novel human embryonic stem cell lines C612 and C910. Tsitologiia 2009; 51:551 - 8; PMID: 19764646