Abstract
Oocytes undergo extremely asymmetric divisions in terms of size. Coordinating spindle assembly and positioning in the absence of canonical centrosomes appears to be a challenge for oocytes, which divide with an elevated rate of errors in chromosome segregation. Here we highlight recent work on the characteristics of oocyte meiotic divisions, giving special emphasis on MTOCs clustering, generation of aneuploidy, and cortex softening, properties shared by cancer cells. While the loss of canonical centrosomes in oocytes might favor the asymmetry in size of meiotic divisions by reducing the distance between spindle poles and the cortex, we propose that this acentrosomal pathway might also render meiotic spindles less robust and, so, be responsible for the high error rate of female meiosis.
Keywords: :
Asymmetric Division in Mitotic Cells
The development of any given multicellular organism requires a fine equilibrium between proliferation and differentiation in order to promote cell diversity. This equilibrium can be regulated by the ratio between symmetric vs. asymmetric cell divisions. Whereas symmetric divisions are conservative and induce the formation of daughter cells identical to their mother, asymmetric divisions allow a mother cell to generate 2 daughter cells of distinct fates. In addition to their role in development, cell divisions are required for tissue homeostasis in adults, as stem cells can generate differentiated cells constituting the tissue via asymmetric divisions and, via symmetric divisions, undergo self-renewal, thus preserving their pool. In addition to their importance during normal development and adult life, asymmetric cell divisions can be involved in a variety of pathologies such as tumorigenesis, neurodevelopmental disorders, or polycystic kidney disease. Notably, the loss of asymmetric divisions following mutation of several tumor suppressors genes involved in mitotic spindle orientation, such as APC, Lgl, Brat, or p63, is associated with tumor initiation and progression, in particular in the brain and colon (for a review, see refs. Citation1 and Citation2). The positioning of the spindle along the polarity axis instructs the production of either symmetric or asymmetric division. Spindle positioning indeed specifies the geometry of cell division (for a review see ref. Citation3). In most animal cells, centrosomes act as dominant microtubule-organizing centers and establish both the mitotic spindle axisCitation4 as well as indirectly control its orientation within the cell (; for a review see ref. Citation5). Canonical centrosomes are composed of a pair of centrioles that recruit their surrounding pericentriolar material (PCM) necessary for microtubule nucleation. Mitotic spindle positioning depends on complexes of Dynein–Dynactin anchored on one side at the cell cortex and associated on the other side with plus-ends of astral microtubules that emanate from the 2 centrosomes. These complexes containing the minus-end-directed microtubule motor Dynein, which walks along astral microtubules, are therefore pulling on the 2 spindle poles (for a review see ref. Citation6). In Drosophila, cortical adaptors such as Mud display a polarized localization following their association with cortical cues, such as Pins. Mud associates with Dynein–Dynactin complexes, resulting in unequal pulling force at one spindle pole, since Mud is asymmetrically localized in the cell (for a review see ref. Citation2). To generate efficient Dynein–Dynactin-dependent forces while preventing membrane invagination, the cell cortex stiffens at the beginning of mitosis. This stiffening is established following an increase in cortical tension, which leads to cell rounding (). A rigid cell cortex is thus essential for proper mitotic spindle positioning. The rigidity of the cortex, mediated by changes in the actin cytoskeleton at M-phase entry, also influences spindle morphogenesis and acts as an interface between the spindle and the cell environment (for a review see refs. Citation7 and Citation8).
Asymmetric Division in Oocytes
Changes in the actin cytoskeleton are also instrumental during mouse female meiosis and are the main driving force in the control of meiotic spindle positioning, required in oocytes to produce extremely asymmetric divisions. Indeed, during female meiosis, 2 highly asymmetric divisions without an intermediate replicative phase produce a large functional haploid female gamete, the oocyte, and 2 small degenerating polar bodies (). The asymmetry in size and fate of those divisions is important for the production of a unique cell capable of being fertilized and preserving most of the maternal stores that will sustain embryo developmentCitation9 (for a review see ref. Citation10). The asymmetry established during mouse oocyte divisions results mainly from 2 events: off-center positioning of the meiotic spindle and chromatin-induced polarization of the cortex (for a review see ref. Citation11). Hence, whereas in mitotic cells, cortical cues influence spindle positioning to produce either symmetric or asymmetric divisions, in mouse female meiosis, cortical cues and cell polarity are both defined as a downstream consequence of spindle positioning. In mammals, oocytes arrest in prophase I of meiosis I in the ovaries with a slightly off-centered nucleus and no apparent sign of polarization, harboring both a uniform cytoplasm and cortex.Citation12,Citation13 The resumption of meiosis will be triggered periodically by the LH (luteinizing hormone) surge after puberty. Meiosis resumption and exit from the block in prophase I is evidenced by nuclear envelope breakdown (NEBD). It is immediately followed by the formation of the first meiotic spindle at the exact location where the nucleus was, more or less in the center of the cell (). Most female meiotic spindles do not have centrioles and, thus, lack canonical centrosomes.Citation14 Instead, microtubules are nucleated by microtubule-organizing centers (MTOCs) composed only of pericentriolar material (PCM). In mouse and human oocytes, centrioles are lost at the beginning of follicle growth at puberty, imposing very peculiar modes of spindle morphogenesis and spindle positioning. In mouse oocytes, meiotic spindle axis forms randomly and is not influenced by cortical cues.Citation15 Late in meiosis I, the spindle migrates along its long axis toward the closest cortexCitation15,Citation16 (). Since the meiotic spindle axis forms randomly, there is no predetermined direction of spindle migration. At the end of this migration process, the chromosomes are close to the cortex of the oocyte, and their Ran GTP-associated gradient specifies a cortical region above them where the fist polar body will be extruded.Citation17,Citation18 This cortical region lacks microvilli, is enriched in actin filaments, and accumulates activated Rac-GTP;Citation13,Citation19 after this specification the oocyte is polarized. Since oocytes lack canonical centrosomes, they also lack astral microtubules. Therefore their positioning does not depend on microtubules, as in mitosis, () but depends on actin filaments.Citation15,Citation20 Once the spindle reaches the cortex, anaphase takes place, with the segregation of homologous chromosomes and the formation of the first polar body. The meiosis II spindle reforms directly under the cortex and is maintained there by actin-mediated cytoplasmic streaming.Citation21 Mouse oocytes, like the majority of vertebrate oocytes, arrest in metaphase II (). Fertilization will trigger second anaphase and meiosis exit, the emission of a second polar body via another asymmetric division and will lead to embryo formation and development.
Mouse Oocyte, a Paradigm of Cancer Cell
At least 10% of human pregnancies end up in the formation of aneuploid embryos (gain or loss of a whole chromosome—trisomy or monosomy). Aneuploidy frequently results from non-disjunction events that take place in the oocyte. The increase in maternal age further contributes to the occurrence of aneuploidy, a crucial phenomenom for modern societies, where women tend to post-pone child bearing.Citation22 Chromosome segregation in female meiosis is unusual in that meiotic spindle poles do not have centrioles, are not anchored to the cortex via astral microtubules, and therefore lack canonical centrosomes, imposing modes of spindle assembly and positioning that might be responsible for the high error rate of female meiosis. In that regard, oocytes share some common features with cancer cells, namely a certain degree of aneuploidy, the ability to sort and cluster MTOCs, and the importance of cortical softening for the division.
Cancer cells are aneuploid, gaining or losing whole chromosome sets. Even if the link between aneuploidy and tumorigenesis is far from being clear at present, it appears that cancer cells seem to be able to cope with high rates of aneuploidy and keep proliferating. Surprisingly, oocytes and early embryos also cope with a certain degree of aneuploidy. Meiosis I in human females is error-prone and is a leading cause of the occurrence of trisomies as well as other congenital defects due to the formation of aneuploid embryos.Citation23,Citation24 In mitosis, the spindle assembly checkpoint (SAC) is essential to ensure accurate chromosome segregation by postponing anaphase onset until all the chromosomes are under tension, attached and correctly aligned onto the mitotic spindle. As a result, the SAC prevents chromosome mis-segregation and aneuploidy, and its dysfunction is implicated in tumorigenesis (for a review see ref. Citation25). The SAC functions by preventing an ubiquitin ligase called the anaphase-promoting complex/cyclosome (APC/C) from ubiquitinating proteins whose degradation is required for anaphase onset and mitotic exit, namely Securin and Cyclin B (for a review see ref. Citation26). In mitosis, a single unoccupied kinetochore can generate a Mad2-based diffusible “wait-anaphase” signalCitation27 sufficient to inhibit the APC/C. The SAC does act during meiosis I in mouse oocytes to ensure successful attachment of the kinetochores of homologously paired bivalent chromosomes.Citation28-Citation31 However, despite being present and functional in meiosis I, it does not prevent errors from happening at a high rate. It has been recently established that during mammalian meiosis I, the SAC has a surprisingly low threshold for satisfaction.Citation32-Citation35 In mitosis, Mad2 is displaced from the chromosomes when they are bi-oriented at the metaphase plate via kinetochore-microtubule attachment. In meiosis I, Mad2 is lost from kinetochores 3 to 4 h before anaphase, even when all K-fibers are not assembled and in the presence of chromosomes that have not become aligned at the metaphase plate and that are not bi-oriented.Citation33,Citation34 These few free kinetochores or erroneous attachments of bivalent kinetochores are not able to generate a sufficient SAC “wait-anaphase” signal to inhibit the APC/C, but instead the SAC is silenced, predisposing to aneuploidy.Citation33-Citation35 Furthermore, oocytes expressing a non-functional form of NuMA, a microtubule-associated protein (MAP), present major spindle pole defects. Interestingly, in these oocytes, the SAC is silenced even when tension between the kinetochores of homologs is reduced and accompanied by alignment defects.Citation32 These findings establish that in mammalian meiosis I, the SAC is unable to sustain meiotic arrest in the presence of one or few misaligned and/or misattached kinetochores with reduced inter-kinetochore tension, thereby offering an explanation for why meiosis I in mammals is so error-prone. In addition, it has been established that the rate of trisomy increases with maternal age, and this in a frightening exponential way starting at 35-y-old.Citation23 The age-dependent increase of errors during meiosis I could be due to the fact that the glue holding the bivalent chromosomes together, namely cohesins, becomes weaker with age, oocytes being arrested in prophase I for decades in human.Citation36,Citation37 Such a defect would result in a decrease in inter-kinetochore tension, which would not be sensed by the meiotic SAC.Citation32 The origin for such an important rate of aneuploidy in oocytes could be due to the high volume of these cells and the low DNA/cytoplasm ratio inducing a strong dilution of the SAC signaling emitted from unattached or mis-attached kinetochores. Consistent with this hypothesis, in larger eggs, such as the ones from Xenopus, oocytes and embryos do not harbor any spindle assembly checkpoint until the mid-blastula transition, where in the early embryo, dividing only by cleavage with no increase in its total size, the ratio of DNA vs. cytoplasm increases, becoming closer to that encountered in mitotic cells.Citation38-Citation43 Additionally, aneuploidy could be inherent to the less robust mode of spindle assembly of female meiosis due to the absence of centrosomes.
Most healthy cells, with the exception of oocytes, possess 1 or 2 canonical centrosomes, depending on their cell cycle stage (centrosome duplication takes place in S phase). Extra centrosomes can induce multipolar spindle formation, resulting in chromosome mis-segregation and leading to cell death.Citation44 However, many cancer cells, as well as some healthy cells, do harbor an excess of centrosomes but manage to avoid cell death. They keep dividing, correlating with an increased rate of aneuploidy. The presence of extra-centrosomes can even induce tumorigenesis, as shown in Drosophila.Citation45 Cancer cells with extra centrosomes have developed a strategy to keep dividing and avoid multipolar spindle formation: they cluster their extra centrosomes prior to division.Citation46,Citation47 Mouse oocytes also sort and cluster acentriolar MTOCs that are present in the egg at meiosis resumption. Following centriole loss at the beginning of follicle growth phase, oocytes reorganize their microtubule cytoskeleton by inducing the coalescence of few amorphous patches of PCM around the nuclear envelope by an unknown process.Citation48 Before entry into meiosis and NEBD, these discrete patches spread around the nuclear envelope via a microtubule-dependent process, such that they are evenly distributed around the chromatin when NEBD occurs.Citation48 The setup of spindle bipolarity is a slow process following NEBD and results from the sorting and coalescence of multiple MTOCs around the chromatinCitation49,Citation50 (). The small GTPase Ran, present in a gradient around the chromosomes in the oocyte, locally activates spindle assembly factors to promote microtubule nucleation and stabilization.Citation17,Citation51 Then the chromosomes congress toward the metaphase plate, showing highly oscillatory movements until the very end of prometaphase,Citation52 K-fibers being established only late in meiosis ICitation33,Citation34,Citation49,Citation53 (). Interestingly, proper spindle assembly in oocytes requires the sorting and coalescence of multiple acentriolar MTOCs,Citation49 a process reminiscent centrosome clustering.Citation47 In particular, HURP, a MAP and Ran effector, is essential for the assembly of a robust central microtubule domain, acting as a scaffold for the sorting of MTOCs to spindle poles. HURP is essential in meiosis but dispensable for mitosis, since HURP-knockout mice are viable but female sterile. However, it is also required in cancer cells bearing multiple centrosomes to cluster them, suggesting that the pathway of acentriolar MTOC clustering in meiosis is also used by cancer cells to cluster their extra centrosomes.Citation49 HURP is not required for normal dividing cells; thus, like HSET, it would be a good therapeutic target to specifically slow down cancer cells but not somatic cell division, since cancer cells that do not cluster their extra centrosomes will eventually die.
Figure 3. Spindle assembly in mitosis and meiosis. NEBD, nuclear envelope breakdown. Chromosomes in red, kinetochores in yellow, microtubules in green, K-fibers in dark green, centrioles in black, pericentriolar material (PCM) in brown, nucleus in light gray.
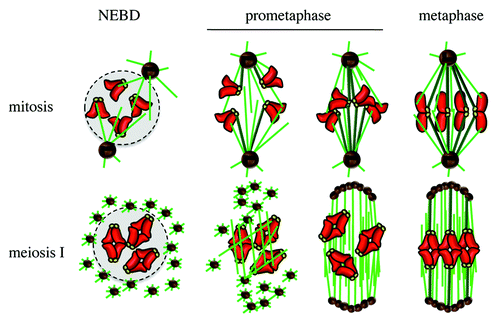
Whereas most normal cells undergoing mitosis present a stiff cortex, which helps them read external constraints coming from their environing tissue, mouse oocytes soften their cortex, like malignant cells do, freeing them from external forces and allowing them to position their spindle off-center.Citation54 More precisely, meiosis I spindle migration to the cortex depends on the presence of a dynamic cytoplasmic F-actin meshwork (), nucleated by Formin-2Citation16,Citation55-Citation58 and Spire 1/2.Citation59 These nucleators are enriched in Rab11a- and Myosin-Vb-positive vesicles that drive the network dynamics and density.Citation60,Citation61 This cytoplasmic F-actin meshwork is present in prophase I-arrested oocytes, then dismantled at NEBD, and reforms progressively during meiosis I.Citation62 It includes an actin cage surrounding the microtubule spindle, connected via straight actin filaments to the cortex.Citation16,Citation57 In addition and contrary to what is observed during mitosis, cortical tension decreases during meiosis,Citation63 a puzzling observation, since oocytes are round cells stable in shape that actively position their spindles (). Softening of the cortex during meiosis I is due to cortical myosin II exclusion and to the nucleation of a cortical F-actin thickening by the Arp2/3 complex (). These 2 events are triggered by the Mos/MAPK pathway. Mos is a MAPKKK, which activates MAPKs ERK1 and ERK2 in oocytes after NEBD.Citation15 This pathway is required for the arrest of the cell cycle in metaphase II and for spindle migration in meiosis ICitation15 (). The softening of the cortex, absent from mos−/− oocytes, is essential for asymmetric positioning of the first meiotic spindle, since artificial stiffening of the cortex prevents spindle migration. Theoretical modeling of spindle migration as a function of cortical tension shows that a soft cortex amplifies a slight imbalance of forces generated at spindle poles by myosin II,Citation16 since the spindle does not form at the center of the cell after NEBD, but a little off-centerCitation54 (). Toward the end of meiosis I, spindle migration is accelerated by actin-mediated cytoplasmic streaming.Citation64 Invasive tumor cells mechanically soften (up to 70% softer than their neighboring healthy cells) and modify their adhesion to the extracellular matrix, enhancing their capacity to escape the initial site of primary tumor growth. Cortex softening is a general feature of cancer cells and can be found in a variety of tumor cells from breast, gastrointestinal, ovarian, lungs, and pancreas cancers.Citation65-Citation67 It has even been proposed that cell stiffness may be a useful biomarker to evaluate the relative metastatic potential of cancer cells.Citation65,Citation68-Citation71 In ovarian cancer, it has been shown that the reduced stiffness of cancer cells is associated with actin cytoskeleton remodeling.Citation71 In the neighboring stiffer healthy cells, the actin filaments are distributed through the cell body, with most F-actin bundles aligned along the long axis of the cell with well-defined stress fibers and focal contacts. In contrast, F-actin in the softer ovarian cancer cells maintains a cortical structure, with most filaments lying in the peripheral region of the cell, but F-actin in the cytoplasm is less organized.Citation71 This cortical organization recalls the cortical actin thickening present in mouse oocytes that also soften their cortex.Citation54 Oocytes might use the same strategy of cancer cells to break free from the ovaries during ovulation. It is interesting that mos−/− oocytes, that keep a high cortical tension, fail to arrest in metaphase II and activate parthenogenetically in the absence of a sperm, inducing ovarian teratomas at a high frequency.Citation72,Citation73 However, if the decrease in cortical tension may favor ovulation and extrusion from the ovary, it is certainly not the major driver of ovulation. Indeed females that overexpress the sperm protein PLCZ1 in oocytes show normal oocyte meiotic progression, yet induce parthenogenetic activation of their eggs and display even greater rates of teratocarninomas than mos−/− females.Citation74 Altogether, these results argue that it is mostly parthenogenetic activation and not increase in cortical tension that causes ovarian teratomas.
Conclusion
Oocytes development is strictly dependent on asymmetric divisions. In mouse oocytes, the asymmetry of the divisions relies mostly on actin and on a drop of cortical tension, an actin-dependent exclusive way to position the spindle, which may have evolved from the absence of canonical centrosomes and their associated astral microtubules. Both features make this model relevant to study processes used by cancer cells to divide, since they are the dominant mode in oocytes. Hence mouse oocytes may be used as test tubes to test new potential targets for therapies against cancer. Even though oocytes share features present in certain types of cancer cells, contrary to them, they also acquired the ability to stop dividing. Indeed the meiotic cell cycle is characterized by multiple arrests, i.e., in prophase I, in metaphase I or II, or even later in G1, adapted to the reproductive pace specific to the species. It is puzzling that the same oncogenic pathway, namely the Mos/MAPK, actually controls both the asymmetry of meiotic divisions and actin-dependent cortex remodeling as well as the brake on the meiotic cell cycle. This unique feature has been conserved through evolution and is observed in species as distant as the jellyfish and the mouse, suggesting that the 2 functions may have been acquired simultaneously, avoiding gametes turning into teratomas.Citation72,Citation73,Citation75
Abbreviations, | ||
MTOC | = | microtubule organizing centers |
APC | = | adenomatous polyposis coli |
Lgl | = | lethal giant larvae |
Brat | = | brain tumor |
PCM | = | pericentriolar material |
Mud | = | mushroom body defect |
Pins | = | partner of inscuteable |
NEBD | = | nuclear envelope breakdown |
LH | = | luteinizing hormone |
SAC | = | spindle assembly checkpoint |
APC/C | = | anaphase-promoting complex/cyclosome |
K-fibers | = | kinetochore fibers |
NuMA | = | nuclear mitotic apparatus |
MAP | = | microtubule associated protein |
HURP | = | hepatoma up-regulated protein |
HSET | = | human spleen embryonic tissue and testes, also known as KIFC1 |
Arp2/3 | = | actin related protein 2/3 |
MAPK | = | mitogen activated protein kinase |
PLCZ1 | = | phospholipase C-zeta |
MT | = | microtubule |
PB1 | = | polar body 1 |
Acknowledgments
We thank Renata Basto for critical reading of this manuscript. This work was supported by grants from the Ligue Nationale Contre le Cancer (EL2012/LNCC/MHV). Agathe Chaigne is a recipient of a fellowship from the Ecole Normale Supérieure (ENS) Paris.
Disclosure of Potential Conflicts of Interest
No potential conflicts of interest were disclosed.
References
- Powell AE, Shung CY, Saylor KW, Müllendorff KA, Weiss JB, Wong MH. Lessons from development: A role for asymmetric stem cell division in cancer. Stem Cell Res 2010; 4:3 - 9; http://dx.doi.org/10.1016/j.scr.2009.09.005; PMID: 19853549
- Lu MS, Johnston CA. Molecular pathways regulating mitotic spindle orientation in animal cells. Development 2013; 140:1843 - 56; http://dx.doi.org/10.1242/dev.087627; PMID: 23571210
- Gillies TE, Cabernard C. Cell division orientation in animals. Curr Biol 2011; 21:R599 - 609; http://dx.doi.org/10.1016/j.cub.2011.06.055; PMID: 21820628
- Toso A, Winter JR, Garrod AJ, Amaro AC, Meraldi P, McAinsh AD. Kinetochore-generated pushing forces separate centrosomes during bipolar spindle assembly. J Cell Biol 2009; 184:365 - 72; http://dx.doi.org/10.1083/jcb.200809055; PMID: 19204145
- Tanenbaum ME, Medema RH. Mechanisms of centrosome separation and bipolar spindle assembly. Dev Cell 2010; 19:797 - 806; http://dx.doi.org/10.1016/j.devcel.2010.11.011; PMID: 21145497
- Moore JK, Cooper JA. Coordinating mitosis with cell polarity: Molecular motors at the cell cortex. Semin Cell Dev Biol 2010; 21:283 - 9; http://dx.doi.org/10.1016/j.semcdb.2010.01.020; PMID: 20109571
- Théry M, Bornens M. Cell shape and cell division. Curr Opin Cell Biol 2006; 18:648 - 57; http://dx.doi.org/10.1016/j.ceb.2006.10.001; PMID: 17046223
- Kunda P, Baum B. The actin cytoskeleton in spindle assembly and positioning. Trends Cell Biol 2009; 19:174 - 9; http://dx.doi.org/10.1016/j.tcb.2009.01.006; PMID: 19285869
- Otsuki J, Nagai Y, Lopata A, Chiba K, Yasmin L, Sankai T. Symmetrical division of mouse oocytes during meiotic maturation can lead to the development of twin embryos that amalgamate to form a chimeric hermaphrodite. Hum Reprod 2012; 27:380 - 7; http://dx.doi.org/10.1093/humrep/der408; PMID: 22147919
- Brunet S, Verlhac MH. Positioning to get out of meiosis: the asymmetry of division. Hum Reprod Update 2011; 17:68 - 75; http://dx.doi.org/10.1093/humupd/dmq044; PMID: 20833637
- Chaigne A, Verlhac MH, Terret ME. Spindle positioning in mammalian oocytes. Exp Cell Res 2012; 318:1442 - 7; http://dx.doi.org/10.1016/j.yexcr.2012.02.019; PMID: 22406266
- FitzHarris G, Marangos P, Carroll J. Changes in endoplasmic reticulum structure during mouse oocyte maturation are controlled by the cytoskeleton and cytoplasmic dynein. Dev Biol 2007; 305:133 - 44; http://dx.doi.org/10.1016/j.ydbio.2007.02.006; PMID: 17368610
- Halet G, Carroll J. Rac activity is polarized and regulates meiotic spindle stability and anchoring in mammalian oocytes. Dev Cell 2007; 12:309 - 17; http://dx.doi.org/10.1016/j.devcel.2006.12.010; PMID: 17276347
- Szollosi D, Calarco P, Donahue RP. Absence of centrioles in the first and second meiotic spindles of mouse oocytes. J Cell Sci 1972; 11:521 - 41; PMID: 5076360
- Verlhac MH, Lefebvre C, Guillaud P, Rassinier P, Maro B. Asymmetric division in mouse oocytes: with or without Mos. Curr Biol 2000; 10:1303 - 6; http://dx.doi.org/10.1016/S0960-9822(00)00753-3; PMID: 11069114
- Schuh M, Ellenberg J. A new model for asymmetric spindle positioning in mouse oocytes. Curr Biol 2008; 18:1986 - 92; http://dx.doi.org/10.1016/j.cub.2008.11.022; PMID: 19062278
- Dumont J, Petri S, Pellegrin F, Terret ME, Bohnsack MT, Rassinier P, Georget V, Kalab P, Gruss OJ, Verlhac MH. A centriole- and RanGTP-independent spindle assembly pathway in meiosis I of vertebrate oocytes. J Cell Biol 2007; a 176:295 - 305; http://dx.doi.org/10.1083/jcb.200605199; PMID: 17261848
- Deng M, Suraneni P, Schultz RM, Li R. The Ran GTPase mediates chromatin signaling to control cortical polarity during polar body extrusion in mouse oocytes. Dev Cell 2007; 12:301 - 8; http://dx.doi.org/10.1016/j.devcel.2006.11.008; PMID: 17276346
- Maro B, Johnson MH, Webb M, Flach G. Mechanism of polar body formation in the mouse oocyte: an interaction between the chromosomes, the cytoskeleton and the plasma membrane. J Embryol Exp Morphol 1986; 92:11 - 32; PMID: 3723057
- Longo FJ, Chen DY. Development of cortical polarity in mouse eggs: involvement of the meiotic apparatus. Dev Biol 1985; 107:382 - 94; http://dx.doi.org/10.1016/0012-1606(85)90320-3; PMID: 4038667
- Yi K, Unruh JR, Deng M, Slaughter BD, Rubinstein B, Li R. Dynamic maintenance of asymmetric meiotic spindle position through Arp2/3-complex-driven cytoplasmic streaming in mouse oocytes. Nat Cell Biol 2011; 13:1252 - 8; http://dx.doi.org/10.1038/ncb2320; PMID: 21874009
- Nagaoka SI, Hassold TJ, Hunt PA. Human aneuploidy: mechanisms and new insights into an age-old problem. Nat Rev Genet 2012; 13:493 - 504; http://dx.doi.org/10.1038/nrg3245; PMID: 22705668
- Hassold T, Hunt P. To err (meiotically) is human: the genesis of human aneuploidy. Nat Rev Genet 2001; 2:280 - 91; http://dx.doi.org/10.1038/35066065; PMID: 11283700
- Hassold T, Hunt P. Maternal age and chromosomally abnormal pregnancies: what we know and what we wish we knew. Curr Opin Pediatr 2009; 21:703 - 8; http://dx.doi.org/10.1097/MOP.0b013e328332c6ab; PMID: 19881348
- Schvartzman JM, Sotillo R, Benezra R. Mitotic chromosomal instability and cancer: mouse modelling of the human disease. Nat Rev Cancer 2010; 10:102 - 15; http://dx.doi.org/10.1038/nrc2781; PMID: 20094045
- Musacchio A, Salmon ED. The spindle-assembly checkpoint in space and time. Nat Rev Mol Cell Biol 2007; 8:379 - 93; http://dx.doi.org/10.1038/nrm2163; PMID: 17426725
- Rieder CL, Cole RW, Khodjakov A, Sluder G. The checkpoint delaying anaphase in response to chromosome monoorientation is mediated by an inhibitory signal produced by unattached kinetochores. J Cell Biol 1995; 130:941 - 8; http://dx.doi.org/10.1083/jcb.130.4.941; PMID: 7642709
- Wassmann K, Niault T, Maro B. Metaphase I arrest upon activation of the Mad2-dependent spindle checkpoint in mouse oocytes. Curr Biol 2003; 13:1596 - 608; http://dx.doi.org/10.1016/j.cub.2003.08.052; PMID: 13678590
- Homer HA, McDougall A, Levasseur M, Herbert M. Restaging the spindle assembly checkpoint in female mammalian meiosis I. Cell Cycle 2005; 4:650 - 3; http://dx.doi.org/10.4161/cc.4.5.1677; PMID: 15846076
- Niault T, Hached K, Sotillo R, Sorger PK, Maro B, Benezra R, Wassmann K. Changing Mad2 levels affects chromosome segregation and spindle assembly checkpoint control in female mouse meiosis I. PLoS One 2007; 2:e1165; http://dx.doi.org/10.1371/journal.pone.0001165; PMID: 18043727
- McGuinness BE, Anger M, Kouznetsova A, Gil-Bernabé AM, Helmhart W, Kudo NR, Wuensche A, Taylor S, Hoog C, Novak B, et al. Regulation of APC/C activity in oocytes by a Bub1-dependent spindle assembly checkpoint. Curr Biol 2009; 19:369 - 80; http://dx.doi.org/10.1016/j.cub.2009.01.064; PMID: 19249208
- Kolano A, Brunet S, Silk AD, Cleveland DW, Verlhac MH. Error-prone mammalian female meiosis from silencing the spindle assembly checkpoint without normal interkinetochore tension. Proc Natl Acad Sci U S A 2012; 109:E1858 - 67; http://dx.doi.org/10.1073/pnas.1204686109; PMID: 22552228
- Lane SI, Yun Y, Jones KT. Timing of anaphase-promoting complex activation in mouse oocytes is predicted by microtubule-kinetochore attachment but not by bivalent alignment or tension. Development 2012; 139:1947 - 55; http://dx.doi.org/10.1242/dev.077040; PMID: 22513370
- Gui L, Homer H. Spindle assembly checkpoint signalling is uncoupled from chromosomal position in mouse oocytes. Development 2012; 139:1941 - 6; http://dx.doi.org/10.1242/dev.078352; PMID: 22513372
- Sebestova J, Danylevska A, Novakova L, Kubelka M, Anger M. Lack of response to unaligned chromosomes in mammalian female gametes. Cell Cycle 2012; 11:3011 - 8; http://dx.doi.org/10.4161/cc.21398; PMID: 22871737
- Chiang T, Duncan FE, Schindler K, Schultz RM, Lampson MA. Evidence that weakened centromere cohesion is a leading cause of age-related aneuploidy in oocytes. Curr Biol 2010; 20:1522 - 8; http://dx.doi.org/10.1016/j.cub.2010.06.069; PMID: 20817534
- Lister LM, Kouznetsova A, Hyslop LA, Kalleas D, Pace SL, Barel JC, Nathan A, Floros V, Adelfalk C, Watanabe Y, et al. Age-related meiotic segregation errors in mammalian oocytes are preceded by depletion of cohesin and Sgo2. Curr Biol 2010; 20:1511 - 21; http://dx.doi.org/10.1016/j.cub.2010.08.023; PMID: 20817533
- Gerhart J, Wu M, Kirschner MW. Cell cycle dynamics of an M-phase-specific cytoplasmic factor in Xenopus laevis oocytes and eggs. J Cell Biol 1984; 98:1247 - 55; http://dx.doi.org/10.1083/jcb.98.4.1247; PMID: 6425302
- Murray AW, Kirschner MW. Dominoes and clocks: the union of two views of the cell cycle. Science 1989; 246:614 - 21; http://dx.doi.org/10.1126/science.2683077; PMID: 2683077
- Clute P, Masui Y. Regulation of the appearance of division asynchrony and microtubule-dependent chromosome cycles in Xenopus laevis embryos. Dev Biol 1995; 171:273 - 85; http://dx.doi.org/10.1006/dbio.1995.1280; PMID: 7556912
- Taieb FE, Gross SD, Lewellyn AL, Maller JL. Activation of the anaphase-promoting complex and degradation of cyclin B is not required for progression from Meiosis I to II in Xenopus oocytes. Curr Biol 2001; 11:508 - 13; http://dx.doi.org/10.1016/S0960-9822(01)00145-2; PMID: 11413001
- Peter M, Castro A, Lorca T, Le Peuch C, Magnaghi-Jaulin L, Dorée M, Labbé JC. The APC is dispensable for first meiotic anaphase in Xenopus oocytes. Nat Cell Biol 2001; 3:83 - 7; http://dx.doi.org/10.1038/35050607; PMID: 11146630
- Shao H, Li R, Ma C, Chen E, Liu XJ. Xenopus oocyte meiosis lacks spindle assembly checkpoint control. J Cell Biol 2013; 201:191 - 200; http://dx.doi.org/10.1083/jcb.201211041; PMID: 23569212
- Ganem NJ, Godinho SA, Pellman D. A mechanism linking extra centrosomes to chromosomal instability. Nature 2009; 460:278 - 82; http://dx.doi.org/10.1038/nature08136; PMID: 19506557
- Basto R, Brunk K, Vinadogrova T, Peel N, Franz A, Khodjakov A, Raff JW. Centrosome amplification can initiate tumorigenesis in flies. Cell 2008; 133:1032 - 42; http://dx.doi.org/10.1016/j.cell.2008.05.039; PMID: 18555779
- Quintyne NJ, Reing JE, Hoffelder DR, Gollin SM, Saunders WS. Spindle multipolarity is prevented by centrosomal clustering. Science 2005; 307:127 - 9; http://dx.doi.org/10.1126/science.1104905; PMID: 15637283
- Kwon M, Godinho SA, Chandhok NS, Ganem NJ, Azioune A, Théry M, Pellman D. Mechanisms to suppress multipolar divisions in cancer cells with extra centrosomes. Genes Dev 2008; 22:2189 - 203; http://dx.doi.org/10.1101/gad.1700908; PMID: 18662975
- Łuksza M, Queguigner I, Verlhac M-H, Brunet S. Rebuilding MTOCs upon centriole loss during mouse oogenesis. Dev Biol 2013; In press PMID: 23954884
- Breuer M, Kolano A, Kwon M, Li CC, Tsai TF, Pellman D, Brunet S, Verlhac MH. HURP permits MTOC sorting for robust meiotic spindle bipolarity, similar to extra centrosome clustering in cancer cells. J Cell Biol 2010; 191:1251 - 60; http://dx.doi.org/10.1083/jcb.201005065; PMID: 21173113
- Schuh M, Ellenberg J. Self-organization of MTOCs replaces centrosome function during acentrosomal spindle assembly in live mouse oocytes. Cell 2007; 130:484 - 98; http://dx.doi.org/10.1016/j.cell.2007.06.025; PMID: 17693257
- Brunet S, Dumont J, Lee KW, Kinoshita K, Hikal P, Gruss OJ, Maro B, Verlhac MH. Meiotic regulation of TPX2 protein levels governs cell cycle progression in mouse oocytes. PLoS One 2008; 3:e3338; http://dx.doi.org/10.1371/journal.pone.0003338; PMID: 18833336
- Kitajima TS, Ohsugi M, Ellenberg J. Complete kinetochore tracking reveals error-prone homologous chromosome biorientation in mammalian oocytes. Cell 2011; 146:568 - 81; http://dx.doi.org/10.1016/j.cell.2011.07.031; PMID: 21854982
- Brunet S, Maria AS, Guillaud P, Dujardin D, Kubiak JZ, Maro B. Kinetochore fibers are not involved in the formation of the first meiotic spindle in mouse oocytes, but control the exit from the first meiotic M phase. J Cell Biol 1999; 146:1 - 12; http://dx.doi.org/10.1083/jcb.146.1.1; PMID: 10402455
- Chaigne A, Campillo C, Gov NS, Voituriez R, Azoury J, Umaña-Diaz C, Almonacid M, Queguiner I, Nassoy P, Sykes C, et al. A soft cortex is essential for asymmetric spindle positioning in mouse oocytes. Nat Cell Biol 2013; 15:958 - 66; http://dx.doi.org/10.1038/ncb2799; PMID: 23851486
- Leader B, Lim H, Carabatsos MJ, Harrington A, Ecsedy J, Pellman D, Maas R, Leder P. Formin-2, polyploidy, hypofertility and positioning of the meiotic spindle in mouse oocytes. Nat Cell Biol 2002; 4:921 - 8; http://dx.doi.org/10.1038/ncb880; PMID: 12447394
- Dumont J, Million K, Sunderland K, Rassinier P, Lim H, Leader B, Verlhac MH. Formin-2 is required for spindle migration and for the late steps of cytokinesis in mouse oocytes. Dev Biol 2007; b 301:254 - 65; http://dx.doi.org/10.1016/j.ydbio.2006.08.044; PMID: 16989804
- Azoury J, Lee KW, Georget V, Rassinier P, Leader B, Verlhac MH. Spindle positioning in mouse oocytes relies on a dynamic meshwork of actin filaments. Curr Biol 2008; 18:1514 - 9; http://dx.doi.org/10.1016/j.cub.2008.08.044; PMID: 18848445
- Li H, Guo F, Rubinstein B, Li R. Actin-driven chromosomal motility leads to symmetry breaking in mammalian meiotic oocytes. Nat Cell Biol 2008; 10:1301 - 8; http://dx.doi.org/10.1038/ncb1788; PMID: 18836438
- Pfender S, Kuznetsov V, Pleiser S, Kerkhoff E, Schuh M. Spire-type actin nucleators cooperate with Formin-2 to drive asymmetric oocyte division. Curr Biol 2011; 21:955 - 60; http://dx.doi.org/10.1016/j.cub.2011.04.029; PMID: 21620703
- Schuh M. An actin-dependent mechanism for long-range vesicle transport. Nat Cell Biol 2011; 13:1431 - 6; http://dx.doi.org/10.1038/ncb2353; PMID: 21983562
- Holubcová Z, Howard G, Schuh M. Vesicles modulate an actin network for asymmetric spindle positioning. Nat Cell Biol 2013; 15:937 - 47; http://dx.doi.org/10.1038/ncb2802; PMID: 23873150
- Azoury J, Lee KW, Georget V, Hikal P, Verlhac MH. Symmetry breaking in mouse oocytes requires transient F-actin meshwork destabilization. Development 2011; 138:2903 - 8; http://dx.doi.org/10.1242/dev.060269; PMID: 21653611
- Larson SM, Lee HJ, Hung PH, Matthews LM, Robinson DN, Evans JP. Cortical mechanics and meiosis II completion in mammalian oocytes are mediated by myosin-II and Ezrin-Radixin-Moesin (ERM) proteins. Mol Biol Cell 2010; 21:3182 - 92; http://dx.doi.org/10.1091/mbc.E10-01-0066; PMID: 20660156
- Yi K, Rubinstein B, Unruh JR, Guo F, Slaughter BD, Li R. Sequential actin-based pushing forces drive meiosis I chromosome migration and symmetry breaking in oocytes. J Cell Biol 2013; 200:567 - 76; http://dx.doi.org/10.1083/jcb.201211068; PMID: 23439682
- Guck J, Schinkinger S, Lincoln B, Wottawah F, Ebert S, Romeyke M, Lenz D, Erickson HM, Ananthakrishnan R, Mitchell D, et al. Optical deformability as an inherent cell marker for testing malignant transformation and metastatic competence. Biophys J 2005; 88:3689 - 98; http://dx.doi.org/10.1529/biophysj.104.045476; PMID: 15722433
- Suresh S, Spatz J, Mills JP, Micoulet A, Dao M, Lim CT, Beil M, Seufferlein T. Connections between single-cell biomechanics and human disease states: gastrointestinal cancer and malaria. Acta Biomater 2005; 1:15 - 30; http://dx.doi.org/10.1016/j.actbio.2004.09.001; PMID: 16701777
- Hou HW, Li QS, Lee GY, Kumar AP, Ong CN, Lim CT. Deformability study of breast cancer cells using microfluidics. Biomed Microdevices 2009; 11:557 - 64; http://dx.doi.org/10.1007/s10544-008-9262-8; PMID: 19082733
- Cross SE, Jin YS, Rao J, Gimzewski JK. Nanomechanical analysis of cells from cancer patients. Nat Nanotechnol 2007; 2:780 - 3; http://dx.doi.org/10.1038/nnano.2007.388; PMID: 18654431
- Lekka M, Laidler P. Applicability of AFM in cancer detection. Nat Nanotechnol 2009; 4:72 - 72, author reply 72-3; http://dx.doi.org/10.1038/nnano.2009.004; PMID: 19197298
- Lee GYH, Lim CT. Biomechanics approaches to studying human diseases. Trends Biotechnol 2007; 25:111 - 8; http://dx.doi.org/10.1016/j.tibtech.2007.01.005; PMID: 17257698
- Xu W, Mezencev R, Kim B, Wang L, McDonald J, Sulchek T. Cell stiffness is a biomarker of the metastatic potential of ovarian cancer cells. PLoS One 2012; 7:e46609; http://dx.doi.org/10.1371/journal.pone.0046609; PMID: 23056368
- Colledge WH, Carlton MB, Udy GB, Evans MJ. Disruption of c-mos causes parthenogenetic development of unfertilized mouse eggs. Nature 1994; 370:65 - 8; http://dx.doi.org/10.1038/370065a0; PMID: 8015609
- Hashimoto N, Watanabe N, Furuta Y, Tamemoto H, Sagata N, Yokoyama M, Okazaki K, Nagayoshi M, Takeda N, Ikawa Y, et al. Parthenogenetic activation of oocytes in c-mos-deficient mice. Nature 1994; 370:68 - 71; http://dx.doi.org/10.1038/370068a0; PMID: 8015610
- Yoshida N, Amanai M, Fukui T, Kajikawa E, Brahmajosyula M, Iwahori A, Nakano Y, Shoji S, Diebold J, Hessel H, et al. Broad, ectopic expression of the sperm protein PLCZ1 induces parthenogenesis and ovarian tumours in mice. Development 2007; 134:3941 - 52; http://dx.doi.org/10.1242/dev.007930; PMID: 17933795
- Amiel A, Leclère L, Robert L, Chevalier S, Houliston E. Conserved functions for Mos in eumetazoan oocyte maturation revealed by studies in a cnidarian. Curr Biol 2009; 19:305 - 11; http://dx.doi.org/10.1016/j.cub.2008.12.054; PMID: 19230670