Abstract
In budding yeast, septins are assembled into structures that undergo dramatic changes during the cell cycle. The molecular mechanisms that drive these remodelings are not fully uncovered. In this study, we describe a characterization of Vhs2, a nonessential protein that revealed to be a new player in septin dynamics. In particular, we report that Vhs2 is important to maintain the stability of the double septin ring structure until telophase. In addition, we show that Vhs2 undergoes multiple phosphorylations during the cell cycle, being phosphorylated during S phase until nuclear division and dephosphorylated just before cell division. Importantly we report that cyclin-dependent protein kinase Cdk1 and protein phosphatase Cdc14 control these Vhs2 post-translational modifications. These results reveal that Vhs2 is a novel Cdc14 substrate that is involved in the control of septin organization.
Keywords: :
Introduction
Septins are conserved GTP-binding proteins that were first discovered in S. cerevisiae as “neck filaments” surrounding the bud neck.Citation1 These proteins are conserved from yeast to human but are absent in plants,Citation2 even if the number of septin genes varies between different organisms. The budding yeast expresses 7 septins: Cdc3, Cdc10, Cdc11, Cdc12, Shs1 (present during the mitotic cell cycle), Spr3, and Spr28 (specific for the meiotic process where they replace Cdc12 and Shs1, respectively).Citation3,Citation4 These proteins can form rod-shaped hetero-oligomeric complexes, which polymerize end-to-end into long paired linear filaments. These filaments are further assembled into rings or hourglass structures during different phases of the cell cycle.Citation5 These structures are associated with actin and tubulin cytoskeleton and localize to a distinct region of the plasma membrane, where they act as scaffold and diffusion barrier to mediate several processes, including cell cycle checkpoints, mitosis, cytokinesis, cell polarity, and exocytosis.Citation5 The regulation of septin architecture is very complex, and the fidelity of this process is important for septin function. In addition, the dynamics of these structures must be temporally coordinated with all the other cell cycle events, particularly with nuclear division.
In budding yeast, at the beginning of a new cell cycle, a septin ring is assembled at the presumptive bud site, and this complex is dynamic, as indicated by FRAP analysis.Citation6 Coincident with bud emergence, or shortly after, the septin ring expands into a stable hourglass structure that surrounds the bud neck. At the onset of cytokinesis, the septin hourglass structure is split into 2 dynamic rings that sandwich the cytokinesis machinery. All these morphological changes are driven by cyclin-dependent protein kinase 1 (Cdk1), polarity factors and post-translational modifications of septins (phosphorylation and SUMOylation) that regulate septin organization and dynamics. An initial septin ring assembly requires the action of the polarity factor Cdc42 and direct phosphorylation of septins by G1 cyclin-bound Cdk.Citation7,Citation8 Septin ring transition into a stable hourglass structure is driven by the phosphorylation of septins by 2 bud neck-localized kinases: the PAK kinase Cla4 and the Nim1-related kinase Gin4.Citation9-Citation11 Later in the cell cycle, the septin hourglass structure splitting is controlled by the mitotic exit network (MEN) activity.Citation12 This network allows Cdc14 phosphatase full activation that, in turn, causes the inactivation of mitotic Cdk1; this event seems sufficient to cause septin hourglass splitting.Citation12,Citation13 After cytokinesis, the old septin ring at the division site is disassembled, and a new septin ring is formed adjacent to the old one.Citation14 The correct old septin ring disassembly is important for the assembly and the function of the new ring, since the septin subunits are recycled in order to build the new structure.Citation15 Disassembly of the old septin ring appears to be regulated by dephosphorylation events driven by the protein phosphatase PP2ARts1.Citation6 In addition, septins are controlled by SUMOylation, and the inhibition of this process causes septin ring stabilization;Citation16,Citation17 however, the significance of septin SUMOylation and the interconnections with other modifications remain unclear. The picture of septin regulation in yeast is very well detailed, but several issues remain undetermined. Among these, a crucial point is how septin structure stability is maintained until chromosomes are properly segregated to opposite poles.
Vhs2 is a 436-amino acid protein encoded by YIL135c ORF. It is a cytoplasmic protein of unknown function; it has no known enzimatic activity; and its sequence does not have any conserved domain that can shed light on its cellular function.Citation18 Vhs2 was identified as a high-copy number suppressor of the synthetic lethality of a hal3 sit4 double mutant,Citation19 inviable because of an irreversible G1 arrest, suggesting a role for Vhs2 in G1/S-phase progression. However this issue has not been further investigated. Vhs2 was also found as a high-copy number suppressor of gic1 gic2 thermosensitivity;Citation20 these proteins are Cdc42 effectors that promote polarized cell growth, and the deletion of both GIC1 and GIC2 causes the accumulation of enlarged and round cells and results in cell lethality at elevated temperatures. Therefore, Vhs2 was implicated in the regulation of the actin cytoskeleton polarization in a parallel pathway with respect to GIC1 and GIC2. VHS2 has a paralog, MLF3, likely arisen by gene duplication;Citation21 their primary sequences share 30% identity and 42% of similarity.Citation20 However, the function of Vhs2 and Mlf3 have not been characterized, and their sequences provide no obvious clues regarding their cellular functions. Previous analysis indicates that Vhs2 helps to regulate the actin cytoskeleton, cell wall integrity, and polarized cell growth.Citation20
In this paper, we report evidence that Vhs2 is involved in the regulation of septin dynamics at the bud neck after mitotic entry, likely by stabilizing septin structure. In addition, we show that Vhs2 is a protein regulated at post-translational level by cell cycle-dependent phosphorylation. In particular, Vhs2 is likely phosphorylated by Cdk1 and dephosphorylated by Cdc14 before cytokinesis.
Results
The lack and the overproduction of Vhs2 do not alter cell cycle progression
We previously demonstrated that the Dma2 ubiquitin ligase acts as a negative regulator of cytokinesis by controlling actomyosin ring contraction and primary septum deposition in a parallel pathway with respect to Hof1.Citation22 Indeed, Dma2 excess causes cytokinesis failure in Hof1-lacking cells, leading to their arrest as chains of cells with connected cytoplasms. In order to find new cytokinesis regulators, we performed a genetic screen looking for multicopy suppressors of the GAL1-DMA2 hof1Δ lethality on galactose-containing platesCitation22 by transformation with a library of yeast episomic plasmids containing DNA inserts of the yeast genome (see “Materials and Methods”). We isolated 14 library plasmids that allowed the GAL1-DMA2 hof1Δ strain to grow on YEPRG plates, one of them contained a piece of chromosome IX including several genes, among which VHS2 seemed to be involved in the suppression phenotype.
In order to functionally characterize its gene product, we deleted VHS2 in wild-type haploid cells. The vhs2Δ strain turned out to be viable and therefore suitable for further analysis. To investigate the role of Vhs2 in the yeast cell cycle, we synchronized wild-type and vhs2Δ cells in G1, followed by release into the cell cycle at 25 °C. α-factor was readded after 95% of cells were budded in order to arrest them at the following G1 phase. After the release, the kinetics of DNA replication (), budding, nuclear division, and cytokinesis () showed a very similar profile in both strains. So the lack of Vhs2 does not significantly alter the progression of an unperturbed cell cycle. We also tested if vhs2Δ cells were sensitive to several kinds of stress: osmotic (NaCl 500 mM or 1 M), replication stress (hydroxyurea 100 mM or 200 mM) and mitotic spindle damage (Benomyl 10 µg/ml or 15 µg/ml), but vhs2Δ cells did not show any sensitivity (data not shown).
Figure 1. The lack and the overproduction of Vhs2 do not significantly alter cell cycle progression. (A and B) Exponentially YEPD growing cultures of wild-type and vhs2Δ cells were arrested in G1 by α-factor and released from G1 arrest at 25 °C (time 0). At the indicated times after release, cell samples were taken for FACS analysis of DNA contents (A) and for scoring budding and nuclear division (B). (C and D) Exponentially YEPR growing cultures of wild-type cells and cells carrying multiple integration of GAL1-VHS2 construct were arrested in G1 by α-factor and released from G1 arrest in YEPRG at 25 °C (time 0). At the indicated times after release, cell samples were taken for FACS analysis of DNA contents (C) and for scoring budding and nuclear division (D).
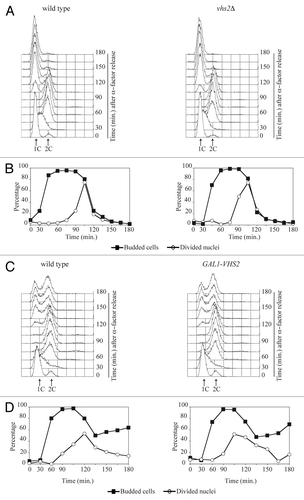
In addition, we also analyzed the effects of the galactose-induced overexpression of VHS2 (GAL1-VHS2) from single or multiple copy integration in the yeast genome, but we observed that it does not cause evident defects, neither on cell viability (data not shown) nor in cell cycle progression of cells released from a G1 arrest into galactose-containing medium ( and data not shown).
Since VHS2 was isolated as possible multicopy suppressor of the lethal cytokinetic defect of the GAL1-DMA2 hof1Δ combination, we looked for synthetic effects between the VHS2 deletion and mutations in genes implicated in cytokinesis. We analyzed the growth ability of different double mutants derived from meiotic segregants of the diploids obtained by crossing vhs2Δ cells with iqg1-1, ase1Δ, cyk3Δ, and chs2Δ cells. None of the double mutants showed a synthetic growth defect on YEPD plates at different temperatures or accumulation of misshapened or chained cells in liquid medium (data not shown). Taken together, these data suggest that Vhs2 is not involved in cytokinesis control.
The lack of Vhs2 causes septin ring instability
Since septins are involved in cytokinesis, and Vhs2 seems to interact with these proteins (data from high-throughput screening described in Costanzo et al., 2010),Citation23 we decided to test if VHS2 deletion could have a synthetic effect in combination with mutations that perturb septin structure. By tetrad dissection of the appropriate diploids, we identified strains containing vhs2Δ and different septin mutant alleles (cdc3–1, cdc10–1, cdc12–1, cdc12–6, shs1Δ, shs1–100c) and strains carrying the deletion of both VHS2 and RTS1, the regulatory subunit of protein phosphatase 2A involved in septin ring stability.Citation6 The spores carrying the deletion of VHS2 combined with cdc3–1 and cdc12–6 formed a very small colony (not shown); however, the double mutants were available for further analysis. All the double mutants and control strains were inoculated in YPD at 25 °C, and serial diluitions of these cultures were spotted on YEPD plates and incubated at different temperatures (). Interestingly, VHS2 deletion showed synthetic growth defects in combination with several mutants known to alter the septin structures: cdc3–1, cdc12–6, shs1Δ, shs1–100c, and rts1Δ (), while it did not cause any effect when combined with cdc10–1 or cdc12–1 alleles that do not destabilize septin ring at the bud neck (). These data suggest that Vhs2 might be involved in septin dynamics.
Figure 2. The lack of Vhs2 causes synthetic growth defects in combination with septin mutants. Serial dilutions of stationary phase cultures of strains with the indicated genotypes were spotted on YEPD plates, which were then incubated for 2 d at the indicated temperatures.
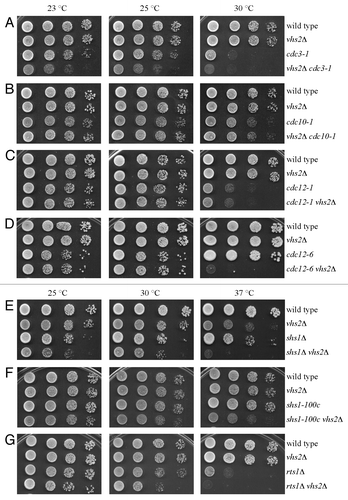
Then we analyzed the shape, the actin localization, and the septin ring at the bud neck in the above-mentioned double mutants that share a synthetic growth defect with VHS2 deletion, grown at different temperatures. Double mutants and control cell cultures were inoculated in YEPD at 25 °C and then shifted to 30 °C or 37 °C overnight, and below we present a description of the phenotypes of double mutants at the temperature at which the corresponding septin mutants have a normal shape. Interestingly, we observed that vhs2Δ cells show a normal actin distribution but a hyperpolarized bud growth when shifted at 37 °C (), suggesting that Vhs2 might have a role in septin organization (see below). Despite being able to form colonies at 25 °C, vhs2Δ cdc12–6 cells exhibit an aberrant shape (), and this phenotype gets worse at higher temperatures (not shown). vhs2Δ cdc3–1 double mutant shows some cells with elongated and distorted buds at 25 °C, while they form large clumps with broad bud necks at 30 °C (). vhs2Δ shs1Δ and vhs2Δ rts1Δ accumulated clusters of elongated cells only at 37 °C (), while vhs2Δ shs1–100c cells showed a normal shape at all temperatures. These data are consistent with the notion that perturbations of septin structures often cause the accumulation of misshapened cells.Citation24 Actin staining of the same cells indicates that all the growth defects (that are reflected or not in an aberrant shape) observed in the double mutants grown at the relative restrictive temperatures are not ascribable to an actin polarization defect. All the double mutants were unable to assemble normal septin structures at the bud neck, but this phenotype was difficult to quantify, since the single septin mutants showed severe defects, per se, even at 25 °C (data not shown). Taken together these data indicate that the growth defects of the combination of the VHS2 deletion with septin mutants might be due to an exacerbation of the defect in septin organization.
Figure 3. Cell morphology and actin deposition in several double mutants. Exponentially growing cultures of cells with the indicated genotype were grown at 25 °C in YEPD (A) and then shifted to 30 °C (B) or 37 °C (C) overnight. Cell samples were taken to visualize cell shape and to analyze actin patches, cables, and rings by using rhodamine–phalloidine staining. Bar: 5 μm.
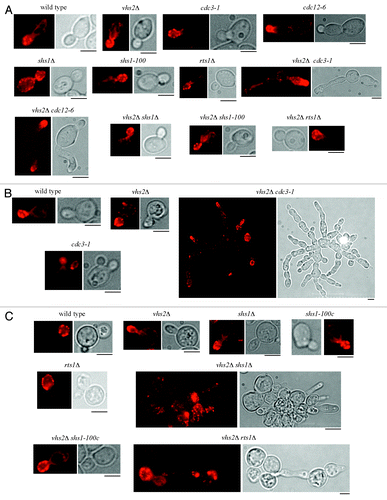
We then carefully analyzed septin dynamics of vhs2Δ cycling cells respect to wild-type cells grown at 25 °C. We did not detect any obvious defect in septin ring assembly or dynamics until mitotic exit (), but we observed that 30.7% of vhs2Δ dumbbell cells with divided nuclei exhibited long mitotic spindle but no septin structures, while only 11.3% of wild-type cells showed this phenotype (), suggesting that vhs2Δ cells disassemble septin ring earlier than wild-type cells. This defect was more evident in vhs2Δ cells grown at 37 °C; indeed, 71% of dumbbell cells with long mitotic spindle had no septin ring at the bud neck, compared to 34% of wild-type cells with the same phenotype (our observation). This phenotype is consistent with the observation that vhs2Δ cells show hyperpolarized bud growth when shifted at 37 °C ().
Figure 4. The lack of Vhs2 affects septin ring dynamics. (A and B) In situ immunofluorescence analysis of nuclei (DAPI), mitotic spindles (MTs), and septin ring deposition (Cdc11) of exponentially YEPD growing cultures of wild-type and vhs2Δ cells at 25 °C. (C) Exponentially growing cultures of wild-type and vhs2Δ cells, all expressing fully functional SHS1-Cherry and TUB1-GFP fusions, were arrested in G1 by α-factor and released from G1 arrest at 25 °C in synthetic medium plates for time-lapse analysis. Pictures as shown in the panel were taken every 2 min for 5 h, and time-lapsed images were assembled to show Shs1–Cherry and Tub1–GFP dynamics; time = 0 is the time of mitotic spindle breakdown occurrence (see also Videos S1 and S2). Bar: 5 μm.
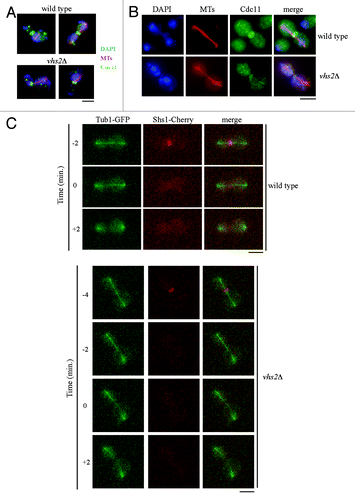
In order to analyze in detail this phenotype, we generated wild-type and vhs2Δ strains expressing a functional fluorescent variant of the septin Shs1 (Shs1-Cherry) and the mitotic spindle marker Tub1-GFP. We then performed live-cell imaging of wt and vhs2Δ syncronized cell cultures released from a G1 arrest. We observed that the septin ring disappeared from the bud neck of wild-type cells almost concomitantly (+0,23 minutes, mean of 71 cells) with mitotic spindle breakdown (considered time 0), while in cells that lack Vhs2, septin ring is displaced before this event (−2,25 min, mean of 51 cells) (; Video S1 and S2). The difference between the 2 strains is significant (P value obtained by Wilcoxon rank-sum test P = 43311 10−11). Interestingly, also in this experiment, we observed that, considering dumbbell cells with long mitotic spindle, 84% of vhs2Δ cells (54 over 71) do not show assembled septin structures at the bud neck vs. 25% of wild-type cells (12 over 51).
Together, these data suggest that the lack of Vhs2 affects septin ring dynamics; in particular, Vhs2 seems to stabilize the double ring structure before and during mitotic spindle breakdown.
Vhs2 levels and phosphorylation
Vhs2 was described as a multi-phosphorylated protein from high-throughput analysis,Citation25 but its levels and modifications have not been analyzed in vivo. We produced cells expressing a Vhs2-6HA-tagged variant, and we observed by western blot analysis that Vhs2 appears as multiple bands with different electroforetic mobility in denatured and native protein extracts (our data not shown). We then analyzed Vhs2 total levels and modifications during an unperturbed cell cycle. VHS2-6HA cells were inoculated in YEPD, arrested in G1 with α-factor, and released in fresh medium; when about 90–95% of the cells were budded, we readded α-factor in order to follow a single cell cycle. The analyzed cells progressed normally through the cell cycle (compare to cell cycle progression of wild-type cells in ) as indicated by FACS profiles of DNA content (), budding (data not shown), and nuclear division (). Vhs2 is always present throughout the cell cycle and appears as multiple bands; interestingly, some slower migrating bands are absent in G1-arrested cells, then they accumulate during S-G2 and decrease concomitantly with nuclear division (). Thus, Vhs2 is subjected to post-translational modifications that alter its electrophoretic mobility during the cell cycle, and these modifications decrease during mitosis.
Figure 5. Vhs2 undergoes multiple post-translational modifications during the cell cycle. (A and C) Exponentially growing cultures of VHS2-6HA cells were arrested in G1 by α-factor and released at 25 °C in YEPD medium (time = 0). At the indicated times, cell samples were taken for FACS analysis of DNA contents (A), for scoring nuclear division, and for determining Vhs2 levels and phosphorylation by western blot analysis with anti-HA and anti-Pgk1 (loading control) antibodies (C). (B and D) Exponentially growing cultures of VHS2-6HA cells were arrested in G2 by nocodazole (time = 0) and released at 25 °C in YEPD medium containing α-factor. At the indicated times, cell samples were taken for FACS analysis of DNA contents (B), for scoring nuclear division, and for determining Vhs2 levels and post-translational modifications by western blot analysis with anti-HA and anti-Pgk1 (loading control) antibodies (D). The 2 panels on the right show a higher exposure of the western blots presented on the left; they are cropped in order to better visualize the changes in Vhs2 mobility shift. Higher exposure of the western blots without cropping is shown in Figure S2A and B.
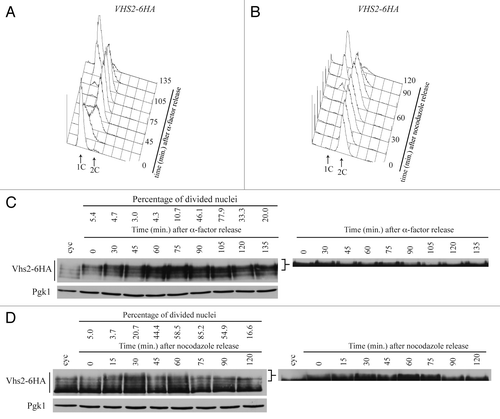
In order to better understand the timing of Vhs2 modifications during mitosis, VHS2-6HA cells were inoculated in YEPD, arrested in G2 with nocodazole, and released in fresh medium containing α-factor to block the cells in the following G1 phase. Samples were taken at different time points to monitor the kinetics of cell division (), nuclear division, and Vhs2 mobility by western blot analysis (). Accordingly to the previous experiment, in G2-arrested cells (time 0) some slower migrating bands are present, and they decrease starting from 75 min after the release, together with nuclear division.
In order to clarify if the slowly migrating Vhs2 species were due to phosphorylation events, as suggested by data from high-throughput analysis,Citation25 we performed an in vitro phosphatase assay. Vhs2-6HA was purified from total extract of yeast cycling cells by immunoprecipitation using anti-HA antibodies bound to protein A–sepharose resin, and the purified Vhs2-6HA was subjected to lambda phosphatase treatment for 7 min at 30 °C. The slower Vhs2 species disappeared after incubation with the phosphatase (Fig. S1), indicating that those bands are due to phosphorylation events, and accordingly, those bands disappear upon overexpression of a phosphatase in vivo (see below).
Since Vhs2 sequence contains a consensus phosphorylation site for Cdk1, and a large-scale mass spectrometry analysis indicates Vhs2 as a Cdk1 substrate,Citation26 we asked if Cdk1 could be involved in Vhs2 phosphorylation. To test this hypothesis, we analyzed the effect of inhibition of Cdc28 kinase activity in cells released from a G1 block. VHS2-6HA and VHS2-6HA cdc28-as1 (whose kinase activity is blocked by the ATP analog 1NM-PP1)Citation27 cells were arrested in G1 by α-factor and released either in the presence of the inhibitor or in DMSO. As shown in , in control cells (DMSO) Vhs2 accumulates slow migrating forms, while inhibition of Cdc28-as1 significantly reduces the appearance of Vhs2-phosphorylated bands, suggesting that Cdc28 may phosphorylate Vhs2 in vivo.
Figure 6. Vhs2 is dephosphorylated by the protein phosphatase Cdc14. (A) Exponentially growing cultures of VHS2-6HA and VHS2-6HA cdc28-as1 cells were arrested in G1 by α-factor (time = 0) and released in YEPD medium containing DMSO or 0.5 μM 1NM-PP1. At the indicated times, cell samples were taken for determining Vhs2 levels and phosphorylation by western blot analysis with anti-HA antibodies. The panel on the right show a higher exposure of the western blot presented on the left; it is cropped in order to better visualize the changes in Vhs2 mobility shift. Higher exposure of the western blot without cropping in shown in Figure S2C. (B) Cell cultures of VHS2-6HA, dbf2–2 VHS2-6HA and cdc14–1 VHS2-6HA strains were grown in YEPD medium at 25 °C to exponentially phase and then shifted to 37 °C for 2 h. Cell samples were taken for determining Vhs2 levels and phosphorylation by western blot analysis with anti-HA antibodies. (C) Cell cultures of GAL1-CDC14 VHS2-6HA and GAL1-cdc14-C283A VHS2-6HA strains were grown in YEPR medium at 25 °C, arrested in G2 with nocodazole, then galactose was added to induce GAL1 promoter activation. Cell samples were taken after 1 h and 2 h for determining Vhs2 levels and phosphorylation by western blot analysis with anti-HA antibodies. In (A–C) Pgk1 is a loading control. (D) Total protein extracts of VHS2-6HA CDC14–3FLAG, and CDC14–3FLAG strains (input) were incubated with anti-HA protein A-sepharose, separated by SDS-PAGE, and visualized by western blot with anti-HA and anti-FLAG (IP). The asterisk indicates the immunoglobulin heavy chain.
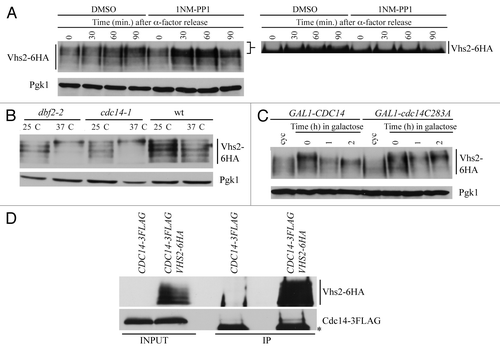
Data from another high-throughput screening analysis and from a proteome array screening indicated that Vhs2 could be phosphorylated by Dbf2 protein kinase,Citation28,Citation29 which is part of the MEN and functions during mitotic exit and cytokinesis. So we analyzed if Vhs2 levels and phosphorylation could change when Dbf2 is inactivated. To this purpose, we constructed a strain containing both VHS2-6HA and a thermo-sensitive allele of DBF2 that is inactive at the restrictive temperature of 37 °C (dbf2–2). VHS2-6HA and VHS2-6HA dbf2-2 cells were grown in YEPD at 25 °C and then shifted to 37 °C for 2 h. Cell arrest in telophase was controlled by FACS analysis of DNA content, cell morphology, and nuclear division (data not shown). The western blot shown in indicates that Vhs2 accumulates hyperphosphorylated forms when Dbf2 kinase is inactivated, indicating that this kinase is not responsible for Vhs2 phosphorylation that is instead brought about by other kinase(s) that are active before telophase. Since Vhs2 is still phosphorylated during telophase, and Vhs2 phosphorylation decreases together with nuclear division (), we wondered whether Vhs2 dephosphorylation could depend on a protein phosphatase that is active during mitotic exit and/or cytokinesis. For this reason, we chose the protein phosphatase Cdc14, which regulates several events during mitosis and cytokinesis by targeting multiple substrates. So we tested the effect of its lack on Vhs2 phosphorylation by the use of a thermo-sensitive allele of CDC14 (cdc14–1). VHS2-6HA cdc14–1 cells were grown in YEPD at 25 °C and shifted at the restrictive temperature (37 °C) for 2 h; in these conditions, Vhs2 accumulates hyperphosphorylated forms (), indicating that Cdc14 might regulate Vhs2 dephosphorylation directly or indirectly. This observation is consistent with the accumulation of Vhs2 hyperphosphorylated forms in dbf2-2 cells at the restrictive temperature (), since these cells fail to properly release from the nucleolus and activate Cdc14.Citation30
In order to better understand if Vhs2 could be directly dephosphorylated by Cdc14, we analyzed the phosphorylation state of Vhs2 upon overproduction of wild-type Cdc14 or of a phosphatase-dead variant (cdc14-C283A)Citation31 in G2, the phase of the cell cycle in which Vhs2 is heavily phosphorylated. VHS2-6HA GAL1-CDC14 and VHS2-6HA GAL1-cdc14-C283A cells were grown in YEPR at 25 °C, arrested in G2 by the addition of nocodazole, and then galactose was added to induce GAL1 promoter activation. By the observation of propidium iodide-stained cells, we made sure that the cells were arrested as dumbbell with one nucleus until the end of the experiment (not shown). As shown in , when Cdc14 is overproduced, Vhs2 slower electrophoretic mobility bands are reduced conversely, when the phosphatase-dead version of Cdc14 is overproduced, Vhs2 electrophoretic mobility is not altered. These data are in agreement with our previous findings (), which led us to hypothesize a role of Cdc14 phosphatase on Vhs2 dephosphorylation.
We then tested if Vhs2 could interact with Cdc14 in vivo by co-immunoprecipitation experiments using protein extracts from strains expressing both VHS2-6HA and Cdc14–3FLAG. Indeed, Vhs2 could immunoprecipitate specifically Cdc14 (; lane 4), indicating that they form a complex. Vhs2 can interact also with a phosphatase-dead variant of Cdc14 (our data not shown), indicating that this biochemical activity is not required for protein complex formation, and that the lack of dephosphorylation of Vhs2 in VHS2-6HA GAL1-cdc14-C283A cells shown in is not due to lack of interaction between the phosphatase and its substrate. Altogether, our data indicate that Vhs2 interacts with Cdc14, which, in turn, is able to revert Vhs2 phosphorylation.
Discussion
The coordination of nuclear division with the completion of cytokinesis is a vital issue for all eukaryotic cells in order to maintain genome stability. Septins form high-ordered structures that are dynamic and are required to preserve the integrity of the division site, to recruit proteins that signal proper cell cycle progression, and to ensure timely cytokinesis. Thus, the dynamics and the function of septins must be tightly coordinated with cell cycle progression. Nowadays several players in septin dynamics organization have been described that participate in the coordination of septin structure with cell cycle progression.
Budding yeast Vhs2 is a nonessential protein that has been poorly characterized. In this study, we carefully analyzed the phenotype of vsh2Δ cells, and we observed that the lack of Vhs2 did not cause evident defects in cell cycle progression. Interestingly, our genetic studies revealed that the lack of Vhs2 caused a synthetic growth defect in combination with septin mutants and with the lack of septin regulator Rts1, indicating a possible role of Vhs2 in regulating septin dynamics. Indeed, a deeper analysis of vhs2Δ cells phenotype revealed that the lack of Vhs2 caused a defect in septin dynamics at the bud neck. In particular, in the absence of Vhs2, the 2 septin rings, which usually persist until cell separation, are unstable and are disassembled earlier than in wild-type cells, i.e. when the mitotic spindle is not yet broken. This phenotype, which is more evident at high temperature, results in a hyperpolarization of bud growth, consistent with the notion that septin-defective cells fail to maintain the correct bud localization of polarity factors during isotropic bud growth, thus causing the formation of elongated buds.Citation32 This defect is tolerated by otherwise wild-type cells, and, accordingly, the persistence of the diffusion barrier formed by septins is not essential for execution of cytokinesis and for cell viability.Citation33
So, Vhs2 plays a role in mantaining septin structure stability after mitotic entry, but how Vhs2 participates in septin organization is not fully understood. Vhs2 could control septin structure stabilization by interfering with septin post-translational modifications. Since Shs1 dephosphorylation is important for septin ring destabilization during citokinesis,Citation6 we tested if the lack of Vhs2 could affect this process. However, through all the phases of an unperturbed cell cycle, Shs1 phosphorylated forms appearance and amount is very similar in the absence of Vhs2 with respect to wild-type cells (our data not shown), indicating that Vhs2 does not control Shs1 phosphorylation, and it may act independently of this event. In addition, septins Cdc3, Cdc11, and Shs1 undergo SUMOylation, and the inhibition of this process causes septin rings stabilization,Citation16,Citation17 so we tested if the presence of SUMOylation-deficient alleles of Cdc3, Cdc11, and Shs1 could revert the septin ring defect of vsh2Δ cells. Instead, the lack of septin SUMOylation does not cause any effect, indicating that Vhs2 could act upstream of this modification. A more precise understanding of the interplay between septin modifications and Vhs2 action will need the identification of its binding partner in vivo.
It was reported that Vhs2 has a diffuse cytoplasmatic localization;Citation20 in order to gain new information on its cellular role, we reinvestigated this issue on VHS2-6HA- and VHS2-Cherry-expressing cells, thinking to visualize Vhs2 at the bud neck, since several septin regulators localize there. Unfortunately we could not observe any specific subcellular localization (our observation). This study also describes that Vhs2 is subject to post-translational modifications during all phases of the cell cycle, and it is a phosphoprotein in vivo. In particular, Vhs2 is mostly dephosphorylated in G1, then the phosphorylated forms accumulate during S-G2-M until telophase and decrease concomitantly with cell division. The timing of Vhs2 phosphorylation together with our previous findings led us to hypothesize that phosphorylated Vhs2 likely plays a role in preserving septin structure until nuclear division is complete; later its dephosphorylation allows septin ring disassembly and recycling of the septin subunits.
We also investigated which kinases and phosphatases could drive Vhs2 modifications. Cdk1 is the master regulator of cell cycle progression, and it associates with different cyclins to regulate the phosphorylation state of several target proteins. A large-scale analysis of Cdk1 substrates identified Vhs2 as phosphorylated in vivo,Citation26 and in this study we report that inhibition of Cdk1 kinase activity indeed reduces Vhs2 phosphorylation, indicating that Vhs2 may be a physiological substrate of Cdk1. This data could reveal a new role for Cdk1 in the control of septin dynamics. Searching for a protein phosphatase that could regulate Vhs2 dephosphorylation after telophase, we considered the possible role of Rts1 and Cdc14. Rts1 is the regulatory subunit of protein phosphatase 2A (PP2A), which localizes at the bud neck after mitotic exit and plays a role in septin ring stability by dephosphorylating Shs1.Citation6 It was likely that PP2ARts1 could act also on Vhs2, but we observed that the deletion of RTS1 does not impair the appearance of Vhs2 faster migrating bands (our unpublished data), indicating that Rts1 is not required for proper Vhs2 dephosphorylation. Cdc14 protein phosphatase is a key regulator of cell cycle progression; indeed, it has multiple substrates that act in several processes: licensing of SPB duplication, spindle stabilization, nuclear segregation, and cytokinesis.Citation34 Cdc14’s timely activation ensures proper coordination between nuclear division, spindle disassembly, and cytokinesis. Here we show that Vhs2 interacts with Cdc14 in vivo, and its phosphorylated bands disappear in the presence of functional Cdc14 but persist in the presence of a phosphatase-dead Cdc14 variant. These data are consistent with the finding that Cdc14 has a preference for Cdk1-phosphorylated sites,Citation35 and that many Cdc14-interacting proteins are Cdk1 substrates,Citation36 so these proteins are likely regulated by both Cdk1 and Cdc14. We hypothesize that Cdc14 might modulate Vhs2 function in order to allow septin ring disassembly at the end of the cell cycle.
In conclusion, in this study, we have characterized the genetic and cellular role of Vhs2, a new player in the regulation of septin dynamics. In addition, our findings indicate that Vhs2 is likely a novel substrate of both Cdk1 and Cdc14, revealing a new indirect role for these enzymes in the control of septin dynamics and linking septin ring stability to cell cycle progression, thus allowing timely regulation of cytokinesis with respect to nuclear division and spindle disassembly.
Septin structures are important for the determination of cell morphology, a characteristic that changes during yeast life depending on environment conditions and during the invasive filamentous growth essential for the virulence of pathogenic yeasts. Indeed, the ability of yeast cells to switch to hyphae has been described as a way to resist and survive phagocytic action,Citation37-Citation39 and this ability is an important yeast virulence determinant. Even if no biochemical function has been associated to Vhs2 so far, its sequence is conserved in S. cerevisiae and in pathogenic yeasts; therefore, our findings could have important implications in the field of antifungal therapies.
Materials and Methods
Strains, media, reagents, and genetic manipulations
All yeast strains (Table S1) were derivatives of W303 (ade2–1, trp1–1, leu2–3,112, his3–11,15, ura3, ssd1). Unless otherwise stated, α-factor was used at 2 μg/ml or at 10 μg/ml, nocodazole was used at 15 μg/ml, 1NM-PP1 was used at 0.5 μM, and cells were grown at 25 °C in YEP (1% yeast extract, 2% bactopeptone, 50 mg/l adenine) medium supplemented with 2% glucose (YEPD) or 2% raffinose (YEPR) and 2% raffinose + 1% galactose (YEPRG).
Standard techniques were used for genetic manipulations.Citation40,Citation41 VHS2 deletion was generated by one-step gene replacement.Citation42 One-step tagging techniques were used to create 6HA-tagged Vhs2 variant and 3FLAG-tagged Cdc14-,Citation43 GAL-CDC14-, and GAL-cdc14-C283A-expressing strains were a kind gift of Elmar Schiebel. Shs1-Cherry-expressing strain and cdc28-as1 mutant were a kind gift of Gislene Pereira. Tub1-GFP-expressing strain was a kind gift of Tony Hyman. cdc3–1, cdc10–1, shs1–100c mutants were a kind gift of Masayuki Iwase. All gene replacements and tagging were controlled by PCR-based methods or Southern blot analysis.
Cloning of high-copy suppressors of GAL1-DMA2 hof1Δ
GAL1-DMA2 hof1Δ strain, which fails to grow on galactose-containing plates, was transformed with a yeast genomic DNA library constructed in the high-copy number LEU2-plasmid YEp13 (from Kim Nasmyth). Leu+ transformants were trasferred on YEPRG plates, and after 3 d at 25 °C Leu+ transformants viable on YEPRG were identified. Approximately 50 000 independent transformants were screened, which yielded 71 transformants able to grow in the presence of galactose. Plasmids were recovered, and 14 of them confirmed the ability to suppress the GAL1-DMA2 hof1Δ lethality. Plasmids inserts were sequenced and compared with the whole yeast genome sequence in order to identify the ORFs responsible for the suppression. Every plasmid contained a different chromosomic region, each carrying several ORFs, derived from chromosomes II, IV, V, VI, VII, IX, XIII, XV, XVI. One plasmid contained a region of chromosome IX (coordinates 92227–97578) that includes VHS2 and other genes; we analyzed the published information on those gene functions and on that basis VHS2 seemed to be the more plausible gene responsible for the suppression.
Fluorescence microscopy and live imaging
In situ immunofluorescence was performed on formaldehyde-fixed cells and performed as previously described.Citation44 Nuclei were visualized by staining with DAPI 0.05 μg/ml. Visualization of septin rings was performed using anti-Cdc11 polyclonal antibodies (1:200, sc-7170 Santa Cruz) followed by indirect immunofluorescence with Alexa Fluor 488-conjugated anti-rabbit antibody (1:100, Invitrogen). To detect spindle formation and elongation, α-tubulin immunostaining was performed with the YOL34 monoclonal antibody (1:100, Serotec) followed by indirect immunofluorescence using rhodamine-conjugated anti-rat Ab (1:500, Pierce Chemical Co).
For actin staining, cell were fixed adding 4% formaldehyde in the culture medium for 2 h; cells were then washed with PBS and treated with PBS containing 20 units of rhodamine phalloidine (purchased by Sigma-Aldrich) for 2 h. Digital images were taken with a Leica DC350F charge-coupled device camera mounted on a Nikon Eclipse 600 and controlled by the Leica FW4000 software or with the MetaMorph imaging system software on a fluorescent microscope (Eclipse 90i; Nikon), equipped with a charge-coupled device camera (Coolsnap, Photometrics) with an oil 100X 0.5–1.3 PlanFluor oil objective (Nikon).
For time-lapse movies, cells were grown in synthetic complete medium with 2% glucose, arrested with α-factor, then washed, collected, and imaged on agar in synthetic complete medium with 2% glucose using a Delta Vision Elite imaging system (Applied Precision) based on an IX71 inverted microscope (Olympus) with a camera CoolSNAP HQ2 from Photometrics, and a UPlanApo 60x (1.4 NA) oil immersion objective (Olympus). After the release every 3 min, 4 Z-stacks at 1.2-micron intervals were taken for each fluorescent channel; after 90 min, Z-stacks were taken every 2 min. In the Supplemental Videos, time 0 corresponds to 90 min after α-factor release. All images were then projected onto a single image per channel using Fiji software.Citation45
Protein analyses
For monitoring Vhs2 levels and post-translational modifications, total protein extracts were prepared by native protein extract as previously describedCitation46 or by TCA precipitation as previously described.Citation47 Co-immunoprecipitations were performed as previously described,Citation48 except that cells were lysed in 50 mM Na phosphate, 100 mM NaCl, 10% glycerol, 5 mM EDTA supplemented with a cocktail of protease inhibitors (Complete Mini, Roche). Phosphatase assay was performed as previously described.Citation49
For western blot analysis, proteins were transferred to Protran membranes (Schleicher and Schuell) and probed with monoclonal anti-HA (1:3000), anti-Pgk1 antibodies (1:40 000), and anti-FLAG antibodies (1:3000). Secondary antibodies were purchased from Amersham, and proteins were detected by an enhanced chemioluminescence system according to the manufacturer.
Statistical analyses and other techniques
The significance of the differences between septin disassembly and mitotic spindle breakage was statistically tested by the Wilcoxon-signed rank-sum test using MATLAB software; samples are significantly different if P value is P < 0.05.
Flow cytometric DNA quantification was performed according to Fraschini et al.Citation50 on a Becton- Dickinson FACScan.
Additional material
Download Zip (3.4 MB)Disclosure of Potential Conflicts of Interest
No potential conflicts of interest were disclosed.
Acknowledgments
We are grateful to Tony Hyman, Masayuki Iwase, Kim Nasmyth, Gislene Pereira, Simonetta Piatti, and Elmar Schiebel for strains and plasmids. We thank Giulia Rancati for critical reading of the manuscript. This work has been supported by grants from PRIN (Progetti di Ricerca di Interesse Nazionale) 2008 to R.F. C.C. was supported by a fellowship from Fondazione Confalonieri. E.R. was supported by a fellowship from Fondazione Buzzati-Traverso. E.C. was supported by supported by “Young Investigator Programme 2013” fellowship from Fondazione Veronesi.
References
- Byers B, Goetsch L. A highly ordered ring of membrane-associated filaments in budding yeast. J Cell Biol 1976; 69:717 - 21; http://dx.doi.org/10.1083/jcb.69.3.717; PMID: 773946
- Pan F, Malmberg RL, Momany M. Analysis of septins across kingdoms reveals orthology and new motifs. BMC Evol Biol 2007; 7:103; http://dx.doi.org/10.1186/1471-2148-7-103; PMID: 17601340
- De Virgilio C, DeMarini DJ, Pringle JR. SPR28, a sixth member of the septin gene family in Saccharomyces cerevisiae that is expressed specifically in sporulating cells. Microbiology 1996; 142:2897 - 905; http://dx.doi.org/10.1099/13500872-142-10-2897; PMID: 8885406
- Fares H, Goetsch L, Pringle JR. Identification of a developmentally regulated septin and involvement of the septins in spore formation in Saccharomyces cerevisiae.. J Cell Biol 1996; 132:399 - 411; http://dx.doi.org/10.1083/jcb.132.3.399; PMID: 8636217
- Oh Y, Bi E. Septin structure and function in yeast and beyond. Trends Cell Biol 2011; 21:141 - 8; http://dx.doi.org/10.1016/j.tcb.2010.11.006; PMID: 21177106
- Dobbelaere J, Gentry MS, Hallberg RL, Barral Y. Phosphorylation-dependent regulation of septin dynamics during the cell cycle. Dev Cell 2003; 4:345 - 57; http://dx.doi.org/10.1016/S1534-5807(03)00061-3; PMID: 12636916
- Park HO, Bi E. Central roles of small GTPases in the development of cell polarity in yeast and beyond. Microbiol Mol Biol Rev 2007; 71:48 - 96; http://dx.doi.org/10.1128/MMBR.00028-06; PMID: 17347519
- Egelhofer TA, Villén J, McCusker D, Gygi SP, Kellogg DR. The septins function in G1 pathways that influence the pattern of cell growth in budding yeast. PLoS One 2008; 3:e2022; http://dx.doi.org/10.1371/journal.pone.0002022; PMID: 18431499
- Gladfelter AS, Zyla TR, Lew DJ. Genetic interactions among regulators of septin organization. Eukaryot Cell 2004; 3:847 - 54; http://dx.doi.org/10.1128/EC.3.4.847-854.2004; PMID: 15302817
- Longtine MS, DeMarini DJ, Valencik ML, Al-Awar OS, Fares H, De Virgilio C, Pringle JR. The septins: roles in cytokinesis and other processes. Curr Opin Cell Biol 1996; 8:106 - 19; http://dx.doi.org/10.1016/S0955-0674(96)80054-8; PMID: 8791410
- Versele M, Thorner J. Septin collar formation in budding yeast requires GTP binding and direct phosphorylation by the PAK, Cla4. J Cell Biol 2004; 164:701 - 15; http://dx.doi.org/10.1083/jcb.200312070; PMID: 14993234
- Cid VJ, Adamiková L, Sánchez M, Molina M, Nombela C. Cell cycle control of septin ring dynamics in the budding yeast. Microbiology 2001; 147:1437 - 50; PMID: 11390675
- Meitinger F, Petrova B, Lombardi IM, Bertazzi DT, Hub B, Zentgraf H, Pereira G. Targeted localization of Inn1, Cyk3 and Chs2 by the mitotic-exit network regulates cytokinesis in budding yeast. J Cell Sci 2010; 123:1851 - 61; http://dx.doi.org/10.1242/jcs.063891; PMID: 20442249
- Iwase M, Luo J, Nagaraj S, Longtine M, Kim HB, Haarer BK, Caruso C, Tong Z, Pringle JR, Bi E. Role of a Cdc42p effector pathway in recruitment of the yeast septins to the presumptive bud site. Mol Biol Cell 2006; 17:1110 - 25; http://dx.doi.org/10.1091/mbc.E05-08-0793; PMID: 16371506
- McMurray MA, Thorner J. Septin stability and recycling during dynamic structural transitions in cell division and development. Curr Biol 2008; 18:1203 - 8; http://dx.doi.org/10.1016/j.cub.2008.07.020; PMID: 18701287
- Johnson ES, Blobel G. Cell cycle-regulated attachment of the ubiquitin-related protein SUMO to the yeast septins. J Cell Biol 1999; 147:981 - 94; http://dx.doi.org/10.1083/jcb.147.5.981; PMID: 10579719
- Johnson ES, Gupta AA. An E3-like factor that promotes SUMO conjugation to the yeast septins. Cell 2001; 106:735 - 44; http://dx.doi.org/10.1016/S0092-8674(01)00491-3; PMID: 11572779
- Huh WK, Falvo JV, Gerke LC, Carroll AS, Howson RW, Weissman JS, O’Shea EK. Global analysis of protein localization in budding yeast. Nature 2003; 425:686 - 91; http://dx.doi.org/10.1038/nature02026; PMID: 14562095
- Muñoz I, Simón E, Casals N, Clotet J, Ariño J. Identification of multicopy suppressors of cell cycle arrest at the G1-S transition in Saccharomyces cerevisiae.. Yeast 2003; 20:157 - 69; http://dx.doi.org/10.1002/yea.938; PMID: 12518319
- Gandhi M, Goode BL, Chan CS. Four novel suppressors of gic1 gic2 and their roles in cytokinesis and polarized cell growth in Saccharomyces cerevisiae. Genetics 2006; 174:665 - 78; http://dx.doi.org/10.1534/genetics.106.058180; PMID: 16816427
- Byrne KP, Wolfe KH. The Yeast Gene Order Browser: combining curated homology and syntenic context reveals gene fate in polyploid species. Genome Res 2005; 15:1456 - 61; http://dx.doi.org/10.1101/gr.3672305; PMID: 16169922
- Cassani C, Raspelli E, Santo N, Chiroli E, Lucchini G, Fraschini R. Saccharomyces cerevisiae Dma proteins participate in cytokinesis by controlling two different pathways. Cell Cycle 2013; 12:2794 - 808; http://dx.doi.org/10.4161/cc.25869; PMID: 23966170
- Costanzo M, Baryshnikova A, Bellay J, Kim Y, Spear ED, Sevier CS, Ding H, Koh JL, Toufighi K, Mostafavi S, et al. The genetic landscape of a cell. Science 2010; 327:425 - 31; http://dx.doi.org/10.1126/science.1180823; PMID: 20093466
- Gladfelter AS, Kozubowski L, Zyla TR, Lew DJ. Interplay between septin organization, cell cycle and cell shape in yeast. J Cell Sci 2005; 118:1617 - 28; http://dx.doi.org/10.1242/jcs.02286; PMID: 15784684
- Oliveira AP, Ludwig C, Picotti P, Kogadeeva M, Aebersold R, Sauer U. Regulation of yeast central metabolism by enzyme phosphorylation. Mol Syst Biol 2012; 8:623; http://dx.doi.org/10.1038/msb.2012.55; PMID: 23149688
- Holt LJ, Tuch BB, Villén J, Johnson AD, Gygi SP, Morgan DO. Global analysis of Cdk1 substrate phosphorylation sites provides insights into evolution. Science 2009; 325:1682 - 6; http://dx.doi.org/10.1126/science.1172867; PMID: 19779198
- Bishop AC, Ubersax JA, Petsch DT, Matheos DP, Gray NS, Blethrow J, Shimizu E, Tsien JZ, Schultz PG, Rose MD, et al. A chemical switch for inhibitor-sensitive alleles of any protein kinase. Nature 2000; 407:395 - 401; http://dx.doi.org/10.1038/35030148; PMID: 11014197
- Mah AS, Elia AE, Devgan G, Ptacek J, Schutkowski M, Snyder M, Yaffe MB, Deshaies RJ. Substrate specificity analysis of protein kinase complex Dbf2-Mob1 by peptide library and proteome array screening. BMC Biochem 2005; 6:22; http://dx.doi.org/10.1186/1471-2091-6-22; PMID: 16242037
- Ptacek J, Devgan G, Michaud G, Zhu H, Zhu X, Fasolo J, Guo H, Jona G, Breitkreutz A, Sopko R, et al. Global analysis of protein phosphorylation in yeast. Nature 2005; 438:679 - 84; http://dx.doi.org/10.1038/nature04187; PMID: 16319894
- Stegmeier F, Amon A. Closing mitosis: the functions of the Cdc14 phosphatase and its regulation. Annu Rev Genet 2004; 38:203 - 32; http://dx.doi.org/10.1146/annurev.genet.38.072902.093051; PMID: 15568976
- Khmelinskii A, Roostalu J, Roque H, Antony C, Schiebel E. Phosphorylation-dependent protein interactions at the spindle midzone mediate cell cycle regulation of spindle elongation. Dev Cell 2009; 17:244 - 56; http://dx.doi.org/10.1016/j.devcel.2009.06.011; PMID: 19686685
- Barral Y, Mermall V, Mooseker MS, Snyder M. Compartmentalization of the cell cortex by septins is required for maintenance of cell polarity in yeast. Mol Cell 2000; 5:841 - 51; http://dx.doi.org/10.1016/S1097-2765(00)80324-X; PMID: 10882120
- Wloka C, Nishihama R, Onishi M, Oh Y, Hanna J, Pringle JR, Krauss M, Bi E. Evidence that a septin diffusion barrier is dispensable for cytokinesis in budding yeast. Biol Chem 2011; 392:813 - 29; http://dx.doi.org/10.1515/BC.2011.083; PMID: 21824009
- Bloom J, Cristea IM, Procko AL, Lubkov V, Chait BT, Snyder M, Cross FR. Global analysis of Cdc14 phosphatase reveals diverse roles in mitotic processes. J Biol Chem 2011; 286:5434 - 45; http://dx.doi.org/10.1074/jbc.M110.205054; PMID: 21127052
- Gray CH, Good VM, Tonks NK, Barford D. The structure of the cell cycle protein Cdc14 reveals a proline-directed protein phosphatase. EMBO J 2003; 22:3524 - 35; http://dx.doi.org/10.1093/emboj/cdg348; PMID: 12853468
- Ubersax JA, Woodbury EL, Quang PN, Paraz M, Blethrow JD, Shah K, Shokat KM, Morgan DO. Targets of the cyclin-dependent kinase Cdk1. Nature 2003; 425:859 - 64; http://dx.doi.org/10.1038/nature02062; PMID: 14574415
- Clemons KV, Calich VL, Burger E, Filler SG, Grazziutti M, Murphy J, Roilides E, Campa A, Dias MR, Edwards JE Jr., et al. Pathogenesis I: interactions of host cells and fungi. Med Mycol 2000; 38:Suppl 1 99 - 111; http://dx.doi.org/10.1080/mmy.38.s1.99.111; PMID: 11204170
- Albrecht A, Felk A, Pichova I, Naglik JR, Schaller M, de Groot P, Maccallum D, Odds FC, Schäfer W, Klis F, et al. Glycosylphosphatidylinositol-anchored proteases of Candida albicans target proteins necessary for both cellular processes and host-pathogen interactions. J Biol Chem 2006; 281:688 - 94; http://dx.doi.org/10.1074/jbc.M509297200; PMID: 16269404
- Ibrahim AS, Filler SG, Sanglard D, Edwards JE Jr., Hube B. Secreted aspartyl proteinases and interactions of Candida albicans with human endothelial cells. Infect Immun 1998; 66:3003 - 5; PMID: 9596782
- Maniatis T, Fritsch EF, Sambrook J. Molecular cloning: a laboratory manual. Cold Spring Harbor Laboratory Press, Cold Spring Harbor Laboratory, NY. 1992.
- Sherman F. Getting started with yeast. Methods Enzymol 1991; 194:3 - 21; http://dx.doi.org/10.1016/0076-6879(91)94004-V; PMID: 2005794
- Wach A, Brachat A, Pöhlmann R, Philippsen P. New heterologous modules for classical or PCR-based gene disruptions in Saccharomyces cerevisiae.. Yeast 1994; 10:1793 - 808; http://dx.doi.org/10.1002/yea.320101310; PMID: 7747518
- Janke C, Magiera MM, Rathfelder N, Taxis C, Reber S, Maekawa H, Moreno-Borchart A, Doenges G, Schwob E, Schiebel E, et al. A versatile toolbox for PCR-based tagging of yeast genes: new fluorescent proteins, more markers and promoter substitution cassettes. Yeast 2004; 21:947 - 62; http://dx.doi.org/10.1002/yea.1142; PMID: 15334558
- Fraschini R, D’Ambrosio C, Venturetti M, Lucchini G, Piatti S. Disappearance of the budding yeast Bub2-Bfa1 complex from the mother-bound spindle pole contributes to mitotic exit. J Cell Biol 2006; 172:335 - 46; http://dx.doi.org/10.1083/jcb.200507162; PMID: 16449187
- Schindelin J, Arganda-Carreras I, Frise E, Kaynig V, Longair M, Pietzsch T, Preibisch S, Rueden C, Saalfeld S, Schmid B, et al. Fiji: an open-source platform for biological-image analysis. Nat Methods 2012; 9:676 - 82; http://dx.doi.org/10.1038/nmeth.2019; PMID: 22743772
- Fraschini R, Beretta A, Lucchini G, Piatti S. Role of the kinetochore protein Ndc10 in mitotic checkpoint activation in Saccharomyces cerevisiae.. Mol Genet Genomics 2001; 266:115 - 25; http://dx.doi.org/10.1007/s004380100533; PMID: 11589568
- Piatti S, Böhm T, Cocker JH, Diffley JF, Nasmyth K. Activation of S-phase-promoting CDKs in late G1 defines a “point of no return” after which Cdc6 synthesis cannot promote DNA replication in yeast. Genes Dev 1996; 10:1516 - 31; http://dx.doi.org/10.1101/gad.10.12.1516; PMID: 8666235
- Fraschini R, Beretta A, Sironi L, Musacchio A, Lucchini G, Piatti S. Bub3 interaction with Mad2, Mad3 and Cdc20 is mediated by WD40 repeats and does not require intact kinetochores. EMBO J 2001; 20:6648 - 59; http://dx.doi.org/10.1093/emboj/20.23.6648; PMID: 11726501
- Raspelli E, Cassani C, Lucchini G, Fraschini R. Budding yeast Dma1 and Dma2 participate in regulation of Swe1 levels and localization. Mol Biol Cell 2011; 22:2185 - 97; http://dx.doi.org/10.1091/mbc.E11-02-0127; PMID: 21562220
- Fraschini R, Formenti E, Lucchini G, Piatti S. Budding yeast Bub2 is localized at spindle pole bodies and activates the mitotic checkpoint via a different pathway from Mad2. J Cell Biol 1999; 145:979 - 91; http://dx.doi.org/10.1083/jcb.145.5.979; PMID: 10352016