Abstract
Mutations in the BRCA1 tumor suppressor gene are commonly found in hereditary ovarian cancers. Here, we used a co-culture approach to study the metabolic effects of BRCA1-null ovarian cancer cells on adjacent tumor-associated stromal fibroblasts. Our results directly show that BRCA1-null ovarian cancer cells produce large amounts of hydrogen peroxide, which can be abolished either by administration of simple antioxidants (N-acetyl-cysteine; NAC) or by replacement of the BRCA1 gene. Thus, the BRCA1 gene normally suppresses tumor growth by functioning as an antioxidant. Importantly, hydrogen peroxide produced by BRCA1-null ovarian cancer cells induces oxidative stress and catabolic processes in adjacent stromal fibroblasts, such as autophagy, mitophagy and glycolysis, via stromal NFκB activation. Catabolism in stromal fibroblasts was also accompanied by the upregulation of MCT4 and a loss of Cav-1 expression, which are established markers of a lethal tumor microenvironment. In summary, loss of the BRCA1 tumor suppressor gene induces hydrogen peroxide production, which then leads to metabolic reprogramming of the tumor stroma, driving stromal-epithelial metabolic coupling. Our results suggest that new cancer prevention trials with antioxidants are clearly warranted in patients that harbor hereditary/familial BRCA1 mutations.
Introduction
Epidemiology of ovarian cancer: Pathogenic role of the BRCA1 gene
Ovarian cancer is the fifth leading cause of cancer death in American women.Citation1 Ovarian cancer remains incurable in the majority of cases, and although novel therapies are life-prolonging, the 5 y overall survival rate has only increased from 36% in the 1970s to 44% in the last decade in the United States.Citation1 A family history of ovarian and/or breast cancer associated with mutations in the BRCA1 and BRCA2 genes is the strongest risk factor for ovarian cancer.Citation2 Hereditary ovarian cancer is most commonly transmitted in an autosomal dominant fashion due to inheritance of a mutation in the BRCA1 gene.Citation3 Carriers of a BRCA1 mutation subsequently have somatic inactivation of the wild-type BRCA1 allele, which drives the development of ovarian cancer and conforms to Knudson’s two-hit model for a tumor suppressor.Citation4 Mutations in the BRCA1 and BRCA2 genes are the greatest risk factors for ovarian cancer, increasing the lifetime risk to 30–50%, which is 10–30 times higher as compared with the general population.Citation5,Citation6
The breast cancer type 1 susceptibility protein (BRCA1) is a well-recognized tumor suppressor gene.Citation7 Among the best characterized BRCA1 function(s) are DNA repair by homologous recombination, cell cycle regulation, ubiquitination, transcription regulation and chromatin remodeling.Citation8 BRCA1-mutated ovarian cancers are more frequently poorly differentiated papillary serous adenocarcinomas, which are diagnosed at an advanced stage and are associated with p53 mutations.Citation9-Citation12 BRCA1 mutation status can predict sensitivity to therapy. Ovarian cancers with BRCA1 mutations are more frequently resistant to taxane chemotherapy, but are more sensitive to platinum-based chemotherapy.Citation13-Citation15
In summary, BRCA1 mutations are commonly found in ovarian cancers and are the most common cause of hereditary breast cancer. They are usually aggressive cancers that are resistant to taxanes but sensitive to platinum-based chemotherapy regimens.
Most pathogenic BRCA1 mutations block BRCA1 protein production by functioning as truncating mutations or are mis-sense mutations that interfere with critical regions of the gene, such as the RING finger motif or BRCT region of the gene.Citation16 The majority of patients with BRCA1-mutated breast cancers have loss of BRCA1 nuclear protein expression.Citation17 However, the IHC expression pattern of the BRCA1 protein in hereditary ovarian cancers is unknown. The majority of high-grade ovarian cancers have low BRCA1 protein nuclear expression, while the majority of normal ovarian tissue has high BRCA1 expression.Citation18,Citation19 It is recognized that hereditary ovarian cancer associated with BRCA mutations and sporadic cancers share a phenotype, with an impairment in homologous recombination being frequently found.Citation20,Citation21 In one study, 82% of ovarian cancers had mutations, absent mRNA or loss of heterozygosity for BRCA1 or BRCA2,Citation22 which may explain frequent anomalies in DNA damage repair.
Oxidative stress and BRCA1 mutations in cancer
Little is known about oxidative stress in ovarian cancer. However, there is an increase in metabolites associated with oxidative stress in human ovarian cancer samples as compared with control ovarian tissue.Citation23 8-hydroxydeoxyguanosine (8OHdG), which is a marker of oxidative stress-induced DNA damage, correlates with poor outcomes in ovarian cancers.Citation24
BRCA1 downregulation via siRNA induces oxidative stress, with increased generation of superoxide anion and hydrogen peroxide, in human breast cancer MCF-7 cells.Citation25 It is hypothesized that proliferation in the breast and ovary, during each menstrual cycle, induces oxidative stress and DNA damage, which would require BRCA1-mediated DNA repair, and this would explain why BRCA1 mutations predispose to breast and ovarian cancer predominantly.Citation6 BRCA wild-type overexpression in MCF-7 cells, T47D and DU-145 increases the activity of the antioxidant NRF-2 pathway, increases mRNA expression of antioxidant proteins, such as glutathione S-transferases, and cells with overexpression of wild-type BRCA1 show greater cell viability upon exposure to hydrogen peroxide; the opposite was seen with BRCA1 deficiency, with lower cell viability.Citation26 BRCA1 wild-type overexpression in these same cell types decreases levels of reactive oxygen species (ROS), as measured by DCFDA fluorescence.Citation27 Lymphoblasts of women with BRCA1 mutations have increased oxidative DNA damage after ionizing radiation-induced DNA damage.Citation28 Subsequently, it has been shown that leukocytes of women with BRCA1 mutations also have increased oxidative DNA damage at baseline.Citation29 No studies, to our knowledge, have evaluated the effects of antioxidant substances on apoptosis rates in BRCA-mutated cancer cells.
Stromal-epithelial metabolic coupling and tumor metabolism
Stromal-epithelial metabolic coupling has been found in several types of cancer.Citation30-Citation35 Caveolin 1, FABP4 and MCT4 are markers in stromal cells of metabolic coupling, and their expression has prognostic significance.Citation32,Citation36-Citation39 It is well-known that cancer cells, and ovarian cancer cells in particular, generate high levels of reactive oxygen species.Citation40-Citation44 Cancer cells have been shown to regulate stromal levels of reactive oxygen species.Citation45-Citation49
Ovaries resected because of the high risk of development of ovarian cancer, due to BRCA1 and BRCA2 mutations, have been compared histologically to control ovaries removed for reasons unrelated to cancer risk in several studies. In one study, BRCA1-mutated ovaries more frequently had cortical stromal hyperplasia, which is a risk factor for the development of ovarian cancer.Citation50
Here, we set out to study the effects of the BRCA1 gene on ROS and stromal metabolism in ovarian cancers.
Results
BRCA1 rescue abolishes hydrogen peroxide generation in BRCA1-null ovarian cancer cells
To investigate the relationship between BRCA1 mutations, oxidative stress and epithelial-stromal interactions, we conducted in vitro experiments with the human ovarian cell line, UWB1.289, which lacks a wild-type BRCA1 gene. This cell line is derived from a recurrent papillary serous ovarian cancer specimen in a patient with hereditary breast and ovarian cancer. More specifically, there is a germ-line mutation in BRCA1 gene, which leads to a stop at codon 845 and an acquired loss of the wild-type BRCA1 coding sequence. The cell line UWB1.289+BRCA1 is a stable cell line derived from UWB1.289 in which wild-type BRCA1 was restored via a pcDNA3 plasmid-carrying wild-type BRCA1 transfected into the parental line.Citation51
We demonstrated BRCA1 expression in the UWB1.289 cell line, where the BRCA1 gene was overexpressed (). Both UWB1.289 and UWB1.289+BRCA1 cells have been extensively characterized, and functionally UWB1.289 lacks DNA repair and cell cycle checkpoint abilities in response to DNA damage induced by ionizing radiation, while UWB1.289+BRCA1 cells show a partial rescue of the response to DNA damage.Citation51
Figure 1. BRCA1 rescue in BRCA1-null ovarian cancer cells decreases DNA damage. (A) UWB1.289 cells with BRCA1 rescue. UWB 1.289 cells with wild-type BRCA1 overexpression (UWB1.289+BRCA1) were previously generated. Immunoblotting was performed to confirm BRCA1 protein expression. UWB 1.289 cells were cultured in DMEM with 10% FBS for 72 h, achieving a confluent state. Equal loading was assessed by β-tubulin immunoblotting. (B) Wild-type BRCA1 decreases DNA damage. Control UWB1.289 cells and UWB1.289+BRCA1 were cultured in DMEM with 10% FBS for 72 h, achieving a confluent state. Immunoblotting was performed to assess γH2AX expression, which correlates with DNA damage. Equal loading was assessed by β-actin immunoblotting. Note that overexpression of wild-type BRCA1 decreases γH2AX levels.
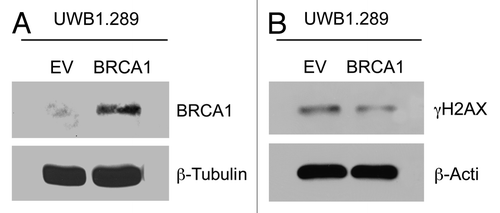
To demonstrate that overexpression of wild-type BRCA1 affects DNA damage, we studied levels of phosphorylated H2AX at Ser139 (γH2AX), which is a measure of DNA double-strand breaks.Citation52 Levels of γH2AX decrease modestly with wild-type BRCA1 overexpression (UWB1.289+BRCA1), as compared with the control cell line UWB1.289 ().
Next, we studied the effects of overexpression of wild-type BRCA1 on hydrogen peroxide generation in homotypic cultures. Remarkably, UWB1.289+BRCA1 cells have a 2.9-fold reduction in the intracellular levels of hydrogen peroxide in homotypic cultures as compared with UWB1.289 cells (). Hydrogen peroxide levels are also decreased in UWB1.289 cells overexpressing wild-type BRCA1, in co-culture with hTERT-immortalized human fibroblasts (BJ-1 cells), with a 2.1-fold decrease in levels compared to co-culture with the UWB1.289 cells ().
Figure 2. BRCA1-null ovarian cancer cells produce large amounts of hydrogen peroxide: Rescue with wild-type BRCA1 overexpression. (A and B) Control UWB1.289 cells and UWB1.289 cells with wild-type BRCA1 overexpression (UWB1.289+BRCA1) were cultured alone or with BJ-1 fibroblasts (A and B, respectively) for 96 h and H2O2 levels were measured using PFBS-F. Note that BRCA1 overexpression decreases H2O2 generation by 2.9-fold in homo-typic culture and by 2.1-fold in co-culture.
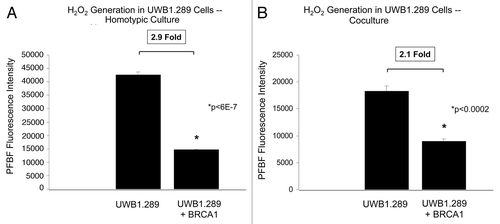
BRCA1-null ovarian cancer cells drive the generation of ROS species in stromal fibroblasts
Next, we studied the effects of co-culture of BJ-1 fibroblasts with UWB1.289 cells. Fibroblasts in co-culture with cancer cells have a 1.4-fold increase in reactive oxygen species (ROS) levels (). In contrast, fibroblasts co-cultured with UWB1.289+BRCA1 cells, which overexpress wild-type BRCA1, generate 1.4-fold less hydrogen peroxide than fibroblasts cultured with UWB1.289 cells, which lack wild-type BRCA1 (). In summary, BRCA1-null ovarian cancer cells generate more ROS and hydrogen peroxide, in particular when cultured alone and with fibroblasts in co-culture.
Figure 3. BRCA1-null ovarian cancer cells induce the generation of reactive oxygen species in adjacent fibroblasts: Rescue with BRCA1 overexpression. (A) hTERT-immortalized fibroblasts were cultured alone and with control UWB1.289 cells for 96 h and reactive oxygen species levels (ROS) was measured using CellROX. Note that co-culture induces a 1.4-fold increase in ROS generation in fibroblasts compared with homotypic culture. (B) hTERT immortalized fibroblasts were co-cultured with UWB1.289 cells and with UWB1.289+BRCA1 cells for 96 h and H2O2 levels were measured using PFBS-F. Note that wild-type BRCA1 overexpression decreases H2O2 generation 1.4-fold in fibroblasts.
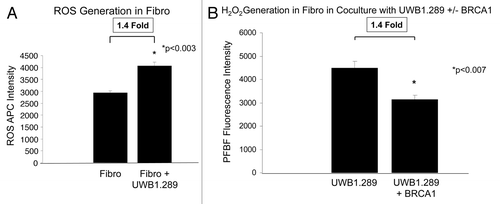
The simple antioxidant NAC abolishes hydrogen peroxide generation in BRCA1-null ovarian cancer cells and in co-cultured stromal fibroblasts
We studied the effects of the antioxidant N-acetyl cysteine (NAC) on hydrogen peroxide generation in UWB1.289 cells and fibroblasts. Addition of 10 mM NAC for 48 h to UWB1.289 cells in homotypic culture decreases hydrogen peroxide generation nearly 80-fold (). Similalry, NAC induces a near 10-fold decrease in hydrogen peroxide in UWB1.289 cells co-cultured with fibroblasts (). NAC also decreases hydrogen peroxide generation in fibroblasts in co-culture with UWB1.289 cells by 5.8-fold (). As a critical control experiment, fibroblasts in homotypic culture were treated with NAC or the vehicle-alone as a control (). However, NAC only decreases hydrogen peroxide in fibroblasts if it is generated during co-culture with UWB1.289 cells, since no significant changes in hydrogen peroxide levels were observed with NAC treatment in fibroblast homotypic cultures ().
Figure 4. The antioxidant NAC decreases hydrogen peroxide generation in BRCA1-null ovarian cancer cells. (A and B) Homotypic UWB1.289 cell cultures and co-cultures with human BJ-1-GFP fibroblasts (A and B, respectively) were performed for 5 d. Vehicle or 10 mM NAC was then added every 24 h to the cell culture media starting 48 h prior to measurement of H2O2 generation with PFBS-F. Six hours prior to measurement, the media was changed to HBSS with and without 10 mM NAC. Note that addition of NAC decreases the generation of H2O2 by 77.9-fold and 9.7-fold in UWB1.289 cells, in homo-typic cultures and in fibroblast co-cultures, respectively.
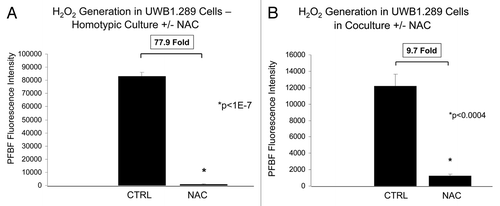
Figure 5. NAC decreases hydrogen peroxide generation in fibroblasts in co-culture with BRCA1-null ovarian cancer cells. (A and B) Homotypic hTERT fibroblast culture and co-culture with UWB1.289 cells (A and B, respectively) was performed for 5 d. Vehicle or 10 mM NAC was then added every 24 h to the cell culture media for 48 h prior to measurement of H2O2 generation with PFBS-F. Six hours prior to measurement, media was changed to HBSS with and without 10 mM NAC. Note that NAC decreases H2O2 generation by 5.8-fold in fibroblasts co-cultured with UWB1.289 cells, as compared with the control co-cultures. However, under homo-typic culture conditions, addition of NAC did not significantly change the levels of H2O2 in fibroblasts.
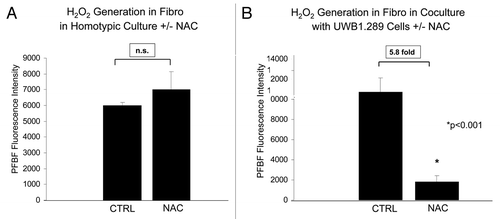
NAC treatment increases the apoptotic death rate of BRCA1-null ovarian cancer cells, especially during co-culture with stromal fibroblasts
Having found a link between BRCA1 and generation of reactive oxygen species, we set out to determine the effects of the antioxidant NAC on apoptosis rates in UWB1.289 cells, in homotypic culture and during co-culture with fibroblasts. NAC induces a 1.2-fold increase in apoptosis of UWB1.289 cells in homotypic culture and a 1.7-fold increase in apoptosis during co-culture with fibroblasts (). Co-culture with fibroblasts protects UWB1.289 cells from apoptosis under control conditions, with a 1.3-fold decrease in the rate of apoptosis as compared with homotypic cultures (p < 0.009). Importantly, NAC is able to overcome the apoptosis resistance induced by the co-culture.
Figure 6. NAC induces apoptosis in BRCA1-null ovarian cancer cells, especially during co-culture with fibroblasts. Homo-typic UWB1.289 cell cultures and co-cultures with human BJ-1-GFP fibroblasts were performed for 5 d. Then, 10 mM NAC or vehicle was added to the cell culture media daily starting 48 h prior to the measurement of apoptosis with Annexin V-APC and PI. Six hours prior to measuring apoptosis, the media was changed to HBSS with and without 10 mM NAC. Note that addition of NAC increases apoptosis by 1.7-fold under co-culture conditions, while it only increases apoptosis by 1.2-fold under homo-typic cell culture conditions (p < 0.02). Also, note that fibroblasts exert a positive protective effect on UWB1.289 cells, with a 1.3-fold decrease in the rate of apoptosis, as compared with control homotypic cultures (p < 0.009), and NAC is able to overcome the positive protective effects of stromal fibroblasts.
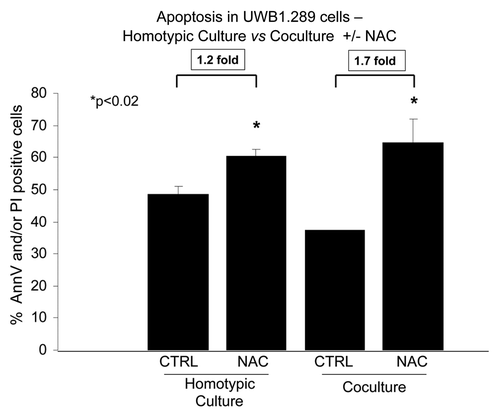
BRCA1 rescue or treatment with NAC downregulates MCT4 expression in BRCA1-null ovarian cancer cells
We assessed MCT4 expression in UWB1.289 cells in homotypic culture by immunofluorescence, flow cytometry and quantitative RT-PCR. MCT4 is the main transporter/exporter of L-lactate out of cells; it is induced by HIF-1α binding to its promoter, and it is a marker of glycolytic metabolism and oxidative stress.Citation53 As predicted, NAC and overexpression of wild-type BRCA1 both downregulate MCT4 protein expression in UWB1.289 cells ().
Figure 7. MCT4 expression is high in BRCA1-null ovarian cancer cells: Rescue with antioxidant treatment (NAC) or BRCA1 overexpression. (A) UWB1.289 parental and UWB 1.289-BRCA1-overexpressing cells were cultured for 5 d. Then 10 mM NAC or vehicle was added every 24 h for 48 h. Cells were then fixed and immunostained with anti-MCT4 (red) and anti-K8–18 (green) antibodies. MCT4 staining (red) is shown in the top panels, while keratin and DAPI (as a nuclear counter-stain) are shown in the bottom panels. Original magnification, 40x. Note that treatment with NAC or wild-type BRCA1-overexpression downregulates MCT4 expression in UWB1.289 cells in homo-typic cultures. (B) MCT4 expression by flow cytometry (FACS) in UWB1.289 cells and UWB1.289+BRCA1 cells. Cells were cultured as described above, then they were fixed and immunostained with an anti-MCT4 antibody. Expression was measured by flow cytometry. Note that overexpression of wild-type BRCA1 leads to a 2.9-fold downregulation of MCT4 in homo-typic cultures. (C) MCT4 mRNA expression in UWB1.289 cells and UWB1.289+BRCA1 cells. The cells were cultured as described above, then the mRNA expression of MCT4 was measured by real-time reverse transcriptase PCR. Note that overexpression of wild-type BRCA1 leads to an 8-fold reduction in MCT4 mRNA expression.
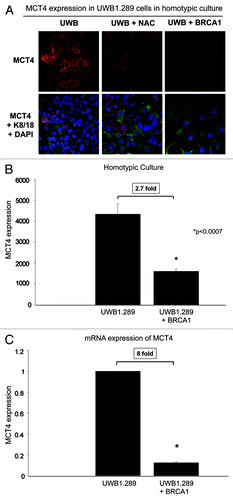
We also performed experiments measuring MCT4 expression by flow cytometry (FACS) to validate our immunofluorescence results and found a 2.7-fold decrease in MCT4 expression in UWB1.289 cells, which overexpress wild-type BRCA1, as compared with control cells (). To determine if transcription is playing a role in MCT4 downregulation mediated by BRCA1 wild-type overexpression, we measured MCT4 mRNA expression. BRCA1 wild-type overexpression in UWB1.289+BRCA1 cells decreases MCT4 transcription by 8-fold, as compared with UWB1.289 cells ().
BRCA1-null ovarian cancer cells induce MCT4 expression in stromal fibroblasts: Rescue with BRCA1 or the antioxidant NAC
As mentioned above, MCT4 expression is a marker of oxidative stress and glycolytic metabolism, secondary to mitochondrial dysfunction. BJ-1 fibroblasts were cultured alone or with UWB1.289 cells, and then MCT4 expression was evaluated by immunofluorescence. Co-culture with UWB1.289 cells induces a striking upregulation of MCT4 in fibroblasts (). Co-culture of fibroblasts with UWB1.289 and UWB1.289+BRCA1 cells, with and without NAC, was performed and MCT4 expression was evaluated by immunofluorescence, using the same settings as in . Remarkably, MCT4 expression is high in fibroblasts co-cultured with UWB1.289 cells and is dramatically downregulated by treatment with NAC or by BRCA1 rescue of UWB1.289 cells ().
Figure 8. MCT4 expression is induced in stromal fibroblasts co-cultured with BRCA1-null ovarian cancer cells: Rescue with NAC or wild-type BRCA1. (A and B) UWB1.289 cells and UWB1.289+BRCA1 cells were cultured with BJ-1 fibroblasts for 5 d. Then, 10 mM NAC or vehicle alone was added every 24 h for 48 h. Cells were then fixed and immunostained with anti-MCT4 (red) and anti-K8–18 (green) antibodies. MCT4 staining (red) is shown in the top panels and MCT4, keratin and DAPI as a nuclear counterstain are shown in the bottom panels. Original magnification, 40x. Note that UWB1.289 cells induce high MCT4 expression in fibroblasts and that MCT4 expression in fibroblasts is abolished by addition of NAC or by BRCA1 rescue in UWB1.289 cells.
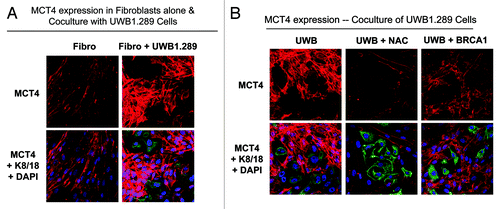
BRCA1-null ovarian cancer cells drive the paracrine activation of NFκB in adjacent stromal fibroblasts: Rescue with epithelial BRCA1 replacement
Here, we show that wild-type BRCA1 rescue of ovarian cancer cells decreases oxidative stress. Interestingly, it is known that high levels of reactive oxygen species (ROS) can hyperactivate NFκB signaling.Citation54 Hence, we investigated the effects of BRCA1 wild-type rescue on NFκB expression. Subunit p65 of NFκB is downregulated upon BRCA1 wild-type overexpression in UWB1.289 cells, as well as MCT4 expression ().
Figure 9. BRCA1-null ovarian cancer cells promote activation of the pro-autophagic and pro-glycolytic NFκB pathway in the tumor stroma: rescue with wild-type BRCA1. (A) NFκB p65 subunit and MCT4 expression. UWB 1.289 cells with wild-type BRCA1 overexpression (UWB1.289+BRCA1) were cultured in 10% FBS DMEM for 72 h, achieving a confluent state. Western blot was performed to assess NFκB p65 subunit expression and MCT4 expression. Equal loading was assessed by β-tubulin immunoblotting. Note that BRCA1 wild-type overexpression leads to downregulation of NFκB and MCT4. (B) NFκB activation in the tumor stroma. NIH3T3-NFκB-Luc reporter fibroblasts were plated with UWB1.289 cells or with UWB1.289+BRCA1 cells. The next day, the media was changed and that was considered day 0. Cells were then cultured for up to 3 d. As a control, homo-typic cultures of fibroblasts alone were established and processed in parallel. Luciferase activity was measured daily. Note that luciferase activity is increased by 24-fold on day 0 in NFκB-Luc fibroblasts co-cultured with UWB1.289 cells, as compared with homo-typic cultures. These results suggest that the NFκB pathway is potently activated as an early event during co-culture. Although the fold difference in luciferase activity is highest at day 0, the activity remains elevated by ~4-fold at day 3 in NIH3T3-NFκB-Luc co-cultured cells with UWB1.289 cells, as compared with homotypic cultures. Thus, NFκB activation in stromal fibroblasts persists at later time-points. *p < 0.0002 for day 0, *p < 1.5E-5 for day 1, *p < 0.002 for day 2, *p < 0.0006 for day 3; NFκB-Luc homo-typic cultures (fibroblasts alone) vs. UWB1.289 comparisons (fibroblasts plus ovarian cancer cells) (Student’s t-test).
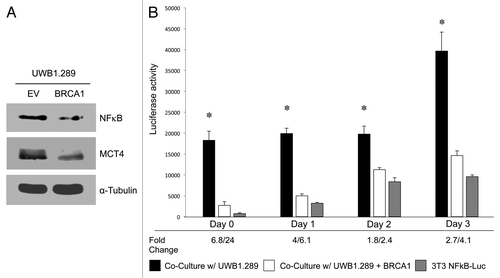
We next investigated the effects of BRCA1 mutation status in epithelial cancer cells on NFκB signaling in the stroma. Autophagy, glycolysis and oxidative stress are induced in the stroma by activation of NFκB signaling.Citation55 In order to detect NFκB activation in the stroma, we employed a luciferase-reporter approach. NIH3T3 firbroblasts were engineered to express a luciferase reporter gene under the control of an NFκB-responsive element (NFκB-Luc cells). These fibroblasts were then co-cultured with UWB1.289 cells or UWB1.289+BRCA1 for up to 3 d. Luciferase activity was measured daily. Homotypic cultures of NFκB-Luc cells were evaluated in parallel.
shows that stromal luciferase activity is increased in NFκB-Luc fibroblasts by 24-fold on day 0 in co-culture with UWB1.289 cells, as compared with homotypic fibroblast cultures (p < 0.0002). Importantly, stromal activation of NFκB was effectively blocked or rescued by the overexpression of wild-type BRCA1 in the BRCA1-null ovarian cancer cells (UWB1.289+BRCA1). Quantitatively similar results were obtained on days 1, 2 and 3 of co-culture, with BRCA1-null ovarian cancer cells maximally activating stromal NFκB activity at all time points. This suggests that the relative increase in NFκB activation is highest during early co-culture. In this experiment, it is important to note that the day 0 time point actually represents 24 h of co-culture (see Experimental Procedures).
In summary, BRCA1-null ovarian cancer cells induce NFκB activation in adjacent stromal fibroblasts, ultimately leading to an acute and chronic inflammatory response, as well as autophagy, mitophagy and glycolysis in the tumor stroma.
BRCA1-null ovarian cancer cells and stromal fibroblasts show evidence of reciprocal metabolic reprogramming during co-culture
Glucose uptake in UWB1.289 cells and BJ-1-RFP fibroblasts cultured alone or in co-culture with one another was performed. Co-culture exerts differential effects on UWB1.289 cells and fibroblasts, with 1.3-fold decreased glucose uptake in the cancer cells () and a 1.2-fold increase in the stromal cells (). Glucose uptake correlates with glycolysis and the Warburg effect. Thus, under co-culture conditions, the stromal cells are predominantly undergoing the Warburg effect, as compared with the epithelial cancer cells in co-culture with a 2.5-fold higher rate of glucose uptake than the UWB1.289 cells (). Note that the Warburg effect occurs to a greater extent in UWB1.289 cells in homotypic culture than in co-culture with fibroblasts ().
Figure 10. Reciprocal metabolic reprogramming in BRCA1-null ovarian cancer cells and co-cultured stromal fibroblasts. (A) Glucose uptake in UWB1.289 cells and fibroblasts was measured under homo-typic and co-culture conditions. Cells were cultured for 7 d, and glucose uptake was measured by NBDG uptake. Note that NBDG uptake decreases by 1.3-fold in UWB1.289 cells in co-culture with fibroblasts. (B) Glucose uptake in fibroblasts cultured alone and with UWB1.289 cells. Cells were cultured alone and with UWB1.289 for 5 d. Then, the media was changed to HBSS for 16 h and glucose uptake was measured by measuring NBDG uptake. Note that NBDG uptake decreases by 1.2-fold during co-culture with fibroblasts.
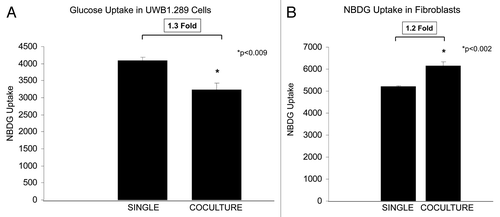
BRCA1-null ovarian cancer cells induce a loss of Cav-1 in stromal fibroblasts via oxidative stress and autophagy: Rescue with the antioxidant NAC or via epithelial BRCA1 replacement
Loss of Cav-1 in fibroblasts is associated with a poor prognosis in many types of human cancers.Citation38,Citation39,Citation56,Citation57 Fibroblasts with loss of Cav-1 have a CAF-like phenotype, with high levels of ROS, glycolysis and autophagy.Citation45,Citation46,Citation56,Citation58
Thus, co-culture experiments with UWB1.289 and UWB1.289+BRCA1 cells were performed to study the effect of BRCA1 and NAC on Cav-1 expression in fibroblasts. As predicted, co-culture with UWB1.289 cells leads to a loss of Cav-1 in fibroblasts, which is rescued by treatment with 10 mM NAC or by BRCA1 wild-type overexpression in UWB1.289 cells (). This observation is consistent with the idea that Cav-1 is downregulated by autophagic-lysosomal degradation that is induced by oxidative stress in stromal fibroblasts, as we have shown previously, under other experimental conditions.
Figure 11. Cav-1 expression is downregulated in stromal fibroblasts co-cultured with BRCA1-null ovarian cancer cells: Rescue with NAC or wild-type BRCA1. (A and B) UWB1.289 cells and UWB1.289+BRCA1 cells were cultured with BJ-1 fibroblasts for 5 d. Then, 10 mM NAC or vehicle alone was added every 24 h for 48 h. Cells were then fixed and immunostained with anti-Cav-1 (red) and anti-K8–18 (green) antibodies. Cav-1 staining (red) is shown in the top panels, with MCT4, keratin and DAPI (as a nuclear counter-stain) shown in the bottom panels. Original magnification, 40x. Note that Cav-1 is downregulated in fibroblasts co-cultured with UWB1.289 cells and that Cav-1 expression in fibroblasts is rescued by the addition of NAC or the BRCA1 wild-type gene in UWB1.289 cells (A). Also, note that NAC does not exert any effects on Cav-1 expression in fibroblasts under homo-typic culture conditions (B).
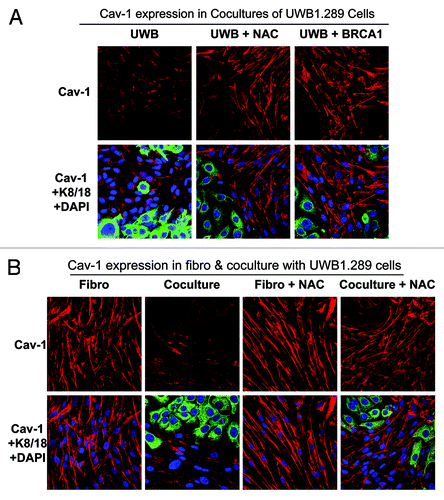
Stromal fibroblasts lacking Cav-1 are known to produce more ROS and undergo further oxidative stress-induced autophagy via a feedforward mechanism. Thus, the loss of stromal Cav-1 should be “contagious.” To investigate if a loss of Cav-1 can be further amplified by the surrounding stromal fibroblasts, fibroblasts with Cav-1 downregulation via siRNA and fibroblasts not exposed to Cav-1 siRNA were co-cultured, and the appropriate controls were performed in parallel. Note that loss of Cav-1 in fibroblasts leads to further loss of Cav-1 in non-transfected fibroblasts in close proximity (). Thus, oxidative stress spreads like an infectious agent, from one fibroblast to another, in the tumor microenvironment.
Figure 12. Cav-1-deficient fibroblasts induce the downregulation of Cav-1 in normal adjacent fibroblasts, when the two fibroblasts subtypes are co-cultured. hTERT-GFP(+) fibroblasts and hTERT-GFP(-) fibroblasts (transfected with Cav-1 siRNA or control siRNA) were co-cultured for 72 h. Note that the presence of GFP(-) fibroblasts harboring Cav-1 siRNA induces the loss of Cav-1 in surrounding normal GFP(+)-fibroblasts, suggesting that loss of Cav-1 can also exert its effects at a distance, creating a Cav-1-deficient field of fibroblasts. Cells were then fixed and immunostained with an anti-Cav-1 (red) antibody and green fluorescence in the BJ-1-GFP cells was detected (green). Cav-1 staining (red) is shown in the top panels, and GFP (which marks BJ-1 cells not treated with siRNA) and DAPI (as a nuclear counterstain) are shown in the bottom panels. Original magnification, 40x.
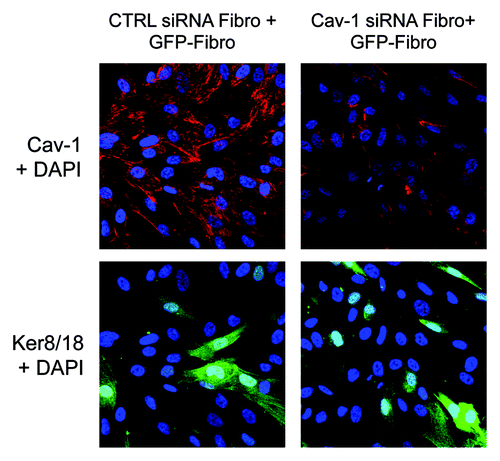
Finally, co-cultures of fibroblasts and UWB1.289 cells were assessed for additional markers of autophagy and mitophagy. shows that fibroblasts in co-culture with UWB1.289 cells have much higher levels of Beclin-1, Cathepsin B and BNIP3L than those cultured with UWB1.289+BRCA1 cells. Thus, BRCA1-null ovarian cancer cells induce oxidative stress, driving autophagy and mitophagy in the tumor microenvironment.
Figure 13. BRCA1-null ovarian cancer cells induce autophagy and mitophagy in adjacent fibroblasts: Rescue with wild-type BRCA1. BJ-1 fibroblasts were co-cultured with either UWB1.289 cells or UWB1.289+BRCA1 cells for 3 d. Cells were then fixed and immunostained with anti-Beclin-1, Cathepsin B or BNIP3L (red) and anti-K8–18 (green) antibodies. Co-culture of BJ-1 fibroblasts with UWB1.289 cells is shown in the top panels and the co-culture with UWB1.289+BRCA1 cells is shown in the bottom panels. Original magnification, 40x. Note that Beclin-1, Cathepsin B and BNIP3L are upregulated in fibroblasts co-cultured with UWB1.289 cells, as compared with co-culture with UWB1.289+BRCA1 cells.
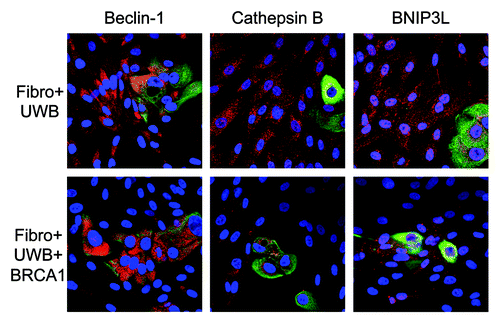
Discussion
Here, we show that the BRCA1-null mutation induces hydrogen peroxide generation in epithelial cancer cells and adjacent stromal cells. Moreover, this paracrine interaction drives reciprocal metabolic coupling, with increased glycolysis and MCT4 expression in stromal cells and, conversely, decreased glycolysis and MCT4 expression in epithelial cells. This metabolic coupling and MCT4 expression in fibroblasts leads to protection from apoptosis in the epithelial cancer cells ().
Figure 14. Epithelial loss of BRCA1 induces hydrogen peroxide production, driving oxidative stress and NFκB activation in the tumor stroma. A schematic diagram summarizing our current findings is shown. Our results directly show that BRCA1 normally functions as an antioxidant that prevents hydrogen peroxide production. When BRCA1 is lost or inactivated, this leads to increased hydrogen peroxide production, which results in DNA damage in cancer cells. However, cancer cell-derived hydrogen peroxide also has paracrine effects on adjacent stromal fibroblasts, which converts them to a more catabolic phenotype. Catabolic cancer-associated fibroblasts then produce more ROS and undergo autophagy and mitophagy (due to NFκB activation), leading to the loss of Cav-1 (via autophagic digestion) and MCT4 upregulation (reflecting the onset of oxidative stress and glycolysis, due to mitochondrial dysfunction). Finally, the catabolic fibroblast phenotype is contagious and spreads to other neighboring fibroblasts, via more ROS production, leading to Cav-1 downregulation in normal adjacent fibroblasts. This explains the “field effect,” also known as “field cancerization.” It represents a field or cascade of oxidative stress.
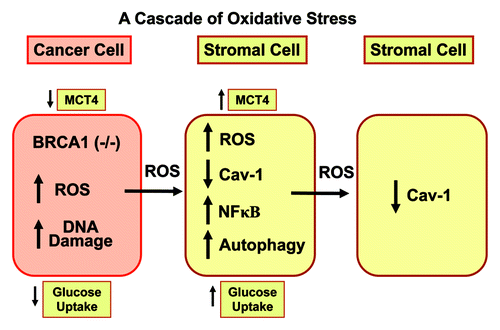
Most importantly, treatment with the simple antioxidant NAC is able to phenocopy the effects of BRCA1 rescue by decreasing hydrogen peroxide generation. Thus, systemic antioxidants can metabolically uncouple both cell types with loss of fibroblast MCT4 expression and induce apoptosis of the epithelial cancer cells. To our knowledge, this is the first study to evaluate the effects of BRCA1 mutations and antioxidants on oxidative stress and cancer metabolism, all in the context of epithelial-stromal interactions.
The fact that stromal cells in co-culture with BRCA1-null ovarian cancer cells increase MCT4 expression and glucose uptake indicates that the fibroblast compartment is undergoing the Warburg effect, instead of the epithelial cancer cell compartment, as was previously proposed based on homotypic culture experiments.Citation59 Also, ovarian cancers that lack stroma have features consistent with the Warburg effect (high GLUT1 and HIF1ɑ), while this is not seen with a highly vascularized stroma.Citation60
This has important implications when assessing drugs that target glycolysis, since they will alter predominantly the metabolism of stromal cells. Also, FDG-PET scanning in patients with BRCA1-null ovarian cancer, although valuable to assess disease status and response to therapy,Citation61,Citation62 may in fact be evaluating the burden of reactive stroma, rather than that of epithelial cancer cells. Supporting this is the fact that necrotic peri-tumoral areas have high FDG-PET avidity in many different types of cancers.Citation63 It is now well-established that myofibroblast transformation promotes tumor growth, and that ROS and a glycolytic phenotype in fibroblasts is sufficient to drive a CAF conversion with myofibroblastic features.Citation64-Citation66
Role of antioxidants in the management of BRCA1-related disease
BRCA1-related ovarian cancers are difficult to detect by conventional screening methods and are frequently diagnosed at an advanced stage, which makes this disease incurable.Citation67 Prophylactic bilateral mastectomies and oophorectomies improve clinical outcomes, although the morbidity and negative effects on quality of life are significant.Citation68-Citation70
Hence, the use of antioxidants may be valuable in primary and secondary prevention of breast and ovarian cancers in carriers of BRCA1 mutations. Use of resveratrol decreased reactive oxygen species in BRCA1-mutated cells and decreased DNA damage while, at the same time, increasing levels of the antioxidant NRF2.Citation71 Another study showed that the antioxidant selenium reduced oxidative DNA damage in women carriers of BRCA1 mutations.Citation72 Also, selenium normalizes the rates of chromosome breakage in women carriers of BRCA1 mutations.Citation73 High selenium intake is associated with a reduced risk of ovarian cancer in a population-based, case-control study.Citation74 A clinical trial studying the use of soy isoflavones, which have antioxidant properties, in patients with BRCA1 mutations is ongoing (NCT 01219075).
It is apparent that biomarker(s) of risk endpoints for antioxidant primary and secondary prevention studies need to be incorporated.Citation75 Downregulation of MCT4 in fibroblasts via NAC is sufficient to induce apoptosis of UWB1.289 cells. We previously discovered that stromal MCT4 expression in MCF7-fibroblasts co-cultures could be downregulated via NAC.Citation31 The oncogenic importance of stromal MCT4 is supported by the fact that high MCT4 expression in fibroblasts in triple-negative breast cancer (ER/PR/HER-2 negative) is associated with an extremely poor prognosis.Citation37 Triple-negative breast cancer shares many features with BRCA1-null cancers.Citation20,Citation76 Not only will it be important to determine if stromal MCT4 expression has prognostic value in ovarian cancer and whether use of antioxidants can decrease stromal MCT4 expression in patients with ovarian cancer, but also whether NAC can be used for primary or secondary prevention of BRCA1-null cancers (breast and ovarian). However, window studies should be performed first to determine in which BRCA1 mutation carriers NAC downregulates stromal MCT4 expression.
BRCA1 mutation carriers develop aggressive high-grade, advanced-stage ovarian cancer, with increased susceptibility to platinum and resistance to taxane chemotherapy.Citation12 Although our work has focused on hereditary ovarian cancer, with mutations in the BRCA1 gene which lead to decreased expression,Citation51 it may also be more broadly applicable to sporadic ovarian cancers. Approximately 15% of sporadic ovarian cancers have BRCA1 promoter hyper-methylation, with absent expression of BRCA1.Citation77 Reduced BRCA1 protein expression in sporadic ovarian cancer is found in up to 90% of these cancers.Citation78,Citation79 Since BRCA1 expression is absent or reduced in the majority of ovarian cancers, oxidative stress may be the driver of this cancer phenotype, and it will be important to determine if suppression of oxidative stress (in addition to its use as a prevention strategy) can change the phenotype to a less aggressive one when a patient has been diagnosed with ovarian cancer.
Limitations of in vitro models in ovarian cancer
It is important to recognize the many limitations of using in vitro studies and an ovarian cell line, with multiple mutations in addition to BRCA1.Citation51,Citation80,Citation81 UWB1.289 cells have mutations in TP53 in addition to BRCA1. UWB1.289 cells are also polyploid with multiple additional genetic abnormalities. The UWB1.289 cell line recapitulates many features seen in human BRCA1-mutated ovarian cancer, since, as previously mentioned, these ovarian cancers are very aggressive with aneuploidy and have multiple mutations including mutations in TP53.Citation67
It now appears that TP53 mutations are induced by BRCA1 mutations and occur in the majority of patients with these BRCA1 mutations.Citation82 BRCA1 mutations associated with increased risk of cancer are most frequently frameshift or non-sense mutations that lead to a premature stop codon and a truncated protein product as occurs in the UWB1.289 cells.Citation51,Citation83 Using a wild-type BRCA1 overexpresssion approach in our experiments allowed us to determine the effects of BRCA1 mutations on oxidative stress and metabolism, taking into account the role of the tumor microenvironment.
We believe that although in vitro studies cannot recapitulate the in vivo conditions, with incorporation of the tumor microenvironment, we can more accurately assess tumor metabolism. We and others have shown that homotypic cancer cell culture experiments induce a metabolic phenotype that is different than that observed in human cancer and in models including the stroma, where metabolic coupling is frequently encountered.Citation30-Citation32,Citation45,Citation84,Citation85
For example, one study in ovarian cancer demonstrates that the presence of adipocytes induces tumor aggressiveness and drives metabolic coupling with mitochondrial metabolism in the ovarian epithelial cancer cells.Citation32 Our current study shows similar results, with MCT4 expression in fibroblasts being associated with apoptosis resistance in the epithelial cancer cells. More specifically, MCT4 expression and glucose uptake in homotypic UWB1.289 cell culture experiments is high. Conversely, during co-culture, the UWB1.289 cells loose MCT4 expression and have decreased glycolysis, and the stromal cells have high MCT4 expression and increased glycolysis.
The “stromal field effect” in ovarian cancer
Ovarian cancer is the leading cause of gynecological cancer death in the United States.Citation1 Approximately two-thirds of patients with ovarian cancer have metastatic disease at diagnosis, which is incurable.Citation67,Citation86 BRCA1 mutations, or altered expression, correlates with genomic instability in ovarian cancer and the presence of multiple clones.Citation67
The results presented here on the “stromal field effect” may explain, in part, why advanced disease at diagnosis is so common in hereditary and sporadic ovarian cancers. Multiple studies have shown that a “field cancerization effect” exists in ovarian cancer, with relapses having new clones that were previously undetected and allowing for re-treatment at the time of relapse with the same chemotherapeutic agents that were used at diagnosis if the duration of remission is prolonged.Citation87-Citation91
The term “field cancerization” was first coined by Slaughter, who hypothesized that in head and neck cancers, there is progressive transformation of cells adjacent to a tumor rather than the spread of tumor cells by pre-existing cancer cells,Citation92 and this hypothesis has been confirmed with modern genetic techniques to evaluate clonality and cancer cell evolution.Citation93,Citation94 However, it is still unclear how cancer fields develop.Citation94
We have previously shown that a tumor stroma with high levels of oxidative stress drives genomic instability in adjacent cancer cells.Citation45 It is known that there are high levels of reactive oxygen species systemically in subjects with ovarian cancer.Citation95 The tumor stroma and host tissues in general may drive these high levels of ROS and have an amplifying effect on ovarian cancer genomic instability. We describe a novel mechanism by which BRCA1 inactivation drives ovarian cancer aggressiveness; namely, that BRCA1 inactivation induces epithelial oxidative stress, which is amplified in the stroma via the field effect, and this reduces the apoptotic death of the ovarian cancer cells. We show in this study that BRCA-mutated ovarian cancer cells induce ROS in stromal cells, which leads to a “transformed” stromal phenotype, with a loss of fibroblast expression of Cav-1, NFκB activation, thereby driving autophagy and mitophagy in the tumor stroma.
In summary, we show that BRCA1-null mutations and loss of expression drives hydrogen peroxide generation in both ovarian cancer cells and stromal cells, which induces resistance to apoptosis, accompanied by metabolic coupling and stromal glycolysis, as well as high autophagy and a loss of Cav-1 in the tumor stroma.
Experimental Procedures
Materials
Antibodies were as follows: BRCA1 (CS9010, Cell Signaling), γH2AX (ab18311, Abcam), MCT4 (generous gift of Dr. Nancy Philp, which is an isoform-specific rabbit polyclonal antibody against the 18-mer synthetic oligo-peptide corresponding to the C-terminal amino acids of human MCT4Citation96); cytokeratin 8/18 (20R-CP004, Fitzgerald Industries International); tubulin (T4026, Sigma) and actin (A5441, Sigma). Secondary antibodies for immunofluorescence and flow cytometry were Alexa green 488 nm and Alexa Orange-Red 546 nm (Invitrogen). Other reagents were as follows: N-acetyl cysteine (NAC) was from Sigma, 2-[N-(7-nitrobenz-2-oxa-1,3-diazol-4-yl)amino]-2-deoxy-D-glucose (2-NBDG), 4,6-diamidino-2- phenylindole (DAPI), propidium iodide (PI) were from Invitrogen. The Annexin-V-APC conjugate for measuring apoptosis was from BD; the CellROX assay for measuring reactive oxygen species was from Invitrogen, and PFBS-F (Pentafluorobenzenesulfonyl fluorescein) for measuring hydrogen peroxide was from Cayman Chemicals.
Cell cultures
The ovarian cancer cell lines UWB1.289 and UWB1.289+BRCA1 were purchased from ATCC, and experiments were performed in the recommended complete growth medium (50% RPMI-1640 and 50% mammary epithelial growth medium from Clonetics/Lonza with 3% FBS and Penicillin 100 units/mL-Streptomycin 100 µg/mL). Human skin fibroblasts (BJ-1) immortalized with the telomerase reverse transcriptase catalytic domain (hTERT) were originally purchased from Clontech, Inc., and clones were generated with either GFP or RFP overexpression. BJ-1 fibroblasts were cultured in UWB1.289 complete growth media.
Co-cultures of UWB1.289 and HCC 1937 cells and fibroblasts
BJ-1 fibroblasts overexpressing GFP or RFP and either the ovarian cancer cell line (UWB1.289 or UWB1.289+BRCA1) were plated on glass coverslips in 12-well plates in 1 ml of complete media. Epithelial cells were plated within 2 h of fibroblast plating. The total number of cells per well was 1 × 105. Experiments were performed at a 5:1 fibroblast-to-epithelial cell ratio. As controls, homotypic mono-cultures of fibroblasts and epithelial cells were seeded using the same number of cells as the corresponding co-cultures. Cells were maintained at 37°C in a humidified atmosphere containing 5% CO2.
Immunoblotting
Immunoblotting was performed as previously described.Citation55 Briefly, cells were scraped in urea lysis buffer (6.7 M urea, 10% glycerol, 1% SDS, 10 mM TRIS-HCl pH 6.6, 1% Triton X-100, protease inhibitors), homogenized for 5 sec and incubated on ice for 10 min. After centrifugation, protein concentration was determined using the Bradford assay (BioRad). Thirty μg of proteins were loaded and separated by SDS-PAGE and transferred to a 0.2 μm nitrocellulose membrane (Fisher Scientific). After blocking for 30 min in TBST (10 mM TRIS-HCl pH 8.0, 150 mM NaCl, 0.05% Tween-20) with 5% nonfat dry milk, membranes were incubated with the primary antibody for 1 h, washed and incubated for 30 min with horseradish peroxidase-conjugated secondary antibodies. The membranes were washed and incubated with enhanced chemiluminescence substrate (Thermo Scientific).
Flow cytometric analysis
UWB1.289 and UWB1.289+BRCA1 cells were plated in co-culture with BJ-1 fibroblasts [which were either GFP (+) or RFP (+)] or plated in mono-culture. Cells were grown for a total culture duration of 7 d. Then, to isolate the GFP (+) or RFP (+) BJ-1 cell population, co-cultured cells were sorted using a 488 nm and a 543 nm laser. As a critical control, mono-cultures of UWB1.289 or UWB1.289+BRCA1 cells, which are GFP (-) and RFP (-), and BJ-1 fibroblast cells, which are GFP (+) or RFP(+), were sorted in parallel.
For MCT4 expression, cells were collected by ultracentrifugation and supernatant aspiration and were resuspended in 1 mL PBS. They were fixed in 2% PFA for 15 min at room temperature, then permeabilized by adding ice-cold 100% methanol slowly to pre-chilled cells and incubated at -20°C for 5 min. Then 1 × 106 cells were aliquoted into each assay tube and 2 mL of incubation buffer (PBS, 1% BSA, 0.1% Tween 20) and incubated for 15 min. The unconjugated anti-MCT4 antibody was added and incubated for 1 h at room temperature. The cells were rinsed by centrifugation in incubation buffer, resuspended in fluorochrome-conjugated secondary antibody and incubated at room temperature for 30 min. Then, cells were rinsed again in incubation buffer, resuspended in PBS and analyzed by flow cytometry. Analysis was performed using FlowJo 8.8 software.
Measurement of hydrogen peroxide
PFBS-F (Pentafluorobenzenesulfonyl fluorescein) is a cell-permeable molecule. PFBS-F is a non-fluorescent molecule that, when taken up by cells, emits fluorescence in the presence of hydrogen peroxide, since it undergoes per-hydrolysis of the sulfonyl linkage. Fluorescence emission is hence a measure of intracellular hydrogen peroxide levels. The PFBS-F fluorescence method was used according to the manufacturer’s instructions. Briefly, UWB1.289 or UWB1.289+BRCA1, cells either in homo-typic culture or co-cultured with BJ-1 fibroblasts were treated as described above. Cells were then washed twice with PBS, incubated with 2.5 μM PFBS-F for 45 min at 37°C. Cells were then washed in PBS, harvested and re-suspended in 500 μL of PBS on ice. Cells were analyzed by flow cytometry (FACS) using a FITC signal detector to detect intensity of fluorescein emission. Data analysis was performed using FlowJo 8.8 software.
Measurement of reactive oxygen species
CellROX (cat #C10422, Invitrogen) is a cell-permeable dye used to measure intracellular ROS. CellROX is non-fluorescent in a reduced state. When oxidized by reactive oxygen species witihin cells, it has high fluorescence, with absorption/emission maxima of ~644⁄665 nm. The fluorescence method was used according to the manufacturer’s instructions. Briefly, BJ-1 fibroblasts which were RFP (+) were cultured homotypically or in coculture with UWB1.289 cells. Cells were then incubated with 5 μM CellROX in DMEM with 10% FBS for 30 min at 37°C. Cells were then washed in PBS × 3, harvested and re-suspended in 500 μL of PBS. Cells were analyzed by flow cytometry using an RFP signal detector to distinguish fibroblasts from UWB1.289 cells (fibroblasts are the RFP-positive population), and an APC signal detector to measure levels of intracellular-reactive oxygen species.
Measurement of glucose uptake
Glucose uptake was performed using 2-NBDG. UWB1.289 cells in homo-typic culture or co-cultured with BJ-1 + RFP fibroblasts were cultured for 7 d. Then, the media was changed to HBSS for 16 h, and the cells were incubated with pre-warmed 2-NBDG solution (diluted in phenol red-free 10% DMEM to a final concentration of 250 μM) for 30 min at 37°C. All subsequent steps are performed in the dark. Cells were then washed in PBS, harvested and re-suspended in 500 μL of PBS. Cells were then analyzed by flow cytometry using a GFP signal detector with excitation wavelength of 488 nm and emission of 530 nm (to detect 2-NBDG), and an RFP signal detector with excitation wavelength of 496 nm and emission of 615 (to detect RFP-positive BJ-1 cells). Thus, UWB1.289 cells in co-culture are the RFP-negative population.
Annexin V-PI apoptosis detection
Cell death was quantified by flow cytometry using propidium iodide (PI) and Annexin-V-APC, as previously described with minor modifications.Citation97 Briefly, BJ1-GFP or BJ1-RFP cells were plated in 12-well plates with either UWB1.289 or UWB1.289+BRCA1 cells for 7 d of culture. Ten mM NAC was added to the media 48 h prior to harvesting. Cells were collected by centrifugation and re-suspended in 500 μL of PBS. Then, the annexin V-APC conjugate (4 μL) and PI (1 μL) was added and incubated in the dark at room temperature for 5 min. Cells were then analyzed by flow cytometry using a GFP or RFP signal detector (to detect BJ1-GFP or BJ1-RFP cells), a PE Texas Red signal detector for PI staining and an APC signal detector to detect Annexin V binding. GFP-negative cells were the UWB1.289 or UWB1.289+BRCA1 cells.
Cav-1 knockdown
siRNA-mediated Cav-1 knockdown was performed using the HiPerFect transfection reagent (Qiagen) as per manufacturer instructions, with minor modifications. Briefly, 1 × 105 cells were seeded in 12-well plates in 350 μL of complete media and were transfected within 30 min of plating. One hundred ng of Cav-1 siRNA (SI00299635, Qiagen) or 100 ng control siRNA (1022076, Qiagen) were mixed with 100 μL of serum-free media and 6 μL of HiPerFect reagent. After vortexing for 30 sec, the mixture was incubated for 15 min at room temperature and then added drop-wise to the freshly seeded cells. Three hours post-transfection, 800 μL of complete media was added to the cells. Cells were harvested after 24 h and plated in co-culture with BJ-1-GFP cells. Cav-1 expression was monitored at 72 h post-transfection.
Immunocytochemistry
Cells were fixed after 2–7 d of culture. Then, the ICC protocol was performed as previously described with minor modifications.Citation31 Briefly, cells were fixed for 30 min at room temperature in 2% para-formaldehyde diluted in PBS, after which they were permeabilized with cold methanol at -20°C for 5 min. The cells were rinsed with PBS with 0.1 mM calcium chloride and 1 mM magnesium chloride (PBS/CM). Then, cells were incubated with NH4Cl in PBS to quench-free aldehyde groups. Rinsing with PBS/CM was followed by blocking with immunofluorescence (IF) buffer (PBS, 1% BSA, 0.1% Tween 20) for 1 h at room temperature. Primary anti-MCT4 and Keratin 8/18 antibodies were incubated in IF buffer for 1 h at room temperature. After washing with IF buffer (3x, 10 min each), cells were incubated for 30 min at room temperature with fluorochrome-conjugated anti-rabbit and anti-guinea pig secondary antibodies diluted in IF buffer. Finally, slides were washed at room temperature with IF buffer (3x, 10 min each), rinsed with PBS/CM and counter-stained with DAPI (10 μg/ml) in PBS and mounted with Prolong Gold anti-fade Reagent.
Confocal microscopy
Images were collected with a Zeiss LSM510 meta confocal system using a 405 nm Diode excitation laser with a band pass filter of 420–480 nm, a 488 nm Argon excitation laser with a band pass filter of 505–550 nm and a 543 nm HeNe excitation laser with a 561–604 nm filter. Images were acquired with a 40x objectives as stated in the figure legends.
Luciferase activity
NIH3T3 fibroblasts stably transfected with an NFκB luciferase reporter (#RC0015, Panomics) were seeded in complete growth media in 24-well plates. For co-culture, 5 × 104 cells were seeded at a fibroblast-to-UWB1.289 or UWB1.289+BRCA1 cell ratio of 5:1. As controls, fibroblasts were plated in mono-culture using the same cell numbers, as in the corresponding co-cultures. After cells attached, i.e., 24 h later, this was considered day 0. Luciferase activity was measured at day 0, 1, 2 and 3, as previously described.Citation55 In brief, cell lysis buffer was added to each well. Then, the ATP mix was added to the lysates to serve as the substrate for the chemiluminescent oxidation-reduction reaction, which leads to light emission and oxy-luciferin generation. A luminometer was used to measure light emitted at 560 nm.
Acknowledgments
U.E.M. was supported by a Young Investigator Award from the Margaret Q. Landenberger Research Foundation. Funds were also contributed by the Margaret Q. Landenberger Research Foundation (to M.P.L.). F.S. was the recipient of a Young Investigator Award from the Breast Cancer Alliance. This work was also supported, in part, by a Centre grant in Manchester from Breakthrough Breast Cancer in the U.K. and an Advanced ERC Grant from the European Research Council.
Disclosure of Potential Conflicts of Interest
No potential conflicts of interest were disclosed.
References
- Siegel R, Naishadham D, Jemal A. Cancer statistics, 2012. CA Cancer J Clin 2012; 62:10 - 29; http://dx.doi.org/10.3322/caac.20138; PMID: 22237781
- Narod SA, Dubé MP, Klijn J, Lubinski J, Lynch HT, Ghadirian P, et al. Oral contraceptives and the risk of breast cancer in BRCA1 and BRCA2 mutation carriers. J Natl Cancer Inst 2002; 94:1773 - 9; http://dx.doi.org/10.1093/jnci/94.23.1773; PMID: 12464649
- Darbary H, Stoler DL, Anderson GR. Family cancer syndromes: inherited deficiencies in systems for the maintenance of genomic integrity. [vii.] Surg Oncol Clin N Am 2009; 18:1 - 17, vii; http://dx.doi.org/10.1016/j.soc.2008.08.001; PMID: 19056039
- Weberpals JI, Clark-Knowles KV, Vanderhyden BC. Sporadic epithelial ovarian cancer: clinical relevance of BRCA1 inhibition in the DNA damage and repair pathway. J Clin Oncol 2008; 26:3259 - 67; http://dx.doi.org/10.1200/JCO.2007.11.3902; PMID: 18591560
- Antoniou A, Pharoah PD, Narod S, Risch HA, Eyfjord JE, Hopper JL, et al. Average risks of breast and ovarian cancer associated with BRCA1 or BRCA2 mutations detected in case Series unselected for family history: a combined analysis of 22 studies. Am J Hum Genet 2003; 72:1117 - 30; http://dx.doi.org/10.1086/375033; PMID: 12677558
- Roy R, Chun J, Powell SN. BRCA1 and BRCA2: different roles in a common pathway of genome protection. Nat Rev Cancer 2012; 12:68 - 78; http://dx.doi.org/10.1038/nrc3181; PMID: 22193408
- Huen MS, Sy SM, Chen J. BRCA1 and its toolbox for the maintenance of genome integrity. Nat Rev Mol Cell Biol 2010; 11:138 - 48; http://dx.doi.org/10.1038/nrm2831; PMID: 20029420
- Foulkes WD. Inherited susceptibility to common cancers. N Engl J Med 2008; 359:2143 - 53; http://dx.doi.org/10.1056/NEJMra0802968; PMID: 19005198
- Rubin SC, Benjamin I, Behbakht K, Takahashi H, Morgan MA, LiVolsi VA, et al. Clinical and pathological features of ovarian cancer in women with germ-line mutations of BRCA1. N Engl J Med 1996; 335:1413 - 6; http://dx.doi.org/10.1056/NEJM199611073351901; PMID: 8875917
- Boyd J, Sonoda Y, Federici MG, Bogomolniy F, Rhei E, Maresco DL, et al. Clinicopathologic features of BRCA-linked and sporadic ovarian cancer. JAMA 2000; 283:2260 - 5; http://dx.doi.org/10.1001/jama.283.17.2260; PMID: 10807385
- Majdak EJ, De Bock GH, Brozek I, Perkowska M, Ochman K, Debniak J, et al. Prevalence and clinical correlations of BRCA1/BRCA2 unclassified variant carriers among unselected primary ovarian cancer cases - preliminary report. Eur J Cancer 2005; 41:143 - 50; http://dx.doi.org/10.1016/j.ejca.2004.10.011; PMID: 15617999
- Quinn JE, Carser JE, James CR, Kennedy RD, Harkin DP. BRCA1 and implications for response to chemotherapy in ovarian cancer. Gynecol Oncol 2009; 113:134 - 42; http://dx.doi.org/10.1016/j.ygyno.2008.12.015; PMID: 19168207
- Ben David Y, Chetrit A, Hirsh-Yechezkel G, Friedman E, Beck BD, Beller U, et al, National Israeli Study of Ovarian Cancer. Effect of BRCA mutations on the length of survival in epithelial ovarian tumors. J Clin Oncol 2002; 20:463 - 6; http://dx.doi.org/10.1200/JCO.20.2.463; PMID: 11786575
- Cass I, Baldwin RL, Varkey T, Moslehi R, Narod SA, Karlan BY. Improved survival in women with BRCA-associated ovarian carcinoma. Cancer 2003; 97:2187 - 95; http://dx.doi.org/10.1002/cncr.11310; PMID: 12712470
- Byrski T, Gronwald J, Huzarski T, Grzybowska E, Budryk M, Stawicka M, et al, Polish Hereditary Breast Cancer Consortium. Response to neo-adjuvant chemotherapy in women with BRCA1-positive breast cancers. Breast Cancer Res Treat 2008; 108:289 - 96; http://dx.doi.org/10.1007/s10549-007-9600-1; PMID: 17492376
- Easton DF, Deffenbaugh AM, Pruss D, Frye C, Wenstrup RJ, Allen-Brady K, et al. A systematic genetic assessment of 1,433 sequence variants of unknown clinical significance in the BRCA1 and BRCA2 breast cancer-predisposition genes. Am J Hum Genet 2007; 81:873 - 83; http://dx.doi.org/10.1086/521032; PMID: 17924331
- Yoshikawa K, Honda K, Inamoto T, Shinohara H, Yamauchi A, Suga K, et al. Reduction of BRCA1 protein expression in Japanese sporadic breast carcinomas and its frequent loss in BRCA1-associated cases. Clin Cancer Res 1999; 5:1249 - 61; PMID: 10389907
- Wilson CA, Ramos L, Villaseñor MR, Anders KH, Press MF, Clarke K, et al. Localization of human BRCA1 and its loss in high-grade, non-inherited breast carcinomas. Nat Genet 1999; 21:236 - 40; http://dx.doi.org/10.1038/6029; PMID: 9988281
- Thrall M, Gallion HH, Kryscio R, Kapali M, Armstrong DK, DeLoia JA. BRCA1 expression in a large series of sporadic ovarian carcinomas: a Gynecologic Oncology Group study. Int J Gynecol Cancer 2006; 16:Suppl 1 166 - 71; http://dx.doi.org/10.1111/j.1525-1438.2006.00504.x; PMID: 16515585
- Turner N, Tutt A, Ashworth A. Hallmarks of ‘BRCAness’ in sporadic cancers. Nat Rev Cancer 2004; 4:814 - 9; http://dx.doi.org/10.1038/nrc1457; PMID: 15510162
- Cancer Genome Atlas Research Network. Integrated genomic analyses of ovarian carcinoma. Nature 2011; 474:609 - 15; http://dx.doi.org/10.1038/nature10166; PMID: 21720365
- Hilton JL, Geisler JP, Rathe JA, Hattermann-Zogg MA, DeYoung B, Buller RE. Inactivation of BRCA1 and BRCA2 in ovarian cancer. J Natl Cancer Inst 2002; 94:1396 - 406; http://dx.doi.org/10.1093/jnci/94.18.1396; PMID: 12237285
- Fong MY, McDunn J, Kakar SS. Identification of metabolites in the normal ovary and their transformation in primary and metastatic ovarian cancer. PLoS One 2011; 6:e19963; http://dx.doi.org/10.1371/journal.pone.0019963; PMID: 21625518
- Karihtala P, Soini Y, Vaskivuo L, Bloigu R, Puistola U. DNA adduct 8-hydroxydeoxyguanosine, a novel putative marker of prognostic significance in ovarian carcinoma. Int J Gynecol Cancer 2009; 19:1047 - 51; http://dx.doi.org/10.1111/IGC.0b013e3181ad0f0d; PMID: 19820366
- Esteve JM, Armengod ME, Knecht E. BRCA1 negatively regulates formation of autophagic vacuoles in MCF-7 breast cancer cells. Exp Cell Res 2010; 316:2618 - 29; http://dx.doi.org/10.1016/j.yexcr.2010.06.019; PMID: 20599945
- Bae I, Fan S, Meng Q, Rih JK, Kim HJ, Kang HJ, et al. BRCA1 induces antioxidant gene expression and resistance to oxidative stress. Cancer Res 2004; 64:7893 - 909; http://dx.doi.org/10.1158/0008-5472.CAN-04-1119; PMID: 15520196
- Saha T, Rih JK, Rosen EM. BRCA1 down-regulates cellular levels of reactive oxygen species. FEBS Lett 2009; 583:1535 - 43; http://dx.doi.org/10.1016/j.febslet.2009.04.005; PMID: 19364506
- Rodriguez H, Jaruga P, Leber D, Nyaga SG, Evans MK, Dizdaroglu M. Lymphoblasts of women with BRCA1 mutations are deficient in cellular repair of 8,5′-Cyclopurine-2′-deoxynucleosides and 8-hydroxy-2′-deoxyguanosine. Biochemistry 2007; 46:2488 - 96; http://dx.doi.org/10.1021/bi062022p; PMID: 17288454
- Dziaman T, Huzarski T, Gackowski D, Rozalski R, Siomek A, Szpila A, et al. Elevated level of 8-oxo-7,8-dihydro-2′-deoxyguanosine in leukocytes of BRCA1 mutation carriers compared to healthy controls. Int J Cancer 2009; 125:2209 - 13; http://dx.doi.org/10.1002/ijc.24600; PMID: 19623658
- Pavlides S, Whitaker-Menezes D, Castello-Cros R, Flomenberg N, Witkiewicz AK, Frank PG, et al. The reverse Warburg effect: aerobic glycolysis in cancer associated fibroblasts and the tumor stroma. Cell Cycle 2009; 8:3984 - 4001; http://dx.doi.org/10.4161/cc.8.23.10238; PMID: 19923890
- Whitaker-Menezes D, Martinez-Outschoorn UE, Lin Z, Ertel A, Flomenberg N, Witkiewicz AK, et al. Evidence for a stromal-epithelial “lactate shuttle” in human tumors: MCT4 is a marker of oxidative stress in cancer-associated fibroblasts. Cell Cycle 2011; 10:1772 - 83; http://dx.doi.org/10.4161/cc.10.11.15659; PMID: 21558814
- Nieman KM, Kenny HA, Penicka CV, Ladanyi A, Buell-Gutbrod R, Zillhardt MR, et al. Adipocytes promote ovarian cancer metastasis and provide energy for rapid tumor growth. Nat Med 2011; 17:1498 - 503; http://dx.doi.org/10.1038/nm.2492; PMID: 22037646
- Koukourakis MI, Giatromanolaki A, Harris AL, Sivridis E. Comparison of metabolic pathways between cancer cells and stromal cells in colorectal carcinomas: a metabolic survival role for tumor-associated stroma. Cancer Res 2006; 66:632 - 7; http://dx.doi.org/10.1158/0008-5472.CAN-05-3260; PMID: 16423989
- Fiaschi T, Marini A, Giannoni E, Taddei ML, Gandellini P, De Donatis A, et al. Reciprocal Metabolic Reprogramming through Lactate Shuttle Coordinately Influences Tumor-Stroma Interplay. Cancer Res 2012; http://dx.doi.org/10.1158/0008-5472.CAN-12-1949; PMID: 22850421
- Giatromanolaki A, Koukourakis MI, Koutsopoulos A, Mendrinos S, Sivridis E. The metabolic interactions between tumor cells and tumor-associated stroma (TAS) in prostatic cancer. Cancer Biol Ther 2012; 13:13; PMID: 22895074
- Witkiewicz AK, Dasgupta A, Sotgia F, Mercier I, Pestell RG, Sabel M, et al. An absence of stromal caveolin-1 expression predicts early tumor recurrence and poor clinical outcome in human breast cancers. Am J Pathol 2009; 174:2023 - 34; http://dx.doi.org/10.2353/ajpath.2009.080873; PMID: 19411448
- Witkiewicz AK, Whitaker-Menezes D, Dasgupta A, Philp NJ, Lin Z, Gandara R, et al. Using the “reverse Warburg effect” to identify high-risk breast cancer patients: stromal MCT4 predicts poor clinical outcome in triple-negative breast cancers. Cell Cycle 2012; 11:1108 - 17; http://dx.doi.org/10.4161/cc.11.6.19530; PMID: 22313602
- Wu KN, Queenan M, Brody JR, Potoczek M, Sotgia F, Lisanti MP, et al. Loss of stromal caveolin-1 expression in malignant melanoma metastases predicts poor survival. Cell Cycle 2011; 10:4250 - 5; http://dx.doi.org/10.4161/cc.10.24.18551; PMID: 22134245
- Di Vizio D, Morello M, Sotgia F, Pestell RG, Freeman MR, Lisanti MP. An absence of stromal caveolin-1 is associated with advanced prostate cancer, metastatic disease and epithelial Akt activation. Cell Cycle 2009; 8:2420 - 4; http://dx.doi.org/10.4161/cc.8.15.9116; PMID: 19556867
- Xia C, Meng Q, Liu LZ, Rojanasakul Y, Wang XR, Jiang BH. Reactive oxygen species regulate angiogenesis and tumor growth through vascular endothelial growth factor. Cancer Res 2007; 67:10823 - 30; http://dx.doi.org/10.1158/0008-5472.CAN-07-0783; PMID: 18006827
- Saunders JA, Rogers LC, Klomsiri C, Poole LB, Daniel LW. Reactive oxygen species mediate lysophosphatidic acid induced signaling in ovarian cancer cells. Free Radic Biol Med 2010; 49:2058 - 67; http://dx.doi.org/10.1016/j.freeradbiomed.2010.10.663; PMID: 20934509
- Ralph SJ, Rodríguez-Enríquez S, Neuzil J, Saavedra E, Moreno-Sánchez R. The causes of cancer revisited: “mitochondrial malignancy” and ROS-induced oncogenic transformation - why mitochondria are targets for cancer therapy. Mol Aspects Med 2010; 31:145 - 70; http://dx.doi.org/10.1016/j.mam.2010.02.008; PMID: 20206201
- Cui X. Reactive oxygen species: the achilles’ heel of cancer cells?. Antioxid Redox Signal 2012; 16:1212 - 4; http://dx.doi.org/10.1089/ars.2012.4532; PMID: 22304673
- Enns L, Ladiges W. Mitochondrial redox signaling and cancer invasiveness. J Bioenerg Biomembr 2012; http://dx.doi.org/10.1007/s10863-012-9467-7; PMID: 22886605
- Martinez-Outschoorn UE, Balliet RM, Rivadeneira DB, Chiavarina B, Pavlides S, Wang C, et al. Oxidative stress in cancer associated fibroblasts drives tumor-stroma co-evolution: A new paradigm for understanding tumor metabolism, the field effect and genomic instability in cancer cells. Cell Cycle 2010; 9:3256 - 76; http://dx.doi.org/10.4161/cc.9.16.12553; PMID: 20814239
- Martinez-Outschoorn UE, Lin Z, Trimmer C, Flomenberg N, Wang C, Pavlides S, et al. Cancer cells metabolically “fertilize” the tumor microenvironment with hydrogen peroxide, driving the Warburg effect: implications for PET imaging of human tumors. Cell Cycle 2011; 10:2504 - 20; http://dx.doi.org/10.4161/cc.10.15.16585; PMID: 21778829
- Ohkouchi S, Block GJ, Katsha AM, Kanehira M, Ebina M, Kikuchi T, et al. Mesenchymal stromal cells protect cancer cells from ROS-induced apoptosis and enhance the Warburg effect by secreting STC1. Mol Ther 2012; 20:417 - 23; http://dx.doi.org/10.1038/mt.2011.259; PMID: 22146344
- Pavlides S, Vera I, Gandara R, Sneddon S, Pestell RG, Mercier I, et al. Warburg meets autophagy: cancer-associated fibroblasts accelerate tumor growth and metastasis via oxidative stress, mitophagy, and aerobic glycolysis. Antioxid Redox Signal 2012; 16:1264 - 84; http://dx.doi.org/10.1089/ars.2011.4243; PMID: 21883043
- Sivridis E, Giatromanolaki A, Koukourakis MI. Proliferating fibroblasts at the invading tumour edge of colorectal adenocarcinomas are associated with endogenous markers of hypoxia, acidity, and oxidative stress. J Clin Pathol 2005; 58:1033 - 8; http://dx.doi.org/10.1136/jcp.2005.026260; PMID: 16189147
- Salazar H, Godwin AK, Daly MB, Laub PB, Hogan WM, Rosenblum N, et al. Microscopic benign and invasive malignant neoplasms and a cancer-prone phenotype in prophylactic oophorectomies. J Natl Cancer Inst 1996; 88:1810 - 20; http://dx.doi.org/10.1093/jnci/88.24.1810; PMID: 8961970
- DelloRusso C, Welcsh PL, Wang W, Garcia RL, King MC, Swisher EM. Functional characterization of a novel BRCA1-null ovarian cancer cell line in response to ionizing radiation. Mol Cancer Res 2007; 5:35 - 45; http://dx.doi.org/10.1158/1541-7786.MCR-06-0234; PMID: 17259345
- Pilch DR, Sedelnikova OA, Redon C, Celeste A, Nussenzweig A, Bonner WM. Characteristics of gamma-H2AX foci at DNA double-strand breaks sites. Biochem Cell Biol 2003; 81:123 - 9; http://dx.doi.org/10.1139/o03-042; PMID: 12897845
- Ullah MS, Davies AJ, Halestrap AP. The plasma membrane lactate transporter MCT4, but not MCT1, is up-regulated by hypoxia through a HIF-1alpha-dependent mechanism. J Biol Chem 2006; 281:9030 - 7; http://dx.doi.org/10.1074/jbc.M511397200; PMID: 16452478
- Bubici C, Papa S, Dean K, Franzoso G. Mutual cross-talk between reactive oxygen species and nuclear factor-kappa B: molecular basis and biological significance. Oncogene 2006; 25:6731 - 48; http://dx.doi.org/10.1038/sj.onc.1209936; PMID: 17072325
- Martinez-Outschoorn UE, Trimmer C, Lin Z, Whitaker-Menezes D, Chiavarina B, Zhou J, et al. Autophagy in cancer associated fibroblasts promotes tumor cell survival: Role of hypoxia, HIF1 induction and NFκB activation in the tumor stromal microenvironment. Cell Cycle 2010; 9:3515 - 33; http://dx.doi.org/10.4161/cc.9.17.12928; PMID: 20855962
- Sotgia F, Martinez-Outschoorn UE, Howell A, Pestell RG, Pavlides S, Lisanti MP. Caveolin-1 and cancer metabolism in the tumor microenvironment: markers, models, and mechanisms. Annu Rev Pathol 2012; 7:423 - 67; http://dx.doi.org/10.1146/annurev-pathol-011811-120856; PMID: 22077552
- Simpkins SA, Hanby AM, Holliday DL, Speirs V. Clinical and functional significance of loss of caveolin-1 expression in breast cancer-associated fibroblasts. J Pathol 2012; 227:490 - 8; http://dx.doi.org/10.1002/path.4034; PMID: 22488553
- Martinez-Outschoorn UE, Pavlides S, Whitaker-Menezes D, Daumer KM, Milliman JN, Chiavarina B, et al. Tumor cells induce the cancer associated fibroblast phenotype via caveolin-1 degradation: implications for breast cancer and DCIS therapy with autophagy inhibitors. Cell Cycle 2010; 9:2423 - 33; http://dx.doi.org/10.4161/cc.9.12.12048; PMID: 20562526
- Cantuaria G, Magalhaes A, Penalver M, Angioli R, Braunschweiger P, Gomez-Marin O, et al. Expression of GLUT-1 glucose transporter in borderline and malignant epithelial tumors of the ovary. Gynecol Oncol 2000; 79:33 - 7; http://dx.doi.org/10.1006/gyno.2000.5910; PMID: 11006027
- Yasuda M, Miyazawa M, Fujita M, Kajiwara H, Iida T, Hirasawa T, et al. Expression of hypoxia inducible factor-1alpha (HIF-1alpha) and glucose transporter-1 (GLUT-1) in ovarian adenocarcinomas: difference in hypoxic status depending on histological character. Oncol Rep 2008; 19:111 - 6; PMID: 18097583
- Yoshida Y, Kurokawa T, Tsujikawa T, Okazawa H, Kotsuji F. Positron emission tomography in ovarian cancer: 18F-deoxy-glucose and 16alpha-18F-fluoro-17beta-estradiol PET. J Ovarian Res 2009; 2:7; http://dx.doi.org/10.1186/1757-2215-2-7; PMID: 19527525
- Nanni C, Rubello D, Farsad M, De Iaco P, Sansovini M, Erba P, et al. (18)F-FDG PET/CT in the evaluation of recurrent ovarian cancer: a prospective study on forty-one patients. Eur J Surg Oncol 2005; 31:792 - 7; http://dx.doi.org/10.1016/j.ejso.2005.02.029; PMID: 15893908
- Airley R, Evans A, Mobasheri A, Hewitt SM. Glucose transporter Glut-1 is detectable in peri-necrotic regions in many human tumor types but not normal tissues: Study using tissue microarrays. Ann Anat 2010; 192:133 - 8; http://dx.doi.org/10.1016/j.aanat.2010.03.001; PMID: 20395120
- Migneco G, Whitaker-Menezes D, Chiavarina B, Castello-Cros R, Pavlides S, Pestell RG, et al. Glycolytic cancer associated fibroblasts promote breast cancer tumor growth, without a measurable increase in angiogenesis: evidence for stromal-epithelial metabolic coupling. Cell Cycle 2010; 9:2412 - 22; http://dx.doi.org/10.4161/cc.9.12.11989; PMID: 20562527
- Sampson N, Koziel R, Zenzmaier C, Bubendorf L, Plas E, Jansen-Dürr P, et al. ROS signaling by NOX4 drives fibroblast-to-myofibroblast differentiation in the diseased prostatic stroma. Mol Endocrinol 2011; 25:503 - 15; http://dx.doi.org/10.1210/me.2010-0340; PMID: 21273445
- Yao Q, Qu X, Yang Q, Good DA, Dai S, Kong B, et al. Blockage of transdifferentiation from fibroblast to myofibroblast in experimental ovarian cancer models. Mol Cancer 2009; 8:78; http://dx.doi.org/10.1186/1476-4598-8-78; PMID: 19781102
- Lynch HT, Casey MJ, Snyder CL, Bewtra C, Lynch JF, Butts M, et al. Hereditary ovarian carcinoma: heterogeneity, molecular genetics, pathology, and management. Mol Oncol 2009; 3:97 - 137; http://dx.doi.org/10.1016/j.molonc.2009.02.004; PMID: 19383374
- Schrag D, Kuntz KM, Garber JE, Weeks JC. Life expectancy gains from cancer prevention strategies for women with breast cancer and BRCA1 or BRCA2 mutations. JAMA 2000; 283:617 - 24; http://dx.doi.org/10.1001/jama.283.5.617; PMID: 10665701
- Anderson K, Jacobson JS, Heitjan DF, Zivin JG, Hershman D, Neugut AI, et al. Cost-effectiveness of preventive strategies for women with a BRCA1 or a BRCA2 mutation. Ann Intern Med 2006; 144:397 - 406; PMID: 16549852
- Kauff ND, Domchek SM, Friebel TM, Robson ME, Lee J, Garber JE, et al. Risk-reducing salpingo-oophorectomy for the prevention of BRCA1- and BRCA2-associated breast and gynecologic cancer: a multicenter, prospective study. J Clin Oncol 2008; 26:1331 - 7; http://dx.doi.org/10.1200/JCO.2007.13.9626; PMID: 18268356
- Kang HJ, Hong YB, Kim HJ, Wang A, Bae I. Bioactive food components prevent carcinogenic stress via Nrf2 activation in BRCA1 deficient breast epithelial cells. Toxicol Lett 2012; 209:154 - 60; http://dx.doi.org/10.1016/j.toxlet.2011.12.002; PMID: 22192953
- Dziaman T, Huzarski T, Gackowski D, Rozalski R, Siomek A, Szpila A, et al. Selenium supplementation reduced oxidative DNA damage in adnexectomized BRCA1 mutations carriers. Cancer Epidemiol Biomarkers Prev 2009; 18:2923 - 8; http://dx.doi.org/10.1158/1055-9965.EPI-09-0529; PMID: 19843683
- Kowalska E, Narod SA, Huzarski T, Zajaczek S, Huzarska J, Gorski B, et al. Increased rates of chromosome breakage in BRCA1 carriers are normalized by oral selenium supplementation. Cancer Epidemiol Biomarkers Prev 2005; 14:1302 - 6; http://dx.doi.org/10.1158/1055-9965.EPI-03-0448; PMID: 15894690
- Gifkins D, Olson SH, Paddock L, King M, Demissie K, Lu SE, et al. Total and individual antioxidant intake and risk of epithelial ovarian cancer. BMC Cancer 2012; 12:211; http://dx.doi.org/10.1186/1471-2407-12-211; PMID: 22656322
- Goodman M, Bostick RM, Kucuk O, Jones DP. Clinical trials of antioxidants as cancer prevention agents: past, present, and future. Free Radic Biol Med 2011; 51:1068 - 84; http://dx.doi.org/10.1016/j.freeradbiomed.2011.05.018; PMID: 21683786
- Foulkes WD, Smith IE, Reis-Filho JS. Triple-negative breast cancer. N Engl J Med 2010; 363:1938 - 48; http://dx.doi.org/10.1056/NEJMra1001389; PMID: 21067385
- Baldwin RL, Nemeth E, Tran H, Shvartsman H, Cass I, Narod S, et al. BRCA1 promoter region hypermethylation in ovarian carcinoma: a population-based study. Cancer Res 2000; 60:5329 - 33; PMID: 11034065
- Russell PA, Pharoah PD, De Foy K, Ramus SJ, Symmonds I, Wilson A, et al. Frequent loss of BRCA1 mRNA and protein expression in sporadic ovarian cancers. Int J Cancer 2000; 87:317 - 21; http://dx.doi.org/10.1002/1097-0215(20000801)87:3<317::AID-IJC2>3.0.CO;2-B; PMID: 10897034
- Hennessy BT, Timms KM, Carey MS, Gutin A, Meyer LA, Flake DD 2nd, et al. Somatic mutations in BRCA1 and BRCA2 could expand the number of patients that benefit from poly (ADP ribose) polymerase inhibitors in ovarian cancer. J Clin Oncol 2010; 28:3570 - 6; http://dx.doi.org/10.1200/JCO.2009.27.2997; PMID: 20606085
- Gillet JP, Calcagno AM, Varma S, Marino M, Green LJ, Vora MI, et al. Redefining the relevance of established cancer cell lines to the study of mechanisms of clinical anti-cancer drug resistance. Proc Natl Acad Sci USA 2011; 108:18708 - 13; http://dx.doi.org/10.1073/pnas.1111840108; PMID: 22068913
- Zietarska M, Maugard CM, Filali-Mouhim A, Alam-Fahmy M, Tonin PN, Provencher DM, et al. Molecular description of a 3D in vitro model for the study of epithelial ovarian cancer (EOC). Mol Carcinog 2007; 46:872 - 85; http://dx.doi.org/10.1002/mc.20315; PMID: 17455221
- Ramus SJ, Bobrow LG, Pharoah PD, Finnigan DS, Fishman A, Altaras M, et al. Increased frequency of TP53 mutations in BRCA1 and BRCA2 ovarian tumours. Genes Chromosomes Cancer 1999; 25:91 - 6; http://dx.doi.org/10.1002/(SICI)1098-2264(199906)25:2<91::AID-GCC3>3.0.CO;2-5; PMID: 10337991
- Linger RJ, Kruk PA. BRCA1 16 years later: risk-associated BRCA1 mutations and their functional implications. FEBS J 2010; 277:3086 - 96; http://dx.doi.org/10.1111/j.1742-4658.2010.07735.x; PMID: 20608970
- Martinez-Outschoorn UE, Lin Z, Trimmer C, Flomenberg N, Wang C, Pavlides S, et al. Cancer cells metabolically “fertilize” the tumor microenvironment with hydrogen peroxide, driving the Warburg effect: implications for PET imaging of human tumors. Cell Cycle 2011; 10:2504 - 20; http://dx.doi.org/10.4161/cc.10.15.16585; PMID: 21778829
- Whitaker-Menezes D, Martinez-Outschoorn UE, Flomenberg N, Birbe RC, Witkiewicz AK, Howell A, et al. Hyperactivation of oxidative mitochondrial metabolism in epithelial cancer cells in situ: visualizing the therapeutic effects of metformin in tumor tissue. Cell Cycle 2011; 10:4047 - 64; http://dx.doi.org/10.4161/cc.10.23.18151; PMID: 22134189
- Cannistra SA. Cancer of the ovary. N Engl J Med 2004; 351:2519 - 29; http://dx.doi.org/10.1056/NEJMra041842; PMID: 15590954
- Furlan D, Carnevali I, Marcomini B, Cerutti R, Dainese E, Capella C, et al. The high frequency of de novo promoter methylation in synchronous primary endometrial and ovarian carcinomas. Clin Cancer Res 2006; 12:3329 - 36; http://dx.doi.org/10.1158/1078-0432.CCR-05-2679; PMID: 16740754
- Lu KH, Bell DA, Welch WR, Berkowitz RS, Mok SC. Evidence for the multifocal origin of bilateral and advanced human serous borderline ovarian tumors. Cancer Res 1998; 58:2328 - 30; PMID: 9622067
- Gu J, Roth LM, Younger C, Michael H, Abdul-Karim FW, Zhang S, et al. Molecular evidence for the independent origin of extra-ovarian papillary serous tumors of low malignant potential. J Natl Cancer Inst 2001; 93:1147 - 52; http://dx.doi.org/10.1093/jnci/93.15.1147; PMID: 11481386
- Wang J, Lin L, Parkash V, Schwartz PE, Lauchlan SC, Zheng W. Quantitative analysis of follicle-stimulating hormone receptor in ovarian epithelial tumors: a novel approach to explain the field effect of ovarian cancer development in secondary mullerian systems. Int J Cancer 2003; 103:328 - 34; http://dx.doi.org/10.1002/ijc.10848; PMID: 12471615
- Buller RE, Skilling JS, Sood AK, Plaxe S, Baergen RN, Lager DJ. Field cancerization: why late “recurrent” ovarian cancer is not recurrent. Am J Obstet Gynecol 1998; 178:641 - 9; http://dx.doi.org/10.1016/S0002-9378(98)70473-9; PMID: 9579425
- Slaughter DP. Multicentric origin of intraoral carcinoma. Surgery 1946; 20:133 - 46; PMID: 20992244
- Braakhuis BJ, Tabor MP, Kummer JA, Leemans CR, Brakenhoff RH. A genetic explanation of Slaughter’s concept of field cancerization: evidence and clinical implications. Cancer Res 2003; 63:1727 - 30; PMID: 12702551
- Dakubo GD, Jakupciak JP, Birch-Machin MA, Parr RL. Clinical implications and utility of field cancerization. Cancer Cell Int 2007; 7:2; http://dx.doi.org/10.1186/1475-2867-7-2; PMID: 17362521
- Senthil K, Aranganathan S, Nalini N. Evidence of oxidative stress in the circulation of ovarian cancer patients. Clin Chim Acta 2004; 339:27 - 32; http://dx.doi.org/10.1016/j.cccn.2003.08.017; PMID: 14687890
- Gallagher SM, Castorino JJ, Wang D, Philp NJ. Monocarboxylate transporter 4 regulates maturation and trafficking of CD147 to the plasma membrane in the metastatic breast cancer cell line MDA-MB-231. Cancer Res 2007; 67:4182 - 9; http://dx.doi.org/10.1158/0008-5472.CAN-06-3184; PMID: 17483329
- Martinez-Outschoorn UE, Goldberg A, Lin Z, Ko YH, Flomenberg N, Wang C, et al. Anti-estrogen resistance in breast cancer is induced by the tumor microenvironment and can be overcome by inhibiting mitochondrial function in epithelial cancer cells. Cancer Biol Ther 2011; 12:924 - 38; http://dx.doi.org/10.4161/cbt.12.10.17780; PMID: 22041887