Abstract
Long non-coding RNAs are estimated to qualitatively represent ~98% of expressed transcripts in human cells, a large proportion of which is antisense to protein-coding and non-coding transcripts. Here we review evidence from several experimental systems that suggests long antisense non-coding RNAs are involved in the transcriptional regulation of gene expression by altering epigenetic states at both adjacent and distal loci. We also review the initial evidence for a role of endogenous long antisense non-coding RNAs in oncogenic cellular transformation.
Several recent studies have demonstrated pervasive antisense transcription in human cells.Citation1–Citation8 Many of these antisense RNAs are unspliced, lack a poly-adenylation signal and are generally more than 200 bp long.Citation2,Citation9 The transcriptional landscape becomes even more complex when intergenic and size-selected RNAs are assessed.Citation10–Citation12 Clearly, much more of the human genome is transcribed than we have previously appreciated. Estimates for human and murine cells suggest that ∼61–72% of all transcribed regions express long non-coding RNAs that are in the antisense orientation relative to adjacent protein-coding genes.Citation5,Citation13 There appears to be little conservation of non-coding RNAs across species.Citation14 Most of these transcripts are not annotated; they might play a role in determining species-specific traits.Citation15 Here we consider evidence that suggests a role of long, antisense noncoding RNAs in the regulation of transcription.
Antisense non-coding RNAs as regulators of gene transcription have emerged from early observations in prokaryotes where antisense RNAs were shown to regulate transcription.Citation16 Several examples of human genes regulated by antisense RNAs had been documented by the mid-1990s.Citation17 In human cells, antisense RNAs were found to suppress the expression of their sense counterparts.Citation18 This mode of regulation was thought to result from secondary structures generated by the sense and antisense RNAs or from a block in translation due to sense-antisense pairing.Citation16 While there were several plausible explanations for the regulation of sense RNAs by their antisense counterparts, there was little mechanistic information supporting these suppositions.Citation16 Data generated over the last year have begun to provide insights into the mechanisms of transcriptional regulation mediated by long antisense non-coding RNAs. These mechanisms involve antisense RNA-directed remodeling of chromatin at target loci.
The importance of such epigenetic changes and their role in the regulation of gene expression has long been recognized.Citation19,Citation20 Transcriptional activation and transcriptional silencing are mediated and accompanied by changes in the chromatin.Citation21 Common transcriptional silencing marks include methylation of genomic DNA at CpG sites and methylation of histones. The principal marks for actively transcribed chromatin are acetylation of histone 3 at specific sites. At gene promoters, these epigenetic changes have profound effects on gene expression, presumably because they affect the ability of RNA polymerase II and of transcription factors to localize to the promoter. Epigenetic marks can be passed on to daughter cells, and a particular epigenetic change can profoundly affect generations of daughter cells.Citation22 However, it is not known how the molecular machinery that induces epigenetic changes is recruited to a specific genetic locus. In plants, small non-coding double stranded RNAs direct DNA methylation to loci showing sequence homology.Citation23 Similar observations have been made in human cells with small interfering RNAs (siRNAs) designed to target gene promoters.Citation24 Small non-coding RNAs, either siRNAs or antisense RNAs, can function in human cells to direct the enzymatic machineries for methylation of histone 3 at lysines 9 and 27 (H3K9 and H3K27) and for methylation of DNA to genomic loci showing sequence homology to the particular non-coding RNA. These activities are correlated with transcriptional gene silencing (TGS) of the targeted locus.Citation25,Citation26 The observations suggest that it is possible to affect gene transcription in a targeted manner. The effector molecules governing such epigenetic control of transcription remained unidentified.
Two key observations with small RNA-directed TGS in human cells shed some light on possible endogenous mechanisms: (i) Only the antisense strand of a promoter-targeted siRNA was required to direct TGS; and (ii) DNA methyltransferase 3a (DNMT3a) was essential for TGS and was localized to the targeted locus.Citation27 These findings were strikingly similar to previous observations showing that, in human cells, long antisense non-coding RNAs direct epigenetic marks to imprinted loci.Citation28,Citation29 Furthermore, X chromosome inactivation in females, which involves Xist and its counterpart antisense RNA, Tsix, requires the localization of DNMT3a to targeted loci.Citation30
The similarities between imprinted genes and TGS suggested a common endogenous pathway, possibly involving long antisense non-coding RNAs in the recruitment of chromatin-modifying machinery.Citation26,Citation27 The role of small non-coding RNAs as mediators of TGS might then be to usurp an endogenous pathway that includes long non-coding RNAs. Recent evidence supports this supposition and suggests that long non-coding RNAs mediate both locus- and allele-specific regulation of non-imprinted genes.Citation31,Citation32
Observations on tumor suppressor genes in human cells have identified long antisense non-coding RNAs as regulators of transcription. Overexpression of the particular non-coding RNA resulted in stable, epigenetic silencing of the sense mRNA counterpart at the promoter,Citation31 whereas the loss of the long antisense non-coding RNA induced a significant increase in the expression of the sense mRNA counterpart and hence gene activation.Citation32 These non-coding RNAs may function by recruiting epigenetic modulatory proteins to their homologous target loci.Citation33 By localizing to the target gene, they can modify the local chromatin architecture in a manner that would affect transcription (). Recent observations also document transcriptional regulation by long antisense non-coding RNAs in S. cerevisiae,Citation34,Citation35 bacteriaCitation36 and plants.Citation37 Thus yeast, bacteria, plants and humans share a siRNA-independent mechanism involving long non-coding RNAs in the control of transcription. These observations imply the widespread occurrence of an RNA-based transcriptional regulation that operates through long non-coding transcripts.
Among cancer-relevant genes, those encoding p21, E-cadherin,Citation32 p15,Citation31 p53,Citation38 Myc,Citation39 epidermal growth factor homology domain-1 (tie-1)Citation40 and PU.1Citation41 have all been shown to be regulated to some extent by long non-coding RNAs. Several of the genes listed in can be epigenetically silenced in cancer.Citation42 It is tempting to speculate that such epigenetic silencing might involve the dysregulated production of long antisense non-coding RNA. This supposition leads to the question of what regulates the expression of long antisense non-coding RNAs. One possible explanation is that microRNAs (miRNAs) control the output of bi-directionally transcribed loci.Citation43 In the case of E-cadherin, the miRNA miR373 was found to bind to the E-cadherin antisense non-coding RNA and to affect the expression of E-cadherin.Citation32 Another possible explanation assigns a regulatory role to tiny RNAs (tiRNAs). These are predominantly sense-oriented and centered just downstream of transcriptional start sitesCitation44 apparently related to the position of the first intragenic nucleosome,Citation45 and could conceivably obstruct or influence gene-specific effects of long antisense non-coding RNAs.
The observations reviewed in this article set the stage for more extensive investigations of the role of antisense non-coding RNAs in the control of gene expression. The abundance of non-coding transcription continues to pose a huge challenge to our understanding of the genome and its regulation.Citation46 Determining the functions of long antisense non-coding RNAs is part of that challenge. Whatever the exact natural mechanisms involved, this system of endogenous RNA-mediated transcriptional regulation could be made subject to control by exogenous small RNAs. With appropriate constructs, targeted to the antisense non-coding RNAs or designed to mimic the antisense non-coding RNAs, it should be possible to either activate or suppress gene expression transcriptionally,Citation26 with enormous practical implications.
Figures and Tables
Figure 1 Model for long antisense non-coding RNA-mediated transcriptional regulation. (A) Long antisense non-coding RNAs are generated at bidirectionally transcribed loci (antisense strand in red, sense strand in black). (B) The long antisense non-coding RNA is assumed to interact with regions of homology in a sense promoter-associated transcript.Citation50 Alternatively, the long antisense non-coding RNA could interact with the plus strand of the unwound DNA. Such interactions are postulated to trigger the recruitment of chromatin-remodeling proteins DNA methyltransferase 3a (DNMT3a), Argonaute 1 (Ago-1), Enhancer of Zeste (Ezh2), Suv39 h1, histone deacetylase 1 (HDAC-1) and G9a. (C) These chromatin-remodeling complexes would induce changes of the local chromatin architecture, including methylation of DNA, specifically at loci targeted by the long antisense non-coding RNAs.
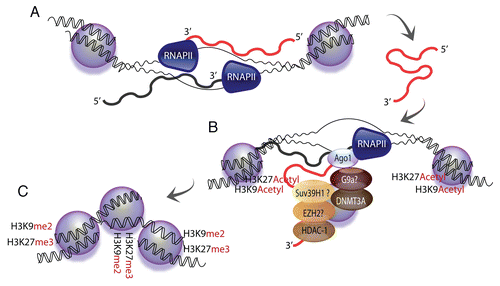
Table 1 Cancer-relevant genes that generate long antisense non-coding RNAsTable Footnote1
Acknowledgements
Work of the authors is supported by National Institutes of Health grants R01 HL083473-02 and RO1AI084406-02 (K.V.M.), R01 CA078230-12 (P.K.V.) and P01 CA078045-10 (P.K.V.). This is manuscript 20611 of The Scripps Research Institute. We thank Art Riggs, John S. Mattick, Jonathan R. Hart and Minghao Sun for valuable discussions and suggestions.
References
- Finocchiaro G, Carro MS, Francois S, Parise P, DiNinni V, Muller H. Localizing hotspots of antisense transcription. Nucleic Acids Res 2007; 35:1488 - 1500
- He Y, Vogelstein B, Velculescu VE, Papadopoulos N, Kinzler KW. The antisense transcriptomes of human cells. Science 2008; 322:1855 - 1857
- Osato N, Suzuki Y, Ikeo K, Gojobori T. Transcriptional interferences in cis natural antisense transcripts of humans and mice. Genetics 2007; 176:1299 - 1306
- Yelin R, Dahary D, Sorek R, Levanon EY, Goldstein O, Shoshan A, et al. Widespread occurrence of antisense transcription in the human genome. Nat Biotechnol 2003; 21:379 - 386
- Katayama S, Tomaru Y, Kasukawa T, Waki K, Nakanishi M, Nakamura M, et al. Antisense transcription in the mammalian transcriptome. Science 2005; 309:1564 - 1566
- Rosok O, Sioud M. Systematic identification of sense-antisense transcripts in mammalian cells. Nat Biotechnol 2004; 22:104 - 108
- Conley AB, Miller WJ, Jordan IK. Human cis natural antisense transcripts initiated by transposable elements. Trends Genet 2008; 24:53 - 56
- Furuno M, Pang KC, Ninomiya N, Fukuda S, Frith MC, Bult C, et al. Clusters of internally primed transcripts reveal novel long noncoding RNAs. PLoS Genet 2006; 2:37
- Kiyosawa H, Mise N, Iwase S, Hayashizaki Y, Abe K. Disclosing hidden transcripts: mouse natural sense-antisense transcripts tend to be poly(A) negative and nuclear localized. Genome Res 2005; 15:463 - 474
- Khalil AM, Guttman M, Huarte M, Garber M, Raj A, Rivea Morales D, et al. Many human large intergenic noncoding RNAs associate with chromatin-modifying complexes and affect gene expression. Proc Natl Acad Sci USA 2009; 106:11667 - 11672
- Kapranov P, Cheng J, Dike S, Nix DA, Duttagupta R, Willingham AT, et al. RNA maps reveal new RNA classes and a possible function for pervasive transcription. Science 2007; 316:1484 - 1488
- Guttman M, Amit I, Garber M, French C, Lin MF, Feldser D, et al. Chromatin signature reveals over a thousand highly conserved large non-coding RNAs in mammals. Nature 2009; 458:223 - 227
- Cheng J, Kapranov P, Drenkow J, Dike S, Brubaker S, Patel S, et al. Transcriptional maps of 10 human chromosomes at 5-nucleotide resolution. Science 2005; 308:1149 - 1154
- Babak T, Blencowe BJ, Hughes TR. A systematic search for new mammalian noncoding RNAs indicates little conserved intergenic transcription. BMC Genomics 2005; 6:104
- Taft RJ, Pheasant M, Mattick JS. The relationship between non-protein-coding DNA and eukaryotic complexity. Bioessays 2007; 29:288 - 299
- Stolt P, Zillig W. Antisense RNA mediates transcriptional processing in an archaebacterium, indicating a novel kind of RNase activity. Mol Microbiol 1993; 7:875 - 882
- Knee R, Murphy PR. Regulation of gene expression by natural antisense RNA transcripts. Neurochem Int 1997; 31:379 - 392
- Izant JG, Weintraub H. Constitutive and conditional suppression of exogenous and endogenous genes by anti-sense RNA. Science 1985; 229:345 - 352
- Tsanev R, Sendov B. An epigenetic mechanism for carcinogenesis. Z Krebsforsch Klin Onkol Cancer Res Clin Oncol 1971; 76:299 - 319
- Egger G, Liang G, Aparicio A, Jones PA. Epigenetics in human disease and prospects for epigenetic therapy. Nature 2004; 429:457 - 463
- Kouzarides T. Chromatin modifications and their function. Cell 2007; 128:693 - 705
- Morris KV. Non-coding RNAs, epigenetic memory and the passage of information to progeny. RNA Biol 2009; 6:242 - 247
- Matzke MA, Primig M, Trnovsky J, Matzke AJ. Reversible methylation and inactivation of marker genes in sequentially transformed tobacco plants. EMBO J 1989; 8:643 - 649
- Morris KV, Chan SW, Jacobsen SE, Looney DJ. Small interfering RNA-induced transcriptional gene silencing in human cells. Science 2004; 305:1289 - 1292
- Hawkins PG, Morris KV. RNA and transcriptional modulation of gene expression. Cell Cycle 2008; 7:602 - 607
- Morris KV. RNA-directed transcriptional gene silencing and activation in human cells. Oligonucleotides 2009; 19:299 - 306
- Weinberg MS, Villeneuve LM, Ehsani A, Amarzguioui M, Aagaard L, Chen ZX, et al. The antisense strand of small interfering RNAs directs histone methylation and transcriptional gene silencing in human cells. RNA 2006; 12:256 - 262
- Latos PA, Barlow DP. Regulation of imprinted expression by macro non-coding RNAs. RNA Biol 2009; 6:100 - 106
- Zaratiegui M, Irvine DV, Martienssen RA. Noncoding RNAs and gene silencing. Cell 2007; 128:763 - 776
- Lee JT. Lessons from X-chromosome inactivation: long ncRNA as guides and tethers to the epigenome. Genes Dev 2009; 23:1831 - 1842
- Yu W, Gius D, Onyango P, Muldoon-Jacobs K, Karp J, Feinberg AP, et al. Epigenetic silencing of tumour suppressor gene p15 by its antisense RNA. Nature 2008; 451:202 - 206
- Morris KV, Santoso S, Turner AM, Pastori C, Hawkins PG. Bidirectional transcription directs both transcriptional gene activation and suppression in human cells. PLoS Genet 2008; 4:1000258
- Morris KV. Long antisense non-coding RNAs function to direct epigenetic complexes that regulate transcription in human cells. Epigenetics 2009; 4:296 - 301
- Camblong J, Iglesias N, Fickentscher C, Dieppois G, Stutz F. Antisense RNA stabilization induces transcriptional gene silencing via histone deacetylation in S. cerevisiae. Cell 2007; 131:706 - 717
- Camblong J, Beyrouthy N, Guffanti E, Schlaepfer G, Steinmetz LM, Stutz F. Trans-acting antisense RNAs mediate transcriptional gene cosuppression in S. cerevisiae. Genes Dev 2009; 23:1534 - 1545
- Guell M, van Noort V, Yus E, Chen WH, Leigh-Bell J, Michalodimitrakis K, et al. Transcriptome complexity in a genome-reduced bacterium. Science 2009; 326:1268 - 1271
- Swiezewski S, Liu F, Magusin A, Dean C. Cold-induced silencing by long antisense transcripts of an Arabidopsis Polycomb target. Nature 2009; 462:799 - 802
- Mahmoudi S, Henriksson S, Corcoran M, Mendez-Vidal C, Wiman KG, Farnebo M. Wrap53, a natural p53 antisense transcript required for p53 induction upon DNA damage. Mol Cell 2009; 33:462 - 471
- Celano P, Berchtold CM, Kizer DL, Weeraratna A, Nelkin BD, Baylin SB, et al. Characterization of an endogenous RNA transcript with homology to the antisense strand of the human c-myc gene. J Biol Chem 1992; 267:15092 - 15096
- Li K, Blum Y, Verma A, Liu Z, Pramanik K, Leigh NR, et al. A noncoding antisense RNA in tie-1 locus regulates tie-1 function in vivo. Blood 2010; 115:133 - 139
- Ebralidze AK, Guibal FC, Steidl U, Zhang P, Lee S, Bartholdy B, et al. PU.1 expression is modulated by the balance of functional sense and antisense RNAs regulated by a shared cis-regulatory element. Genes Dev 2008; 22:2085 - 2092
- Cortez CC, Jones PA. Chromatin, cancer and drug therapies. Mutat Res 2008; 647:44 - 51
- Khraiwesh B, Arif MA, Seumel GI, Ossowski S, Weigel D, Reski R, et al. Transcriptional control of gene expression by microRNAs. Cell 2010; 140:111 - 122
- Taft RJ, Glazov EA, Cloonan N, Simons C, Stephen S, Faulkner GJ, et al. Tiny RNAs associated with transcription start sites in animals. Nat Genet 2009; 41:572 - 578
- Taft RJ, Kaplan CD, Simons C, Mattick JS. Evolution, biogenesis and function of promoter-associated RNAs. Cell Cycle 2009; 8:2332 - 2338
- Tomkins GM, Gelehrter TD, Granner D, Martin DJr, Samuels HH, Thompson EB. Control of specific gene expression in higher organisms. Expression of mammalian genes may be controlled by repressors acting on the translation of messenger RNA. Science 1969; 166:1474 - 1480
- Chiba M, Kiyosawa H, Hiraiwa N, Ohkohchi N, Yasue H. Existence of Pink1 antisense RNAs in mouse and their localization. Cytogenet Genome Res 2009; 126:259 - 270
- Chiba M, Kubo M, Miura T, Sato T, Rezaeian AH, Kiyosawa H, et al. Localization of sense and antisense transcripts of Prdx2 gene in mouse tissues. Cytogenet Genome Res 2008; 121:222 - 231
- Spicer DB, Sonenshein GE. An antisense promoter of the murine c-myc gene is localized within intron 2. Mol Cell Biol 1992; 12:1324 - 1329
- Han J, Kim D, Morris KV. Promoter-associated RNA is required for RNA-directed transcriptional gene silencing in human cells. Proc Natl Acad Sci USA 2007; 104:12422 - 12427