Abstract
High levels of transforming growth factor-β (TGFβ) correlate with poor prognosis for patients with prostate cancer and other cancers. TGFβ is a multifunctional cytokine and crucial regulator of cell fate, such as epithelial to mesenchymal transition (EMT), which is implicated in cancer invasion and progression. TGFβ conveys its signals upon binding to type I and type II serine/threonine kinase receptors (TβRI/II); phosphorylation of Smad2 and Smad3 promotes their association with Smad4, which regulates expression of targets genes, such as Smad7, p21, and c-Jun. TGFβ also activates the ubiquitin ligase tumor necrosis factor receptor-associated factor 6 (TRAF6), which associates with TβRI and activates the p38 mitogen-activated protein kinase (MAPK) pathway. Snail1 is a key transcription factor, induced by TGFβ that promotes migration and invasion of cancer cells. In this study, we have identified a novel binding site for c-Jun in the promoter of the Snail1 gene and report that the activation of the TGFβ–TRAF6–p38 MAPK pathway promotes both c-Jun expression and its activation via p38α-dependent phosphorylation of c-Jun at Ser63. The TRAF6-dependent activation of p38 also leads to increased stability of c-Jun, due to p38-dependent inactivation of glycogen synthase kinase (GSK) 3β by phosphorylation at Ser9. Thus, our findings elucidate a novel role for the p38 MAPK pathway in stimulated cells, leading to activation of c-Jun and its binding to the promoter of Snail1, thereby triggering motility and invasiveness of aggressive human prostate cancer cells.
Introduction
Transforming growth factor-β (TGFβ) is a ubiquitous cytokine with pleiotrophic cellular effects, including growth arrest, differentiation, angiogenesis, immunosuppression and apoptosis.Citation1,Citation2 TGFβ exerts its cellular effects by binding to the constitutively active type II serine/threonine kinase receptor (TβRII), whereby it heteromerizes with and activates the type I serine/threonine kinase TGFβ receptor (TβRI). The active TβRI phosphorylates the receptor-activated Smads (R-Smads), Smad 2 and 3, which form trimeric complexes with the co-Smad (Smad4) and translocate to the nucleus, where they regulate gene transcription by associating with other DNA-binding cofactors.Citation1-Citation4 Apart from the canonical Smad pathway, TGFβ signals also through non-Smad pathways. For instance TGFβ activates the extracellular signal-regulated kinase (Erk), c-Jun N-terminal kinase (JNK) and p38 mitogen activated protein kinases (MAPKs), although the precise mechanisms of activation of these pathways remain poorly understood.Citation5-Citation8 The tumor necrosis factor receptor-associated factors (TRAFs) play an important role in the ligand-induced activation of various signaling pathways, including the JNK and p38 MAPK, and nuclear factor kappa β (NF-κB).Citation9,Citation10 TRAF6 was recently shown to be involved in the TGFβ-dependent activation of p38 by TGFβ-associated kinase (TAK1).Citation11,Citation12
TRAF's function as adaptor proteins and exist as 7 members (TRAF 1-7), in which few members have E3 ubiquitin ligase activity (eg; TRAF6, TRAF4).Citation13-Citation16 They contain a conserved C-terminal TRAF domain which interacts with different receptors and is responsible for homo- and hetero-oligomerization of TRAFs,Citation17 and a less conserved N-terminal subdomain containing a RING domain (except for TRAF1).Citation18 TRAF6 interacts with a consensus motif in TβRI and is involved in activation of a Smad-independent pathway; the TβRI-TRAF6 interaction is required for the TGFβ-induced autoubiquitination of TRAF6 and subsequent activation of the TAK1-p38/JNK pathway, leading to apoptosis and epithelial to mesenchymal transition of cells.Citation11,Citation12,Citation19,Citation20
Glycogen synthase kinase 3 (GSK-3) is an important multifunctional serine/threonine kinase implicated in glycogen metabolism, and in signaling via Wnt and tyrosine kinase receptors. GSK-3 exists in 2 isoforms, GSK-3α and GSK-3β, and negatively regulates the Wnt signaling cascade by phosphorylating β-catenin, thereby marking it for degradation. GSK-3β also phosphorylates several residues in c-Jun to promote its proteasomal degradation.Citation21 Therefore, inhibition of the GSK-3 kinase activity may lead to increased levels of c-Jun with subsequent stimulation of transcription of certain genes, including cyclin D1.Citation22,Citation23 GSK-3 has also been reported to be required for increased expression of the proapoptotic protein Bim and subsequently for activator protein 1 (AP-1)-induced cell death.Citation24
c-Jun homo- or heterodimerizes with other members of the Jun/Fos family to form AP-1 transcription factors, which regulate the expression of genes involved in differentiation, proliferation, migration and apoptosis.Citation25 c-Jun also mediates transcriptional responses to stress, and its function is conserved from yeast to mammals.Citation23,Citation26 c-Jun is phosphorylated at its N-terminal Ser63 and Ser73 by the c-Jun N-terminal kinase (JNK) family leading to its activation.Citation27
In this study, we report that TGFβ induces gene transcription of the pro-invasive Snail1. Knockdown of TRAF6 by siRNA prevents TGFβ-induced expression of c-Jun both at RNA and protein level. Moreover, active p38 causes inactivation of GSK-3β, by phosphorylation on Ser9. Finally, we identify Snail1 as a transcriptional target of c-Jun, and show in the current study that c-Jun binds to nucleotides -765 to -696 in the Snail1 promoter. In addition, we show that TGFβ-dependent activation of c-Jun and induction of Snail1 is required for TGFβ-induced migratory responses and invasion of prostate cancer cells.
Results
TGFβ-induced expression of the pro-invasive gene Snail1 in PC-3U cells is dependent on c-Jun and Smad proteins
The transcription factor Snail1 plays a crucial role to confer invasive properties to cancer cells and is known to be induced by TGFβ in a Smad-dependent manner.Citation8,Citation28-Citation30 As we have previously found Snail1 to be a target for the TGFβ-TRAF6 pathway, we wanted to explore further the importance of activated Smad proteins and the transcription factor c-Jun for regulation of Snail1 expression.Citation31 First, we investigated if TGFβ regulated the expression of c-Jun and Snail1 in the human prostate cancer (PC-3U) cells, in which both the canonical Smad and the TRAF6-p38 pathways are active.Citation11,Citation31
Knock- down of either Smad4, Smad2 or Smad3 in PC-3U cells, caused a reduction of TGFβ-induced expression of c-Jun (). Moreover, in human breast carcinoma (MDA-MB-468) cells, lacking expression of Smad4, no TGFβ-induced mRNA or protein expression of c-Jun and Snail1 was observed (). Taken together, these observations confirm that the canonical Smad pathway regulates expression of c-Jun and Snail1, in response to TGFβ, in agreement with the previous reports from Wong et al.,Citation32 and Peinado et al.Citation33
Figure 1. c-Jun is regulated by Smad2, Smad3 and Smad4 in the canonical TGFβ-regulated pathway in breast and prostate cancer cells. (A–C) Cell lysates from PC-3U cells transiently transfected with non-targeting control siRNA (CTRL siRNA) or siRNA specific for Smad4 (A) Smad2 (B) or Smad3 (C) specific siRNA were subjected to immunoblotting for Smad4, Smad2, and Smad3, respectively, and total c-Jun. Actin served as internal control. (D and E) RNA isolated from PC-3U cells and Smad4 homozygous null MDA-MB-468 cells (MD468) treated with TGFβ, as indicated, were subjected to qRT-PCR, to analyze mRNA expression of c-Jun (D) and Snail1 (E). The cell lysates derived from PC-3U cells and MD468 cells treated with TGFβ, were subjected to immunoblotting to determine the amounts of total c-Jun, Snail1 and Smad4 proteins (F). Actin served as internal control.
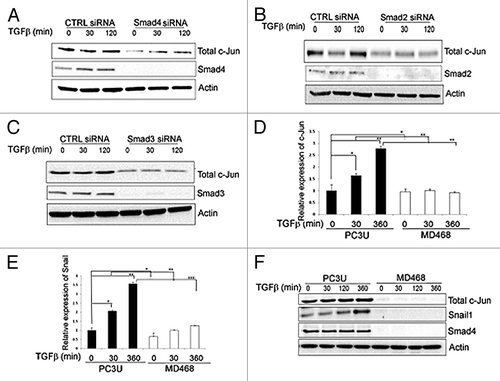
TRAF6 is important for TGFβ-induced regulation of the expression of c-Jun and p21, and for the phosphorylation of GSK-3β
To examine if TRAF6 is required for the TGFβ-induced activation of c-Jun, PC-3U cells were transfected with TRAF6-specific siRNA, or non-targeting control siRNA, and the cells were treated with or without TGFβ for different time periods. TRAF6 mRNA and protein expression were strongly suppressed in cells transfected with siRNA, demonstrating the efficiency of the siTRAF6 treatment (). Knockdown of TRAF6 inhibited the expression of c-Jun mRNA expression when compared with cells transfected with control siRNA (). Jun proteins form homo- or heterodimers with Fos family members (c-Fos, FosB); therefore we investigated also the expression of c-Fos. Interestingly, the expression of c-Fos was found to be significantly reduced after 6 h stimulation by TGFβ both in control cells and in TRAF6 knock-down cells (). Thus, TRAF6 mediates TGFβ-induced expression of c-Jun, but not c-Fos, in PC-3U cells. Moreover, in TGFβ-treated human immortalized keratinocytes (HaCaT) cells, expression of c-Jun increased in response to TGFβ more efficiently in the presence of TRAF6, while no repression of c-Fos was observed (Fig. S1A–C). Interestingly, the expression of p21, a target gene for TGFβ, was only partly downregulated upon knock-down of TRAF6 in PC-3U cells (), suggesting that TRAF6 is crucial for the expression of c-Jun and contributes to the regulation of p21. In contrast, TGFβ-induced expression of Smad7, a direct target gene for the activated Smad-complex, was not affected by TRAF6 knock-down (), while the expression of another conventional TGFβ-regulated gene, PAI-1, was found to be partially decreased in TRAF6 knock-down cells (Fig. S1D). Finally, a decrease in TGFβ-induced activation of the Smad binding element SBE4-Lux, an artificial Smad3/4-specific construct,Citation34 was observed in PC-3U cells after knock-down of c-Jun (Fig. S1D), consistent with the recent finding that c-Jun is required for TGFβ-induced binding of Smad2/3 to the MMP-10 and PAI-1 promoters.Citation35
Figure 2. TGFβ regulates c-Jun in a TRAF6-dependent manner in human prostate cancer (PC-3U) cells. (A and B) RNA and cell lysates derived from PC-3U cells transiently transfected with non-targeting control siRNA (CTRL. siRNA) or TRAF6 specific siRNA (TRAF6 siRNA) and treated with or without TGFβ, were subjected to qRT-PCR (A) or immunoblotting for TRAF6 (B). GAPDH served as an internal control for equal loading of proteins. (C and D) RNA derived from PC-3U cells treated as indicated, were subjected to qRT-PCR analysis for c-Jun and c-Fos. (E and F) RNA and cell lysates derived from PC-3U cells treated as indicated, were subjected to qRT-PCR (E) and immunoblotting for p21 (F). (G) RNA derived from PC-3U cells treated as indicated, were subjected to qRT-PCR for analysis of Smad7 mRNA expression. (H) Cell lysates derived from PC-3U cells treated as indicated, were subjected to immunoblotting for p-Ser63-c-Jun, total c-Jun, p-GSK-3β Ser9, total GSK-3β, p21 and TRAF6. Actin is used as an internal control for equal loading of proteins. (** P < 0.01 and *** P < 0.001 when compared with control siRNA treated with TGFβ). Data are presented as mean values (± S.D.) in 3 independent experiments.
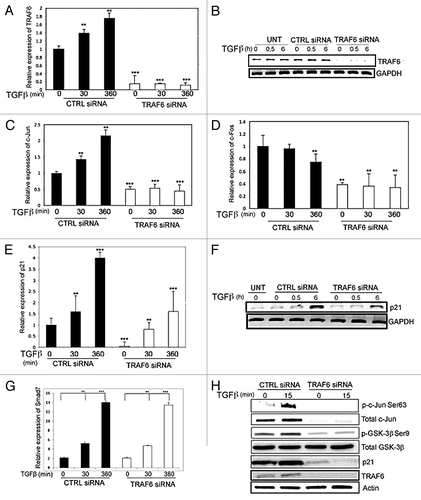
Active GSK3β phosphorylates c-Jun, thereby marking it for proteasomal degradation. We investigated if TRAF6 promotes the inactivation of GSK3β, by phosphorylation on Ser9. PC-3U cells were transfected with TRAF6-specific siRNA and probed with an antiserum against phospho-Ser9 GSK-3β. As shown in , the TGFβ-induced phosphorylation of GSK-3β in cells treated with control siRNA, was not seen upon knock-down of TRAF6, suggesting that TRAF6 is required for inhibition of GSK-3β. This observation is consistent with our previous observation that TRAF6 is required for TGFβ-induced activation of p38 and JNK MAPKs.Citation11
We also investigated the role of TRAF6 for TGFβ-induced activation of p21 and c-Jun in other epithelial cells, such as HaCaT cells. Upon knock-down of TRAF6, the expression of p21 and c-Jun was suppressed, and there was also a clear reduction of TGFβ-induced phosphorylation of c-Jun in HaCaT cells (Fig. S2A). However, the phosphorylation of Smad2 was not inhibited by knock-down of TRAF6.
To investigate further the role of TRAF6 in TGFβ-induced expression of p21 and c-Jun, wild-type and TRAF6-deficient mouse embryonic fibroblasts (MEFs) were used. We found that the expression of p21 and c-Jun, and the phosphorylation of Ser63 in c-Jun, were decreased in the TRAF6-deficient MEFs compared with wild-type cells, while the phosphorylation of Smad2 was similar in wild-type and TRAF6−/− MEFs (Fig. S2B).
To confirm the specificity of the TRAF6 siRNA used in this study, a rescue experiment was performed. PC-3U cells were transfected with Flag-tagged-TRAF6, and after 24 h of transfection, cells were transfected with control siRNA, TRAF6 siRNA, or TRAF6 UTR siRNA that recognizes only the endogenous TRAF6 and not ectopically expressed Flag-TRAF6. Transfected TRAF6 promoted TGFβ-induced phosphorylation of c-Jun at Ser63 and of GSK-3β at Ser9, resulting in robust increase of total c-Jun, in cells co-transfected with control siRNA as well as TRAF6 UTR siRNA, thus demonstrating the specificity of the used TRAF6 siRNA (Fig. S2C).
Taken together, these observations support the notion that TGFβ-induced increase of c-Jun expression is dependent on TRAF6.
p38 regulates the expression and phosphorylation of c-Jun
To investigate if the TRAF6-induced effects on p21 and c-Jun expression, involves the p38 MAPK pathway, the p38 inhibitor SB203580 was used. In the presence of the p38 inhibitor the TGFβ-induced phosphorylation of c-Jun was suppressed, whereas the phosphorylation of JNK was unaltered (). Osmotic shock was used as a positive control to detect p-JNK.
Figure 3. TGFβ regulates c-Jun in a p38-dependent manner in PC-3U cells. (A and B) Cell lysates derived from PC-3U cells, treated or transfected as indicated, were subjected to immunoblotting for p-Ser63-c-Jun, total c-Jun, p-p38, p-GSK-3β Ser9, total GSK-3β and HA (p38). Actin served as internal control. (C) PC-3U cells transiently transfected with HA-p38 wild-type or HA-p38DN were subjected to a non-radioactive in vitro kinase assay using recombinant c-Jun, and cell lysates were subsequently subjected to immunoprecipitation (IP) with HA-antibodies. The product was immunoblotted with p-Ser63-c-Jun antibodies. (D) Inhibitor of p38 (SB203580) were added 1 h before treatment of cells. (E) PC-3U cells transiently transfected with wild-type or Ser9Ala mutant GSK-3β were treated with or without TGFβ, as indicated. The cells were then lysed and subjected to immunoblotting using p-Ser63-c-Jun and GSK-3β antisera. β-tubulin served as internal control for equal loading of proteins.
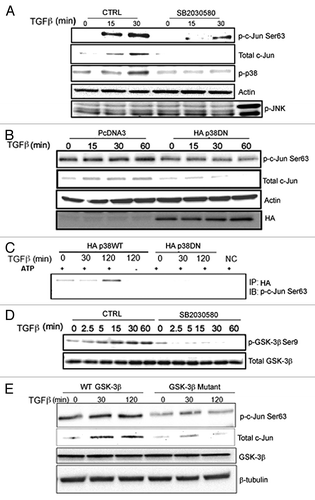
To further explore the possible involvement of p38, a plasmid encoding a HA-tagged dominant-negative p38 (HA-p38DN) was transfected in PC-3U cells, and cells were treated with or without TGFβ. The Ser63 phosphorylation of c-Jun was inhibited in HA-p38 DN transfected cells compared with control cells (), supporting the notion that p38, directly or indirectly, is involved in the phosphorylation of c-Jun.
To further investigate if c-Jun is a specific target for p38α kinase activity in PC-3U cells, an in vitro kinase assay for ectopically expressed and immunoprecipitated p38 was conducted, using lysate from PC-3U cells treated with TGFβ. Wild-type p38α was found to phosphorylate c-Jun in vitro, while kinase-dead p38 did not ().
The stability of c-Jun protein is regulated by active GSK-3β and therefore we investigated if p38 MAPK causes phosphorylation of GSK-3β on Ser9, which is leading to its inhibition. Treatment of PC-3U cells with the p38-inhibitor SB203580, resulted in an inhibition of TGFβ-induced phosphorylation of GSK-3β on Ser9 (), suggesting that p38 causes inactivation of GSK-3β, consistent with previous observations.Citation36,Citation37
To further investigate the importance of TGFβ- and p38α-dependent inactivation of GSK-3β for subsequent increase of c-Jun expression, cell lysates derived from PC-3U cells transfected with wild-type and a Ser9Ala mutant GSK-3β were analyzed by immunoblotting. As demonstrated in , TGFβ-induced phosphorylation and increased expression of c-Jun, was seen only in PC-3U cells transfected with wild-type GSK-3β, but not in cells transfected with Ser9Ala mutant GSK-3β.
GSK-3β is also known to regulate the stability of Smad proteins.Citation38 To investigate the role of GSK-3β for the stability of Smad2 and Smad3 proteins in PC-3U cells, we used siRNA to knock down GSK-3β expression in PC-3U cells. A decrease in the amounts of phosphorylated Smad3 and Smad2 were observed after 120 min of TGFβ-stimulation, when compared with control cells, due to decreased levels of Smad2 and Smad3 in PC-3U cells, upon knock-down of GSK-3β (data not shown). These data proposes that TGFβ causes phosphorylation of c-Jun in a p38α-dependent manner and that active GSK-3β contributes to degradation of c-Jun, as well as of Smad2 and Smad3.
Finally, in order to investigate if the TGFβ-p38 MAPK-c-Jun pathway is differently activated in fibroblasts, we treated p38-deficient and wt MEFs with inhibitors for p38 (SB203580), PI3-kinase (LY294002) or JNK (SP600125). We observed, as expected, that TGFβ-activation of c-Jun is dependent on JNK in MEFs in line with previous reportsCitation39 (Fig. S2D).
TGFβ causes increased expression of c-Jun and p21
To analyze the subcellular localization of active c-Jun, nuclear and cytoplasmic fractions of cells were analyzed separately. Increased expression and phosphorylation of c-Jun, and phosphorylation of Smad2, were observed in the nuclear fraction, but not in the cytoplasmic fraction, after TGFβ stimulation (). We further validated the expression patterns of c-Jun and p21 at the protein level. The expression of p21 and c-Jun was enhanced upon stimulation with TGFβ, whereas phosphorylation of Smad2 was enhanced up to 60 min and thereafter declined (). Upon stimulation with TGFβ, the c-Jun mRNA expression in PC-3U cells increased until 120 min () and thereafter decreased, whereas the expression of p21 mRNA increased until 12 h ().
Figure 4. TGFβ regulates c-Jun and p21 in PC-3U cells. (A) PC-3U cells treated with or without TGFβ were subjected to nuclear and cytoplasmic fractionation; the fractions were then analyzed by immunoblotting with p-Ser63-c-Jun, total c-Jun and p-Smad2 antibodies. Beta-tubulin and the transcription factor YY1 served as controls for cytoplasmic and nuclear fractions, respectively. (B) Cell lysates derived from PC-3U cells were treated with TGFβ as indicated, and then subjected to immunoblotting for p21. The expression of p21 is shown in comparison with immunoblotting for p-Ser63-c-Jun, total c-Jun and p-Smad2. Actin served as internal control. (C and D) RNA derived from PC-3U cells treated with TGFβ for the indicated time periods, was subjected to qRT-PCR for c-Jun and p21.
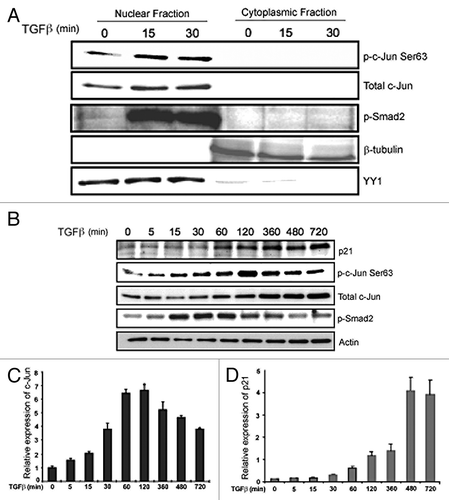
c-Jun regulates the expression of itself and p21
To investigate the effect of c-Jun on its own expression, a dominant negative c-Jun plasmid (Flag-tagged DN c-Jun; lacking 168 amino-acid residues in its N-terminal part, containing the transactivation domain) was transfected into PC-3U cells. The expression of p21 and c-Jun was inhibited by DN c-Jun supporting the notion that c-Jun regulates its own expression (), as expected.Citation40 In PC-3U cells transfected with DN c-Jun, the mRNA expression of PAI1, a target gene of TGFβ, was slightly decreased in DN c-Jun transfected cells (), while expression of p21 and c-Jun mRNA was inhibited (). Moreover, knock-down of c-Jun by siRNA, led to inhibition of TGFβ-induced expression of p21, further illustrating that c-Jun is required for the expression of p21 (). From these data, we conclude that c-Jun contributes to the TGFβ-induced regulation of p21 expression.
Figure 5. TGFβ regulates c-Jun and p21 in PC-3U cells in a c-Jun-dependent manner. (A) Cell lysates derived from PC-3U cells transiently transfected with pcDNA3 or Flag- c-Jun DN and treated with or without TGFβ, were subjected to immunoblotting for p21, p-Ser63-c-Jun, total c-Jun and Flag (c-Jun-DN). (B–D) RNA derived from PC-3U cells treated with or without TGFβ were subjected to qRT-PCR for PAI1, p21 and c-Jun.
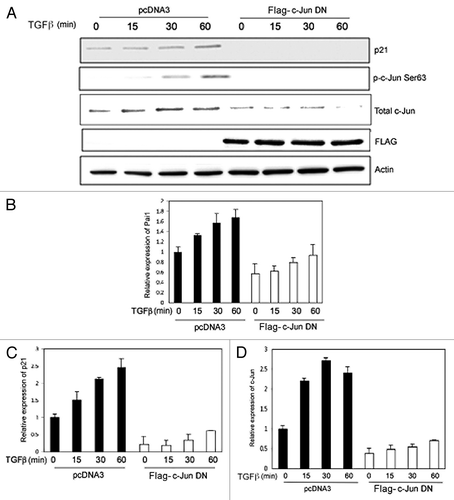
Figure 6. c-Jun is required for TGFβ-induced p21 expression. Cell lysates derived from PC-3U cells transiently transfected with si-CTRL or si-c-Jun, and treated or not with TGFβ for the indicated time periods, were subjected to immunoblotting with antiserum that recognize p21, p-Ser63-c-Jun, total c-Jun and actin. Data from non-treated (NT) PC-3U cells are also shown.
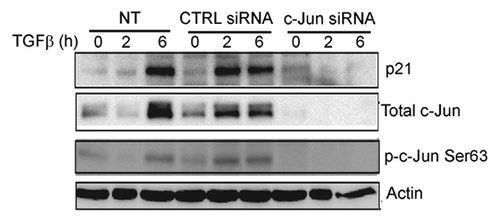
Active p38α and c-Jun colocalize in nuclei of PC-3U cells in response to TGFβ stimulation
To further explore the p38-c-Jun pathway, we investigated whether p38 and c-Jun co-localize in the nuclei of PC-3U cells upon stimulation with TGFβ. Active p38 was found to be co-localized with p-c-Jun Ser63 in the nucleus of TGFβ-stimulated cells (), while neither active p38 nor c-Jun was detected in PC-3U cells treated with p38α siRNA, consistent with our previous observations of a p38-induced phosphorylation of c-Jun. Knock-down of p38α prevented TGFβ-induced activation of c-Jun, by phosphorylation of c-Jun at Ser63, as well as increase of total c-Jun, as demonstrated by immunoblotting (). These observations support the notion that p38α phosphorylates and activates c-Jun in response to TGFβ in PC-3U cells.
Figure 7. p38α is required for TGFβ-induced c-Jun activation. (A) PC-3U cells were transiently transfected with control (CTRL) or p38α (p38) siRNA treated or not with TGFβ for 30 min, and then subjected to a cell culture wound healing assay. Thereafter, the cells were fixed and subjected to co-immunofluorescence stainings for p-p38 (red) and p-Ser63 c-Jun (Scale bar 20 μM). (B) The activation status of c-Jun was analyzed in PC-3U cells transiently transfected with control (CTRL) or p38α (p38) siRNA, treated with or without TGFβ for indicated time periods. The cells were lysed and subjected to immunoblotting using p-Ser63-c-Jun, total c-Jun and p38α antibodies. Actin served as internal control.
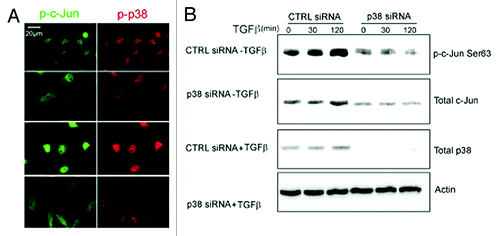
TGFβ-induced expression of the pro-invasive gene Snail1 is dependent on c-Jun
To further investigate the transcriptional role of TGFβ-activated c-Jun, we performed RT-PCR experiments and analysis of protein levels by immunoblotting. We found that c-Jun is required both for TGFβ-induced expression of the pro-invasive gene Snail1, as well as PAI1 (; Fig. S3A). From these findings, we conclude that TGFβ-induced activation of c-Jun regulates the expression of the pro-invasive transcription factor Snail1, known to play a crucial role in TGFβ-driven EMT.
Figure 8. c-Jun promotes TGFβ-induced expression of pro-invasive Snail in prostate cancer cells. RNA and cell lysates were derived from PC-3U cells transiently transfected with non-targeting control siRNA (CTRL siRNA) or c-Jun specific siRNA (c-Jun siRNA) and treated as indicated, were subjected to qRT-PCR (A–C). (D) Cell lysates were subjected to immunoblotting for Snail and PAI1.Actin served as internal control.
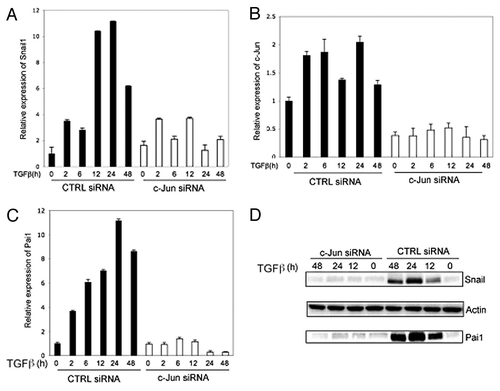
Identification of a c-Jun binding site in the Snail1 promoter
Since we observed that c-Jun was required for induction of Snail1 mRNA in response to TGFβ stimulation, we examined if c-Jun activated by TGFβ, could directly promote transcriptional regulation of Snail1.To test this possibility, we performed DNA precipitation (DNAP) and chromatin immunoprecipitation (ChIP) assays. By using DNAP, a binding site for c-Jun in the human Snail promoter at −756 to −696, was identified (). By performing a ChIP assay, we observed that c-Jun bound to the Snail1 promoter in TGFβ-stimulated PC-3U cells in a TRAF6-dependent manner (; Fig. S3B and C). By knock-down of endogenous TRAF6 in the human breast carcinoma MDA-MB-231 cell line, we found that TRAF6 is required for TGFβ-induced increase of both c-Jun and Snail proteins (Fig. S3D) also in these cells, suggesting a general function for TRAF6 in this pathway. Only a minor binding of Smad2 or Smad3 to the −756 to −696 region of the human Snail1 promoter was seen after TGFβ stimulation of cells (; Fig. S3E). We conclude that TGFβ-induced activation of c-Jun results in its binding to the Snail1 promoter and to an increased expression of this pro-invasive transcription factor.
Figure 9. c-Jun binds to the Snail1 promoter in a TGFβ-dependent manner. (A and B) A c-Jun binding motif is present in the Snail1 promoter. Biotinylated Snail1 oligonucleotide affinity precipitation (DNAp) of endogenous c-Jun from PC-3U cells treated with TGFβ for indicated time periods. * indicates a background band; IgG heavy chain. (C and D) ChIP assay for endogenous c-Jun from PC-3U cells shows enhanced recruitment of c-Jun but not Smad2/3 to this specific region Snail1 promoter in response to TGFβ stimulation. (** P < 0.01 and *** P < 0.001 when compared with control; NC). Data are presented as mean values (± S.D.) in 3 independent experiments.
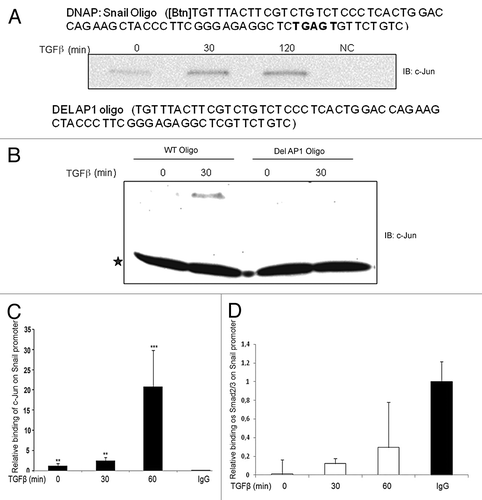
TGFβ-induced cell motility and invasion in PC-3U cells is dependent on c-Jun
Both p38 and c-Jun have been implicated in cell migration during embryogenesis and wound healing.Citation7,Citation37,Citation39,Citation41 To investigate the possible role of activated c-Jun in TGFβ-induced migration of PC-3U cells, we used siRNA to knock-down c-Jun or TRAF6. Silencing of c-Jun or TRAF6 caused a complete inhibition of the TGFβ-induced cell culture wound healing of PC-3U cells (). Importantly, rescue experiments, in which c-Jun was transiently overexpressed in PC-3U cells with TRAF6 silenced, showed an increased cell migration (). Moreover, c-Jun was found to be required for TGFβ-induced invasion of PC-3U cells through Matrigel (). Ectopic expression of HA-tagged Snail1, partially rescued the loss of TGFβ-induced invasive properties in PC-3U cells when endogenous c-Jun was knocked down by siRNA, demonstrating that Snail1acts downstream of c-Jun, to promote invasion (). TGFβ-induced growth inhibition in PC-3U cells and knock-down of c-Jun reduced the basal proliferative response in PC-3U cells (). We noticed that silencing of c-Jun also reduced both the basal migratory capability and the invasiveness of PC-3U cells. These effects may be explained by the fact that PC-3U cells are known to secrete TGFβ2 in an autocrine fashion.Citation42 From these observations we conclude that the TGFβ-induced invasive properties of PC-3U cells are conferred by activation of c-Jun, causing induction of Snail1.
Figure 10. c-Jun promotes TGFβ-induced migration and invasion of prostate cancer cells. (A and B) PC-3U cells transiently transfected with CTRL or c-Jun siRNA were subjected to a cell culture wound migration assay. The wound space at the beginning of the experiment was approximately 0.6 mm. After TGFβ stimulation of cells, the cell movement into the gap was imaged with a digital camera using a Zeiss microscope. The width of the wound was measured with Zeiss Axiovision 4.6.3 software. Wound healing was calculated by dividing the area that had been filled with cells by the originally open area. (C and D) Knock-down of TRAF6 in PC-3U cells, significantly prevented TGFβ-induced cell migration in wound healing assays, while transient transfection of wild-type c-Jun rescues cell migration. (** P < 0.01 and *** P < 0.001 when compared with control siRNA treated with TGFβ). Data are presented as mean values (± S.D.) in 3 independent experiments.
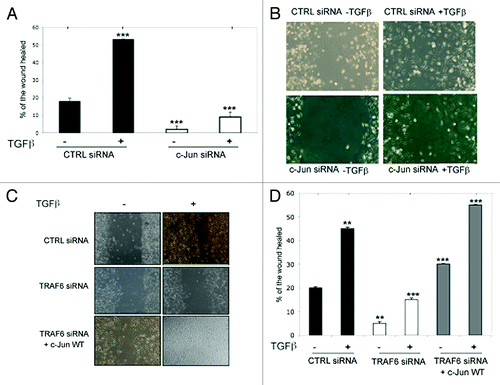
Figure 11. c-Jun promotes TGFβ-induced invasion of prostate cancer cells. (A) Knock-down of c-Jun in PC-3U cells significantly prevented TGFβ-induced invasion. Data are presented as mean values (± S.D.) for invasive cells in 3 independent experiments (***, P < 0.001, when compared with control siRNA). (B) Invasion assay was performed in PC-3U cells transiently transfected with control (CTRL) or c-Jun siRNA, and treated as indicated. Cells were then visualized by staining with crystal violet cell stain solution. (C and D) Invasion assay was performed in PC-3U cells transiently transfected with CTRL, c-Jun siRNA alone or together with HA-tagged Snail1, and treated as indicated. (E) Cell lysates derived from a part of the cells in (C) was subjected to immunoblotting for total c-Jun and HA (Snail). Actin served as internal control for equal loading of proteins. (F) The number of proliferating PC-3U cells transiently transfected with control (CTRL) or c-Jun siRNA, and treated as indicated, was subjected to immunofluorescence stainings of phospo-Histone3 (p-H3). Data are presented as mean values (± S.D.) in 3 independent experiments. (**, P < 0.01, ***, P < 0.001, when compared with control siRNA).
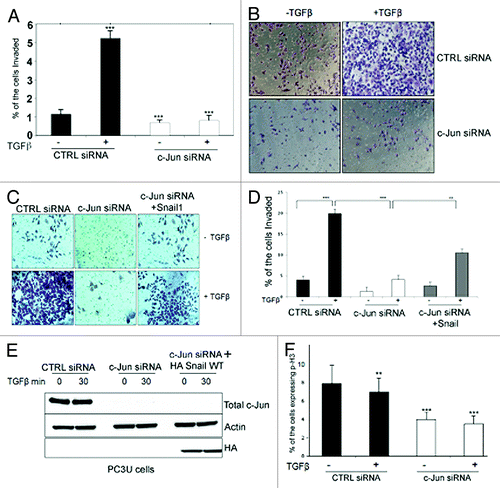
Immunohistochemical stainings for p-Smad2, p-p38, and p-c-JunSer63 in prostate cancer tissues
To investigate the possibility that activation of c-Jun is implicated in prostate cancer, we performed immunohistochemical stainings for p-Ser63-c-Jun, p-p38, and p-Smad2 in prostate cancer tissues. Increased positive stainings for p-Ser63-c-Jun, p-p38, and p-Smad2 were found in the investigated prostate cancer tissues () when compared with normal prostate tissues or highly differentiated prostate cancer tissues (; and data not shown). These data suggest that TGFβ promotes prostate cancer progression, as demonstrated by immunohistochemical stainings of phosphorylated Smad2, and that p38 and c-Jun are activated in prostate cancer cells.
Figure 12. Immunohistochemical detection of p-Smad2, p-p38 and p-c-Jun in prostate cancer tissues. Serial, paraffin-embedded sections of normal prostate and prostate cancer tissues were immunostained with p-Smad2, p-p38 and p-JunSer63 antibodies and biotin-streptavidin amplified peroxidase immunodetection. 3,3′-Diaminobenzidine was used as chromogen and Mayer hematoxylin as counterstain. (A–C), representative images, from normal or highly differentiated prostate cancer tissues. (D–F), representative images from low differentiated prostate cancer tissues. (G–I), Negative control for immunohistochemical stainings, where the primary antibodies, respectively, was omitted. Representative images from cancer tissues (Scale bar 200 μm).
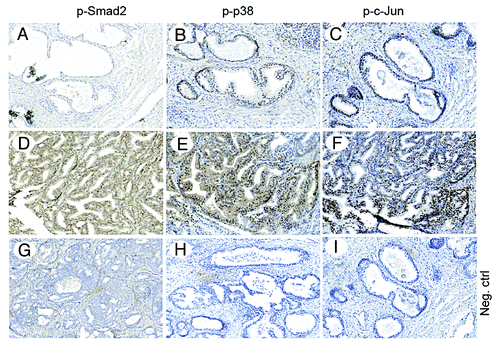
Discussion
TGFβ has been reported to potently induce invasion of cancer cellsCitation3,Citation8,Citation43-Citation46; it is therefore important to elucidate the functional roles of Smad and non-Smad pathways to mediate this effect. Our present study shows that TGFβ signals both via Smad-dependent and Smad-independent pathways to regulate the expression of c-Jun. We confirm that activated Smads in the canonical TGFβ pathway promotes increased expression of c-Jun in breast and prostate cancer cells (MDA-MB 231, MDA-MB 468, and PC-3U), in line with previous findings.Citation32 Moreover, TRAF6 specifically regulates both expression and activity of c-Jun, by p38α MAPK-dependent phosphorylation of c-Jun at Ser63 in cancer cells. TRAF6 was recently shown to be crucial for the TGFβ-induced activation of the TAK1- p38 pathway,Citation8,Citation11,Citation12 while the target genes for this pathway have so far not been well characterized. c-Jun has been shown to be regulated by TGFβ independent of Smad4 in HaCaT and Colo-357 cells,Citation48 which is in line with data in our current study, where we identify TRAF6 as a co-regulator of c-Jun expression in HaCaT cells.
Phosphorylation of Ser63 in c-Jun is crucial for its transcriptional activation. Herein we demonstrate that TRAF6 plays an important role for activation of c-Jun in cancer cells, due to its ability to promote activation of p38α MAPK, which subsequently phosphorylates c-Jun on Ser63, thereby causing its activation. In p38α-deficient MEFs, TGFβ treatment instead caused activation of c-Jun in a JNK-dependent manner, as expected and in line with previous reports.Citation39
In prostate cancer cells, TRAF6-induced activation of p38 was also found to promote phosphorylation of GSK-3β at Ser9, inhibiting its kinase activity and causing increased levels of both c-Jun, as well as of activated Smad 2 and Smad3 proteins, suggesting that TRAF6 both enhances and prolongs Smad-dependent signals evoked by the canonical TGFβ-Smad pathway, probably resulting in the formation of c-Jun homodimers. TRAF6 was previously found to promote TGFβ-induced invasiveness in human breast cancer MDA-MB 231 cells and in human lung carcinoma A549 cells, further supporting its oncogenic regulatory properties in TGFβ-induced responses.Citation31,Citation49 It is of interest to note that UV-light induces expression of Snail1 via c-Jun,Citation50 suggesting that UV-light thereby could promote progression of epithelial tumors.
Interestingly, overexpressed Smad3 has been shown to associate with c-JunCitation51 and the c-Jun gene possesses Smad-binding elements in its promoter,Citation32,Citation52,Citation53 demonstrating that some of the TGFβ target genes might be activated by both pathways, as Smad signaling and JNK/p38 MAPK signaling converge at AP-1-binding promoter sites in specific genes.Citation9,Citation35 The findings presented here, suggests that TRAF6 is required for efficient TGFβ and Smad-induced activation of c-Jun leading to migration and invasion of PC-3U cells. Intriguingly, we also observed that TGFβ-induced transcription of Snail1 requires c-Jun and we identified a c-Jun binding site in the distal region of the Snail1 promoter compared to the Smad3 binding region localized at the proximal region on the Snail1-promoter (−170 to −110 relative to the ATG codon).Citation1,Citation44,Citation54 It is possible that c-Jun recruits other transcriptional co-regulators, to control efficient transcription of this gene. It is also possible that p38 by phosphorylation of c-Jun confer prolonged TGFβ-induced activation of Snail1. Thus, our observations support the notion that TGFβ-initiated transcription of the Snail1-promoter is dependent both on activation of the Smad complex, in the early phase of TGFβ-stimulation of cells and on activation of c-Jun, at later stages, as outlined in a schematic figure (Fig. S4).
In conclusion, our data shows that TGFβ induces c-Jun in a Smad-dependent and TRAF6-dependent manner, by promoting p38α-dependent phosphorylation of c-Jun at Ser63, causing its subsequent transactivation. Thereafter, c-Jun binds to the promoter of Snail1 and induces its expression, conferring migratory and invasive properties to cancer cells. Further experiments are required to investigate if endogenous c-Jun recruits other transcriptional factors to this region of the Snail1 promoter. It will also be important to explore the possibility that active c-Jun can be used as a biomarker for aggressive prostate cancer and to search for pharmacological inhibitors of this pro-invasive pathway.
Materials and Methods
Cell culture
The human prostate cancer cell line (PC-3U), a sub-line originating from PC-3 cells,55 and breast carcinoma cell lines; MDA-MB-231, and Smad4 homozygous null MDA-MB-468, were cultured in RPMI 1640 medium, supplemented with 10% fetal bovine serum (FBS), glutamine and penicillin/streptomycin. Cells were cultured at 37 °C in an atmosphere of 5% CO2. Transient transfection of PC-3U cells was performed as described earlier.Citation11 Wild-type and TRAF6−/− mouse embryo fibroblasts (MEFs), and HaCaT cells, were grown in Dulbecco modified Eagle medium (DMEM) containing 10% FBS and penicillin/streptomycin. Cells were cultured at 37 °C in an atmosphere of 5% CO2. Transient transfection of wild-type and TRAF6−/− MEFs was performed as described earlier.Citation11 Cells were stimulated with TGFβ after starvation for at least 12 h in medium containing 0.5% FBS.
Antibodies and other reagents
TGFβ1 was purchased from R&D Systems. Antibodies to c-Jun, c-Jun phospho-Ser63, p21, GSK-3β, and GSK-3β phospho-Ser9, were from Cell Signaling. Monoclonal anti-β-actin antibody was from Sigma. The rabbit anti-TRAF6 (C-term) was from Zymed laboratories. Phospho-Smad2, p-JNK, and p-Histone 3 antibodies were from Cell Signaling. The p38 α and β inhibitor 4-(4-fluorophenyl)-2-(4-methylsulfinylphenyl)-5-(4-pyridyl)1H-imidazole (SB203580) and the PI3K inhibitor 2-(4-morpholinyl)-8-phenyl-4H-1-benzopyran-4-one (LY294002) were from Calbiochem; inhibitors were used at a concentration of 10 µM, and were added to cells 1 h before TGFß treatment. The JNK1 inhibitor (SP 600125) was purchased from Alexis Biochemicals. Rabbit HA antibody was from Santa Cruz Biotechnology. β-tubulin antibody was from Sigma. The YY1 antibody was a kind gift from Johan Ericsson. A mouse anti-Flag M2 monoclonal antibody was from Sigma. Pefabloc was from Roche. Secondary HRP conjugated anti-mouse, anti-rabbit and anti-goat Ig was from GE Healthcare.
Immunoblotting and in vivo protein interactions
PC-3U cells were grown in 10-cm dishes and starved for 12–18 h in medium containing 1% FBS, glutamine and penicillin/streptomycin. Wild-type and TRAF6−/− MEFs were grown in 10 cm dishes and starved for at least 12 h in medium containing 0.5% FBS. The cells were then treated with 10 ng/ml TGFβ for the indicated time periods, and then harvested for subsequent analysis. Equal amounts of proteins were used for immunoprecipitations and subjected to SDS–PAGE in 8, 10 or 12% polyacrylamide gels, followed by blotting onto polyvinylidene difluoride membranes, as described previously.Citation5,Citation11
RNA preparation
TRAF6 SMART pool siRNA was used as previously described.Citation11 Twenty-one-base-pair siRNA duplexes for TRAF6 and c-Jun were synthesized by Dharmacon Research. The targeted sequence for c-Jun siRNA duplex (5′ NNGUCAUGAA CCACGUUAAC A3′), or a nonspecific duplex oligonucleotide (5′ AACAGUCGCG UUUGCGACUG G3′) as a negative control, were used in transient transfections of cells (12.8 µg/10-cm plate) by transfection with Lipofectamine (Invitrogen) at a ratio of 1 µg of RNA to 3 µl of Lipofectamine. Signal silence p38α MAPK siRNA II, cataloge number #6277 was purchased from Cell Signaling Technology and transiently transfected into PC-3U cells to knock down endogenous p38α. TGFβ was added 24 h after transfection to the cells starved in medium containing 1% FBS, glutamine and penicillin/streptomycin for 12 h.
Plasmids
A dominant-negative (DN) p38 plasmid was a kind gift from Dr J. Han. The Flag-tagged DN c-Jun plasmid, consists of amino acid residues 169–334 of c-Jun, which contains the dimerization and DNA binding domains, was a kind gift from Dr M. Karin. Hemagglutinin (HA)-tagged Snail1, was a kind gift from Dr A. Moustakas.
Nuclear and cytoplasmic fractionation assay
PC-3U cells were grown in 10-cm dishes and starved for at least 12 h in medium containing 1% FBS, glutamine and penicillin/streptomycin. The starved cells were then treated with TGFβ for the indicated time periods and the cells were washed two times with ice-cold PBS, then scraped in ice-cold PBS and centrifuged; the pellet was collected and ice-cold buffer 1 was added (20 mM TRIS-HCl pH 7.0, 10 mM KCl, 2 mM MgCl2, 0.5% NP40, 1 mM aprotinin, 1 mM Pefabloc). The cells were sheared mechanically with a syringe and needle, then samples were centrifuged and the supernatant, i.e., the cytoplasmic fraction, was collected. The remaining pellet was washed 3 times with buffer 1, and then re-suspended in buffer 2 (buffer 1, supplemented with 0.5 M NaCl) and centrifuged; the supernatant was collected as the nuclear protein fraction.
Preparation of total RNA and cDNA
Total RNA from the cells were isolated with an RNeasy mini kit (Qiagen), according to the manufacturer’s instructions. The isolated RNA was quantified using a Nanodrop ND-100 spectrophotometer. Two micrograms (2 μg) of total RNA was used for cDNA (cDNA) preparation. For cDNA synthesis, we used Thermoscript RT PCR system (Invitrogen), following the manufacturer’s instructions. The cDNA obtained was quantified for its purity by using a Nanodrop spectrophotometer. After quantification the cDNA was diluted 10-fold with RNase-free water.
Analysis of mRNA levels by quantitative Real Time PCR (qRT PCR)
Two microgram (2 μg) of cDNA purified from the cells was amplified and measured in duplicates with RT-PCR using Stratagene system, with SYBR green (Applied Biosystems) to detect the PCR product. Specific primers for TRAF6, p21, c-Jun and c-Fos were constructed with the aid of the Primer3plus free software http://www.bioinformatics.nl/cgiin/primer3plus/primer3plus.cgi and the primers were purchased from Sigma Aldrich. The primer sequences were: TRAF6 forward (for), CTGCAAAGCC TGCATCATAA; TRAF6 reverse (rev), GGGGACAATC CATAAGAGCA; PAI (for), CTGCAAAGCC TGCATCATAA; PAI (rev), GTGGAGAGGC TCTTGGTCTG; C-FOS (for), AGAATCCGAA GGGAAAGGAA; C-FOS (rev), CTTCTCCTTC AGCAGGTTGG; GAPDH (for), GAAGATGGTG ATGGGATTTT C; GAPDH (rev), GAAGGTGAAG GTCGGAGT; CJUN (for), CCCCAAGATC CTGAAACAGA; CJUN (rev), CCGTTGCTGG ACTGGATTAT; p21 (for), ATGAAATTCA CCCCCTTTCC; p21 (rev), CCCTAGGCTG TGCTCACTTC.
Snail sequences have been previously reported (11). A 25 µl reaction mixture containing 12.5 µl of SYBR green PCR mix, 2 µl of diluted cDNA, 10 pmoles per μl of forward and reverse primers and RNase free water was used. GAPDH gene was chosen as an internal reference in the real-time PCR protocol. As a negative control, one sample with no cDNA (only with RNase free water) was included in each run of the RT-PCR assay, for each primer pair.
Wound healing assays
Wound healing assays were performed using serum-starved confluent cells growing on non-coated sterile cover-slides in 6-well plates without coating; a 1000-μl pipette tip was used for scratching creating an approximately 0.6 mm wide wound, as previously described in detail in Ekman et al., 2012.Citation37 Photomicrographs were obtained by a microscope (Axioplan 2; Carl Zeiss MicroImaging, Inc) with a digital camera (C4742-95; Hamamatsu), using a Plan-neofluar 40×/0.75 objective lens (Carl Zeiss MicroImaging, Inc); photographs were taken at room temperature. Primary images were acquired with the camera's QED software.
Invasion assay
Invasion assays were performed by using the BD BioCoat Growth Factor Reduced MATRIGEL Invasion chamber according to manufacturer’s protocol (BD 1).
Histology of human prostate cancer tissues
Tissue sections of normal and malignant tissues were stained using anti-phospho-Smad2, anti-phospho p-p38, and anti-phospho-Ser63-c-Jun. The stained tissues were scanned. Ethical permit to use tumor tissues for generation of tissue slides was provided by the Umeå ethical review board in full agreement with the Swedish Ethical Review Act (540/03, Dnr 03-482).
Statistical Analysis
Differences between means were evaluated by Student’s t test. The values of control and samples were compared with chi-square test (χ2 statistics). A value of P ≤ 0.05 was considered as a statistically significant difference between groups. The calculations were performed with the aid of the Windows version of MS Excel.
In vitro kinase assay
PC-3U cells transfected with HA-p38WT or HA-p38DN were stimulated with TGFβ for indicated time periods and lysed in TGN buffer (50 mM Tris, pH 7.5, 50 mM glycerophosphate, 150 mM NaCl, 10% glycerol, 1% Tween 20, 1 mM NaF, 1 mM NaVO4, 1 mM phenylmethylsulfonylfluoride, 2 μg/ml Pepstatin, 5 μg/ml Leupeptin, 10 μg/ml aprotinin, 1 mM dithiothreitol [DTT]). A part of the lysates was subjected to immunoblotting for HA, and the remaining part was used for the in vitro kinase assay. Lysates were subjected to immunoprecipitation with 2 μg of rabbit anti-HA antibody (Santa Cruz; 1 h incubation at 4 °). Immunocomplexes were isolated with Protein G Sepharose (50 μl of 50% slurry in PBS), and washed 2 times in TGN buffer and 2 times in kinase buffer (10 mM HEPES, pH 7.5, 50 mM glycerophosphate, 50 mM NaCl, 10 mM MgCl2, 10 mM MnCl2, 5 μM ATP, 1 mM DTT). Beads containing the immunoprecipitated p38 were gently resuspended in 30 μl of kinase buffer, 2 μl of 5 μM ATP, 1 μg of recombinant c-Jun fusion protein (Cell Signaling), and were incubated for 1 h at 30 °. Samples were gently centrifuged for 5 min and the supernatant was transferred in to a new tube. Sample buffer with reducing agent was added to the supernatants thereafter samples were heated at 95 ° for 5 min and loaded on a 10% polyacrylamide gel. After electrophoresis, proteins were transferred to polyvinylidene difluoride membranes and subjected to immunoblotting with a rabbit pSer63-cJun antibody from Cell Signaling.
DNAP assays
PC3U cells were plated on 10 cm dishes at 50% confluence and grown for 60–72 h before stimulation for different time periods with 10 ng/ml TGFβ. Proteins were extracted in NP-40 lysis buffer (1% NP-40, 150 mM NaCl, and 50 mM Tris pH 8.0), incubated with the double-stranded biotin-Snail1 probe or the control probe with oligonucleotides −765 to −696 deleted in the presence of 8 mg poly(dI-dC) for 2 h at 4 °C, followed by incubation for 45 min with streptavidin-beads and centrifugation. The DNA-bound protein complexes were washed four times in NP-40 lysis buffer and then resolved by SDS-PAGE followed by immunoblotting. The oligonucleotide sequences used are shown in .
Additional material
Download Zip (521 KB)Disclosure of Potential Conflicts of Interest
No potential conflicts of interest were disclosed.
Acknowledgments
We are grateful to Drs J Han, J Inoue, C Kampf, M Karin, K Miyazono, A Moustakas, F Pontén, and P ten Dijke for their kind supply of expression vectors, reagents and cells. We have used the Cell Analysis and Uppsala Genome Center core facilities at the Rudbeck Laboratory, Uppsala University. We thank Nikolay Aksenov for valuable discussions and the use of bioinformatics programs and Pernilla Andersson for kind help with scanning of tissue samples. We also thank Aris Moustakas and our colleges at the Ludwig Institute for Cancer Research, Uppsala Branch for valuable discussions. This work was partly supported by the Ludwig Institute for Cancer Research and grants to M.L. from the Knut and Alice Wallenberg Foundation, Swedish Medical Research Council, the Swedish Cancer Society, Umeå University, Lions Cancerfond in Umeå, and a regional agreement between Umeå University and Västerbotten County Council on cooperation in the field of Medicine, Odontology and Health.
References
- Heldin CH, Landström M, Moustakas A. Mechanism of TGF-beta signaling to growth arrest, apoptosis, and epithelial-mesenchymal transition. Curr Opin Cell Biol 2009; 21:166 - 76; http://dx.doi.org/10.1016/j.ceb.2009.01.021; PMID: 19237272
- Massagué J. TGF-beta signal transduction. Annu Rev Biochem 1998; 67:753 - 91; http://dx.doi.org/10.1146/annurev.biochem.67.1.753; PMID: 9759503
- Massagué J. TGFbeta in Cancer. Cell 2008; 134:215 - 30; http://dx.doi.org/10.1016/j.cell.2008.07.001; PMID: 18662538
- Ross S, Hill CS. How the Smads regulate transcription. Int J Biochem Cell Biol 2008; 40:383 - 408; http://dx.doi.org/10.1016/j.biocel.2007.09.006; PMID: 18061509
- Edlund S, Bu S, Schuster N, Aspenström P, Heuchel R, Heldin NE, ten Dijke P, Heldin CH, Landström M. Transforming growth factor-beta1 (TGF-beta)-induced apoptosis of prostate cancer cells involves Smad7-dependent activation of p38 by TGF-beta-activated kinase 1 and mitogen-activated protein kinase kinase 3. Mol Biol Cell 2003; 14:529 - 44; http://dx.doi.org/10.1091/mbc.02-03-0037; PMID: 12589052
- Derynck R, Zhang YE. Smad-dependent and Smad-independent pathways in TGF-beta family signalling. Nature 2003; 425:577 - 84; http://dx.doi.org/10.1038/nature02006; PMID: 14534577
- Engel ME, McDonnell MA, Law BK, Moses HL. Interdependent SMAD and JNK signaling in transforming growth factor-beta-mediated transcription. J Biol Chem 1999; 274:37413 - 20; http://dx.doi.org/10.1074/jbc.274.52.37413; PMID: 10601313
- Mu Y, Gudey SK, Landström M. Non-Smad signaling pathways. Cell Tissue Res 2012; 347:11 - 20; http://dx.doi.org/10.1007/s00441-011-1201-y; PMID: 21701805
- Landström M. The TAK1-TRAF6 signalling pathway. Int J Biochem Cell Biol 2010; 42:585 - 9; http://dx.doi.org/10.1016/j.biocel.2009.12.023; PMID: 20060931
- Song HY, Régnier CH, Kirschning CJ, Goeddel DV, Rothe M. Tumor necrosis factor (TNF)-mediated kinase cascades: bifurcation of nuclear factor-kappaB and c-jun N-terminal kinase (JNK/SAPK) pathways at TNF receptor-associated factor 2. Proc Natl Acad Sci U S A 1997; 94:9792 - 6; http://dx.doi.org/10.1073/pnas.94.18.9792; PMID: 9275204
- Sorrentino A, Thakur N, Grimsby S, Marcusson A, von Bulow V, Schuster N, Zhang S, Heldin CH, Landström M. The type I TGF-beta receptor engages TRAF6 to activate TAK1 in a receptor kinase-independent manner. Nat Cell Biol 2008; 10:1199 - 207; http://dx.doi.org/10.1038/ncb1780; PMID: 18758450
- Yamashita M, Fatyol K, Jin C, Wang X, Liu Z, Zhang YE. TRAF6 mediates Smad-independent activation of JNK and p38 by TGF-beta. Mol Cell 2008; 31:918 - 24; http://dx.doi.org/10.1016/j.molcel.2008.09.002; PMID: 18922473
- Adhikari A, Xu M, Chen ZJ. Ubiquitin-mediated activation of TAK1 and IKK. Oncogene 2007; 26:3214 - 26; http://dx.doi.org/10.1038/sj.onc.1210413; PMID: 17496917
- Bouwmeester T, Bauch A, Ruffner H, Angrand PO, Bergamini G, Croughton K, Cruciat C, Eberhard D, Gagneur J, Ghidelli S, et al. A physical and functional map of the human TNF-alpha/NF-kappa B signal transduction pathway. Nat Cell Biol 2004; 6:97 - 105; http://dx.doi.org/10.1038/ncb1086; PMID: 14743216
- Bradley JR, Pober JS. Tumor necrosis factor receptor-associated factors (TRAFs). Oncogene 2001; 20:6482 - 91; http://dx.doi.org/10.1038/sj.onc.1204788; PMID: 11607847
- Wajant H, Henkler F, Scheurich P. The TNF-receptor-associated factor family: scaffold molecules for cytokine receptors, kinases and their regulators. Cell Signal 2001; 13:389 - 400; http://dx.doi.org/10.1016/S0898-6568(01)00160-7; PMID: 11384837
- Dempsey PW, Doyle SE, He JQ, Cheng G. The signaling adaptors and pathways activated by TNF superfamily. Cytokine Growth Factor Rev 2003; 14:193 - 209; http://dx.doi.org/10.1016/S1359-6101(03)00021-2; PMID: 12787559
- Zapata JM, Reed JC. TRAF1: lord without a RING. Sci STKE 2002; 2002:pe27; PMID: 12023442
- Thakur N, Sorrentino A, Heldin CH, Landström M. TGF-beta uses the E3-ligase TRAF6 to turn on the kinase TAK1 to kill prostate cancer cells. Future Oncol 2009; 5:1 - 3; http://dx.doi.org/10.2217/14796694.5.1.1; PMID: 19243289
- Landström M, Sundar R. TRAF6. Encyclopedia of Signaling Molecules, 2012:pp 1916-21.
- Nikolakaki E, Coffer PJ, Hemelsoet R, Woodgett JR, Defize LH. Glycogen synthase kinase 3 phosphorylates Jun family members in vitro and negatively regulates their transactivating potential in intact cells. Oncogene 1993; 8:833 - 40; PMID: 8384354
- Sabbah M, Courilleau D, Mester J, Redeuilh G. Estrogen induction of the cyclin D1 promoter: involvement of a cAMP response-like element. Proc Natl Acad Sci U S A 1999; 96:11217 - 22; http://dx.doi.org/10.1073/pnas.96.20.11217; PMID: 10500157
- Shaulian E, Karin M. AP-1 in cell proliferation and survival. Oncogene 2001; 20:2390 - 400; http://dx.doi.org/10.1038/sj.onc.1204383; PMID: 11402335
- Hongisto V, Smeds N, Brecht S, Herdegen T, Courtney MJ, Coffey ET. Lithium blocks the c-Jun stress response and protects neurons via its action on glycogen synthase kinase 3. Mol Cell Biol 2003; 23:6027 - 36; http://dx.doi.org/10.1128/MCB.23.17.6027-6036.2003; PMID: 12917327
- Hess J, Angel P, Schorpp-Kistner M. AP-1 subunits: quarrel and harmony among siblings. J Cell Sci 2004; 117:5965 - 73; http://dx.doi.org/10.1242/jcs.01589; PMID: 15564374
- Toone WM, Morgan BA, Jones N. Redox control of AP-1-like factors in yeast and beyond. Oncogene 2001; 20:2336 - 46; http://dx.doi.org/10.1038/sj.onc.1204384; PMID: 11402331
- Kallunki T, Deng T, Hibi M, Karin M. c-Jun can recruit JNK to phosphorylate dimerization partners via specific docking interactions. Cell 1996; 87:929 - 39; http://dx.doi.org/10.1016/S0092-8674(00)81999-6; PMID: 8945519
- Massagué J. TGFβ signalling in context. Nat Rev Mol Cell Biol 2012; 13:616 - 30; http://dx.doi.org/10.1038/nrm3434; PMID: 22992590
- Moustakas A, Heldin CH. Coordination of TGF-β signaling by ubiquitylation. Mol Cell 2013; 51:555 - 6; http://dx.doi.org/10.1016/j.molcel.2013.08.034; PMID: 24034692
- Gudey SK, Wallenius A, Landström M. Regulated intramembrane proteolysis of the TGFβ type I receptor conveys oncogenic signals. Future Oncol 2014; http://dx.doi.org/10.2217/fon.14.45; PMID: 24597658
- Mu Y, Sundar R, Thakur N, Ekman M, Gudey SK, Yakymovych M, Hermansson A, Dimitriou H, Bengoechea-Alonso MT, Ericsson J, et al. TRAF6 ubiquitinates TGFβ type I receptor to promote its cleavage and nuclear translocation in cancer. Nat Commun 2011; 2:330; http://dx.doi.org/10.1038/ncomms1332; PMID: 21629263
- Wong C, Rougier-Chapman EM, Frederick JP, Datto MB, Liberati NT, Li JM, Wang XF. Smad3-Smad4 and AP-1 complexes synergize in transcriptional activation of the c-Jun promoter by transforming growth factor beta. Mol Cell Biol 1999; 19:1821 - 30; PMID: 10022869
- Peinado H, Quintanilla M, Cano A. Transforming growth factor beta-1 induces snail transcription factor in epithelial cell lines: mechanisms for epithelial mesenchymal transitions. J Biol Chem 2003; 278:21113 - 23; http://dx.doi.org/10.1074/jbc.M211304200; PMID: 12665527
- Dennler S, Itoh S, Vivien D, ten Dijke P, Huet S, Gauthier JM. Direct binding of Smad3 and Smad4 to critical TGF beta-inducible elements in the promoter of human plasminogen activator inhibitor-type 1 gene. EMBO J 1998; 17:3091 - 100; http://dx.doi.org/10.1093/emboj/17.11.3091; PMID: 9606191
- Sundqvist A, Zieba A, Vasilaki E, Herrera Hidalgo C, Söderberg O, Koinuma D, Miyazono K, Heldin CH, Landegren U, Ten Dijke P, et al. Specific interactions between Smad proteins and AP-1 components determine TGFβ-induced breast cancer cell invasion. Oncogene 2013; 32:3606 - 15; http://dx.doi.org/10.1038/onc.2012.370; PMID: 22926518
- Bikkavilli RK, Feigin ME, Malbon CC. p38 mitogen-activated protein kinase regulates canonical Wnt-beta-catenin signaling by inactivation of GSK3beta. J Cell Sci 2008; 121:3598 - 607; http://dx.doi.org/10.1242/jcs.032854; PMID: 18946023
- Ekman M, Mu Y, Lee SY, Edlund S, Kozakai T, Thakur N, Tran H, Qian J, Groeden J, Heldin CH, et al. APC and Smad7 link TGFβ type I receptors to the microtubule system to promote cell migration. Mol Biol Cell 2012; 23:2109 - 21; http://dx.doi.org/10.1091/mbc.E10-12-1000; PMID: 22496417
- Fuentealba LC, Eivers E, Ikeda A, Hurtado C, Kuroda H, Pera EM, De Robertis EM. Integrating patterning signals: Wnt/GSK3 regulates the duration of the BMP/Smad1 signal. Cell 2007; 131:980 - 93; http://dx.doi.org/10.1016/j.cell.2007.09.027; PMID: 18045539
- Wagner EF, Nebreda AR. Signal integration by JNK and p38 MAPK pathways in cancer development. Nat Rev Cancer 2009; 9:537 - 49; http://dx.doi.org/10.1038/nrc2694; PMID: 19629069
- Karin M, Liu Zg, Zandi E. AP-1 function and regulation. Curr Opin Cell Biol 1997; 9:240 - 6; http://dx.doi.org/10.1016/S0955-0674(97)80068-3; PMID: 9069263
- Etienne-Manneville S. Cdc42--the centre of polarity. J Cell Sci 2004; 117:1291 - 300; http://dx.doi.org/10.1242/jcs.01115; PMID: 15020669
- Ikeda T, Lioubin MN, Marquardt H. Human transforming growth factor type beta 2: production by a prostatic adenocarcinoma cell line, purification, and initial characterization. Biochemistry 1987; 26:2406 - 10; http://dx.doi.org/10.1021/bi00383a002; PMID: 3475130
- Thiery JP, Acloque H, Huang RY, Nieto MA. Epithelial-mesenchymal transitions in development and disease. Cell 2009; 139:871 - 90; http://dx.doi.org/10.1016/j.cell.2009.11.007; PMID: 19945376
- Heldin CH, Moustakas A. Role of Smads in TGFβ signaling. Cell Tissue Res 2012; 347:21 - 36; http://dx.doi.org/10.1007/s00441-011-1190-x; PMID: 21643690
- Nieto MA. Epithelial plasticity: a common theme in embryonic and cancer cells. Science 2013; 342:1234850; http://dx.doi.org/10.1126/science.1234850; PMID: 24202173
- Yang J, Weinberg RA. Epithelial-mesenchymal transition: at the crossroads of development and tumor metastasis. Dev Cell 2008; 14:818 - 29; http://dx.doi.org/10.1016/j.devcel.2008.05.009; PMID: 18539112
- Levy L, Hill CS. Alterations in components of the TGF-beta superfamily signaling pathways in human cancer. Cytokine Growth Factor Rev 2006; 17:41 - 58; http://dx.doi.org/10.1016/j.cytogfr.2005.09.009; PMID: 16310402
- Gudey SK, Sundar R, Mu Y, Wallenius A, Zang G, Bergh A, Heldin CH, Landström M. TRAF6 stimulates the tumor-promoting effects of TGFβ type I receptor through polyubiquitination and activation of presenilin 1. Sci Signal 2014; 7:ra2; http://dx.doi.org/10.1126/scisignal.2004207; PMID: 24399296
- Li Y, Liu Y, Xu Y, Voorhees JJ, Fisher GJ. UV irradiation induces Snail expression by AP-1 dependent mechanism in human skin keratinocytes. J Dermatol Sci 2010; 60:105 - 13; http://dx.doi.org/10.1016/j.jdermsci.2010.08.003; PMID: 20851575
- Zhang Y, Feng XH, Derynck R. Smad3 and Smad4 cooperate with c-Jun/c-Fos to mediate TGF-beta-induced transcription. Nature 1998; 394:909 - 13; http://dx.doi.org/10.1038/29814; PMID: 9732876
- Inman GJ, Hill CS. Stoichiometry of active smad-transcription factor complexes on DNA. J Biol Chem 2002; 277:51008 - 16; http://dx.doi.org/10.1074/jbc.M208532200; PMID: 12374795
- Liberati NT, Datto MB, Frederick JP, Shen X, Wong C, Rougier-Chapman EM, Wang XF. Smads bind directly to the Jun family of AP-1 transcription factors. Proc Natl Acad Sci U S A 1999; 96:4844 - 9; http://dx.doi.org/10.1073/pnas.96.9.4844; PMID: 10220381
- Thuault S, Tan EJ, Peinado H, Cano A, Heldin CH, Moustakas A. HMGA2 and Smads co-regulate SNAIL1 expression during induction of epithelial-to-mesenchymal transition. J Biol Chem 2008; 283:33437 - 46; http://dx.doi.org/10.1074/jbc.M802016200; PMID: 18832382
- Franzén P, Ichijo H, Miyazono K. Different signals mediate transforming growth factor-beta 1-induced growth inhibition and extracellular matrix production in prostatic carcinoma cells. Exp Cell Res 1993; 207:1 - 7; http://dx.doi.org/10.1006/excr.1993.1156; PMID: 7686495