Abstract
Errors in chromosome segregation in mammalian oocytes increase in number with advancing maternal age, and are a major cause of pregnancy loss. Why chromosome segregation errors are more common in oocytes from older females remains poorly understood. In mitosis, accurate chromosome segregation is enabled by attachment of kinetochores to microtubules from appropriate spindle poles, and erroneous attachments increase the likelihood of mis-segregation. Whether attachment errors are responsible for age-related oocyte aneuploidy is unknown. Here we report that oocytes from naturally aged mice exhibit substantially increased chromosome misalignment, and fewer kinetochore pairs that make stable end-on attachments to the appropriate spindle poles compared with younger oocytes. The profile of mis-attachments exhibited is consistent with the types of chromosome segregation error observed in aged oocytes. Loss of chromosome cohesion, which is a feature of oocytes from older females, causes altered kinetochore geometry in meiosis-I. However, this has only a minor impact upon MT attachment, indicating that cohesion loss is not the primary cause of aneuploidy in meiosis-I. In meiosis-II, on the other hand, age-related cohesion loss plays a direct role in errors, since prematurely individualized sister chromatids misalign and misattach to spindle MTs. Thus, whereas cohesion loss leading to precocious sister chromatid separation is a direct cause of errors in meiosis-II, cohesion loss plays a more minor role in the etiology of aneuploidy in meiosis-I. Our data introduce altered MT-kinetochore interactions as a lesion that explains aneuploidy in meiosis-I in older females.
Introduction
Ensuring that daughter cells inherit the correct chromosomes at the time of cell division is essential for maintaining cellular health. Whereas chromosome mis-segregation is unusual in most mammalian cell types, errors are relatively common in mammalian oocytes, resulting in aneuploid oocytes that are developmentally compromised. Oocyte aneuploidy is particularly common in older females and is thus a major cause of age-related infertility in humans.Citation1,Citation2 Why oocytes from older females mis-segregate chromosomes more frequently than those from younger females is poorly understood.
Chromosome segregation is executed by the spindle, a dynamic transient organelle assembled from microtubules (MTs).Citation3,Citation4 Chromosomes interact with spindle microtubules via kinetochores, complex proteinaceous structures that assemble on centromeric DNA.Citation5 In mitosis, accurate chromosome segregation requires that kinetochores of sister chromatids (“sister kinetochores”) bind to microtubules from opposite poles of the spindle, such that shortening of kinetochore-bound MTs (kMTs) in anaphase causes one sister chromatid to be inherited by each daughter cell.Citation6 Failure to establish kMTs correctly can lead to chromosome mis-segregation. For example, kinetochore attachment to both spindle poles simultaneously causes chromosomes to experience pulling forces from both poles in anaphase, resulting in “lagging” chromosomes that might be mis-segregated.Citation7 This scenario, termed merotelic attachment, is particularly hazardous, as it is not detected by the spindle assembly checkpoint (SAC) that surveys and prevents other errors.Citation8-Citation10 Merotelic attachments are thus considered a key cause of aneuploidy in somatic cells.
Whereas in mitosis sister chromatids are separated, in meiosis-I sister chromatids remain attached and co-segregate in order to separate homologous chromosomes (see ). Essential for this is that sister chromatid cohesion is maintained during meiosis-I, and sister kinetochores serve together as a single microtubule-binding unit to enable co-segregation.Citation11 Sister cohesion is subsequently lost at anaphase of meiosis-II, generating a haploid genome that forms the maternal contribution at fertilization (). Chromosome segregation errors increase with maternal age both in meiosis-I and meiosis-II. However, the nature of the age-related defect remains mysterious.
Figure 1. Aged oocytes mis-segregate chromosomes without altering the duration of meiosis-I. (A) Cartoon illustrating normal chromosome segregation during mammalian oocyte meiosis-I and meiosis-II. Chromosomes are indicated in blue, kinetochores in red, and microtubules in black. Note that meiosis-I results in the separation of pairs of homologous chromosomes, such that sister chromatids are co-segregated to the same spindle pole. A pair of sister chromatids is also termed a “dyad”. Sisters are subsequently segregated in meiosis-II to generate a haploid genome. Further details in text. (B) One of 3 similar experiments, in which the duration of MI in young and aged oocytes was directly compared. Oocytes were collected and then cultured simultaneously, and the times of germinal vesicle breakdown (GVBD) and polar body extrusion (Pb1) determined. Note there is no difference in the timings of these events with age. The replicate shown comprised 28 young and 17 aged oocytes. (Ci) Two examples of in situ chromosome count experiments from mice aged 15–17 mo. Images shown are confocal Z-projections. Scoring was performed using individual slices. Yellow circles indicate pairs of sister kinetochores. Red circles indicate individual chromatids. (Cii) Zoomed individual optical slices of the 2 chromosomes labeled (a and b) in (Ci). Note that (a) depicts an individual sister, whereas (b) depicts a normal pair of sisters. Optical sections at 0.5-µm intervals.
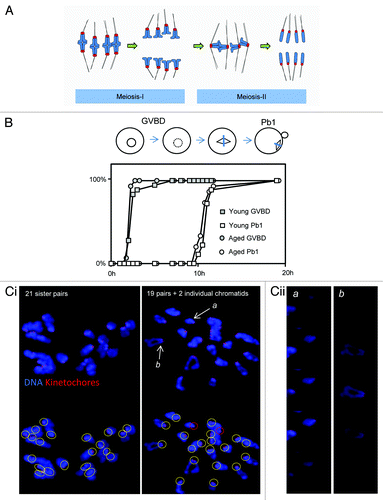
Laboratory mice aged 1–2 years exhibit increased chromosome mis-segregation and aneuploidy analogous to age-related oocyte aneuploidy in humans, and are thus an invaluable model to examine the cause of errors.Citation12 Using this approach, several groups have found that chromosome cohesion is weakened in oocytes from older females.Citation13-Citation16 This conclusion is supported by a reduced abundance of cohesin component proteins on chromosome arms,Citation13,Citation14 a reduced threshold for sister individualization by separase,Citation17 and an increased distance between sister kinetochores in metaphase-II (Met-II) eggs.Citation13-Citation15 As a result of cohesion loss, sister pairs can be prematurely individualized in Met-II in older mice.Citation14 Importantly, similar to mice, chromosome cohesion is lost with increasing age in human oocytes,Citation18 and it has thus been widely suggested that cohesion loss might be a fundamental cause of age-related aneuploidy.
Although the evidence that chromosome cohesion is lost with advancing age is strong, a critical unanswered question is: why should cohesion loss cause chromosome mis-segregation (dyad gain or loss) in meiosis-I? A prevalent hypothesis is that a loosening of sister chromosome cohesion in meiosis-I could change the size and geometry of the effective microtubule-binding area, which might, in turn, promote erroneous kinetochore–microtubule interactions, thereby causing mis-segregation.Citation8,Citation17,Citation19-Citation21 In support of this hypothesis, oocytes from old mothers frequently exhibit lagging anaphase chromosomes, which in mitosis are symptomatic of merotelic attachment errors.Citation13,Citation14,Citation22 However, whether age-related cohesion loss has a direct impact upon chromosome segregation in meiosis-I is yet to be formally examined. Specifically, whether sister kinetochore geometry is indeed altered in aged oocytes in meiosis-I, when MT–kinetochore attachments are being formed, and whether this can affect the fidelity of MT binding are unknown.
In any cellular setting, the occurrence of aneuploidy must be explained in terms of the failure of spindle microtubules to accurately dispatch chromosomes to the forming daughter cells. Here we present a detailed examination of kinetochore–microtubule interactions and chromosome positioning and report substantial differences in the profile of MT attachments between oocytes from young and naturally aged mothers that are consistent with a causal role in the genesis of age-related aneuploidy. Our data suggest a 2-step model for age-related oocyte aneuploidy, in which defects in MT–kinetochore attachments are the primary cause of errors in meiosis-I, whereas cohesion loss is the major cause of errors in meiosis-II.
Results
Premature sister chromatid disjunction and aneuploidy in naturally aged MF1 mice
We first set out to determine the duration of meiosis and the incidence of aneuploidy in MF1 mice. To determine whether maternal age affects the temporal kinetics of meiosis-I, we compared the timings of germinal vesicle breakdown and polar body extrusion, which indicate the length of M-phase. There was no difference in the duration of M-phase between oocytes from 6-wk-old and 15–17-mo-old females, consistent with studies using other mouse strains ().Citation13,Citation23 To assess ploidy, chromosomes were analyzed in the resulting Met-II eggs using the method of Duncan et al.,Citation23,Citation24 which we have used previously.Citation25 Almost all oocytes from young mice (6 wk) exhibit the expected 20 pairs of sister chromatids at Met-II in MF1 mice.Citation25 Similarly, we found here that oocytes from mice aged 12 mo had 20 sister chromatid pairs (10/10 oocytes examined). In contrast, one-third of Met-II eggs were aneuploid in mice aged 15–17 mo (8/24 examined; ). Of the 8 aneuploid oocytes, 3 were hypoploid and 5 were hyperploid. Individualized sister chromatids were evident in most oocytes 15–17-mo-old, consistent with loss of cohesion between sister chromatids (16/24 examined; ). This may be an underestimate, since chromosome congestion can sometimes occur in this assay, making it difficult to be certain that sisters are no longer joined. As described in other strainsCitation14,Citation15 we observed cases in which an oocyte had gained or lost an entire dyad, as well as cases where a single chromatid had been gained or lost (). Thus, as in other mouse strains,Citation13-Citation15 oocytes of aged MF1 mice progress through meiosis-I with normal temporal kinetics but exhibit sister chromosome cohesion loss and aneuploidy. Hereafter, we refer to oocytes from 15–17-mo-old females as “aged oocytes”, and from 6-wk-old mice as “young oocytes”.
Spindle structure and chromosome alignment in oocytes from aged mothers
The impact of sister cohesion loss in meiosis-I has previously only been observed following pharmacological spindle disruption or in chromosome spreads.Citation13,Citation14 In that setting, cohesion loss is evident as a separation of sister kinetochores; whereas sister kinetochore pairs appear as one coherent unit in young mice, the individual kinetochores can be distinguished in old mice using confocal microscopy.Citation13,Citation14 However, it is not known whether cohesion loss also impacts sister kinetochore pair coherence within the physiological setting of the intact spindle. To answer this, and to examine spindle architecture and chromosome alignment in aged oocytes, we generated immunofluorescence images of spindles in mid-meiosis-I. Young and aged oocytes were collected at the GV stage and cultured simultaneously prior to fixation and processing for immunofluorescence 8 h after release from the ovary. Complete confocal Z-stacks of DNA, microtubules, and CREST-labeled kinetochores were created of each spindle to enable faithful comparisons of the 2 groups (). In young oocytes sister kinetochore pairs appeared as a single coherent unit in almost all cases. In contrast, in aged oocytes, individual sister kinetochores could easily be resolved in 37% of cases, consistent with a loss of cohesion (). Although we have not labeled cohesin complex components in the present study, the loss of coherence of sister kinetochores is consistent with the loss of cohesins displayed by oocytes from other mouse strains during aging.Citation13,Citation14,Citation16 Thus age-related loss of chromosome cohesion leads to a change in kinetochore geometry that is evident in intact bivalents within the physiological setting of the mid-MI spindle.
Figure 2. Chromosome misalignment and cohesion loss in mid-late meioisis-I. (A) Examples of young and aged oocytes fixed for immunofluorescence in mid meiosis-I. Image shown is a confocal z-projection. (B) Zoomed image of the area highlighted in (A) to illustrate evidence of cohesion loss. The closed arrowhead highlights a sister kinetochore pair in which the kinetochores remain tightly associated, such that the pair appears as a single coherent unit. The open arrow indicates a sister pair in which the 2 individual kinetochores are separated enough to appear as a “figure 8”. The proportion of kinetochore pairs in which the individual kinetochores are distinguishable in this manner in young and aged oocytes is quantified in (C). *Indicates P < 0.01 using a Student t test. (D) Quantification of chromosome mis-alignment in mid meiosis-I. *Indicates P < 0.01 using a Student CHI2. (E) Comparison of the proportion of dissociated and coherent sister pairs that fall within the center 50% of the spindle by length. Note there is no significant alignment difference between dissociated and coherent kinetochores. Data taken from 22 young and 19 aged oocytes over the course of 3 similar experimental replicates.
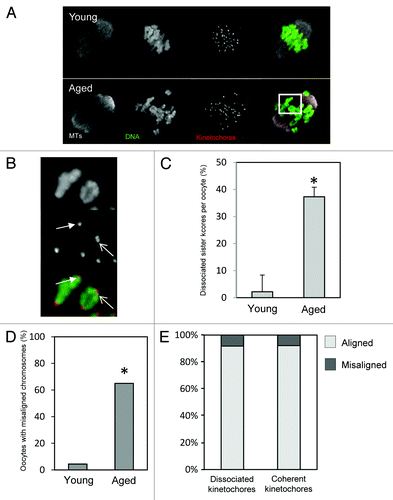
We next analyzed spindle morphology and chromosome alignment. There was no obvious difference in gross spindle geometry between young and aged oocytes, as previously noted.Citation14 All spindles examined had the expected fusiform/barrel shape, and there was no difference in spindle length between young and aged oocytes. Strikingly, however, there was a far higher degree of chromosome misalignment in aged oocytes. We used 2 different analysis methods to confirm this. First we determined whether there were any bivalents clearly separated from the metaphase plate (as in ref. Citation25), and found that whereas chromosomes were all aligned in 20/22 young oocytes (9% misalignment), at least one bivalent was misaligned in 12/19 aged oocytes (63%) (). Second, as an independent method of assessing alignment, we also determined the proportion of CREST-labeled kinetochore pairs falling outside the central 50% of the spindle. More than twice as many kinetochores were outside the central spindle portion in aged oocytes (P < 0.05). As illustrated in , whereas almost all chromosomes at the spindle equator were bi-oriented, regardless of oocyte age, most misaligned chromosomes were mono-oriented. Thus, increased misalignment and mono-orientation are characteristic of mid-meiosis-I in aged oocytes.
We wondered whether chromosomes with loosely associated sister kinetochores are more likely to be misaligned than those with tightly coherent sister kinetochores. Assessment of misaligned chromosomes revealed that cohesion loss, as indicated by the appearance of 2 separately distinguishable sister kinetochores, was no more frequent in misaligned chromosomes compared with aligned chromosomes. There was no difference in the proportion of sister kinetochore pairs displaying cohesion loss in peripheral kinetochores (outside the central 50% of the spindle) compared with those close to the spindle equator (). Thus, oocytes from older mice exhibit misaligned chromosomes, but the identity of the misaligned chromosome does not correlate with the morphology of CREST-labeled sister kinetochore pairs.
Aberrant microtubule–kinetochore attachment in meiosis-I in old oocytes
We next set out to directly visualize kinetochore–microtubule interactions in young and aged oocytes in mid meiosis-I. Whereas non-kinetochore MTs undergo rounds of growth and catastrophe with a T1/2 in the order of 1–2 min, kinetochore microtubules are relatively stable.Citation26 Therefore, a 10 min exposure to ice-cold media results in depolymerization of unstable non-kinetochore microtubules, allowing kinetochore–microtubule interactions to be viewed by immunofluorescence. Using this approach we generated cohorts of young and aged oocytes for examination of MT–kinetochore interactions. As in the previous experiment, a greater proportion of aged oocytes exhibited chromosome misalignment than young oocytes, further corroborating this observation (P < 0.05). To assess kinetochore–MT attachment status, individual confocal slices were examined, and each kinetochore sister pair was categorized as attached to microtubules emanating from the appropriate pole only (polar attached), attached to the side of a passing microtubule bundle (lateral attachment), or unattached to any cold-stable MT bundle (unattached). We were also able to observe sister kinetochore pairs attached to MTs emanating from both poles, which is the meiosis-I equivalent of merotelic attachment (which we term “meiotic–merotelic” attachment; see ). Unattached kinetochores were relatively common in mid meiosis-I, as was recently shown in young oocytes,Citation27 but occurred at a similar frequency regardless of maternal age (~30–35%). In young oocytes 49% of kinetochore pairs were correctly polar attached (). Only 4% were attached meiotic-merotelically, and 17% were laterally attached. However, the profile of attachments was substantially altered in aged oocytes. Significantly fewer kinetochores were polar attached (28%, P < 0.01). Instead, a far greater proportion were laterally (27%, P < 0.05) or meiotic-merotelically attached (10%, P < 0.01). Thus oocytes at the same stage of meiosis have a substantially altered profile of MT–kinetochore attachments depending upon maternal age, with substantially fewer kinetochore pairs correctly attached to a single spindle pole ().
Figure 3. Differences in microtubule attachment in mid meiosis-I in young and aged oocytes. (A) Examples of young and aged oocytes imaged in mid-meiosis-I. (B) Analysis of attachment types in oocytes from young and aged mice. Two examples of each attachment type observed in mid-late meiosis-I. Arrowheads indicate the kinetochore pair with the described attachment. Colored surroundings of each box correspond to the colors in the quantification of attachment types in (C and D). A total of 488 attachments from 17 aged oocytes, and 388 attachments from 15 young oocytes were classified from 3 experimental replicates. All attachments were classified in a blinded manner, using individual optical slices. Statistical significance is indicated by *(P < 0.05) and ** (P < 0.01).
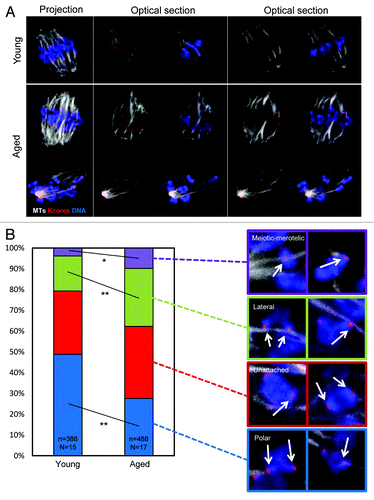
As with the previous experiment (), a greater proportion of kinetochore pairs exhibited evidence of cohesion loss in oocytes from older mothers than in oocytes from young mothers (46% in aged oocytes, 12% in young oocytes; P < 0.01). Therefore, to determine whether cohesion loss is associated with mis-attachment, we recorded attachment status of each sister kinetochore pair and correlated this with whether that kinetochore pair appeared as a coherent unit, or whether 2 individual sister kinetochores were distinguishable. Strikingly, kinetochore pairs were equally likely to be polar attached if the sister kinetochores were coherent, or if they were dissociated such that sister kinetochores were distinguishable (). Similarly, the likelihood of laterally attachment or being unattached was not changed depending upon kinetochore morphology. There was, however, an increase in the likelihood that a given kinetochore pair would be meiotic-merotelically attached if sister cohesion was lost. Notably, an increase in meiotic-merotely correlated with cohesion loss both in aged oocytes (6.1% meiotic-merotely for coherent sisters, 12.4% meiotic-merotely for dissociated sisters; P < 0.03), and also in young oocytes (3.3% meiotic-merotely for coherent sisters, 7.8% meiotic-merotely for dissociated sisters; P < 0.03), consistent with the notion that cohesion loss might influence MT attachment. However, while statistically significant and consistent between age groups, this increase is modest in the context of the overall change in attachment profile across all kinetochore pairs (see ; ).
Table 1. Analysis of microtubule attachment-type data
In summary, aged oocytes exhibit a greatly decreased number of polar microtubule attachments and a substantial increase in meiotic–merotelic and lateral misattachments compared with young oocytes. A proportion of the increase in meiotic–merotelic attachments correlates with altered kinetochore geometry, suggesting that altered kinetochore geometry associated with cohesion loss plays some role in determining microtubule attachment. However, the data indicate that the majority of the shift in attachment profile in oocytes from old mice occurs independently of whether the kinetochore appears as a single coherent unit or 2 optically distinguishable sisters.
Prematurely individualized sister chromatids misalign at metaphase-II
Finally, given that sister chromatids are frequently prematurely dissociated in aged Met-II eggs (), we wondered how individualized sisters would behave on the Met-II spindle. We therefore generated 3D confocal data sets of young and aged in vitro-matured Met-II oocytes using cold-shock treatment to observe stable MTs (). At least one misaligned chromosome was observed in 21/43 of aged oocytes (49%), whereas misalignment was only observed in 1/25 young oocytes (4%; ), as previously reported in MetII eggs (P < 0.01).Citation12,Citation28 Our data set allowed us to examine the kinetochore–MT interactions of misaligned chromosomes. Notably, all misaligned chromosomes in aged oocytes were individualized sisters that were either attached laterally (6/15 examined) or with end-on attachments to the nearest pole (9/15) (). While the high level of congestion of chromosomes makes it impossible for us to exclude the possibility that other individualized sisters reside at the metaphase plate, this nonetheless clearly reveals that premature sister disjunction is the primary cause of chromosome misalignment at Met-II in old females.
Figure 4. Premature sister individualization causes chromatid misalignment at metaphase-II. (A) Confocal z-projections of young and aged in vitro-matured Met-II oocytes were collected. One example of a young oocyte and 2 examples of aged oocytes are shown. Color scheme as indicated. Insets are zooms of the indicated region. (B) Analysis of chromosome alignment in young and aged oocytes. Data from 3 similar replicates. (C) Quantification of attachment status of misaligned chromosomes in aged Met-II oocytes. Data from 2 similar replicates.
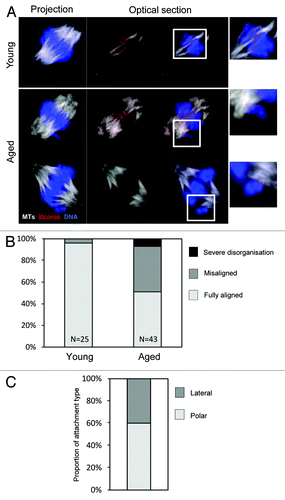
Discussion
Attachment errors and cohesion loss in meiosis-I
Loss of chromosome cohesion in oocytes from aging females has garnered considerable attention as a possible cause of age-related oocyte aneuploidy, but why cohesion loss should cause mis-segregation of sister pairs in meiosis-I has been unclear. Here we were able to directly correlate MT misattachments with kinetochore morphology, and found strikingly little difference in the attachment types between those kinetochore pairs with obvious loss of cohesion and those that were a coherent pair. There was a bias toward meiotic–merotelic attachment loss, which was observed both in young and aged oocytes. However, notably, this was minor compared with a substantial global defect in kMT binding in old oocytes independent of kinetochore geometry. Whereas ~49% of kinetochores were polar attached in young oocytes in mid meiosis-I, only ~28% were polar attached in aged oocytes, with a corresponding increase in meiotic–merotelic and (predominantly) lateral attachments. Thus, establishment of correct attachments, which occurs gradually during meiosis-I,Citation22,Citation27,Citation29 is delayed in aged oocytes. Given that the duration of meiosis-I is not altered in old oocytesCitation13,Citation23 (plus present study), failure to establish correct attachments prior to anaphase presents a risk of aneuploidy. Cold-stable microtubules were previously labeled in aged oocytes from B6D2F1/J mice, but such a difference in attachment was not noted. It is possible that the large numbers of attachments assessed in the current study have enabled us to uncover this difference, or that the age at which different aspects of the aging phenotype become evident differs between strains. Our study therefore introduces defective MT–kinetochore interactions as an age-related lesion that provides an explanation for gains and losses of sister chromosome pairs in meiosis-I.
Attachment errors and misalignment as causes of aneuploidy
The possible involvement of meiotic–merotelic attachments in oocyte aneuploidy has been the focus of considerable speculation. In somatic (mitotic) cells merotelic attachments are hazardous, as they go unnoticed by the spindle assembly checkpoint, but can lead to aneuploidy.Citation7,Citation9 Consistent with a role for equivalent meiotic–merotelic attachments in meiosis-I aneuploidy, lagging chromosomes are common in oocytes from old mothers;Citation13,Citation14 treatments that might promote meiotic–merotelic attachments can promote aneuploidy in oocytes,Citation30,Citation31 and misattachments have been directly observed in meiosis-I in young mice.Citation22,Citation32 Here we find that meiotic–merotelic attachments are relatively uncommon in young mice in mid-late meiosis-I (<4%), consistent with a previous report,Citation32 perhaps explaining why oocytes from young mice correctly segregate chromosomes even after inhibition or depletion of the MT-attachment-error corrector MCAK.Citation25 Importantly, however, we show that the number of meiotic–merotelic attachments is substantially elevated in old mice (~10%). Whether this indicates increased establishment of erroneous attachments, or a failure to correct them, will be important to resolve. Nonetheless, this suggests that the error correction pathway that is essential in somatic cellsCitation33 may be important in aged oocytes.
Our data unexpectedly reveal that lateral kinetochore–MT interactions are common in mid meiosis-I both in young and aged oocytes. In somatic cells, sliding of laterally attached kinetochores can facilitate chromosome congression prior to formation of end-on k-fibers.Citation34-Citation36 We therefore envisage that interactions with unstable MTs and lateral interactions with stable MT serve to position and orient bivalents prior to the establishment of stable end-on kinetochore–MT interactions, which occurs in mid-late MI.Citation27,Citation29 Such a process would explain the relative paucity of bona fide meiotic–merotelic attachments in young oocytes. Increased lateral and meiotic–merotelic attachments and fewer polar-attached kinetochores in aged oocytes therefore indicates a failure of timely establishment of end-on attachments. Whether chromosomes that remain laterally attached at anaphase onset are mis-segregated remains to be determined.
In addition to defective microtubule attachments, our experiments reveal that aged oocytes exhibit increased rates of chromosome misalignment in mid meiosis-I. The dynamics of chromosome and kinetochore sorting in meiosis-I have recently been addressed in great detail in young mouse oocytes, revealing that chromosomes are arranged into a rudimentary metaphase plate at an early stage of spindle assembly prior to elongation to form a barrel-shaped spindle.Citation22 Thus, as such, chromosome congression and alignment in the conventional sense do not occur in mammalian oocyte meiosis-I. Chromosomes found close to spindle poles in aged oocytes are thus likely to have drifted from the metaphase plate after spindle bipolarization. Pole-ward chromosome drifting in meiosis-I has been observed to occur occasionally in young mouse oocytes using live imaging,Citation37 and anaphase can occur in the presence of polar chromosomes in oocyte meiosis-I,Citation29,Citation37,Citation38 presumably resulting in the inheritance of entire bivalents by one daughter cell. Thus, failure to anchor bivalents at the metaphase plate presents a mis-segregation risk in aged oocytes.
Distinct routes to aneuploidy in meiosis-I and meiosis-II
Whereas our data indicate that cohesion loss makes only a minor contribution to attachment errors in MI, our data indicate a substantial impact of cohesion loss in meiosis-II, since premature sister chromatid individualisation causes chromosome misalignment in Met-II eggs. Although congestion at the metaphase plate makes it difficult to discount the possibility that some individualized sisters align correctly, those that were misaligned were in all cases individualized chromatids. Misaligned sister chromatids were always attached either directly to the adjacent pole or laterally to a passing MT fiber and would, therefore, presumably be inherited by the adjacent spindle pole at anaphase-II. Loss of chromosome cohesion thus presents a simple explanation for the increase in meiosis-II segregation error that occurs with advancing maternal age.
To summarize we present a working model (see ) in which MT mis-attachment and chromosome mis-alignment are defective in meiosis-I in aged oocytes. Both lesions provide a potential route to aneuploidy, but neither are fully accounted for by cohesion loss. Importantly, this model for the first time provides a cogent explanation as to how each type of mis-segregation event observed in aging mouse and human oocytes occurs. In contrast, premature sister individualization in Met-II eggs is a direct result of cohesion loss, and provides a simple explanation for gains and losses of single chromatids in meiosis-II. Establishing why kinetochores attach to microtubules erroneously in meiosis-I is an essential next step in understanding age-related infertility.
Figure 5. A 2-step model for chromosome mis-segregation in oocytes from older females. In meiosis-I there is a failure of timely establishment of polar MT attachments and an increased incidence of misattachment (erroneously attached MTs are highlighted in red), including meiotic–merotelic attachment and lateral attachments (not depicted). Misattachments occur both when kinetochore pairs remain tightly coherent, or are partially dissociated (illustrated in the purple box). If uncorrected at the time of anaphase onset, these attachments would cause dyads to experience “pulling forces” from both poles, which may result in a lagging chromosome (see the blue box). We speculate that were meiotic–merotelic attachment to occur at a sister pair where cohesion loss was substantial, anaphase forces could conceivably cause sisters to dissociate in meiosis-I, causing losses and gains of individual chromatids in meiosis-I (green box). In meiosis-II, individualized chromatids misalign at the spindle poles in metaphase (Met-II, red box) and are then inherited at the proximal spindle pole (orange box). Loss of coherence means that mechanisms to ensure that sisters are dispatched to opposite poles are absent. Any individualized chromatids that remain near or at the spindle equator likely segregate randomly (black boxes). Thus, cohesion loss is not the major cause of sister chromosome pair (dyad) mis-segregation in meiosis-I, but is directly responsible for aneuploidy in meiosis-II.
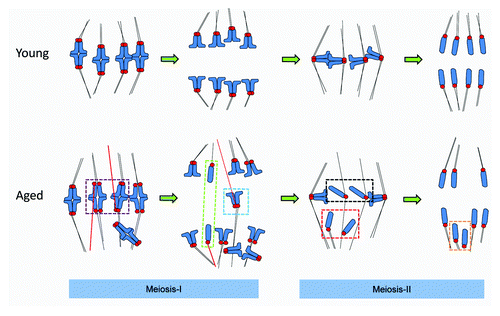
Materials and Methods
Oocyte collection and culture
Oocytes were collected from the ovaries of MF1 mice (Harlan) that were administered with 7IU of pregnant mares serum gonadotrophin (PMSG; Intervet) 44–48 h earlier. “Young” mice were delivered at 3–4-wk-of-age, and experiments performed ~2 wk later. Aged mice were ex-breeders, delivered at 40-wk-of-age and maintained for a further 6–8 mo. All experiments were performed with young and aged oocytes that were collected and cultured simultaneously. Oocytes were released from the ovaries using a 0.22-guage needle into M2 media (Sigma). Following collection, oocytes were immediately transferred to M16 media at 37 °C/5%CO2. To examine the timings of germinal vesicle breakdown (GVBD) and polar body emission (Pb1), oocytes cultured in M16 were examined on a stereoscope nearby the incubator, and the culture dish was exposed to ambient air for ~30 s per examination. Examinations were performed at 30-min intervals.
Immunofluorescence and imaging
Oocytes were fixed in a solution of 4% paraformaldehyde and 0.25% triton-X in PBS for 40 min at 25 °C. Blocking was performed in PBS with 3% BSA for 60 min at 37 °C. All subsequent handling was performed in PBS supplemented with 1% BSA. Antibodies used for immunolabelling: CREST (1:300 gift from William Earnshaw) and mouse anti-tubulin (1/1000, Sigma). Alexa-labeled secondary antibodies (Invitrogen) were used as appropriate. For analysis of cold-stable microtubules, oocytes were exposed to ice-cold M2 media for 10 min immediately prior to fixation, as previously.Citation39 DNA was labeled using 10-min incubation in Hoechst. Spindle imaging was performed in PBS/1%BSA in a glass-bottomed dish. Only spindles planar to the coverslip were examined. For in situ chromosome counts, MII eggs were exposed to 200 µM monastrol (Calbiochem) for 90 min, as previously.Citation25 Oocytes were subsequently fixed, labeled with CREST and Hoechst, as above, and mounted on microscope slides for imaging. All imaging was performed on a Zeiss 710 laser-scanning confocal microscope, as previously described.Citation40
Data analysis
All analysis was performed in ImageJ. Kinetochore pairs for which a pixel intensity linescan yielded a bimodal curve were deemed to be “dissociated”, and assessment was confirmed by examining serial confocal z-planes. For assessment of microtubule attachment, kinetochores were characterized as polar attached, unattached, laterally attached, or meiotic-merotelically attached, as described in the results. Analysis was performed blinded and subsequently confirmed by an independent investigator. CHICitation2 or Student t test were used as appropriate.
Disclosure of Potential Conflicts of Interest
No potential conflicts of interest were disclosed.
Acknowledgments
Funded by a Project Grant from the Medical Research Council to G.F. M.S. was funded by a studentship from the Society for Reproduction and Fertility. We thank Jenny Bormann and Katie Howe for excellent technical assistance.
References
- Hassold T, Hunt P. To err (meiotically) is human: the genesis of human aneuploidy. Nat Rev Genet 2001; 2:280 - 91; http://dx.doi.org/10.1038/35066065; PMID: 11283700
- Nagaoka SI, Hassold TJ, Hunt PA. Human aneuploidy: mechanisms and new insights into an age-old problem. Nat Rev Genet 2012; 13:493 - 504; http://dx.doi.org/10.1038/nrg3245; PMID: 22705668
- Compton DA. Spindle assembly in animal cells. Annu Rev Biochem 2000; 69:95 - 114; http://dx.doi.org/10.1146/annurev.biochem.69.1.95; PMID: 10966454
- Walczak CE, Cai S, Khodjakov A. Mechanisms of chromosome behaviour during mitosis. Nat Rev Mol Cell Biol 2010; 11:91 - 102; PMID: 20068571
- Santaguida S, Musacchio A. The life and miracles of kinetochores. EMBO J 2009; 28:2511 - 31; http://dx.doi.org/10.1038/emboj.2009.173; PMID: 19629042
- Maiato H, Lince-Faria M. The perpetual movements of anaphase. Cell Mol Life Sci 2010; 67:2251 - 69; http://dx.doi.org/10.1007/s00018-010-0327-5; PMID: 20306325
- Thompson SL, Compton DA. Chromosome missegregation in human cells arises through specific types of kinetochore-microtubule attachment errors. Proc Natl Acad Sci U S A 2011; 108:17974 - 8; http://dx.doi.org/10.1073/pnas.1109720108; PMID: 21997207
- Cimini D, Howell B, Maddox P, Khodjakov A, Degrassi F, Salmon ED. Merotelic kinetochore orientation is a major mechanism of aneuploidy in mitotic mammalian tissue cells. J Cell Biol 2001; 153:517 - 27; http://dx.doi.org/10.1083/jcb.153.3.517; PMID: 11331303
- Salmon ED, Cimini D, Cameron LA, DeLuca JG. Merotelic kinetochores in mammalian tissue cells. Philos Trans R Soc Lond B Biol Sci 2005; 360:553 - 68; http://dx.doi.org/10.1098/rstb.2004.1610; PMID: 15897180
- Musacchio A, Salmon ED. The spindle-assembly checkpoint in space and time. Nat Rev Mol Cell Biol 2007; 8:379 - 93; http://dx.doi.org/10.1038/nrm2163; PMID: 17426725
- Lee J, Kitajima TS, Tanno Y, Yoshida K, Morita T, Miyano T, Miyake M, Watanabe Y. Unified mode of centromeric protection by shugoshin in mammalian oocytes and somatic cells. Nat Cell Biol 2008; 10:42 - 52; http://dx.doi.org/10.1038/ncb1667; PMID: 18084284
- Pan H, Ma P, Zhu W, Schultz RM. Age-associated increase in aneuploidy and changes in gene expression in mouse eggs. Dev Biol 2008; 316:397 - 407; http://dx.doi.org/10.1016/j.ydbio.2008.01.048; PMID: 18342300
- Lister LM, Kouznetsova A, Hyslop LA, Kalleas D, Pace SL, Barel JC, Nathan A, Floros V, Adelfalk C, Watanabe Y, et al. Age-related meiotic segregation errors in mammalian oocytes are preceded by depletion of cohesin and Sgo2. Curr Biol 2010; 20:1511 - 21; http://dx.doi.org/10.1016/j.cub.2010.08.023; PMID: 20817533
- Chiang T, Duncan FE, Schindler K, Schultz RM, Lampson MA. Evidence that weakened centromere cohesion is a leading cause of age-related aneuploidy in oocytes. Curr Biol 2010; 20:1522 - 8; http://dx.doi.org/10.1016/j.cub.2010.06.069; PMID: 20817534
- Merriman JA, Jennings PC, McLaughlin EA, Jones KT. Effect of aging on superovulation efficiency, aneuploidy rates, and sister chromatid cohesion in mice aged up to 15 months. Biol Reprod 2012; 86:49; PMID: 22053097
- Liu L, Keefe DL. Defective cohesin is associated with age-dependent misaligned chromosomes in oocytes. Reprod Biomed Online 2008; 16:103 - 12; http://dx.doi.org/10.1016/S1472-6483(10)60562-7; PMID: 18252055
- Chiang T, Schultz RM, Lampson MA. Age-dependent susceptibility of chromosome cohesion to premature separase activation in mouse oocytes. Biol Reprod 2011; 85:1279 - 83; http://dx.doi.org/10.1095/biolreprod.111.094094; PMID: 21865557
- Duncan FE, Hornick JE, Lampson MA, Schultz RM, Shea LD, Woodruff TK. Chromosome cohesion decreases in human eggs with advanced maternal age. Aging Cell 2012; 11:1121 - 4; http://dx.doi.org/10.1111/j.1474-9726.2012.00866.x; PMID: 22823533
- Jones KT, Lane SI. Molecular causes of aneuploidy in mammalian eggs. Development 2013; 140:3719 - 30; http://dx.doi.org/10.1242/dev.090589; PMID: 23981655
- Howe K, FitzHarris G. Recent insights into spindle function in mammalian oocytes and early embryos. Biol Reprod 2013; 89:71; http://dx.doi.org/10.1095/biolreprod.113.112151; PMID: 23966320
- Gregan J, Polakova S, Zhang L, Tolić-Nørrelykke IM, Cimini D. Merotelic kinetochore attachment: causes and effects. Trends Cell Biol 2011; 21:374 - 81; http://dx.doi.org/10.1016/j.tcb.2011.01.003; PMID: 21306900
- Kitajima TS, Ohsugi M, Ellenberg J. Complete kinetochore tracking reveals error-prone homologous chromosome biorientation in mammalian oocytes. Cell 2011; 146:568 - 81; http://dx.doi.org/10.1016/j.cell.2011.07.031; PMID: 21854982
- Duncan FE, Chiang T, Schultz RM, Lampson MA. Evidence that a defective spindle assembly checkpoint is not the primary cause of maternal age-associated aneuploidy in mouse eggs. Biol Reprod 2009; 81:768 - 76; http://dx.doi.org/10.1095/biolreprod.109.077909; PMID: 19553597
- Stein P, Schindler K. Mouse oocyte microinjection, maturation and ploidy assessment. J Vis Exp 2011; 53; PMID: 21808228
- Illingworth C, Pirmadjid N, Serhal P, Howe K, Fitzharris G. MCAK regulates chromosome alignment but is not necessary for preventing aneuploidy in mouse oocyte meiosis I. Development 2010; 137:2133 - 8; http://dx.doi.org/10.1242/dev.048306; PMID: 20504960
- Zhai Y, Kronebusch PJ, Borisy GG. Kinetochore microtubule dynamics and the metaphase-anaphase transition. J Cell Biol 1995; 131:721 - 34; http://dx.doi.org/10.1083/jcb.131.3.721; PMID: 7593192
- Davydenko O, Schultz RM, Lampson MA. Increased CDK1 activity determines the timing of kinetochore-microtubule attachments in meiosis I. J Cell Biol 2013; 202:221 - 9; http://dx.doi.org/10.1083/jcb.201303019; PMID: 23857768
- Selesniemi K, Lee HJ, Muhlhauser A, Tilly JL. Prevention of maternal aging-associated oocyte aneuploidy and meiotic spindle defects in mice by dietary and genetic strategies. Proc Natl Acad Sci U S A 2011; 108:12319 - 24; http://dx.doi.org/10.1073/pnas.1018793108; PMID: 21730149
- Gui L, Homer H. Spindle assembly checkpoint signalling is uncoupled from chromosomal position in mouse oocytes. Development 2012; 139:1941 - 6; http://dx.doi.org/10.1242/dev.078352; PMID: 22513372
- Lane SI, Chang HY, Jennings PC, Jones KT. The Aurora kinase inhibitor ZM447439 accelerates first meiosis in mouse oocytes by overriding the spindle assembly checkpoint. Reproduction 2010; 140:521 - 30; http://dx.doi.org/10.1530/REP-10-0223; PMID: 20660090
- Merriman JA, Lane SI, Holt JE, Jennings PC, García-Higuera I, Moreno S, McLaughlin EA, Jones KT. Reduced chromosome cohesion measured by interkinetochore distance is associated with aneuploidy even in oocytes from young mice. Biol Reprod 2013; 88:31; http://dx.doi.org/10.1095/biolreprod.112.104786; PMID: 23255336
- Yang KT, Li SK, Chang CC, Tang CJ, Lin YN, Lee SC, Tang TK. Aurora-C kinase deficiency causes cytokinesis failure in meiosis I and production of large polyploid oocytes in mice. Mol Biol Cell 2010; 21:2371 - 83; http://dx.doi.org/10.1091/mbc.E10-02-0170; PMID: 20484572
- Compton DA. Mechanisms of aneuploidy. Curr Opin Cell Biol 2011; 23:109 - 13; http://dx.doi.org/10.1016/j.ceb.2010.08.007; PMID: 20810265
- Kapoor TM, Lampson MA, Hergert P, Cameron L, Cimini D, Salmon ED, McEwen BF, Khodjakov A. Chromosomes can congress to the metaphase plate before biorientation. Science 2006; 311:388 - 91; http://dx.doi.org/10.1126/science.1122142; PMID: 16424343
- Cai S, O’Connell CB, Khodjakov A, Walczak CE. Chromosome congression in the absence of kinetochore fibres. Nat Cell Biol 2009; 11:832 - 8; http://dx.doi.org/10.1038/ncb1890; PMID: 19525938
- Wignall SM, Villeneuve AM. Lateral microtubule bundles promote chromosome alignment during acentrosomal oocyte meiosis. Nat Cell Biol 2009; 11:839 - 44; http://dx.doi.org/10.1038/ncb1891; PMID: 19525937
- Lane SI, Yun Y, Jones KT. Timing of anaphase-promoting complex activation in mouse oocytes is predicted by microtubule-kinetochore attachment but not by bivalent alignment or tension. Development 2012; 139:1947 - 55; http://dx.doi.org/10.1242/dev.077040; PMID: 22513370
- Kolano A, Brunet S, Silk AD, Cleveland DW, Verlhac MH. Error-prone mammalian female meiosis from silencing the spindle assembly checkpoint without normal interkinetochore tension. Proc Natl Acad Sci U S A 2012; 109:E1858 - 67; http://dx.doi.org/10.1073/pnas.1204686109; PMID: 22552228
- FitzHarris G, Anaphase B. Anaphase B precedes anaphase A in the mouse egg. Curr Biol 2012; 22:437 - 44; http://dx.doi.org/10.1016/j.cub.2012.01.041; PMID: 22342753
- Howe K, FitzHarris G. A non-canonical mode of microtubule organization operates throughout pre-implantation development in mouse. Cell Cycle 2013; 12:1616 - 24; http://dx.doi.org/10.4161/cc.24755; PMID: 23624836