Abstract
The stability of mammalian telomeres depends upon TRF2, which prevents inappropriate repair and checkpoint activation. By using a plasmid integration assay in yeasts carrying humanized telomeres, we demonstrated that TRF2 possesses the intrinsic property to both stimulate initial homologous recombination events and to prevent their resolution via its basic N-terminal domain. In human cells, we further showed that this TRF2 domain prevents telomere shortening mediated by the resolvase-associated protein SLX4 as well as GEN1 and MUS81, 2 different types of endonucleases with resolvase activities. We propose that various types of resolvase activities are kept in check by the basic N-terminal domain of TRF2 in order to favor an accurate repair of the stalled forks that occur during telomere replication.
Keywords: :
Introduction
The stability of telomeres depends upon 2 main types of mechanisms. The first depends on telomerase activity, which can compensate for replicative erosionCitation1; the second relies on a specific chromatin organization, which protects chromosome ends from aberrant signaling and repair.Citation2 The chromatin-mediated telomere protection involves pathways regulated by several proteins, which bind directly or indirectly telomeric DNA, such as the shelterin complex.Citation3 Among the numerous mammalian telomere associated proteins identified in the last decade, the telomeric DNA-binding protein TRF2 (Telomere repeats binding factor 2) has a central role in orchestrating the molecular events that maintain telomere integrity in mammals.Citation4,Citation5
At the cellular level, suppression of TRF2 induces, depending on the cell type, apoptosis or senescence.Citation6,Citation7 This effect can be explained by the capacities of TRF2 to prevent activation of the DNA damage response (DDR) at telomeresCitation8 and inappropriate repair.Citation7 Moreover, TRF2 helps folding telomeric DNA into t-loop, which might contribute to telomere protection by masking the 3′ overhang.Citation9-Citation11
In telomerase-positive and telomerase-negative primary cells, TRF2 behaves as a negative regulator of telomere length.Citation12-Citation18 Indeed, in telomerase-positive cancer cells, overexpression of TRF2 leads to telomere shortening that does not depend upon telomerase activity.Citation13 XPF-ERCC1, a structure-specific endonuclease involved in nucleotide excision repair, crosslink repair and homologous recombination, is required for TRF2-promoted telomere shortening in mouse and human cells.Citation19,Citation20
Interestingly, the rate of telomere shortening due to TRF2 overexpression is exacerbated when a form of TRF2 lacking the N-terminal basic domain (named B domain hereafter) is expressed, a process that has been proposed to involve t-loop homologous recombination (HR).Citation21 On one hand, TRF2-SLX4 complex might provide the endonuclease activities of SLX1 and MUS81 for t-loop resolution.Citation21-Citation23 On the other hand, the B domain of TRF2 can block, in reconstituted reactions, the cleavage by various recombination endonucleases able to process Holliday junction and replication fork.Citation24 Collectively, these findings suggest that the B domain of TRF2 limits the action of endonucleases, thus regulating t-loop resolution.
In this study, we investigated the recombination properties of TRF2 in yeast cells whose telomerase was reprogrammed to synthesize human-type repeats instead of yeast sequences (named hereafter humanized yeasts)Citation21,Citation25-Citation28 and in human telomerase-positive cells. The results obtained in these 2 cellular systems converge toward a model for a dual role of TRF2 during homologous recombination, acting both to promote initiation and to prevent resolution by inhibiting the action of endonucleases with resolvase activity.
Results
Design of yeast assays to study human telomere recombination
In order to investigate the intrinsic properties of TRF2 regarding recombination, we developed a simple assay in budding yeast, physiologically devoid of TRF2, to study recombination events at human telomeric DNA. This assay is performed in humanized yeasts, which carry a template mutation in the telomerase RNA gene (tlc1-h) that directs the synthesis of human-type telomeric repeats at chromosome ends.Citation25 The replacement of yeast (TG1–3) by human-type (T2AG3) repeats allows the formation of functional telomeres.Citation26,Citation27 In these cells, recombination at telomeres is monitored by determining the rate of telomere integration of a non-replicative circular plasmid carrying a stretch of human-type repeats; a similar plasmid but containing a yeast telomeric tag was used as a control ().
Figure 1. T2AG3-dependant integration in humanized yeasts. (A) Wild-type (TLC1) or humanized yeasts (tlc1-h) are transformed with a non-replicative telomeric plasmid containing either T2AG3 or TG1–3. The rate of integration is determined by selection on G418 plates and normalized with the transformation efficiency obtained with a co-transformed replicative plasmid. (B) Humanized yeasts carrying tlc1-h template were transformed with a plasmid expressing the wild-type TLC1. A plasmid shuffle allows the strain to keep only the TLC1 or both the TLC1 and tlc1-h templates. (C) Genomic DNA was digested by XhoI and 1 kb TRF (telomere restriction fragments) are released. Southern blot was performed and DNA was revealed with 3 different probes: T2AG3, TG1–3 and Y’ (subtelomeric fragment, DNA loading control). Hybridization intensities are measured with Imagequant shareware. T2AG3 and TG1–3 intensities are normalized according to the Y’ intensity corresponding to the total DNA quantity. (D) Clones were transformed with the human-type telomeric plasmid. The rate of integration is determined by selection on G418 plates and normalized with the transformation efficiency obtained with a replicative plasmid co-transformed.
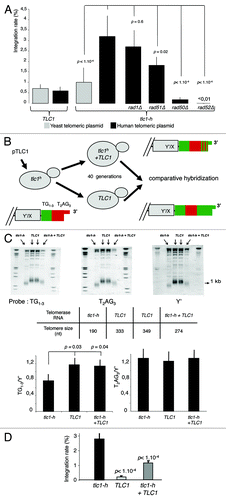
In order to account for differences in the transformation efficiencies, yeast cells were co-transformed with the replicative plasmid pRS415 conferring leucine prototrophy and the non-replicative telomeric plasmid conferring the G418 resistance at a molar ratio of 1:50. The Leu+ transformants were replica-plated on plates containing G418 to determine the integration rates, which correspond to the ratio of colonies that acquired both markers (Leu+ G418R) to the total number of Leu+ colonies. In wild-type yeast cells, the integration rate of plasmids containing yeast telomeric DNA is low and roughly similar to plasmids containing human repeats (). These results are in agreement with previous studies showing that yeast telomeres are poorly recombinogenic.Citation29 In humanized yeasts, the integration rate of the human-type telomeric plasmid is significantly higher than the one of the yeast-type telomeric plasmid (). For the vast majority of G418 resistant clones, the plasmid is integrated as 1 or 2 copy(ies) within a terminal fragment of humanized yeast chromosomes (Fig. S1). Plasmid integration requires RAD50 and RAD52, 2 genes involved in HR, but not RAD1, involved in nucleotide excision repair (). We conclude that the plasmid integration events occurring at humanized telomeres are mediated by homologous recombination.
In tlc1-h cells, the rate of plasmid integration, while being only partially dependent upon RAD51, is almost totally abolished in rad50Δ and rad52Δ cells (). This is reminiscent of break-induced replication mechanisms and suggests that plasmid integration involves one-ended strand invasion.Citation30 Similar events of telomeric plasmid integration were previously observed in wild-type budding yeast.Citation31
In order to study whether the plasmid integration assay depends upon an “end-effect”, i.e., a strand invasion initiated by the most distal repeats of the G-tail, we re-introduced a plasmid expressing the wild-type TLC1 gene in humanized yeasts (). After transformation, it is expected that a significant proportion of human-type telomeric DNA (T2AG3) becomes internal due to the addition of yeast repeats (TG1–3) by telomerase.Citation32 Indeed, 40 generations after transformation, we observed a significant increase in the proportion of yeast repeats while the amount of human type repeats appeared unchanged as determined by comparative hybridization analyses (). Concomitantly to these sequence changes at the very end of chromosomes, we observed a significant decrease in the rate of plasmid integration, which cannot be due to a lower amount of human-type telomeric repeats since, as explained above, we could not observe obvious changes in the amount of human-type telomeric repeats (). We conclude that sequence-dependent invasion of the G-tail into the duplex part of plasmid DNA is required for integration. One possible mechanism involves the extension of the G-tail by copying the plasmid DNA, in a rolling circle-type reaction. However, since only 1 or 2 copy(ies) of the plasmid DNA is found within the telomeric regions (Fig. S1), this replication-based mechanism seems unlikely. We rather believe that invasion of the G-tail results in the formation of a joint molecule followed by its resolution. Furthermore, the critical role played by the G-tail can provide an explanation for the higher rate of plasmid integration observed in humanized yeasts, indeed these cells have longer G-tail than wild-type yeasts.Citation21,Citation33-Citation36
In summary, recombination events between T2AG3 repeats in humanized yeasts are particularly efficient and might be a consequence of G-tail invasion into duplex telomeric DNA. Therefore, the plasmid integration assay appears to reflect some of the recombination events that are thought to occur at mammalian telomeres: recombination of extrachromosomique telomeric DNA, resolution of t-loop structures and 3′ overhang strand invasion into telomeric duplex DNA.Citation37
TRF2 inhibits telomere recombination in yeast via its basic N-terminal domain
Next we investigated the influence of human TRF2 in the integration rate of the human-type telomeric plasmid. First, we verified that ectopically expressed TRF2 is able to bind to telomeres of humanized yeasts by using a telomeric one-hybrid assay where we analyzed the capacity of TRF2, fused to the Gal4 activation domain (AD), to transactivate a subtelomeric UAS-less HIS3 gene (). Then, we introduced a series of galactose-inducible versions of the TERF2 gene in humanized yeast cells and verified their expression upon addition of galactose (). Additionally we verified that once induced, TRF2 expression does not change the growth rate of humanized yeast cells (data not shown).
Figure 2. Recombinogenic properties of TRF2 in humanized yeasts. (A) In humanized yeasts, the VII-L distal end was replaced by the reporter gene HIS3. TRF2 is fused to a transcription activation domain (AD), and expressed in the humanized strain carrying the reporter gene. The humanized yeast strain (SD-URA) is replica-plated on SD-URA-HIS + 3-AT to monitor the expression of HIS3. (B) Scheme and western blots showing the structure and the inducibility of the TRF2, TRF2∆B, TRF2∆M, TRF2∆B∆M, or TRF1 genes. Glc, glucose; G/R, galactose. (C) The humanized yeasts expressing TRF2, TRF2∆B, TRF2∆M, TRF2∆B∆M, or TRF1 were transformed with the human-type telomeric plasmid. The rate of integration is determined by selection on G418 plates and normalized with the transformation efficiency obtained with a replicative plasmid co-transformed. (D) Clones coming from the humanized yeasts expressing TRF2 or TRF2∆B and having integrated the plasmid were restreaked 1 (R1), 2 (R2), 3 (R3), or 4 (R4) times on YPD and after restreaked on selective G418 medium. The rate of excision is determined by selection on G418 plates of clones that does not grown anymore. (E) In gel assay to determine the quantity of 3′ overhang in humanized yeasts containing various forms of human shelterin proteins.
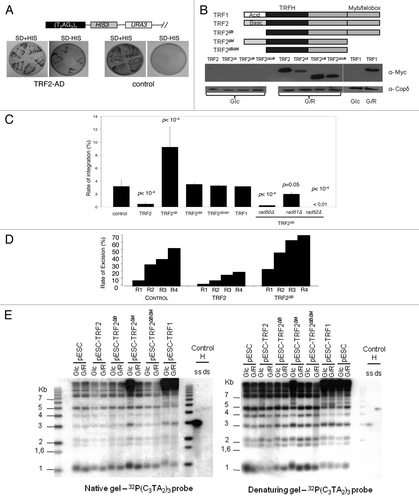
Four generations after galactose induction, the humanized cells were co-transformed with the non-replicative telomeric plasmid and the pRS415 conferring leucine prototrophy. In TRF2-expressing cells, the frequency of plasmid integration drops as compared with non-induced cells grown in glucose or to cells grown in galactose but containing an empty expression plasmid or devoid of any expression plasmid (). This effect is TRF2 specific, as demonstrated by the absence of any effect due to TRF1, and requires the Myb/Telobox DNA binding domain (named M) of TRF2 since expression of TRF2∆M and TRF2∆B∆M do not modify the integration rate. However, although TRF2 binding to telomeric DNA is necessary, it is not sufficient since the expression of TRF2∆B, which maintains the ability to bind to telomeric DNA, does not inhibit plasmid integration ().
Upon serial restreaking, the G418R cells give rise to G418S colonies that have lost the integrated copy of the Kan gene, as demonstrated by Southern blot analysis (Fig. S2). These data show that the Kan gene has been excised, most likely by an HR event between its flanking human-type telomeric repeats. In agreement with the anti-recombination activity of TRF2, the rate of plasmid excision decreased in cells expressing TRF2 but not TRF2∆B suggesting that the B domain inhibits intra-chromosomal HR events in yeast ().
These results show that the B domain of TRF2 maintains its anti-recombination properties in humanized yeasts, validating the use of the humanized yeast assay to study the role of TRF2 in telomere recombination.
TRF2∆B stimulates telomere recombination in yeast
The expression of TRF2∆B not only reverse the anti-integration effects of TRF2 but also markedly increases telomere recombination since the rate of integration augmented by almost 3-folds as compared with control cells that do not express TRF2 or cells that express truncated TRF2 versions unable to bind telomeric DNA (TRF2∆M and TRF2∆B∆M) (). This increase depends upon RAD52, RAD50 and to a much less extend upon RAD51, suggesting that it corresponds to the HR pathway that operates to integrate the telomeric plasmid in the absence of TRF2 expression (; compare with ). In concordance with these results, TRF2∆B also stimulates plasmid excision from humanized telomeres, showing that the lack of B domain enhances intra-chromosomal HR events ().
Since the plasmid integration depends upon the presence of human-type telomeric repeats and of a homologous 3′ overhang, it is possible that TRF2∆B increases the length of the duplex DNA and/or of the 3′ overhang. However, we have been unable to detect any change in telomere length and 3′ overhang size that could account for the pro-recombinogenic properties of TRF2∆B (). We conclude that TRF2∆B possesses an intrinsic activity that stimulates HR. This property might be explained by the capacity of TRF2 to favor the invasion of the telomere 3′ overhang into the target DNA of the transformed plasmidCitation10,Citation38 and/or to promote Holliday junction formation.Citation24
MUS81, GEN1 and SLX4 are required for TRF2∆B -mediated shortening in human cells
Then, we investigated whether the B-domain can also inhibit HR at human telomeres. In particular, we investigated the activity at telomeres of the major recombination endonucleases: MUS81, GEN1 and SLX1-SLX4. In agreement with previous studies 7, 2121, the overexpression of TRF2ΔB in HT1080 supertelomerase cells (named hereafter superHT1080) reduced the average telomere length () by trimming the leading strand telomere, as revealed by quantitative CO-FISH (qCO-FISH) assays (). Downregulation of either SLX4 or MUS81 or GEN1 expression did not notably change telomere length in superHT1080 cells, conversely in cell expressing TRF2ΔB, a marked telomere lengthening effect was observed with respect to cells treated with a scrambled sh construct (). This lengthening was confirmed in qFISH experiments (). Thus, we conclude that the B domain of TRF2 restrains the action of SLX4, MUS81 and GEN1 at telomeres, thereby preventing excessive telomere shortening.
Figure 3. TRF2ΔB reduced the telomere length by trimming the leading strand telomere. (A) The genomic DNA from infected superHT1080 cells expressing an empty vector or TRF2ΔB was purified, digested and then separated on the same gel before to be transferred on a membrane. (T2AG3)n probe, specific to telomeric DNA, was used. The observed signal was analyzed to measure telomere length. Position of the mean telomere length is indicated by a white bar. Molecular weights (kb) are indicated at the bottom of the gel. (B) Preferential deletion of telomeric leading strand detected by qCO-FISH. Metaphase spreads of infected superHT1080 cells, expressing an empty vector or TRF2ΔB and incubated with BrdU/BrdC, were treated to remove the newly synthesized DNA strand. The remaining telomeric DNA strands were detected with PNA-C-Cy3 and LNA-G-FITC probes. Each telomeric signal was quantified as a telomeric intensity value (unit arbitrary, u.a.) and the mean telomeric intensity value was calculated for each strand (± s.e.m) and represented in graphic. Statistical analyses were done using the Wilcoxon rank-sum test. Ns is for no significant and *** is extremely significant with the P value < 0.001.
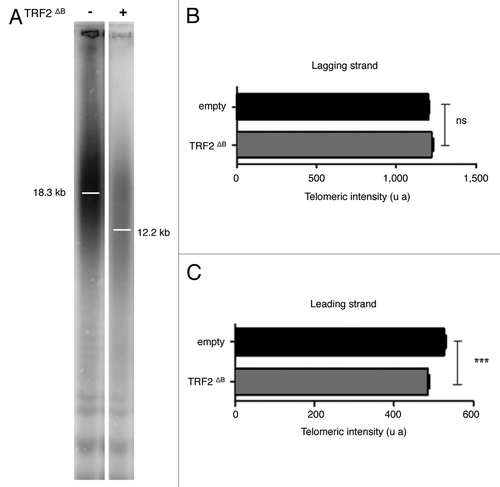
Figure 4. MUS81, GEN1, and SLX4 are required for TRF2∆B-mediated shortening in human cells. (A) At the top, total RNA from infected superHT1080 cells, expressing the pLKO.1 vector shMUS81, shGEN1, shSLX4, or sh non target, was purified and analyzed by RT-qPCR analysis. The graph represents the percent of mRNA inhibition for each MUS81, GEN1, and SLX4 mRNAs. At the bottom, proteins from infected superHT1080 cells were extracted and analyzed by western blot. (B) TRF length analysis of superHT1080 cells infected first with lentivirus expressing shMUS81, shGEN1, shSLX4, or sh non target and then with an empty control or TRF2 ΔB lentivirus. The different conditions are indicated above the gel. The genomic DNA was purified; equal amounts were digested by RsaI and HinfI restriction enzymes, and then separated on the same gel before to be transferred on a membrane. (T2AG3)n probe was used. The bars on the gel image indicate the position of the telomeric average size. On the left, the numbers indicate the size corresponding to the ladder (in kb). (C) Q-FISH analysis to measure the telomere length distribution of superHT1080 cells infected with TRF2 ΔB lentivirus. Both telomeric DNA strands were detected with PNA-C-Cy3 probe. Mean telomere lengths given as mean ± s.e.m. Statistical analyses were done using the Wilcoxon rank–sum test (***P < 0.001 = highly significant).
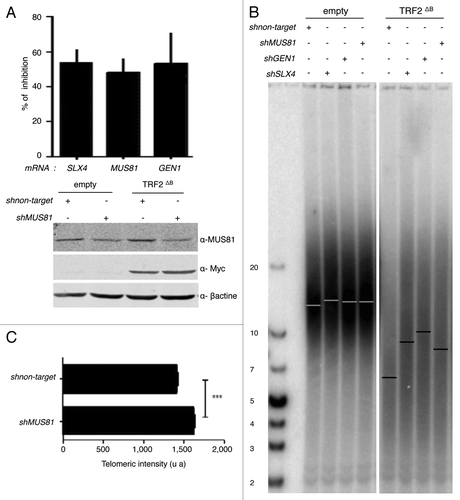
SLX4, MUS81, and GEN1 are required for telomere replication
A reduction in the expression of either Slx4 or Mus81 or Gen1 increases the number of multitelomeric signals (MTS) at chromatid ends (), an aberrant structure that is enhanced by conditions known to cause replication fork collapse and that has been interpreted as a sign of telomere fragility.Citation21,Citation39,Citation40 However, there were no effects of the knock down of these endonucleases on MTS formation in TRF2∆B cells (). These results show that, under wild-type conditions, SLX4, GEN1 and MUS81 are all necessary for telomere replication, as assayed by the MTS phenotype. But how to explain that there is no MTS increase triggered by a reduced expression of these endonucleases in TRF2∆B cells? A simple explanation is that, when replication-derived intermediates are formed under wild-type conditions, the levels of endonucleases required at telomeres to repair them is higher due to the inhibitory activity of the B domain, whereas, when this inhibition is alleviated (e.g., in TRF2∆B cells), lower concentrations of the endonucleases are sufficient.
Figure 5. SLX4, MUS81, and GEN1 are required for telomere stability. (A) Quantification of the average number of multi-telomeric signals (MTS) per superHT1080 metaphase spreads (mean ± s.e.m.). Statistical tests: ***P < 0.001 = highly significant; **P value from 0.001 to 0.01 = very significant; *P value between 0.01 and 0.05 = significant; ns P value > 0.05 = not significant. (B) Images of superHT1080 metaphase spreads showing examples of MTS (white circles). Green, PNA-C-Cy3 probe; blue, DAPI.
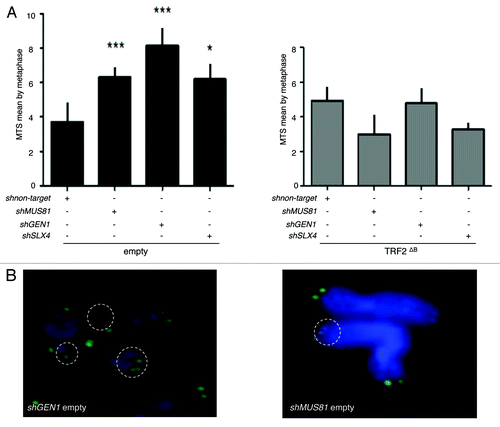
Discussion
The main result of this study is to show in human cells that the basic domain of TRF2 (named B) prevents the telomere shortening triggered by MUS81, GEN1, and a SLX4-associated endonucleases. This finding is in agreement with previous studies showing that, in reconstituted reactions, this domain inhibits the action of endonucleases with resolvase activity, including GEN1.Citation24
We propose that in the presence of unwanted replication intermediates (such as stalled forks) or t-loops, the N-terminal basic domain of TRF2 prevents MUS81, GEN1 and SLX4-associated endonucleases to process these telomere structures at full rein. The strand-specific bias toward the loss of the leading strand telomere in TRF2∆B cellsCitation21 (and this study), together with other studies pointing to a particular role of TRF2 in the protection of the leading telomere,Citation41,Citation42 suggests that an excessive processing of a stalled fork or a t-loop by structure specific endonucleases causes the deletion of the leading strand, while allowing the lagging strand to be fully replicated. This mechanism together with previous findings indicating that the B domain of TRF2 regulates telomere replication through arginine methylationCitation43-Citation45 as well as the activity of WRN helicase,Citation46 FEN-1 flap-endonucleaseCitation47,Citation48 and polymerase βCitation47,Citation49 predict that this TRF2 domain is particularly important for cells experiencing an elevated rate of telomere replication problems.Citation50 This could be particularly true in the case of supertelomerase HT1080 cells, which harbors abnormally long telomeres.Citation21,Citation41,Citation42
It is likely that the TRF2∆B -mediated shortening also stems from the intrinsic properties of this protein to stimulate the initial step of homologous recombination as suggested by its ability to promote strand invasion and Holliday junction in vitroCitation24 and to stimulate plasmid integration in humanized yeasts (). These results suggest a dual role of TRF2 in telomere HR, stimulating initiation and preventing resolution.
Together with the fact the acid N-terminal domain of TRF1 is involved in fork progression,Citation39,Citation52 our results suggest that the recent divergence during chordate evolution between the N-termini of TRF1 and TRF2Citation51 has been selected to decrease the risk of telomere damages during replication and therefore to delay replicative senescence, a process antagonized by p53-mediated TRF2 degradation.Citation53
Materials and methods
Plasmid and yeast procedures
The wild-type strain (TLC1) used is the W303 strain (Mata leu2–3 111 his3–11 15 ura3–1 ade2–1 trp1–1 can1–100). The humanized strain (tlc1-h) (MATa trp1 his3 leu2 ade2 lys2 ura3 TLC1:tlc1-h) derive from spores of the diploid strain C6.1.Citation25
The recombination tests are performed in the humanized strain and derived strain. Gene disruptions are performed in the humanized strain by replacing the entire ORF to be deleted by a cassette containing the NAT1 gene screening for nourseothricin sensitivity and checking for the correct chromosomal insertion by PCR.
The plasmids pLC3, pLC10, pLC11, pLC16, pK1, pMK3, pMK4, pMK5, pMK6 were constructed by cloning proteins ORF in pESC-W plasmid (StratageneTM) and leads to the expression of, respectively, TRF2∆B, TRF2∆M, TRF2∆B∆M, TRF2, TRF1 proteins detectable by western blot upon galactose induction.
The one hybrid test is performed in humanized cells transformed with the SphI/NotI fragment from the modified pHumaTel plasmid,Citation26 containing a portion of ADH4, the full-length HIS3 gene, the URA3 selective marker and a T2AG3 stretch of 60 bp. Because of a recombination event at the ADH4 locus, the 2 genes, HIS3 and URA3, are placed immediately adjacent to a newly formed chromosomal end on the left arm of chromosome VII, and the stretch of T2AG3 formed a new seed for telomere formation. At chromosome VII-L, the HIS3 gene has been used as a reporter gene in the “one-hybrid” system.
pRS415 is used to normalized the integration rate to the transformation efficiency. The non-replicative telomeric plasmids derived from pUC19 (Fermentas) in which the Geneticin gene (G418) is cloned with or without 700 bp stretch of T2AG3 or 280 bp stretch of TG1–3.
The genes encoding the proteins to be tested were cloned on a plasmid, fused to the Gal4 activation domain. The transactivation assay is performed on DS-URA-HIS + 3-amino-1,2,4-triazole (3-AT) (30 mM) (3-AT is a competitive inhibitor of the HIS3 gene product, so that a higher level of HIS3 expression is required for growth when 3-AT is present in the medium).
Plasmid DNA for expression in human cells
Myc-tagged TRF2ΔB was subcloned into pWPIR-GFP lentiviral vector (a gift from D Trono, Ecole Normale Supérieure de Lyon), upstream of the IRES to allow individual translation of the bicistronic mRNA containing both myc-TRF2ΔB and GFP. The pLKO.1-puro lentiviral vectors expressing the short-hairpin (sh) RNA MUS81 or GEN1 or SLX4 or scrambled sequences (called non target), used as control, were purchased from Sigma and contained a puromycine-resistance gene.
Cell culture, transfections, lentiviral production, and infections
Human Embryonic Kidney 293 T cells and superHT1080 fibrosacoma cells were grown in DMEM with 10% fetal calf serum and penicillin-streptomycin at 37 °C and 5% CO2. The superHT1080 fibrosacoma cells (a kindly gift from J Lingner), overexpressing hTERT and hTERC proteins, were maintained in 200 µg/mL of G418 and hygromycine B. VSVg-pseudotyped self-inactivating (SIN) lentiviruses, expressing an empty vector or TRF2ΔB, shMUS81, shGEN1, shSLX4, or shnon target, were produced by transient transfection of Human Embryonic Kidney 293 T cell lines (by calcium phosphate precipitation) with 2 other packaging plasmids, p8.91 and pVSVg. 36 h post transfection, the infectious supernatant was collected, filtered, concentrated, and store at −80 °C. The infection efficiency was investigated by determining the percentage of GFP-positive cells using FACS analysis 3 d after infection or the number of clones after 1 wk of selection with 1 µg/mL of puromycine. All the infections were done at a multiplicity of infection (m.o.i) of 2. First the superHT1080 cells were infected with the lentivirus expressing shMUS81, shGEN1, shSLX4, or shnon target. Seven days after the first transduction, the cells were re-infected with lentivirus expressing an empty vector or TRF2ΔB. For all the experiments the cells were harvested at 14 d after the first infection.
Southern blot
Genomic DNAs are extracted with the DNeasy Blood and Tissue Kit (Qiagen). 8µg of genomic DNA were digested with relevant restriction enzymes (HinfI/RsaI) and separated by field inversion gel electrophoresis (FIGE-BioRad; parameters: forward 160 V, reverse 100V and switch time between 0.1 to 0.8 secondes) on 1% agarose gel/0.5X TBE. After verified the quality of the separation by UV, the gel was denatured, depurinated and neutralized inside the following solutions: 0.25 M HCl, 0.5 M NaOH/1M NaCl, and 1 M NH4 acetate. Then by capillarity (20× SSC), the DNA was blotted onto a nitrocellulose membrane (Hybond N+, Amersham Biosciences) and crosslinked by UV (254 nm and 0.12 Joule). The membranes was neutralized with 250 mM pH 7.2 phosphate buffer at 50 °C, prehybridized one hour at 50 °C (6× SSC, 0.5× SDS, 1% milk) and hybridized O.N. at 50 °C (6× SSC, 0,1× SDS, 1% milk) with radioactive telomeric probes, (T2AG3)n, labeled by random priming with α-dCTP32 (Exo-Klenow DNA pol, Biolabs). The membrane was exposed to a phosphorimager screen, scanned (Fuji FLA5100), and telomere length was analyzed using Multigauge software (Fuji).
The probe (T2AG3)n used was a fragment of 700 nucleotides from pLC14-T2AG3 plasmid (restriction sites: XbaI/SalI).
Q-FISH
Metaphase chromosomes spreads were prepared from 1.106 of superHT1080 infected cells (previously treated with colcemid [1 h, 0.1 μg/ml], following by a hypotonic KCl shock [75 mM, 20 min at 37 °C] and methanol- acetic acid fixation [3:1 and O.N. at 4 °C]). Metaphase spreads, prepared the day before, were fixed with formaldehyde (Sigma, 3.7%) and digested with pepsin (Sigma, 1 mg/ml). Spreads were denatured at 80 °C for 4 min in the presence of a Cy3-(CCCTAA)3 PNA probe (Panagene, in 70% formamide, 25 mM Tris pH 7.4) and incubated at room temperature for 1 h. After washes and ethanol dehydration, preparations were mounted in Vectashield (Vector) with DAPI (1 µg/ml) and visualized with the Axioplan Carl Zeiss UV microscope equipped with appropriate excitation/emission filters. Images were captured with a HQ-Coolsnap camera (Photometrics) using the IPlab software. The images were analyzed by Ivision software. The data are represented as a mean intensity of all metaphases. Statistical analyses were done using the Wilcoxon rank–sum test.
CO-FISH
After release from thymidine/aphidicolin block, superHT1080 cells were incubated with 10 μM BrdU and 3.3 μM BrdC, and then, 9 h after release from the aphidicolin block, cells were arrested in mitosis by an incubation in colcemid (0.1 μg/mL, 1.5 h). Cells were then treated with sodium citrate (0.8 g/L, 40 min at 37 °C) and fixed in ethanol/acetic acid (3:1, O.N. at 4 °C). Metaphase spreads were obtained by dropping suspensions of fixed cells onto clean glass slides and were rapidly used for hybridization. Metaphase spreads were fixed with formaldehyde (Sigma, 3.7%), digested with pepsin (Sigma, 1 mg/ml), treated with 0.5 µg/mL Hoescht 33258, exposed to UV (365 nm) during 30 min at 55 °C, and treated 10 min with 3 U/µL of exonucléase III. Spreads were denatured at 80 °C for 4 min in the presence of a Cy3-(CCCTAA)3 PNA probe (Panagene, in 70% formamide, 25 mM Tris pH 7.4) and incubated at room temperature for 1 h. After washes and ethanol dehydration, preparations were incubated at room temperature for 1 h with a G-rich (Fam)GGGT+TAGGG+T+TAG+GGTTAGGG +T+TAG+GG+T+TAGGG+TTA(Fam) LNA (Exiqon) probe. Then the procedure was performed as described for Q-FISH. The data are represented as a mean intensity of all metaphases. Statistical analyses were done using the Wilcoxon rank-sum test.
Immunoblots
0.5.106 superHT1080 infected cells were lysed by a RIPA solution during 30 min at 4 °C. After centrifugation the supernatant was collected and the amount of proteins was quantified by the method of Micro-Bradford (gamme BSA). Laemmli buffer was added and proteins were boiled 5 min at 90 °C. Fifty (50) µg of proteins samples are separated by SDS-PAGE, transferred to optitran nitrocellulose membranes (Whatman) and probed with antibody followed by secondary detection with dye-conjugated antibodies (DyLight680 Thermo Scientific, 35568, or DyLight 800 Thermo Scientific, 35521, diluted at 1/5000) to be detected at the Odyssey.
Antibodies used: anti-Myc tag antibody 9E10 and anti-actin were from Sigma and anti-Copδ was a generous gift of Pr Letourneur; anti-MUS81, mouse monoclonal (ABCAM; ab14387) 1/200; anti-Beta-actine, rabbit polyclonal (Abca; ab8227); anti-Myc, mouse monoclonal (Cell Signaling; #2276) diluted at 1/750.
qRT–PCR
Total RNA was extracted with the RNeasy miniprep kit (Qiagen) using on-column DNase digestion (RNase-Free DNase Set, Qiagen). RNA concentration was determined by measuring the OD at 260 nm. For quantitative RT–PCR (qRT–PCR), cDNAs were prepared using High Capacity RNA-to-cDNA Master Mix (Applied Biosystems) followed by Stepone qPCR, using the Fast SYBR® Green Master Mix (Applied Biosystems), performed as the manufacturer’s instructions. Serial dilutions of the negative control (shnon target) were used as standard. As a control for DNA contaminations, RT–PCR without RT was performed in parallel for each sample, which did not result in detectable PCR products.
For amplification of the house-keeping RPL19 mRNA, forward and reverse primers were 5′- ATCGATCGCC ACATGTATCA-3′ and 5′- GCGTGCTTCC TTGGTCTTAG-3′, respectively.
Primers used:
qGEN1-F: 5′- CACATCCCCT TGCGTAATCT-3′
qGEN1-R: 5′-CCGAGACTGA TTCCTCTTGC-3′
qMUS81-F: 5′- CGCACAGCAG ACATTAAGGA-3′
qMUS81-R: 5′- ACCGACTGGG CCTTATTCTT-3′
qSLX4-F: 5′- ACACCCAAAG GTGCTAATCG-3′
qSLX4-R: 5′- GTCCAGGGTC TGGTGAGTGT-3′
Additional material
Download Zip (1.1 MB)Disclosure of Potential Conflicts of Interest
No potential conflicts of interest were disclosed.
Acknowledgments
This work was supported by grants from the Ligue Nationale contre le Cancer (E.G. and A.L.V., “équipes labellisées”), from the Institut National du Cancer (E.G. and A.L.V., program TELINCA as well as EG program TELOCHROM), from Agence Nationale de la Recherche (E.G., programs FURETT, TELOREP, and TELOLOOP), from the European Community (RISC-RAD FI6R-CT-2003-508842; TELOMARKER Health-F2-2007-200950) and from Istituto Pasteur-Fondazione Cenci Bolognetti to F.A. This work was performed using the microscopy (PICMI) and cytometry (CYTOMED) facilities of IRCAN.
References
- Cech TR. Beginning to understand the end of the chromosome. Cell 2004; 116:273 - 9; http://dx.doi.org/10.1016/S0092-8674(04)00038-8; PMID: 14744437
- Giraud-Panis MJ, Teixeira MT, Géli V, Gilson E. CST meets shelterin to keep telomeres in check. Mol Cell 2010; 39:665 - 76; http://dx.doi.org/10.1016/j.molcel.2010.08.024; PMID: 20832719
- de Lange T. Shelterin: the protein complex that shapes and safeguards human telomeres. Genes Dev 2005; 19:2100 - 10; http://dx.doi.org/10.1101/gad.1346005; PMID: 16166375
- Broccoli D, Smogorzewska A, Chong L, de Lange T. Human telomeres contain two distinct Myb-related proteins, TRF1 and TRF2. Nat Genet 1997; 17:231 - 5; http://dx.doi.org/10.1038/ng1097-231; PMID: 9326950
- Bilaud T, Brun C, Ancelin K, Koering CE, Laroche T, Gilson E. Telomeric localization of TRF2, a novel human telobox protein. Nat Genet 1997; 17:236 - 9; http://dx.doi.org/10.1038/ng1097-236; PMID: 9326951
- Karlseder J, Broccoli D, Dai Y, Hardy S, de Lange T. p53- and ATM-dependent apoptosis induced by telomeres lacking TRF2. Science 1999; 283:1321 - 5; http://dx.doi.org/10.1126/science.283.5406.1321; PMID: 10037601
- van Steensel B, Smogorzewska A, de Lange T. TRF2 protects human telomeres from end-to-end fusions. Cell 1998; 92:401 - 13; http://dx.doi.org/10.1016/S0092-8674(00)80932-0; PMID: 9476899
- Okamoto K, Bartocci C, Ouzounov I, Diedrich JK, Yates JR 3rd, Denchi EL. A two-step mechanism for TRF2-mediated chromosome-end protection. Nature 2013; 494:502 - 5; http://dx.doi.org/10.1038/nature11873; PMID: 23389450
- Griffith JD, Comeau L, Rosenfield S, Stansel RM, Bianchi A, Moss H, de Lange T. Mammalian telomeres end in a large duplex loop. [see comments] Cell 1999; 97:503 - 14; http://dx.doi.org/10.1016/S0092-8674(00)80760-6; PMID: 10338214
- Amiard S, Doudeau M, Pinte S, Poulet A, Lenain C, Faivre-Moskalenko C, Angelov D, Hug N, Vindigni A, Bouvet P, et al. A topological mechanism for TRF2-enhanced strand invasion. Nat Struct Mol Biol 2007; 14:147 - 54; http://dx.doi.org/10.1038/nsmb1192; PMID: 17220898
- Doksani Y, Wu JY, de Lange T, Zhuang X. Super-resolution fluorescence imaging of telomeres reveals TRF2-dependent T-loop formation. Cell 2013; 155:345 - 56; http://dx.doi.org/10.1016/j.cell.2013.09.048; PMID: 24120135
- Smogorzewska A, van Steensel B, Bianchi A, Oelmann S, Schaefer MR, Schnapp G, de Lange T. Control of human telomere length by TRF1 and TRF2. Mol Cell Biol 2000; 20:1659 - 68; http://dx.doi.org/10.1128/MCB.20.5.1659-1668.2000; PMID: 10669743
- Ancelin K, Brunori M, Bauwens S, Koering CE, Brun C, Ricoul M, Pommier JP, Sabatier L, Gilson E. Targeting assay to study the cis functions of human telomeric proteins: evidence for inhibition of telomerase by TRF1 and for activation of telomere degradation by TRF2. Mol Cell Biol 2002; 22:3474 - 87; http://dx.doi.org/10.1128/MCB.22.10.3474-3487.2002; PMID: 11971978
- Brunori M, Mathieu N, Ricoul M, Bauwens S, Koering CE, Roborel de Climens A, Belleville A, Wang Q, Puisieux I, Décimo D, et al. TRF2 inhibition promotes anchorage-independent growth of telomerase-positive human fibroblasts. Oncogene 2006; 25:990 - 7; http://dx.doi.org/10.1038/sj.onc.1209135; PMID: 16205637
- Takai KK, Hooper S, Blackwood S, Gandhi R, de Lange T. In vivo stoichiometry of shelterin components. J Biol Chem 2010; 285:1457 - 67; http://dx.doi.org/10.1074/jbc.M109.038026; PMID: 19864690
- Karlseder J, Smogorzewska A, de Lange T. Senescence induced by altered telomere state, not telomere loss. Science 2002; 295:2446 - 9; http://dx.doi.org/10.1126/science.1069523; PMID: 11923537
- Stagno D’Alcontres M, Mendez-Bermudez A, Foxon JL, Royle NJ, Salomoni P. Lack of TRF2 in ALT cells causes PML-dependent p53 activation and loss of telomeric DNA. J Cell Biol 2007; 179:855 - 67; http://dx.doi.org/10.1083/jcb.200703020; PMID: 18056407
- Richter T, Saretzki G, Nelson G, Melcher M, Olijslagers S, von Zglinicki T. TRF2 overexpression diminishes repair of telomeric single-strand breaks and accelerates telomere shortening in human fibroblasts. Mech Ageing Dev 2007; 128:340 - 5; http://dx.doi.org/10.1016/j.mad.2007.02.003; PMID: 17395247
- Muñoz P, Blanco R, Flores JM, Blasco MA. XPF nuclease-dependent telomere loss and increased DNA damage in mice overexpressing TRF2 result in premature aging and cancer. Nat Genet 2005; 37:1063 - 71; http://dx.doi.org/10.1038/ng1633; PMID: 16142233
- Wu Y, Mitchell TR, Zhu XD. Human XPF controls TRF2 and telomere length maintenance through distinctive mechanisms. Mech Ageing Dev 2008; 129:602 - 10; http://dx.doi.org/10.1016/j.mad.2008.08.004; PMID: 18812185
- Wang RC, Smogorzewska A, de Lange T. Homologous recombination generates T-loop-sized deletions at human telomeres. Cell 2004; 119:355 - 68; http://dx.doi.org/10.1016/j.cell.2004.10.011; PMID: 15507207
- Wan B, Yin J, Horvath K, Sarkar J, Chen Y, Wu J, Wan K, Lu J, Gu P, Yu EY, et al. SLX4 Assembles a Telomere Maintenance Toolkit by Bridging Multiple Endonucleases with Telomeres. Cell Rep 2013.
- Wilson JS, Tejera AM, Castor D, Toth R, Blasco MA, Rouse J. Localization-dependent and -independent roles of SLX4 in regulating telomeres. Cell Rep 2013; 4:853 - 60; http://dx.doi.org/10.1016/j.celrep.2013.07.033; PMID: 23994477
- Poulet A, Buisson R, Faivre-Moskalenko C, Koelblen M, Amiard S, Montel F, Cuesta-Lopez S, Bornet O, Guerlesquin F, Godet T, et al. TRF2 promotes, remodels and protects telomeric Holliday junctions. EMBO J 2009; 28:641 - 51; http://dx.doi.org/10.1038/emboj.2009.11; PMID: 19197240
- Henning KA, Moskowitz N, Ashlock MA, Liu PP. Humanizing the yeast telomerase template. Proc Natl Acad Sci U S A 1998; 95:5667 - 71; http://dx.doi.org/10.1073/pnas.95.10.5667; PMID: 9576941
- Brevet V, Berthiau AS, Civitelli L, Donini P, Schramke V, Géli V, Ascenzioni F, Gilson E. The number of vertebrate repeats can be regulated at yeast telomeres by Rap1-independent mechanisms. EMBO J 2003; 22:1697 - 706; http://dx.doi.org/10.1093/emboj/cdg155; PMID: 12660175
- Alexander MK, Zakian VA. Rap1p telomere association is not required for mitotic stability of a C(3)TA(2) telomere in yeast. EMBO J 2003; 22:1688 - 96; http://dx.doi.org/10.1093/emboj/cdg154; PMID: 12660174
- Auriche C, Di Domenico EG, Ascenzioni F. Budding yeast with human telomeres: a puzzling structure. Biochimie 2008; 90:108 - 15; http://dx.doi.org/10.1016/j.biochi.2007.09.009; PMID: 17954006
- Stavenhagen JB, Zakian VA. Internal tracts of telomeric DNA act as silencers in Saccharomyces cerevisiae.. Genes Dev 1994; 8:1411 - 22; http://dx.doi.org/10.1101/gad.8.12.1411; PMID: 7926741
- McEachern MJ, Haber JE. Break-induced replication and recombinational telomere elongation in yeast. Annu Rev Biochem 2006; 75:111 - 35; http://dx.doi.org/10.1146/annurev.biochem.74.082803.133234; PMID: 16756487
- Lin CY, Chang HH, Wu KJ, Tseng SF, Lin CC, Lin CP, Teng SC. Extrachromosomal telomeric circles contribute to Rad52-, Rad50-, and polymerase delta-mediated telomere-telomere recombination in Saccharomyces cerevisiae. Eukaryot Cell 2005; 4:327 - 36; http://dx.doi.org/10.1128/EC.4.2.327-336.2005; PMID: 15701795
- Krauskopf A, Blackburn EH. Rap1 protein regulates telomere turnover in yeast. Proc Natl Acad Sci U S A 1998; 95:12486 - 91; http://dx.doi.org/10.1073/pnas.95.21.12486; PMID: 9770512
- Bah A, Bachand F, Clair E, Autexier C, Wellinger RJ. Humanized telomeres and an attempt to express a functional human telomerase in yeast. Nucleic Acids Res 2004; 32:1917 - 27; http://dx.doi.org/10.1093/nar/gkh511; PMID: 15047858
- Bah A, Gilson E, Wellinger RJ. Telomerase is required to protect chromosomes with vertebrate-type T2AG3 3′ ends in Saccharomyces cerevisiae. J Biol Chem 2011; 286:27132 - 8; http://dx.doi.org/10.1074/jbc.M111.220186; PMID: 21676873
- Ira G, Pellicioli A, Balijja A, Wang X, Fiorani S, Carotenuto W, Liberi G, Bressan D, Wan L, Hollingsworth NM, et al. DNA end resection, homologous recombination and DNA damage checkpoint activation require CDK1. Nature 2004; 431:1011 - 7; http://dx.doi.org/10.1038/nature02964; PMID: 15496928
- Di Domenico EG, Mattarocci S, Cimino-Reale G, Parisi P, Cifani N, D’Ambrosio E, Zakian VA, Ascenzioni F. Tel1 and Rad51 are involved in the maintenance of telomeres with capping deficiency. Nucleic Acids Res 2013; 41:6490 - 500; http://dx.doi.org/10.1093/nar/gkt365; PMID: 23677619
- Cesare AJ, Reddel RR. Alternative lengthening of telomeres: models, mechanisms and implications. Nat Rev Genet 2010; 11:319 - 30; http://dx.doi.org/10.1038/nrg2763; PMID: 20351727
- Verdun RE, Karlseder J. The DNA damage machinery and homologous recombination pathway act consecutively to protect human telomeres. Cell 2006; 127:709 - 20; http://dx.doi.org/10.1016/j.cell.2006.09.034; PMID: 17110331
- Sfeir A, Kosiyatrakul ST, Hockemeyer D, MacRae SL, Karlseder J, Schildkraut CL, de Lange T. Mammalian telomeres resemble fragile sites and require TRF1 for efficient replication. Cell 2009; 138:90 - 103; http://dx.doi.org/10.1016/j.cell.2009.06.021; PMID: 19596237
- Martínez P, Thanasoula M, Muñoz P, Liao C, Tejera A, McNees C, Flores JM, Fernández-Capetillo O, Tarsounas M, Blasco MA. Increased telomere fragility and fusions resulting from TRF1 deficiency lead to degenerative pathologies and increased cancer in mice. Genes Dev 2009; 23:2060 - 75; http://dx.doi.org/10.1101/gad.543509; PMID: 19679647
- Bailey SM, Cornforth MN, Kurimasa A, Chen DJ, Goodwin EH. Strand-specific postreplicative processing of mammalian telomeres. Science 2001; 293:2462 - 5; http://dx.doi.org/10.1126/science.1062560; PMID: 11577237
- Cesare AJ, Kaul Z, Cohen SB, Napier CE, Pickett HA, Neumann AA, Reddel RR. Spontaneous occurrence of telomeric DNA damage response in the absence of chromosome fusions. Nat Struct Mol Biol 2009; 16:1244 - 51; http://dx.doi.org/10.1038/nsmb.1725; PMID: 19935685
- Mitchell TR, Glenfield K, Jeyanthan K, Zhu XD. Arginine methylation regulates telomere length and stability. Mol Cell Biol 2009; 29:4918 - 34; http://dx.doi.org/10.1128/MCB.00009-09; PMID: 19596784
- Mitchell TR, Zhu XD. Methylated TRF2 associates with the nuclear matrix and serves as a potential biomarker for cellular senescence. Aging (Albany NY) 2014; 6:248 - 63; PMID: 24721747
- Sheppard SA, Loayza D. LIM-domain proteins TRIP6 and LPP associate with shelterin to mediate telomere protection. Aging (Albany NY) 2010; 2:432 - 44; PMID: 20634563
- Nora GJ, Buncher NA, Opresko PL. Telomeric protein TRF2 protects Holliday junctions with telomeric arms from displacement by the Werner syndrome helicase. Nucleic Acids Res 2010; 38:3984 - 98; http://dx.doi.org/10.1093/nar/gkq144; PMID: 20215438
- Muftuoglu M, Wong HK, Imam SZ, Wilson DM 3rd, Bohr VA, Opresko PL. Telomere repeat binding factor 2 interacts with base excision repair proteins and stimulates DNA synthesis by DNA polymerase beta. Cancer Res 2006; 66:113 - 24; http://dx.doi.org/10.1158/0008-5472.CAN-05-2742; PMID: 16397223
- Saharia A, Guittat L, Crocker S, Lim A, Steffen M, Kulkarni S, Stewart SA. Flap endonuclease 1 contributes to telomere stability. Curr Biol 2008; 18:496 - 500; http://dx.doi.org/10.1016/j.cub.2008.02.071; PMID: 18394896
- Miller AS, Balakrishnan L, Buncher NA, Opresko PL, Bambara RA. Telomere proteins POT1, TRF1 and TRF2 augment long-patch base excision repair in vitro. Cell Cycle 2012; 11:998 - 1007; http://dx.doi.org/10.4161/cc.11.5.19483; PMID: 22336916
- Pickett HA, Cesare AJ, Johnston RL, Neumann AA, Reddel RR. Control of telomere length by a trimming mechanism that involves generation of t-circles. EMBO J 2009; 28:799 - 809; http://dx.doi.org/10.1038/emboj.2009.42; PMID: 19214183
- Poulet A, Pisano S, Faivre-Moskalenko C, Pei B, Tauran Y, Haftek-Terreau Z, Brunet F, Le Bihan YV, Ledu MH, Montel F, et al. The N-terminal domains of TRF1 and TRF2 regulate their ability to condense telomeric DNA. Nucleic Acids Res 2012; 40:2566 - 76; http://dx.doi.org/10.1093/nar/gkr1116; PMID: 22139926
- Leman AR, Dheekollu J, Deng Z, Lee SW, Das MM, Lieberman PM, Noguchi E. Timeless preserves telomere length by promoting efficient DNA replication through human telomeres. Cell Cycle 2012; 11:2337 - 47; http://dx.doi.org/10.4161/cc.20810; PMID: 22672906
- Horikawa I, Fujita K, Harris CC. p53 governs telomere regulation feedback too, via TRF2. Aging (Albany NY) 2011; 3:26 - 32; PMID: 21266744