Abstract
Type 2 diabetes is caused by a limited capacity of insulin-producing pancreatic β cells to increase their mass and function in response to insulin resistance. The signaling pathways that positively regulate functional β cell mass have not been fully elucidated. DYRK1A (also called minibrain/MNB) is a member of the dual-specificity tyrosine phosphorylation-regulated kinase (DYRK) family. A significant amount of data implicates DYRK1A in brain growth and Down syndrome, and recent data indicate that Dyrk1A haploinsufficient mice have a low functional β cell mass. Here we ask whether Dyrk1A upregulation could be a way to increase functional β cell mass.
We used mice overexpressing Dyrk1A under the control of its own regulatory sequences (mBACTgDyrk1A). These mice exhibit decreased glucose levels and hyperinsulinemia in the fasting state. Improved glucose tolerance is observed in these mice as early as 4 weeks of age. Upregulation of Dyrk1A in β cells induces expansion of β cell mass through increased proliferation and cell size. Importantly, mBACTgDyrk1A mice are protected against high-fat-diet-induced β cell failure through increase in β cell mass and insulin sensitivity.
These studies show the crucial role of the DYRK1A pathway in the regulation of β cell mass and carbohydrate metabolism in vivo. Activating the DYRK1A pathway could thus represent an innovative way to increase functional β cell mass.
Keywords: :
Introduction
Pancreatic β cells produce and secrete insulin, which reduces blood glucose by stimulating glucose uptake in skeletal muscle and adipose tissue and by suppressing hepatic neoglucogenesis. Plasma insulin levels are determined largely by β cell mass and β cell secretory function. Type 2 diabetes is characterized by insulin resistance with a failure of adaptive β cell expansion and insulin secretion. This gives rise to glucose intolerance and hyperglycemia. Proliferation, differentiation, and apoptosis are fundamental cellular processes that regulate β cell mass and are tightly regulated and coordinated to produce the correct number of each mature islet cell type. Alterations in these processes generate failures in the adaptive regulation of β cell growth and can cause type 2 diabetes.Citation1 The mechanisms underlying regulation of β cell mass are not yet fully understood, and their dissection is critical to identifying new treatments for type 2 diabetes mellitus.
The dual-specificity tyrosine phosphorylation-regulated kinase DYRK1A and its Drosophila ortholog Minibrain (Mnb), expressed in the neural tissues, are highly conserved through evolution. The Dyrk1a gene is localized in the critical region on chromosome 21 (HSA21) and has been implicated in Down syndrome (DS). Mutations of Dyrk1a in mammals give rise to defects in neuroblast proliferation and brain growth development.Citation2,Citation3 Human patients with truncated mutations of the Dyrk1a gene also exhibit microcephaly and intrauterine growth retardation.Citation4 Pancreatic β cells and neuronal cells share many similarities in terms of gene expression and development.Citation5-Citation8 Based on the impact of DYRK1A on organ growth as brain, and the importance of β cell mass in diabetes, we thought it relevant to test the effect of DYRK1A on β cells.
While little information is available on the role of DYRK1A in β cells, we recently demonstrated that in mice, Dyrk1a haploinsufficiency leads to a decrease in β cell number and size.Citation9 This reduction of β cell mass in Dyrk1a +/− mice gives rise to impaired glucose tolerance associated with hypoinsulinaemia. However, nothing is known about the consequences of increased Dyrk1A expression in islet β cells. The hypothesis to be tested here is that upregulation of Dyrk1A results in an increase in β cell mass through enhanced proliferation. We evaluate β cell function in mice overexpressing Dyrk1A under the control of its own regulatory sequences (mBACTgDyrk1A). Our data indicate that upregulation of Dyrk1A improves glucose tolerance associated with an increase in blood insulin levels. Importantly, mBACTgDyrk1A mice exhibit a higher β cell mass, with greater β cell proliferation and size. Moreover, mBACTgDyrk1A mice are resistant to diabetes induced by a high-fat diet (HFD).
We thus show the potential of DYRK1A in the upregulation of β cell proliferation and size and propose that the Dyrk1A gene represents an exciting prospect for type 2 diabetes treatment.
Results
mBACTgDyrk1A mice are glucose tolerant and hyperinsulinemic
We first examined whether upregulation of DYRK1A resulted in metabolic alterations. There were no significant differences in body weight during the 24 wk of observation (). Interestingly, from week 28, body weight continued to increase in wild-type mice, while it reached a plateau in mBACTgDyrk1A mice. This difference in weight growth rate was not linked to any variation in food consumption between the 2 genotypes (), but associated with a decreased white fat mass observed in aged mBACTgDyrk1A mice (Fig. S1). Glycemia measurements indicate lower fasting blood glucose levels in mBACTgDyrk1A mice compared with wild-type mice (). Lower glycaemia was observed in 4-wk-old mBACTgDyrk1A mice and remained lower at all time points tested up to 48 wk. This was associated with increased glucose tolerance, observed in intraperitoneal glucose tolerance tests (). In parallel, mBACTgDyrk1A mice had higher plasma insulin levels than wild-type mice at all time points tested between weeks 4 and 48 (). Insulin secretory response following i.p. injection of glucose was then assessed. Glucose-stimulated insulin secretion was greater in mBACTgDyrk1A mice than wild-type mice (). In contrast, peripheral glucose assimilation, or clearance, assessed by the insulin tolerance test, was unchanged compared with controls (). Finally, after pyruvate administration, the blood glucose levels were similar in both groups of mice, indicating no defect in the liver in term of gluconeogenesis (). Since Dyrk1a upregulation resulted in improved glucose tolerance and increased insulin levels, while insulin sensitivity was unchanged, we next focused on the effect of DYRK1A on pancreatic β cells.
Figure 1.mBACTgDyrk1A mice exhibit glucose tolerance and improved insulin secretion. (A) Body weight change in wild-type and mBACTgDyrk1A mice. (B) Absence of effect of mBACTgDyrk1A on food intake. (C) Blood glucose concentrations in overnight-fasted wild-type and mBACTgDyrk1A mice. (D) i.p. glucose tolerance tests on 12-wk-old mice. (E) Serum insulin concentrations in 6-h-fasted mice at the indicated age. (F) In vivo insulin secretion in 16-wk-old mBACTgDyrk1A and wild-type mice. (G) Insulin tolerance test in 24-wk-old mice. (H) Pyruvate tolerance test in 24-wk-old mice. Data are shown as the mean ± SEM of at least 3 independent experiments. *P < 0.05; **P < 0.01; ***P < 0.005.
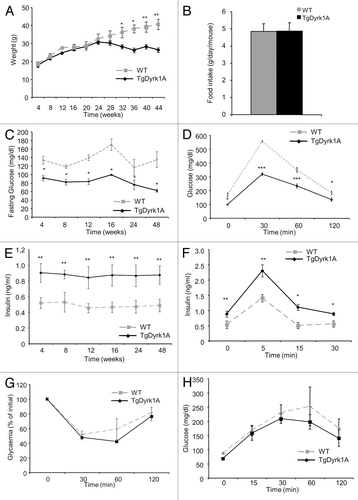
Insulin secretion and gene expression in islets from mBACTgDyrk1A mice
The expression pattern of DYRK1A in mouse pancreas has recently been described.Citation9 In our study, when compared with wild-type mice, mBACTgDyrk1A mice show increased Dyrk1A expression levels in pancreatic samples at all tested experimental time points (). This increased level of expression was observed also in other tissues expressing Dyrk1A (). At the protein level, DYRK1A expression was increased in pancreas of mBACTgDyrk1A mice compared with wild-type mice ().
Figure 2. Pancreatic expression of Dyrk1a. (A) Quantitative PCR analysis of Dyrk1a mRNA expression in pancreases from wild-type and mBACTgDyrk1A mice at different stages of fetal development and in adult islets. Data are presented as fold enrichment over E15 embryonic pancreas. (B) Quantitative PCR analysis of Dyrk1a mRNA expression in pancreases from wild-type and mBACTgDyrk1A mice in different tissues and in adult islets. Data are presented as fold enrichment over wild-type. (C) Immunoblot for DYRK1A and β actin in adult islet extracts. Quantification is presented in (D). Data are shown as the mean ± SEM of at least 3 independent experiments. **P < 0.01.
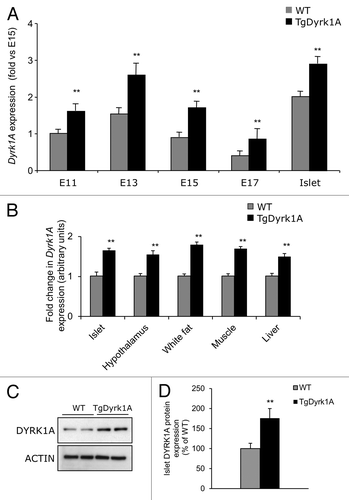
Immunofluorescence staining for insulin and glucagon indicates that the islet architecture is conserved in mBACTgDyrk1A mice, with insulin-positive cells in the core of the islet and non-β cells in the periphery (). The glucagon/insulin ratio is also conserved in mBACTgDyrk1A mice (). We next compared insulin secretion in isolated islets from mBACTgDyrk1A and wild-type mice. When expressed as percentage of insulin content, insulin secretion levels at un-stimulating (2.8 mM) and stimulating (20 mM) glucose levels are similar for mutant and control islets, demonstrating the functionality of islets from mBACTgDyrk1A mice (). Significantly, the total insulin content of islets from mBACTgDyrk1A mice is higher than that of islets from wild-type mice (; Fig. S2A). Thus, quantitatively, when reported per μg of islet protein () or per μg of islet DNA (Fig. S2B), islets from mBACTgDyrk1A mice secrete more insulin than islets from wild-type mice. Therefore, islets from mBACTgDyrk1A mice are functional, and they contain and secrete more insulin than islets from wild-type mice. Comparative gene expression analysis between islets from wild-type and mBACTgDyrk1A mice confirm the upregulation of Dyrk1a in mutant islets (). Insulin, glucagon, and somatostatin expression is increased in islets from mBACTgDyrk1A mice (). In parallel, Mafa and Neurod1 expression, which encode 2 insulin gene transactivators, is higher, while the expression of Pdx1, another insulin transactivator, is not modulated by Dyrk1a upregulation (). Finally, the expression of Glut2 (also known as Slc2a2) and Znt8 (also known as Slc30a8), which are both involved in insulin secretion, is unchanged ().
Figure 3. Insulin secretion in mBACTgDyrk1A mouse islets. (A) Immunofluorescence staining for insulin (green) and glucagon (red) in pancreatic sections from 12-wk-old wild-type and mBACTgDyrk1A mice. Scale bar: 25 μm. (B) Immunohistochemical quantification of the insulin- and glucagon-stained areas showed a similar glucagon/insulin ratio in 12-wk-old mBACTgDyrk1A mice compared with wild-type mice. Data are shown as mean ± SEM of 3 independent experiments. (C–E) Glucose-induced insulin secretion and insulin content in isolated islets from 16-wk-old mBACTgDyrk1A and wild-type mice. Insulin release in (C) is presented as % of insulin content per h, while in (E) it is presented as ng per μg of islet protein per hour. (F and G) Real-time PCR quantifications of Dyrk1a, insulin, glucagon and SST, and Mafa, Neurod1, Pdx1, Znt8, and Glut2 mRNA mRNA were performed on isolated islets from 16-wk-old wild-type and mBACTgDyrk1A mice. Data are shown as mean ± SEM of at least 3 independent experiments. **P < 0.01
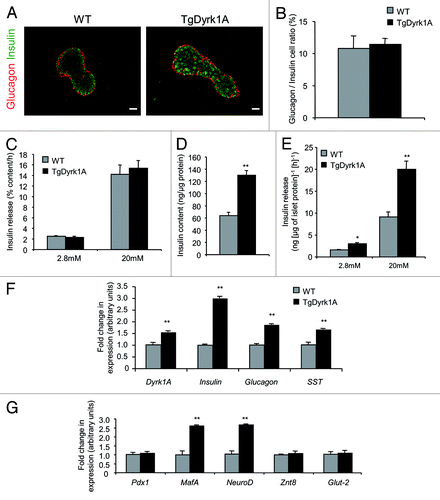
Increased β cell mass, proliferation and size in mBACTgDyrk1A mice
In 16-wk-old adult mice, pancreatic weight () and β cell area, measured following insulin immunostaining (), are both higher in mBACTgDyrk1A mice, showing a 2.16-fold increase in absolute β cell mass when compared wild-type pancreases (). Beta cell size measurement demonstrated a 34% increase in mBACTgDyrk1A mice (). The β cell proliferation rate, measured as the percentage of insulin-positive cells that stained positive for Ki67, is also more than 2-fold higher in mBACTgDyrk1A mice (). Comparative gene expression analysis of genes involved in proliferation indicate that the expression of Ccnd1, Ccnd2, and Ki67, 3 cell cycle-related genes, is also higher in islets from mBACTgDyrk1A mice ().
Figure 4. Islet morphometry in wild-type and mBACTgDyrk1A mice. (A) Increased pancreatic weight in mBACTgDyrk1A mice relative to wild-type mice. (B). Quantification of the insulin-stained area in mBACTgDyrk1A compared with wild-type mice. (C) Immunodetection of insulin (white dots) in pancreatic sections of 12-wk-old mice wild-type and mBACTgDyrk1A mice Scale bar: 5 mm. (D) Absolute β cell mass in mBACTgDyrk1A compared with wild-type mice. Data are shown as mean ± SEM of at least 3 independent experiments. *P < 0.05; **P < 0.01
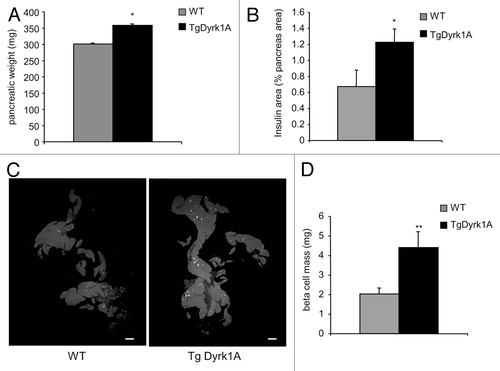
Figure 5. Beta cell proliferation and size in wild-type and mBACTgDyrk1A mice. (A) Quantification of β cell size was performed by measuring at least 500 cells. (B) The proliferative index in sections stained for Ki67 and insulin was established by measurements of at least 2000 β cells. (C) Ki67 (red) and insulin (green) staining on pancreases from 12-wk-old mBACTgDyrk1A and wild-type mice. Nuclei were stained with Hoechst 33342 fluorescent stain (blue). Scale bar: 12.5 μm (D) Real-time PCR quantification of Dyrk1a, Ccnd1, Ccnd2, and Ki67 mRNA was performed on isolated islets from wild-type and mBACTgDyrk1A mice. Data are shown as mean ± SEM from at least 3 pancreases per condition. *P < 0.05, **P < 0.01
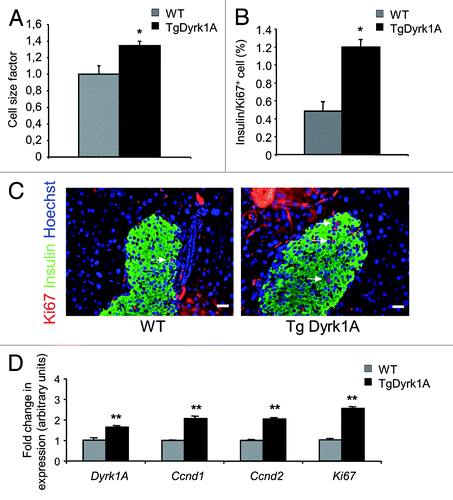
Interestingly, quantification of insulin staining performed at E17, shows that β cell mass is already higher in mBACTgDyrk1A than wild-type mice during prenatal development (). At this prenatal stage, β cell proliferation, measured following Ki67 immunostaining, is also higher in mBACTgDyrk1A mice compared with wild-type mice ().
Figure 6. Islet morphometry and proliferation in E17 mBACTgDyrk1A mice. (A) Immunodetection of insulin (white dots) in pancreatic sections of E17 wild-type and mBACTgDyrk1A mice. (B) Quantification of the insulin-stained area in mBACTgDyrk1A compared with wild-type mice. Scale bar: 50 µm. (C) The proliferative index was established following Ki67 and Insulin staining by measurement of at least 500 β cells. Data are mean ± SEM from at least 3 pancreases per condition. **P < 0.01.
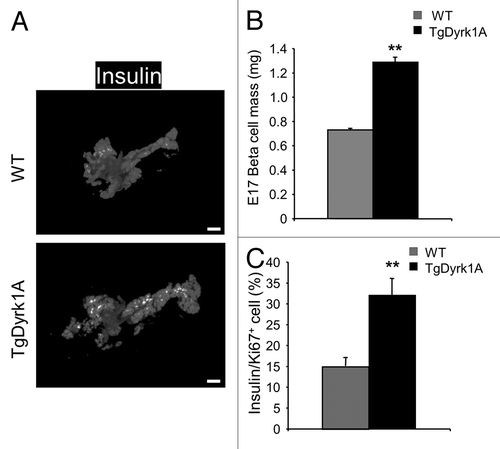
We next asked whether this phenotype was pancreas autonomous. For this purpose, we isolated and cultured islets for 7 d before a pulse of BrdU. Brdu staining shows that in vitro, β cell proliferation is higher in mBACTgDyrk1A mice compared with wild-type mice (Fig. S3A and B), as is the case in vivo.
mBACTgDyrk1A mice are resistant to high-fat diet-induced diabetes
In mice, an HFD results in insulin resistance, hyperinsulinemia, and increased β cell mass. However, β cell hyperplasia is not sufficient to prevent glucose intolerance. As mBACTgDyrk1A mice have an increased β cell mass associated with increased insulin secretion, we tested whether mBACTgDyrk1A would combat high-fat induced diabetes. Four-week-old mBACTgDyrk1A and wild-type mice were fed either 60% fat or a normal diet for 12 wk. On the HFD, wild-type mice gain weight, while mBACTgDyrk1A show a clear resistance to weight gain (). After 12 wk of HFD, wild-type mice develop glucose intolerance, which is not the case in mBACTgDyrk1A mice, which show a curve similar to control mice under a normal diet (). Of note, while in wild-type mice an HFD induces insulin resistance, this is not the case in mBACTgDyrk1A mice (). Consistent with their overall improved metabolic phenotype under HFD, mBACTgDyrk1A mice have increased β cell mass relative to wild-type mice (). Taken together these results indicate that mBACTgDyrk1A mice resist against HFD-induced diabetes.
Figure 7.mBACTgDyrk1A mice are resistant to high-fat diet-induced diabetes. (A) Body weight evolution in wild-type and mBACTgDyrk1A mice under HFD. (B) i.p. glucose tolerance tests after 12-wk of HFD. (C) Insulin tolerance test after 12 wk of HFD. (D) Immunodetection of insulin (brown) in pancreatic sections from wild-type and mBACTgDyrk1A mice after 12 wk of HFD. Hemalun staining (blue) was used to counterstain the tissue. Scale bar: 5 mm. (D) Immunohistochemical quantification of the insulin-stained area showed that the β cell mass was increased in mBACTgDyrk1A compared with wild-type mice under HFD. Data are shown as the mean ± SEM of at least 3 independent experiments. *P < 0.05; **P < 0.01; ***P < 0.005.
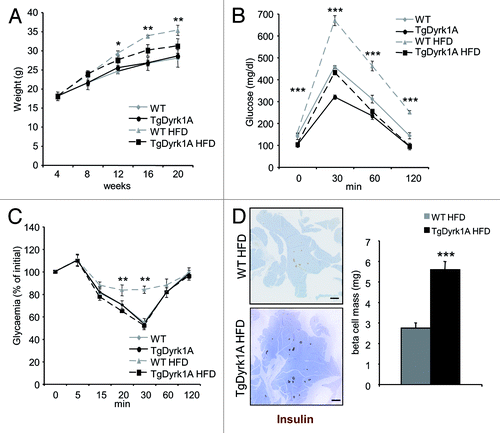
Discussion
In the present study, we have demonstrated that mice which overexpress the dual-specificity tyrosine phosphorylation-regulated kinase (Dyrk1a) develop increased β cell mass. Importantly, we also found that, in this model, the β cells are functional and protect against high-fat diet-induced diabetes.
It is well established that functional β cell mass is impaired in patients with type 2 diabetes, with β cell mass decreasedCitation10 and insulin secretion reduced.Citation11 With this is mind, a great deal of effort is being put into discovering new signaling pathways that increase β cell mass and function.Citation12 We have previously demonstrated that Dyrk1a is expressed in the pancreas and enriched in islets,Citation9 and also that Dyrk1a haploinsufficient mice have a lower functional β cell mass with decreased insulinemia and increased glycemia.Citation9 As a result, we reason that increasing Dyrk1a levels could represent an innovative way to increase functional β cell mass and protect against high-fat diet-induced diabetes.
In the present study, we used a mouse model with an additional copy of Dyrk1a under the control of its own regulatory sequences. This recently described model has been used to study brain development and show the major roles of Dyrk1A in shaping the brain, controlling neuron cell cycles, neuronal machinery, and synaptic functions.Citation13,Citation14 The model has also been used to recapitulate the retinal defect associated with Down syndrome.Citation15
To the best of our knowledge, β cell mass regulation and glucose tolerance has not previously been analyzed in mice overexpressing Dyrk1a. Recently Hong et al. developed and analyzed a model of transgenic mice that overexpress human DYRK1A.Citation16 Glucose metabolism was not described in these mice, but they exhibit increased food intake, which suggests that they would become obese over time.Citation16 In contrast, in the model used in the present study, the transgenic mice did not exhibit a perturbation in food intake and were not obese up to 44 wk of postnatal life, the latest time point tested. The data obtained from these models could appear contradictory. However, the 2 models are different on a number of critical points: (1) a human gene is used in the Hong transgenic Dyrk1a mouse model, while we used mice overexpressing the mouse form of Dyrk1A; (2) the 3′UTR is missing in the Hong transgenic mouse, which could probably lead to a different pattern of expression; and (3) the work by Hong described mice at early time points, which differs from our long-term in vivo approach.Citation16
There are a number of examples where over- and under-expression models do not give rise to mirror phenotypes. This is, for example, the case for β cell-specific transcription factors such as PDX1, where haploinsufficiency or β cell-specific deletion of Pdx1 results in hyperglycemia and decreased β cell mass,Citation17,Citation18 while forced expression of Pdx1 in the pancreatic endocrine pathway does not give rise to major changes in β cell mass.Citation19 Signaling molecules give another example. Reduced Akt activity in β cells induces impaired glucose tolerance due to defective insulin secretion without any β cell mass defect,Citation20 while transgenic mice that express a constitutively active Akt in β cells exhibit greatly increased β cell mass.Citation21 Calcineurin expression in β cells is another example. Calcineurin deficiency and upregulation in mice both lead to decreased β-cell mass.Citation22,Citation23 In the present case, we found a direct relationship between Dyrk1a levels and functional β cell mass. Specifically, while mice haploinsufficient for Dyrk1A are hyperglycemic and hypoinsulinic with a reduced β cell mass,Citation9 we demonstrate here that mice that overexpress Dyrk1a have lower glycaemia and increased insulinemia compared with control mice.
Dyrk1A regulates organ size through different mechanisms. For example, Dyrk1A regulates retinal growth through decreasing apoptotic levelsCitation15 and increases brain size by improving cell proliferation by upregulation of Akt signaling and cyclinD1 expression.Citation13 In the present study, we found that β cell apoptosis is not modified in mBACTgDyrk1A mice (data not shown), while β cell proliferation is increased in mice that overexpress Dyrk1a, with an upregulation of cyclinD1 in islets of mBACTgDyrk1A mice, which has also previously been shown for the brain of such miceCitation13. We also found that β cell size increases in these mice. This could suggest a link between the Dyrk1A and the mTOR pathway as recently posited.Citation24 Whether Dyrk1A modulates the mTOR pathway in pancreatic β cells would be a fruitful avenue for further study.
Our observations on the effects of the DYRK1A in β cell reinforce the potential role of DYRK1A as a new essential regulator of β cell proliferation. To the best of our knowledge, the activators of the Dyrk1a signaling pathway have not yet been described. When they have, it will be of major interest to determine whether they can be used to increase functional β cell mass and protect against high-fat diet-induced diabetes. Activating the DYRK1A pathway might offer a novel approach to increasing β cell mass and treating diabetes.
Materials and Methods
Animals
The generation of mBACTgDyrk1A mice has previously been described.Citation13 Wild-type and Dyrk1a mutant mice were used in accordance with French Animal Care Committee guidelines. The mice were bred on a genetic C57Bl6J background and raised on a 12-h light–dark cycle. They were fed with a standard laboratory chow diet. The high-fat diet consisted of a synthetic high-fat diet with 60% kcal% fat (HFD) (D12492; Research Diets). mBACTgDyrk1A mice under HFD were fed for 12 wk from the 4th week. The first day post-coitum was taken as embryonic day 0.5 (E0.5).
Metabolic studies
Metabolic studies were performed on male mice. At least 8 mice were analyzed per group. Daily food intake was measured in 16-wk-old mice. Blood samples were collected from the tail vein. Blood glucose levels were measured using the OneTouch Vita blood glucose meter (LifeScan). Plasma insulin levels were determined by ELISA kit (ALPCO). Glucose tolerance tests were performed on mice fasted for 16 h by glucose injection (2 g/kg, i.p.) as previously described.Citation25 Insulin tolerance tests were performed on mice that had been fasted for 6 h, and glucose measurements were taken at 30, 60, and 120 min after i.p. injection of insulin (0.75 U/kg). Pyruvate tolerance tests were done on mice that had been fasted for 16 h, and then glucose measurements were taken at 30, 60, and 120 min after i.p. injection of sodium pyruvate (1.5 g/kg; Sigma-Aldrich).
Islet studies
Islets were isolated by collagenase digestion followed by purification through a Histopaque gradient (Sigma-Aldrich). Insulin secretion was assessed by static incubation of isolated islets in Millicell inserts (Millipore). Briefly, following overnight culture in RPMI medium containing 5.6 mmol/l glucose, the islets were pre-incubated for 1 h in Krebs–Ringer medium containing 2.8 mmol/l glucose. Groups of 50 islets in triplicate were then incubated in Krebs–Ringer medium containing 2.8 or 20 mmol/l glucose for 1 h. Secreted insulin and the insulin contents were measured using an ultrasensitive mouse insulin ELISA (ALPCO).Citation9 DNA content was measured using Quant-iT™ PicoGreen® dsDNA Kit from Invitrogen. For cell proliferation analyses, islets were cultured 7 d and pulsed with 10 mM of bromodeoxyuridine (BrdU) for 1 h before fixation.
Western blotting
Protein lysates from pancreases at different stage of life were subjected to immunoblotting as described.Citation9 The following antibodies were used: Dyrk1A (Abnova, 1:1000) and Actin (Sigma, 1:2000). Immunoblotting experiments were performed 3 times. Quantification of the western blots by densitometry was done using NIH ImageJ software and normalized against that of actin.
Immunohistochemistry and quantification
Pancreases were immersed in 10% formalin and embedded in paraffin. Sections (4-μm-thick) were processed for immunohistochemistry as previously described.Citation26 Antibodies were used at the following dilutions: mouse anti-insulin (1:2000; Sigma); rabbit anti-insulin (1:2000; Dako); mouse anti-glucagon (1:2000; Sigma); mouse anti-Ki67 (1:20; BD Biosciences); and mouse anti-BrdU (1:4; Amersham). The fluorescent secondary antibodies were from Jackson ImmunoResearch. The nuclei were stained using the Hoechst 33342 fluorescent stain (0.3 mg/ml; Invitrogen).
Sections were digitized using cooled 3-CCD cameras (C5810 or C7780; Hamamatsu) attached to a fluorescence microscope (Leitz DMRB; Leica). Quantification of insulin staining was performed on 5 equally separated sections for adult pancreases and on every second slide for fetal pancreases. In each section, the β cell area and the pancreatic area were determined using NIH ImageJ software (v1.31; freely available at: http://rsb.info.nih.gov/ij/index.html). For adult pancreases, the percentage of the β cell area in each pancreatic section was determined by dividing the total area of insulin-positive cells by the surface area of the section and the β cell mass was calculated by multiplying the pancreas weight by the % area of β cells. For fetal pancreases, immunostained areas were quantified using NIH ImageJ software on every image, and then summed to obtain the total area per explant in mm2, as previously described.Citation27
Beta cell size was calculated by dividing the β cell area by the number of β cell nuclei using NIH ImageJ software, as previously described.Citation25
RNA extraction and real-time PCR
Total RNA was extracted using an RNeasy Microkit (Qiagen), and then reverse transcribed using Superscript reagents (Invitrogen). Real-time PCR was performed with the 7300 Fast Real-Time PCR System (Applied Biosystems). The oligonucleotide sequences are available upon request. Cyclophilin A was used as the internal reference control.
Statistical analysis
Quantitative data are presented as the mean ± SEM from at least 3 independent experiments, unless indicated. Interaction between the variables was investigated by 2-way analysis of variance, and an unpaired Student t test was used to compare the independent means. Statistical significance was set at P < 0.05.
Additional material
Download Zip (664.7 KB)Disclosure of Potential Conflicts of Interest
No potential conflicts of interest were disclosed.
Acknowledgments
The authors thank Colin Mackenzie (Mackenzie Translation) for his comments and editorial assistance in preparing this manuscript. The authors thank Sophie Berissi and the Necker Histology Facility for technical assistance.
Author Contribution
L.R. and R.S. designed the research and wrote the manuscript. L.R., D.K., and V.A. performed research and acquired the data. All authors made substantial contributions to the analysis and interpretation of data. All authors were involved in drafting the manuscript and all approved the final version.
Funding
This work was supported by grants from INSERM “Junior 5-year Contract” and “la Société Francophone du Diabète” (SFD) (L.R.).The research leading to these results received support from the Innovative Medicines Initiative Joint Undertaking under grant agreement n° 115439, financial contribution from the European Union's Seventh Framework Programme (FP7/2007–2013) and contribution from EFPIA companies. The RS laboratory belongs to the Laboratoire d’Excellence consortium Revive.
References
- Ferrannini E. The stunned beta cell: a brief history. Cell Metab 2010; 11:349 - 52; http://dx.doi.org/10.1016/j.cmet.2010.04.009; PMID: 20444416
- Fotaki V, Dierssen M, Alcántara S, Martínez S, Martí E, Casas C, Visa J, Soriano E, Estivill X, Arbonés ML. Dyrk1A haploinsufficiency affects viability and causes developmental delay and abnormal brain morphology in mice. Mol Cell Biol 2002; 22:6636 - 47; http://dx.doi.org/10.1128/MCB.22.18.6636-6647.2002; PMID: 12192061
- Tejedor FJ, Hämmerle B. MNB/DYRK1A as a multiple regulator of neuronal development. FEBS J 2011; 278:223 - 35; http://dx.doi.org/10.1111/j.1742-4658.2010.07954.x; PMID: 21156027
- Oegema R, de Klein A, Verkerk AJ, Schot R, Dumee B, Douben H, Eussen B, Dubbel L, Poddighe PJ, van der Laar I, et al. Distinctive Phenotypic Abnormalities Associated with Submicroscopic 21q22 Deletion Including DYRK1A. Mol Syndromol 2010; 1:113 - 20; PMID: 21031080
- Le Douarin NM. On the origin of pancreatic endocrine cells. Cell 1988; 53:169 - 71; http://dx.doi.org/10.1016/0092-8674(88)90375-3; PMID: 3282672
- Atouf F, Czernichow P, Scharfmann R. Expression of neuronal traits in pancreatic beta cells. Implication of neuron-restrictive silencing factor/repressor element silencing transcription factor, a neuron-restrictive silencer. J Biol Chem 1997; 272:1929 - 34; http://dx.doi.org/10.1074/jbc.272.3.1929; PMID: 8999882
- Rorsman P. The pancreatic beta-cell as a fuel sensor: an electrophysiologist’s viewpoint. Diabetologia 1997; 40:487 - 95; http://dx.doi.org/10.1007/s001250050706; PMID: 9165215
- van Arensbergen J, García-Hurtado J, Moran I, Maestro MA, Xu X, Van de Casteele M, Skoudy AL, Palassini M, Heimberg H, Ferrer J. Derepression of Polycomb targets during pancreatic organogenesis allows insulin-producing beta-cells to adopt a neural gene activity program. Genome Res 2010; 20:722 - 32; http://dx.doi.org/10.1101/gr.101709.109; PMID: 20395405
- Rachdi L, Kariyawasam D, Guez F, Aïello V, Arbonés ML, Janel N, Delabar J-M, Polak M, Scharfmann R. Dyrk1a haploinsufficiency induces diabetes in mice through decreased pancreatic beta cell mass. Diabetologia 2014; 57:960 - 9; http://dx.doi.org/10.1007/s00125-014-3174-3; PMID: 24477974
- Rahier J, Guiot Y, Goebbels RM, Sempoux C, Henquin JC. Pancreatic beta-cell mass in European subjects with type 2 diabetes. Diabetes Obes Metab 2008; 10:Suppl 4 32 - 42; http://dx.doi.org/10.1111/j.1463-1326.2008.00969.x; PMID: 18834431
- Ashcroft FM, Rorsman P. Diabetes mellitus and the β cell: the last ten years. Cell 2012; 148:1160 - 71; http://dx.doi.org/10.1016/j.cell.2012.02.010; PMID: 22424227
- Drucker DJ. Incretin action in the pancreas: potential promise, possible perils, and pathological pitfalls. Diabetes 2013; 62:3316 - 23; http://dx.doi.org/10.2337/db13-0822; PMID: 23818527
- Guedj F, Pereira PL, Najas S, Barallobre M-J, Chabert C, Souchet B, Sebrie C, Verney C, Herault Y, Arbones M, et al. DYRK1A: a master regulatory protein controlling brain growth. Neurobiol Dis 2012; 46:190 - 203; http://dx.doi.org/10.1016/j.nbd.2012.01.007; PMID: 22293606
- Thomazeau A, Lassalle O, Iafrati J, Souchet B, Guedj F, Janel N, Chavis P, Delabar J, Manzoni OJ. Prefrontal deficits in a murine model overexpressing the down syndrome candidate gene dyrk1a. J Neurosci 2014; 34:1138 - 47; http://dx.doi.org/10.1523/JNEUROSCI.2852-13.2014; PMID: 24453307
- Laguna A, Barallobre M-J, Marchena M-Á, Mateus C, Ramírez E, Martínez-Cue C, Delabar JM, Castelo-Branco M, de la Villa P, Arbonés ML. Triplication of DYRK1A causes retinal structural and functional alterations in Down syndrome. Hum Mol Genet 2013; 22:2775 - 84; http://dx.doi.org/10.1093/hmg/ddt125; PMID: 23512985
- Hong S-H, Lee K-S, Kwak S-J, Kim A-K, Bai H, Jung M-S, Kwon O-Y, Song W-J, Tatar M, Yu K. Minibrain/Dyrk1a regulates food intake through the Sir2-FOXO-sNPF/NPY pathway in Drosophila and mammals. PLoS Genet 2012; 8:e1002857; http://dx.doi.org/10.1371/journal.pgen.1002857; PMID: 22876196
- Johnson JD, Ahmed NT, Luciani DS, Han Z, Tran H, Fujita J, Misler S, Edlund H, Polonsky KS. Increased islet apoptosis in Pdx1+/- mice. J Clin Invest 2003; 111:1147 - 60; http://dx.doi.org/10.1172/JCI200316537; PMID: 12697734
- Gao T, McKenna B, Li C, Reichert M, Nguyen J, Singh T, Yang C, Pannikar A, Doliba N, Zhang T, et al. Pdx1 maintains β cell identity and function by repressing an α cell program. Cell Metab 2014; 19:259 - 71; http://dx.doi.org/10.1016/j.cmet.2013.12.002; PMID: 24506867
- Yang Y-P, Thorel F, Boyer DF, Herrera PL, Wright CVE. Context-specific α- to-β-cell reprogramming by forced Pdx1 expression. Genes Dev 2011; 25:1680 - 5; http://dx.doi.org/10.1101/gad.16875711; PMID: 21852533
- Bernal-Mizrachi E, Fatrai S, Johnson JD, Ohsugi M, Otani K, Han Z, Polonsky KS, Permutt MA. Defective insulin secretion and increased susceptibility to experimental diabetes are induced by reduced Akt activity in pancreatic islet beta cells. J Clin Invest 2004; 114:928 - 36; http://dx.doi.org/10.1172/JCI200420016; PMID: 15467831
- Bernal-Mizrachi E, Wen W, Stahlhut S, Welling CM, Permutt MA. Islet beta cell expression of constitutively active Akt1/PKB alpha induces striking hypertrophy, hyperplasia, and hyperinsulinemia. J Clin Invest 2001; 108:1631 - 8; http://dx.doi.org/10.1172/JCI200113785; PMID: 11733558
- Bernal-Mizrachi E, Cras-Méneur C, Ye BR, Johnson JD, Permutt MA. Transgenic overexpression of active calcineurin in beta-cells results in decreased beta-cell mass and hyperglycemia. PLoS One 2010; 5:e11969; http://dx.doi.org/10.1371/journal.pone.0011969; PMID: 20689817
- Heit JJ, Apelqvist AA, Gu X, Winslow MM, Neilson JR, Crabtree GR, Kim SK. Calcineurin/NFAT signalling regulates pancreatic beta-cell growth and function. Nature 2006; 443:345 - 9; http://dx.doi.org/10.1038/nature05097; PMID: 16988714
- Wippich F, Bodenmiller B, Trajkovska MG, Wanka S, Aebersold R, Pelkmans L. Dual specificity kinase DYRK3 couples stress granule condensation/dissolution to mTORC1 signaling. Cell 2013; 152:791 - 805; http://dx.doi.org/10.1016/j.cell.2013.01.033; PMID: 23415227
- Rachdi L, Balcazar N, Osorio-Duque F, Elghazi L, Weiss A, Gould A, Chang-Chen KJ, Gambello MJ, Bernal-Mizrachi E. Disruption of Tsc2 in pancreatic beta cells induces beta cell mass expansion and improved glucose tolerance in a TORC1-dependent manner. Proc Natl Acad Sci U S A 2008; 105:9250 - 5; http://dx.doi.org/10.1073/pnas.0803047105; PMID: 18587048
- Rachdi L, Marie J-C, Scharfmann R. Role for VPAC2 receptor-mediated signals in pancreas development. Diabetes 2003; 52:85 - 92; http://dx.doi.org/10.2337/diabetes.52.1.85; PMID: 12502497
- Rachdi L, Aïello V, Duvillié B, Scharfmann R. L-leucine alters pancreatic β-cell differentiation and function via the mTor signaling pathway. Diabetes 2012; 61:409 - 17; http://dx.doi.org/10.2337/db11-0765; PMID: 22210321