Abstract
The E2F family of transcription factors contributes to oncogenesis through activation of multiple genes involved in cellular proliferation, a process that is opposed by the Retinoblastoma tumor suppressor protein (RB). RB also increases E2F1 stability by inhibiting its proteasome-mediated degradation, but the consequences of this post-translational regulation of E2F1 remain unknown. To better understand the mechanism of E2F stabilization and its physiological relevance, we examined the streamlined Rbf1-dE2F1 network in Drosophila. During embryonic development, Rbf1 is insulated from ubiquitin-mediated turnover by the COP9 signalosome, a multi-protein complex that modulates E3 ubiquitin ligase activity. Here, we report that the COP9 signalosome also protects the Cullin4-E3 ligase that is responsible for dE2F1 proteasome-mediated destruction. This dual role of the COP9 signalosome may serve to buffer E2F levels, enhancing its turnover via Cul4 protection and its stabilization through protection of Rbf1. We further show that Rbf1-mediated stabilization of dE2F1 and repression of dE2F1 cell cycle-target genes are distinct properties. Removal of an evolutionarily conserved Rbf1 C terminal degron disabled Rbf1 repression without affecting dE2F1 stabilization. This mutant form of Rbf1 also enhanced G1-to-S phase progression when expressed in Rbf1-containing S2 embryonic cells, suggesting that such mutations may generate gain-of-function properties relevant to cellular transformation. Consistent with this idea, several studies have identified mutations in the homologous C terminal domains of RB and p130 in human cancer.
Introduction
The RB/E2F regulatory nexus
The Retinoblastoma family of proteins consists of the RB, p107 and p130 members that control multiple processes associated with cellular proliferation, including cell cycle, differentiation, apoptosis and cellular biosynthetic potential (reviewed in ref. Citation1). Consistent with their regulatory governance of these processes, RB family members are frequently inactivated in human cancers.Citation2,Citation3 In some diseases, such as retinoblastoma and small cell lung carcinoma, mutations in the RB1 gene itself are potentially causative for disease. In other cancer types, deregulation is accomplished through altered function of upstream regulatory factors, including the cyclin-dependent kinases (cdk) and cyclin/cdk inhibitors, with effects encompassing all RB family members.Citation4 Together, these genetic changes are so pervasive as to be recognized as a hallmark of cancer.Citation5,Citation6
One important target for RB family members in gene regulation is the E2F family of transcription factors that like RB are tightly linked to growth control. In humans, at least eight different E2F species (E2F1–E2F8) have been identified, and are classified as either transcriptional activators (E2F1–3) or repressors (E2F4–8), based on their sequence homology and functional properties.Citation7,Citation8 In Drosophila, these pathways are streamlined with two RB family proteins, Rbf1 and Rbf2, contributing to regulation of two E2F proteins, dE2F1 and dE2F2.Citation9 During G0 and early G1 of cell cycle progression, RB family members directly bind to different sets of E2F factors,Citation10,Citation11 and at least for E2F1, RB association reverses regulatory polarity from activation to repression.Citation11-Citation16 Cyclin-cdk kinase mediated phosphorylation of RB in late G1 causes RB/E2F1 dissociation, allowing E2F to activate numerous proliferation genes that drive entry into S phase.Citation17-Citation21 In human cancer, increased E2F activity is frequently observed,Citation22-Citation25 and is associated with poor prognosis, particularly in melanoma and breast cancer,Citation26-Citation29 highlighting the importance of imposing regulatory curbs on E2F1 expression and activity.
The ubiquitin-proteasome system and RB/E2F regulation
In addition to limitation through cyclin/cdk-mediated phosphorylation, the RB/E2F axis is governed by the ubiquitin-proteasome system. Indeed, inappropriate RB turnover contributes to disease as demonstrated during cellular immortalization by viral proteins leading to enhanced RB ubiquitination.Citation30 Although RB levels often appear stable in actively proliferating cells,Citation31,Citation32 steady-state fluctuations have been correlated with phosphorylation changes during cellular stress,Citation33 suggesting that a negative correlation exists between RB levels and its activity in certain contexts. For example, in response to nocodazole blockade, U2OS osteosarcoma cells exhibit marked elevation of RB levels in the G2/M phase of the cell cycle, and upon release into early G1, RB destabilization reestablishes lower baseline steady-state levels (not shown). In seminal experiments linking RB to cell cycle control, microinjected RB induced cellular G1 arrest only when introduced during the window of time immediately after nocodazole release and not when injected in asynchronously proliferating cells,Citation10 suggesting that RB function is correlated with conditions in early G1 amenable to its diminishing steady-state levels. An inverse relationship between steady-state levels and repressor potency was also observed for the Drosophila Retinoblastoma family member Rbf1 wherein unstable Rbf1 proteins were potent for target gene repression while stable mutant proteins were impotent.Citation34 A tight, activity-instability linkage may ensure that RB repression programs remain dynamic and sensitive to growth conditions, such as previously suggested for dynamic p53 fluctuation in response to DNA damage.Citation35,Citation36 Similar to RB, both p107 and p130 exhibit differential expression during the cell cycle with p107 levels peaking in S phase and p130 levels highest in G0,Citation31,Citation33,Citation37 and thus, multiple mechanisms likely influence turnover of these different RB family members. Interestingly, cyclin/cdk kinase activity is correlated with changes in RB family member levels,Citation33,Citation37 suggesting that the cyclin/cdk and ubiquitin/proteasome regulatory arms crosstalk to govern both RB family activity and stability.
In previous studies of the Drosophila embryo, we observed that the Rbf1 and Rbf2 proteins associate with the COP9 signalosome,Citation38 a developmentally regulated complex that controls proteasome-mediated degradation of many proteins through interactions with SCF (SKP1/cullin/F-box) E3 ubiquitin ligase complexes.Citation39 CSN5, the catalytic core of COP9, contains a metalloprotease motif termed JAMM (Jab1/MPN domain-associated metalloisopeptidase) that removes Nedd8 from the cullin subunits.Citation40 As cullin neddylation activates E3 ligase activity, the COP9 signalosome thus serves to protect substrates from turnover. Indeed, both Rbf1 and Rbf2 are destabilized in the absence of COP9 function,Citation38 connecting the regulation of Rbf protein turnover to the ubiquitin-proteasome system. The involvement of a specific ubiquitin ligase remains unknown, although in mammals, RB and p130 turnover has been linked to MDM2Citation41,Citation42 and SCFSkp2 33, respectively. As with the RB family, E2F family members are degraded through ubiquitin-mediated turnover, both at defined points during the cell cycleCitation43 and in response to DNA damageCitation44,Citation45 with E2F1 subjected to ubiquitination via the S-phase-specific F-box protein SCFSkp2, and degradation in the S/G2 phases of the cell cycle.Citation46,Citation47 Other ubiquitin ligases including APC/CCitation43 and ROC-Cullin ligasesCitation48 likely contribute to E2F1 degradation in these contexts. In contrast, a protective role is suggested for the MDM2 ubiquitin ligase,Citation47 and consistently, p19ARF mediated inhibition of MDM2 encourages E2F1 turnover.Citation49,Citation50 Interestingly, a key determinant of E2F1 degradation turns out to be RB itself.Citation51-Citation54 RB can bind to a C terminal instability element in E2F1, and may stabilize E2F1 by occluding the cellular ubiquitination machinery.Citation55 In Drosophila, E2F1 destruction is mediated by the Cul4Cdt2 E3-Ubiquitin ligase,Citation56,Citation57 suggesting that both Rbf1 and COP9 may coordinately influence E2F1 stability. Herein, we show that dE2F1 levels are indeed influenced by Rbf1 and COP9, but through distinct mechanisms. While Rbf1 can stabilize dE2F1 through pocket-domain-dependent protein-protein interactions, the COP9 signalosome complex downregulates dE2F1 levels through modulation of the Cul4 E3 ligase. Rbf1 mediated dE2F1 stabilization and repression activity are separate properties as select mutant Rbf1 forms lacking dE2F1 repression capability retained their capacity to stabilize dE2F1. We further show that this class of repression-inactive Rbf1 mutants enhanced the rate of S-phase entry perhaps through their inappropriate stimulation of dE2F1 levels.
Results and Discussion
The COP9 signalosome regulates the Rbf1/E2F1 pathway
To test whether loss of COP9 function is associated with destabilized E2F1, endogenous dE2F1 steady-state levels were examined in S2 embryonic cells that were depleted of the largest subunit of the COP9 complex, CSN1, using dsRNA. This treatment strongly reduced levels of transfected flag-tagged RBF1 and endogenous RBF2, as expected, while E2F1 levels were increased significantly (). This result shows that the COP9 signalosome stabilizes Rbf proteins, as previously noted,Citation38 but instead of protecting E2F1, the COP9 complex contributes to its turnover. Similar results were obtained during CSN4 and CSN5 knockdowns (not shown), suggesting that the COP9 complex, rather than individual COP9 subunits, contribute to E2F modulation. Previous studies showed that the COP9 signalosome can stabilize cullin E3 ligase-containing SCF complexes,Citation58 and a Cul4-containing SCF complex contributes to dE2F1 ubiquitination in S2 cells.Citation56 Therefore, Cul4 levels were examined in Csn1 knockdown cells, ascertaining that Cul4 was indeed diminished during COP9 knockdown. Direct knockdown of Cul4 but not Cul5 also led to increased levels of E2F1, consistent with previous reports.Citation56 Thus, a pathway emerges wherein the Cul4 E3 ligase responsible for E2F1 turnover is stabilized by the COP9 signalosome (). Direct modulation of Cul4 and Cul5 levels had no discernable effect on Rbf levels, indicating that Rbf1 and E2F1 are ubiquitinated through distinct pathways. We conclude that COP9 signalosome is associated with opposing roles for Rbf1 and E2F1, contributing to Rbf1 stabilization but E2F1 destabilization.
Figure 1. Dual roles of the COP9 signalosome in regulation of the Rbf1-dE2F1 network. (A) The COP9 signalosome complex governs both Rbf and E2F1 stability. S2 cells were treated with indicated dsRNA and proteins were measured by western blot analyses. Endogenous dE2F1 levels were dramatically increased due to reduced Cul4 levels when Cul4 (lane 4 and 6) or its upstream regulator COP9 (CSN1 subunit, lane 3) were depleted. Flag-Rbf1 and endogenous Rbf2 levels were substantially decreased by the CSN1 knockdown, but were not affected by Cullin knockdowns. (B) COP9 is a dual-functional regulator of dE2F1 stability. First, COP9 plays a protective role on Rbf1, which in turn stabilizes dE2F1. Second, COP9 restrains dE2F1 level by stabilizing a Cul4-based E3 ligase, which targets dE2F1 for degradation.
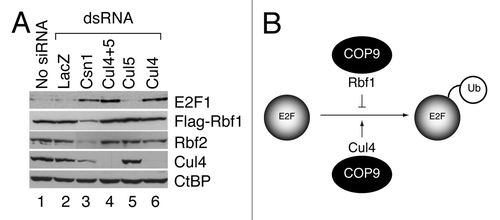
Drosophila RBF1 enhances dE2F1 levels
The data presented above highlights that a complicated network governs E2F stability with the COP9 signalosome contributing to low E2F1 levels during normal function. Previous studies have shown that in humans, RB can stabilize E2F1.Citation51,Citation52,Citation55 We therefore examined dE2F1 levels using Drosophila S2 cells that harbor wild-type COP9 function in the absence or presence of increased Rbf1 expression (). Three days post-transfection, steady-state protein levels of Myc-tagged dE2F1 were measured in the presence or absence of the MG132 proteasome inhibitor. Consistent with previous studies on mammalian E2F1, increased levels of Drosophila dE2F1 were observed during Rbf1 expression and at levels comparable to those observed with MG132 proteasome inhibition (). Under the conditions selected for this experiment, Rbf1 was relatively stable and its levels largely unaffected by MG132 treatment, although under different growth conditions, Rbf1 is proteasome sensitive.Citation34 These data indicate that dE2F1 is targeted by the ubiquitin-proteasome pathway and is responsive to the steady-state levels of Rbf1.
Figure 2. The Rbf1 pocket domain contributes to dE2F1 protection from proteasome-mediated degradation. (A) dE2F1 is sensitive to proteasome inhibition and is robustly stabilized by Rbf1 WT protein but not by forms of Rbf1 lacking the central pocket domain. Under these experimental conditions, Rbf1 WT and ΔPocket forms were expressed at equivalent levels and both are insensitive to proteasome inhibition. Endogenous tubulin levels are shown as loading controls. The experiment shown is representative of three biological replicates. (B) The Rbf1 pocket domain is insufficient for robust dE2F1 stabilization. The Rbf1 WT protein stabilizes dE2F1 protein, whereas at equivalent levels of expression, the Rbf1 pocket deletion mutant is incapable of stabilizing dE2F1 protein, while the Rbf1 pocket-only mutant provides only partial stabilization. Endogenous tubulin levels are shown as loading controls.
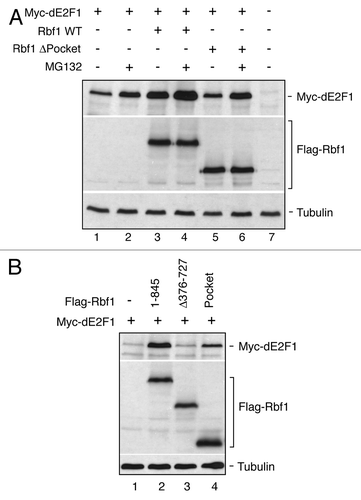
We next tested whether the direct binding of Rbf1 is required for dE2F1 stabilization. The conserved RB-family pocket domain is the primary site for E2F1 interaction,Citation59,Citation60 therefore, a deletion mutant of Rbf1 lacking this domain was tested. Unlike with wild-type Rbf1, dE2F1 levels were unaffected by this mutant form of RBF1, while retaining responsiveness to proteasome inhibition. The pocket domain alone was sufficient to confer at least partial stabilization on dE2F1, suggesting that this domain is necessary but not sufficient for complete stabilization (). These data are consistent with a model wherein dE2F1 is stabilized by direct contacts with Rbf1. Combined with our previous analysis of COP9 function, we conclude that the COP9 signalosome complex influences dE2F1 levels through two separate pathways, positively by stabilization of Rbf1, which binds dE2F1 to enhance cellular levels, and negatively by stabilizing the E3 ligase Cul4.
Our previous studies of Rbf1 indicated that an evolutionarily conserved C terminal instability element (IE) functions as an autonomous degron that stimulates both Rbf1 ubiquitination and repression potencyCitation34,Citation61. RB family proteins require multiple domains to mediate gene repression, including the pocket domain that facilitates E2F interaction and co-factor recruitment,Citation62-Citation65 and the C terminal region that harbors the IE,Citation34 and may provide additional dE2F1 contacts, as was shown for human RB and p107.Citation60 Interestingly, Rbf1 degron deletion mutants retained their capacity to physically associate with dE2F1 at target gene promoters.Citation34 To determine whether Rbf1 repression activity and dE2F1 stabilization are biochemically separable, we generated a series of Rbf1 deletion constructs that were tested for both properties (). Consistent with previous studies, transcription from the PCNA promoter was activated by dE2F1, but was repressed upon co-expression with wild-type Rbf1 (). Also consistent, wild-type Rbf1 (1–845) robustly stabilized dE2F1. Mutant forms of Rbf1 lacking the pocket domain (1–375 and Δ376–727) were inactive for both repression and dE2F1 stabilization, attesting to the importance of this domain for both these properties. Significantly, three different mutant forms of Rbf1 that lacked the IE entirely or had mutations in four key lysines were defective for repression, but continued to stabilize E2F1. Together, these data identify one class of Rbf1 mutations that disable repression without affecting dE2F1 stabilization, and another class that disables both repression and stabilization.
Figure 3. Mutant Rbf1 lacking IE function stabilizes dE2F1 but cannot fully repress its transcriptional activity. (A) Schematic representation of Rbf1 proteins used for functional testing show the relative positions of the pocket domain and the IE region. (B) Functional characterization of Rbf1. Mutations in the IE (Δ728–786 and 4K–A) compromise transcriptional repression activities of Rbf1 proteins measured on the PCNA-luciferase reporter gene (bar graph), but do not affect dE2F1 stabilization property (anti-Myc western blot). Under these transfection conditions, Rbf proteins were expressed at similar levels (anti-Flag western blot). Data represents at least three biological replicates.
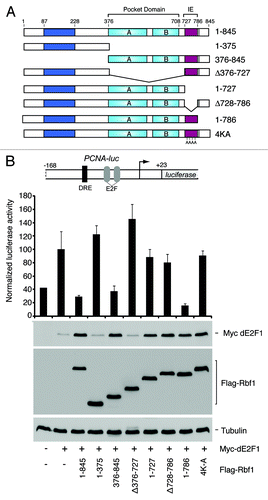
Rbf degron mutations enhance cellular S-phase entry
Previous studies have shown that elevated E2F levels are associated with increased cellular proliferation, and therefore, we hypothesized that enhanced dE2F1 stabilization enabled by repression-incompetent Rbf1 would facilitate ectopic S phase and contribute to deregulated cell growth. To test this possibility, GFP-Rbf1 WT and the Rbf1 ΔIE mutant were expressed in S2 cells and the effect on cell cycle progression was examined by FACS analysis (). Consistent with the established role of Rbf1 in G1-to-S phase transition, GFP-Rbf1 WT induced a strong G1 arrest in the transfected cells (GFP positive) that was not observed in untransfected cells (GFP negative) from the same culture (not shown), or in cells expressing the GFP-Rbf1 ΔIE mutant. The lack of G1 arrest by GFP-Rbf1 ΔIE is consistent with a parallel lack of dE2F1 repression potency associated with IE loss. Interestingly, GFP-Rbf1ΔIE-expressing cells also displayed a modest increase in their S-phase percentage, as estimated by Modfit analysis (, inset). Therefore, as a direct measure of the ability of this mutant form of Rbf1 to stimulate S-phase entry, we performed BrdU incorporation assays. In this assay, cells expressing wild-type or mutant Rbf1 were visualized by anti-Flag epitope immunofluorescence, and cells undergoing de novo DNA synthesis were identified by BrdU staining (). To assess the effect of transfected proteins on cell cycle, we calculated a proliferation index comparing the percentage of BrdU-positive cells in the transfected Rbf1-expressing population to the total population of cells. If a transfected protein exhibits no effect on cell cycle entry, the index should be equal to one, whereas the index should be less than one should the transfected protein cause cell cycle arrest. A protein that induces ectopic S phase entry should result in an index greater than one. As shown in , expression of Rbf1 lacking the pocket domain had no effect on DNA synthesis (P.I. = 1.03), consistent with observations that this protein is defective for repression and is unable to interact with and stabilize dE2F1. Therefore, in the context of S2 cells that express endogenous Rbf1, this defective Rbf1 protein had no effect. Cells expressing wild-type Rbf1 experienced substantial cell cycle arrest (P.I. = 0.63), suggesting that repression-competent Rbf1 downregulated key genes required for S phase entry. Importantly, expression of the repression-defective Rbf1ΔIE protein resulted in a substantial increase in the percentage of cells undergoing DNA replication (P.I. = 1.24), presumably through effects on dE2F1 stabilization. We conclude that expression of mutant Rbf1 harboring alterations to IE function confers a distinct growth advantage due to increased rate of S phase entry. In the model proposed in , dE2F1 activity increases during early G1 concomitant with increases in its steady-state levels mediated, in part, by either wild-type or mutant Rbf1. However, loss of Rbf1-mediated repression associated with IE mutation permits premature S phase entry, a process that would normally be delayed in the presence of wild-type Rbf1 until licensing by cyclin/cdk phosphorylation. Based on these observations, we hypothesized that mutations in the IE domain of human RB family members may be selected for in human cancers. Indeed, in one study of non-small lung carcinoma, a substantial percentage of patients (84%) were found to harbor mutations in the p130 IE region.Citation66 We previously showed that mutant Rbf1 harboring alanine substitutions of lysine residues within the IE diminish repressor potency but do not eliminate Rbf1-dE2F1 protein-protein interactions.Citation34 It is interesting to note that many of the p130 IE cancer-associated mutations were observed in these conserved basic residues. Together, these studies suggest an unexpected role for RB family turnover in cellular proliferation and cancer.
Figure 4. The IE region contributes to Rbf1-mediated G1 arrest. Wild-type or mutant GFP-Rbf1 ΔIE proteins were expressed in Drosophila S2 cells and the effect on cell cycle was determined by propidium iodide staining and FACS analyses. An overlay of the DNA content histograms for wild-type GFP-Rbf1 (gray) and mutant GFP-Rbf1 ΔIE-expressing cells (solid unfilled) shows that the loss of IE function is correlated with a diminished proportion of cells in the G1 phase. (Inset) Bar graph shows the ratios of total S-phase percentages for GFP positive vs. GFP negative populations for GFP-Rbf1 WT and GFP-Rbf1 ΔIE transfected samples. In two separate experiments, GFP-Rbf1 ΔIE expressing cells exhibited an increased proportion of cells in S phase, as estimated using Modfit analysis.
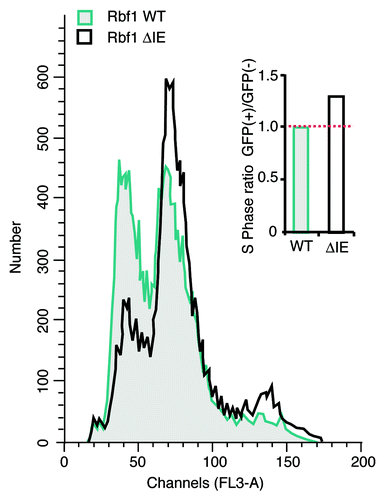
Figure 5. Mutation of the RB-family degron positively influences DNA replication frequency. (A) Rbf1 ∆IE enhances S-phase entry. S2 cells were transfected with the indicated Rbf1 proteins and the effect on DNA synthesis was monitored by BrdU incorporation. Transfected cells and BrdU-positive cells were visualized by immunofluorescent staining with anti-Flag and anti-BrdU antibodies. Arrows indicate representative cells that are transfected/BrdU negative (green) or transfected/BrdU positive (yellow). Rbf1∆IE expression was associated with increased BrdU positive staining compared with Rbf1 WT expressing cells. (B) The effect of Rbf1 proteins on DNA replication was indicated as a proliferation index calculated as a ratio of the percentage of BrdU-positive cells in transfected cells to that in the total population. The Rbf1∆Pocket mutant that is unable to both stabilize and repress dE2F1 also showed no effect on BrdU incorporation. Rbf1 WT expressing cells exhibited diminished BrdU incorporation consistent with increased G1 arrest, whereas Rbf1∆IE expressing cells exhibited enhanced S phase. Data from three biological replicates were analyzed. Error bars indicate standard deviation, and asterisks indicate p < 0.05. (C) A model for the regulation of E2F function and stability during cell cycle progression. In this model, E2F levels are stabilized during early G1 by both wild-type and mutant Rbf1 proteins. The COP9 signalosome contributes to enhanced dE2F1 steady-state levels during this stage by stabilization of Rbf1. However, dE2F1 activity is not restrained by mutant Rbf1 leading to premature S phase entry. After Rbf1-dE2F1 estrangement mediated by cyclin cdk phosphorylation, the COP9 signalosome contributes to dE2F1 destruction via protection of Cul4.
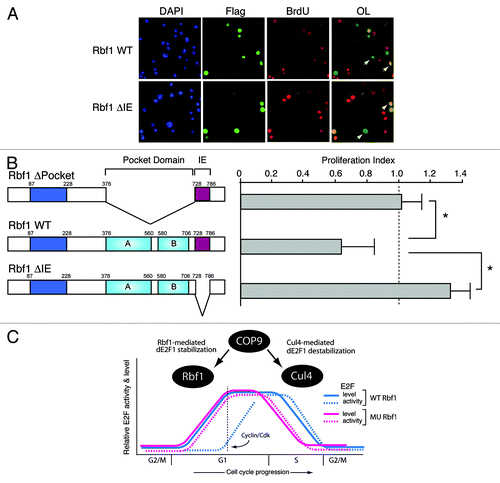
Materials and Methods
Expression constructs
Generation of Rbf1 WT and mutant expression constructs was described previously.Citation34 To generate GFP-Rbf1 fusion proteins, eGFP cDNA was amplified from phs-eGFP and inserted into KpnI site of pAX-Rbf1 WT and pAX-Rbf1 Δ728–786 vectors. The Myc-E2F1 expression construct was a gift from Dr. Maxim Frolov).
E2F1 stabilization assay
1.5 million Drosophila S2 cells were transfected with 0.2 µg of pAXRbf1 WT or mutants and 0.2 µg of pIE-E2F1 constructs using the Effectene transfection reagent (Qiagen) according to the manufacturer’s protocol. The cells were grown for 3 d after which protein levels were analyzed through western blotting. For proteasome inhibition in , the cells were treated with 50 µg/ml MG132 (Sigma-Aldrich) or the vehicle DMSO for 2 h.
Western blot analysis
To measure protein levels in Drosophila S2 cell culture, cells were harvested 3 d post-transfection and lysed by three freeze-thaw cycles in lysis buffer (50 mM TRIS-HCl pH8.0, 150 mM NaCl, 1% Triton X-100, protease inhibitors). Fifty µg of S2 cell lysates were run on 12.5% SDS-PAGE gels, transferred to a PVDF membrane, and probed with M2 anti-Flag (mouse monoclonal, 1:10,000, Sigma; F3165), anti-Myc (mouse monoclonal, 1:3000, Roche; 9E10), anti-tubulin (mouse monoclonal, 1:20,000, Iowa Hybridoma Bank), anti-Cul4 (1:1,000, a gift from Dr. Robert Duronio), anti-E2f1 (1:1,000, gift from Dr. Maki Asano), anti-Rbf2 (rabbit polyclonal, 1:5,000, R2C1) and anti-CtBP (rabbit polyclonal, 1:5,000, DNA208).
Luciferase reporter assay
Drosophila S2 cells were transfected using the Effectene transfection reagent (Qiagen) according to the manufacturer’s protocol. Typically, 1.5 million cells were transfected with 1 µg of PCNA-Luciferase reporter, 0.25 µg of pRL-CMV Renilla luciferase reporter (Promega), 200 ng of pIE-E2F1 and 200 ng of one of pAXRbf1 constructs. Cells were harvested 72 h after transfection and luciferase activity was measured using the Dual-Glo luciferase assay system (Promega) and quantified using the Veritas microplate luminometer (Turner Biosystems). Firefly luciferase activity was normalized to renilla luciferase activity.
Fluorescence-activated cell sorting and cell cycle analysis
To analyze the effects of Rbf1 proteins on Drosophila S2 cell cycle, FACS analyses were performed using cells expressing GFP-tagged Rbf1 proteins. One and a half million cells were transfected with 1 µg of pAXGFP-Rbf1 WT or pAXGFP-Rbf1 ΔIE constructs using the Effectene transfection reagent (Qiagen). Cells were harvested 4 d post-transfection and analyzed by flow cytometry to separate the GFP positive and GFP negative populations. Sorted cells were fixed with 70% ethanol and stained with propidium iodide (PI) for DNA content measurements using a BD Bioscience Vantage SE flow cytometer. The cell cycle data was analyzed through ModFit LT v3.3 (Verity Software House).
BrdU incorporation assay
Cell proliferation was assessed by examining bromodeoxyuridine (BrdU) incorporation 20 h after the addition of BrdU to the S2 cell cultures that were transiently transfected with the indicated pAXRbf1 expression constructs. Two million S2 cells were plated on polylysine coated glass coverslips and transfected with 400 ng pAX vector expressing Flag-tagged Rbf1 WT or mutants. Cells were incubated at 25°C for 1 d and then incubated in medium with 100 uM BrdU for 20 h. Cells were washed once with PBS and fixed in 4% paraformaldehyde for 15 min at room temperature. Cells were permeabilized with 0.4% Triton X-100 for 10 min and blocked with 1% BSA for 1 h. Cells were then incubated with rabbit polyclonal anti-Flag (1:250, Sigma-Aldrich; F7425) for 1 h. After two washes with TBST (20 mM Tris pH 7.5, 120 mM NaCl, 0.1% Tween 20), cells were incubated with Alexa488-cojugated chicken anti-rabbit (1:500). For detection of BrdU, cells were fixed again in 4% paraformaldehyde for 30 min, treated with 2 M HCl for 30 min and blocked with 1% BSA for 30 min. cells were then incubated with mouse monoclonal anti-BrdU (1:250, BD PharMingen) for 1 h. After two washes with TBST, cells were incubated with Alexa555-cojugated goat anti-mouse (1:500). Coverslips were mounted in Vectashield mounting medium containing 1.5 ug/ml DAPI.
RNA interference
Double-stranded RNAs were transcribed with MEGAscript T7 High Yield Transcription Kit (Ambion). S2 cells were maintained in Sf-900 II serum-free medium (GIBCO) supplemented with 0.5% Penicillin-Streptomycin. One and a half million cells were incubated with fresh medium containing 15 µg dsRNA for 30 min and then transfected with 200 ng pAX-Rbf1. Cells were grown for 5 d at 25°C.
Acknowledgments
We thank Nick Dyson for sharing the PCNA-luciferase reporter gene, Maxim Frolov for the pIE-E2F1 construct, Robert Duronio for the anti-Cul4 antibody, Maki Asano for the anti-E2F1 antibody, Louis King for expert assistance with flow cytometry and Satyaki Sengupta for his thoughtful comments. This work was supported by the MSU Gene Expression in Development and Disease Group, and a grant from the NIH to D.N.A and R.W.H (1R01GM079098).
References
- Henley SA, Dick FA. The retinoblastoma family of proteins and their regulatory functions in the mammalian cell division cycle. Cell Div 2012; 7:10; http://dx.doi.org/10.1186/1747-1028-7-10; PMID: 22417103
- Lohmann DR, Gallie BL. Retinoblastoma: revisiting the model prototype of inherited cancer. Am J Med Genet C Semin Med Genet 2004; 129C:23 - 8; http://dx.doi.org/10.1002/ajmg.c.30024; PMID: 15264269
- Lohmann D. Retinoblastoma. Adv Exp Med Biol 2010; 685:220 - 7; http://dx.doi.org/10.1007/978-1-4419-6448-9_21; PMID: 20687510
- Wikenheiser-Brokamp KA. Retinoblastoma regulatory pathway in lung cancer. Curr Mol Med 2006; 6:783 - 93; PMID: 17100603
- Hanahan D, Weinberg RA. The hallmarks of cancer. Cell 2000; 100:57 - 70; http://dx.doi.org/10.1016/S0092-8674(00)81683-9; PMID: 10647931
- Hanahan D, Weinberg RA. Hallmarks of cancer: the next generation. Cell 2011; 144:646 - 74; http://dx.doi.org/10.1016/j.cell.2011.02.013; PMID: 21376230
- van den Heuvel S, Dyson NJ. Conserved functions of the pRB and E2F families. Nat Rev Mol Cell Biol 2008; 9:713 - 24; http://dx.doi.org/10.1038/nrm2469; PMID: 18719710
- Dimova DK, Dyson NJ. The E2F transcriptional network: old acquaintances with new faces. Oncogene 2005; 24:2810 - 26; http://dx.doi.org/10.1038/sj.onc.1208612; PMID: 15838517
- Stevaux O, Dimova D, Frolov MV, Taylor-Harding B, Morris E, Dyson N. Distinct mechanisms of E2F regulation by Drosophila RBF1 and RBF2. EMBO J 2002; 21:4927 - 37; http://dx.doi.org/10.1093/emboj/cdf501; PMID: 12234932
- Goodrich DW, Wang NP, Qian YW, Lee EY, Lee WH. The retinoblastoma gene product regulates progression through the G1 phase of the cell cycle. Cell 1991; 67:293 - 302; http://dx.doi.org/10.1016/0092-8674(91)90181-W; PMID: 1655277
- Takahashi Y, Rayman JB, Dynlacht BD. Analysis of promoter binding by the E2F and pRB families in vivo: distinct E2F proteins mediate activation and repression. Genes Dev 2000; 14:804 - 16; PMID: 10766737
- Flemington EK, Speck SH, Kaelin WG Jr.. E2F-1-mediated transactivation is inhibited by complex formation with the retinoblastoma susceptibility gene product. Proc Natl Acad Sci USA 1993; 90:6914 - 8; http://dx.doi.org/10.1073/pnas.90.15.6914; PMID: 8346196
- Helin K, Harlow E, Fattaey A. Inhibition of E2F-1 transactivation by direct binding of the retinoblastoma protein. Mol Cell Biol 1993; 13:6501 - 8; PMID: 8413249
- Hiebert SW, Chellappan SP, Horowitz JM, Nevins JR. The interaction of RB with E2F coincides with an inhibition of the transcriptional activity of E2F. Genes Dev 1992; 6:177 - 85; http://dx.doi.org/10.1101/gad.6.2.177; PMID: 1531329
- Weintraub SJ, Prater CA, Dean DC. Retinoblastoma protein switches the E2F site from positive to negative element. Nature 1992; 358:259 - 61; http://dx.doi.org/10.1038/358259a0; PMID: 1321348
- Weintraub SJ, Chow KN, Luo RX, Zhang SH, He S, Dean DC. Mechanism of active transcriptional repression by the retinoblastoma protein. Nature 1995; 375:812 - 5; http://dx.doi.org/10.1038/375812a0; PMID: 7596417
- Nevins JR, Chellappan SP, Mudryj M, Hiebert S, Devoto S, Horowitz J, et al. E2F transcription factor is a target for the RB protein and the cyclin A protein. Cold Spring Harb Symp Quant Biol 1991; 56:157 - 62; http://dx.doi.org/10.1101/SQB.1991.056.01.020; PMID: 1840249
- Hinds PW, Mittnacht S, Dulic V, Arnold A, Reed SI, Weinberg RA. Regulation of retinoblastoma protein functions by ectopic expression of human cyclins. Cell 1992; 70:993 - 1006; http://dx.doi.org/10.1016/0092-8674(92)90249-C; PMID: 1388095
- Ewen ME, Sluss HK, Sherr CJ, Matsushime H, Kato J, Livingston DM. Functional interactions of the retinoblastoma protein with mammalian D-type cyclins. Cell 1993; 73:487 - 97; http://dx.doi.org/10.1016/0092-8674(93)90136-E; PMID: 8343202
- Kato J, Matsushime H, Hiebert SW, Ewen ME, Sherr CJ. Direct binding of cyclin D to the retinoblastoma gene product (pRb) and pRb phosphorylation by the cyclin D-dependent kinase CDK4. Genes Dev 1993; 7:331 - 42; http://dx.doi.org/10.1101/gad.7.3.331; PMID: 8449399
- Dynlacht BD, Flores O, Lees JA, Harlow E. Differential regulation of E2F transactivation by cyclin/cdk2 complexes. Genes Dev 1994; 8:1772 - 86; http://dx.doi.org/10.1101/gad.8.15.1772; PMID: 7958856
- Chen HZ, Tsai SY, Leone G. Emerging roles of E2Fs in cancer: an exit from cell cycle control. Nat Rev Cancer 2009; 9:785 - 97; http://dx.doi.org/10.1038/nrc2696; PMID: 19851314
- Eymin B, Karayan L, Séité P, Brambilla C, Brambilla E, Larsen CJ, et al. Human ARF binds E2F1 and inhibits its transcriptional activity. Oncogene 2001; 20:1033 - 41; http://dx.doi.org/10.1038/sj.onc.1204220; PMID: 11314038
- Imai MA, Oda Y, Oda M, Nakanishi I, Kawahara E. Overexpression of E2F1 associated with LOH at RB locus and hyperphosphorylation of RB in non-small cell lung carcinoma. J Cancer Res Clin Oncol 2004; 130:320 - 6; http://dx.doi.org/10.1007/s00432-003-0538-3; PMID: 14997382
- Saberwal G, Lucas S, Janssen I, Deobhakta A, Hu WY, Galili N, et al. Increased levels and activity of E2F1 transcription factor in myelodysplastic bone marrow. Int J Hematol 2004; 80:146 - 54; http://dx.doi.org/10.1532/IJH97.NA0303; PMID: 15481443
- Alla V, Engelmann D, Niemetz A, Pahnke J, Schmidt A, Kunz M, et al. E2F1 in melanoma progression and metastasis. J Natl Cancer Inst 2010; 102:127 - 33; http://dx.doi.org/10.1093/jnci/djp458; PMID: 20026813
- Baldini E, Camerini A, Sgambato A, Prochilo T, Capodanno A, Pasqualetti F, et al. Cyclin A and E2F1 overexpression correlate with reduced disease-free survival in node-negative breast cancer patients. Anticancer Res 2006; 26:6B 4415 - 21; PMID: 17201163
- Hallett RM, Hassell JA. E2F1 and KIAA0191 expression predicts breast cancer patient survival. BMC Res Notes 2011; 4:95; http://dx.doi.org/10.1186/1756-0500-4-95; PMID: 21453498
- Vuaroqueaux V, Urban P, Labuhn M, Delorenzi M, Wirapati P, Benz CC, et al. Low E2F1 transcript levels are a strong determinant of favorable breast cancer outcome. Breast Cancer Res 2007; 9:R33; http://dx.doi.org/10.1186/bcr1681; PMID: 17535433
- Boyer SN, Wazer DE, Band V. E7 protein of human papilloma virus-16 induces degradation of retinoblastoma protein through the ubiquitin-proteasome pathway. Cancer Res 1996; 56:4620 - 4; PMID: 8840974
- Classon M, Harlow E. The retinoblastoma tumour suppressor in development and cancer. Nat Rev Cancer 2002; 2:910 - 7; http://dx.doi.org/10.1038/nrc950; PMID: 12459729
- Haberichter T, Mädge B, Christopher RA, Yoshioka N, Dhiman A, Miller R, et al. A systems biology dynamical model of mammalian G1 cell cycle progression. Mol Syst Biol 2007; 3:84; http://dx.doi.org/10.1038/msb4100126; PMID: 17299420
- Tedesco D, Lukas J, Reed SI. The pRb-related protein p130 is regulated by phosphorylation-dependent proteolysis via the protein-ubiquitin ligase SCF(Skp2). Genes Dev 2002; 16:2946 - 57; http://dx.doi.org/10.1101/gad.1011202; PMID: 12435635
- Acharya P, Raj N, Buckley MS, Zhang L, Duperon S, Williams G, et al. Paradoxical instability-activity relationship defines a novel regulatory pathway for retinoblastoma proteins. Mol Biol Cell 2010; 21:3890 - 901; http://dx.doi.org/10.1091/mbc.E10-06-0520; PMID: 20861300
- Batchelor E, Loewer A, Mock C, Lahav G. Stimulus-dependent dynamics of p53 in single cells. Mol Syst Biol 2011; 7:488; http://dx.doi.org/10.1038/msb.2011.20; PMID: 21556066
- Batchelor E, Mock CS, Bhan I, Loewer A, Lahav G. Recurrent initiation: a mechanism for triggering p53 pulses in response to DNA damage. Mol Cell 2008; 30:277 - 89; http://dx.doi.org/10.1016/j.molcel.2008.03.016; PMID: 18471974
- Beijersbergen RL, Carlée L, Kerkhoven RM, Bernards R. Regulation of the retinoblastoma protein-related p107 by G1 cyclin complexes. Genes Dev 1995; 9:1340 - 53; http://dx.doi.org/10.1101/gad.9.11.1340; PMID: 7797074
- Ullah Z, Buckley MS, Arnosti DN, Henry RW. Retinoblastoma protein regulation by the COP9 signalosome. Mol Biol Cell 2007; 18:1179 - 86; http://dx.doi.org/10.1091/mbc.E06-09-0790; PMID: 17251548
- Wei N, Serino G, Deng XW. The COP9 signalosome: more than a protease. Trends Biochem Sci 2008; 33:592 - 600; http://dx.doi.org/10.1016/j.tibs.2008.09.004; PMID: 18926707
- Cope GA, Suh GS, Aravind L, Schwarz SE, Zipursky SL, Koonin EV, et al. Role of predicted metalloprotease motif of Jab1/Csn5 in cleavage of Nedd8 from Cul1. Science 2002; 298:608 - 11; http://dx.doi.org/10.1126/science.1075901; PMID: 12183637
- Xiao ZX, Chen J, Levine AJ, Modjtahedi N, Xing J, Sellers WR, et al. Interaction between the retinoblastoma protein and the oncoprotein MDM2. Nature 1995; 375:694 - 8; http://dx.doi.org/10.1038/375694a0; PMID: 7791904
- Sdek P, Ying H, Chang DL, Qiu W, Zheng H, Touitou R, et al. MDM2 promotes proteasome-dependent ubiquitin-independent degradation of retinoblastoma protein. Mol Cell 2005; 20:699 - 708; http://dx.doi.org/10.1016/j.molcel.2005.10.017; PMID: 16337594
- Peart MJ, Poyurovsky MV, Kass EM, Urist M, Verschuren EW, Summers MK, et al. APC/C(Cdc20) targets E2F1 for degradation in prometaphase. Cell Cycle 2010; 9:3956 - 64; http://dx.doi.org/10.4161/cc.9.19.13162; PMID: 20948288
- Höfferer M, Wirbelauer C, Humar B, Krek W. Increased levels of E2F-1-dependent DNA binding activity after UV- or gamma-irradiation. Nucleic Acids Res 1999; 27:491 - 5; http://dx.doi.org/10.1093/nar/27.2.491; PMID: 9862970
- Blattner C, Sparks A, Lane D. Transcription factor E2F-1 is upregulated in response to DNA damage in a manner analogous to that of p53. Mol Cell Biol 1999; 19:3704 - 13; PMID: 10207094
- Marti A, Wirbelauer C, Scheffner M, Krek W. Interaction between ubiquitin-protein ligase SCFSKP2 and E2F-1 underlies the regulation of E2F-1 degradation. Nat Cell Biol 1999; 1:14 - 9; http://dx.doi.org/10.1038/8984; PMID: 10559858
- Zhang Z, Wang H, Li M, Rayburn ER, Agrawal S, Zhang R. Stabilization of E2F1 protein by MDM2 through the E2F1 ubiquitination pathway. Oncogene 2005; 24:7238 - 47; http://dx.doi.org/10.1038/sj.onc.1208814; PMID: 16170383
- Ohta T, Xiong Y. Phosphorylation- and Skp1-independent in vitro ubiquitination of E2F1 by multiple ROC-cullin ligases. Cancer Res 2001; 61:1347 - 53; PMID: 11245432
- Martelli F, Hamilton T, Silver DP, Sharpless NE, Bardeesy N, Rokas M, et al. p19ARF targets certain E2F species for degradation. Proc Natl Acad Sci USA 2001; 98:4455 - 60; http://dx.doi.org/10.1073/pnas.081061398; PMID: 11274364
- Chang DL, Qiu W, Ying H, Zhang Y, Chen CY, Xiao ZX. ARF promotes accumulation of retinoblastoma protein through inhibition of MDM2. Oncogene 2007; 26:4627 - 34; http://dx.doi.org/10.1038/sj.onc.1210254; PMID: 17297463
- Hateboer G, Kerkhoven RM, Shvarts A, Bernards R, Beijersbergen RL. Degradation of E2F by the ubiquitin-proteasome pathway: regulation by retinoblastoma family proteins and adenovirus transforming proteins. Genes Dev 1996; 10:2960 - 70; http://dx.doi.org/10.1101/gad.10.23.2960; PMID: 8956997
- Hofmann F, Martelli F, Livingston DM, Wang Z. The retinoblastoma gene product protects E2F-1 from degradation by the ubiquitin-proteasome pathway. Genes Dev 1996; 10:2949 - 59; http://dx.doi.org/10.1101/gad.10.23.2949; PMID: 8956996
- Ikeda MA, Jakoi L, Nevins JR. A unique role for the Rb protein in controlling E2F accumulation during cell growth and differentiation. Proc Natl Acad Sci USA 1996; 93:3215 - 20; http://dx.doi.org/10.1073/pnas.93.8.3215; PMID: 8622916
- Martelli F, Livingston DM. Regulation of endogenous E2F1 stability by the retinoblastoma family proteins. Proc Natl Acad Sci USA 1999; 96:2858 - 63; http://dx.doi.org/10.1073/pnas.96.6.2858; PMID: 10077601
- Campanero MR, Flemington EK. Regulation of E2F through ubiquitin-proteasome-dependent degradation: stabilization by the pRB tumor suppressor protein. Proc Natl Acad Sci USA 1997; 94:2221 - 6; http://dx.doi.org/10.1073/pnas.94.6.2221; PMID: 9122175
- Shibutani ST, de la Cruz AF, Tran V, Turbyfill WJ 3rd, Reis T, Edgar BA, et al. Intrinsic negative cell cycle regulation provided by PIP box- and Cul4Cdt2-mediated destruction of E2f1 during S phase. Dev Cell 2008; 15:890 - 900; http://dx.doi.org/10.1016/j.devcel.2008.10.003; PMID: 19081076
- Zielke N, Kim KJ, Tran V, Shibutani ST, Bravo MJ, Nagarajan S, et al. Control of Drosophila endocycles by E2F and CRL4(CDT2). Nature 2011; 480:123 - 7; http://dx.doi.org/10.1038/nature10579; PMID: 22037307
- Wu JT, Lin HC, Hu YC, Chien CT. Neddylation and deneddylation regulate Cul1 and Cul3 protein accumulation. Nat Cell Biol 2005; 7:1014 - 20; http://dx.doi.org/10.1038/ncb1301; PMID: 16127432
- Lee C, Chang JH, Lee HS, Cho Y. Structural basis for the recognition of the E2F transactivation domain by the retinoblastoma tumor suppressor. Genes Dev 2002; 16:3199 - 212; http://dx.doi.org/10.1101/gad.1046102; PMID: 12502741
- Rubin SM, Gall AL, Zheng N, Pavletich NP. Structure of the Rb C-terminal domain bound to E2F1-DP1: a mechanism for phosphorylation-induced E2F release. Cell 2005; 123:1093 - 106; http://dx.doi.org/10.1016/j.cell.2005.09.044; PMID: 16360038
- Raj N, Zhang L, Wei Y, Arnosti DN, Henry RW. Ubiquitination of Retinoblastoma family protein 1 potentiates gene-specific repression function. J Biol Chem 2012; Submitted
- Luo RX, Postigo AA, Dean DC. Rb interacts with histone deacetylase to repress transcription. Cell 1998; 92:463 - 73; http://dx.doi.org/10.1016/S0092-8674(00)80940-X; PMID: 9491888
- Magnaghi-Jaulin L, Groisman R, Naguibneva I, Robin P, Lorain S, Le Villain JP, et al. Retinoblastoma protein represses transcription by recruiting a histone deacetylase. Nature 1998; 391:601 - 5; http://dx.doi.org/10.1038/35410; PMID: 9468140
- Ferreira R, Magnaghi-Jaulin L, Robin P, Harel-Bellan A, Trouche D. The three members of the pocket proteins family share the ability to repress E2F activity through recruitment of a histone deacetylase. Proc Natl Acad Sci USA 1998; 95:10493 - 8; http://dx.doi.org/10.1073/pnas.95.18.10493; PMID: 9724731
- Chan HM, Smith L, La Thangue NB. Role of LXCXE motif-dependent interactions in the activity of the retinoblastoma protein. Oncogene 2001; 20:6152 - 63; http://dx.doi.org/10.1038/sj.onc.1204793; PMID: 11593423
- Claudio PP, Howard CM, Pacilio C, Cinti C, Romano G, Minimo C, et al. Mutations in the retinoblastoma-related gene RB2/p130 in lung tumors and suppression of tumor growth in vivo by retrovirus-mediated gene transfer. Cancer Res 2000; 60:372 - 82; PMID: 10667590