Abstract
RNA polymerase III transcribes small untranslated RNAs that fulfill essential cellular functions in regulating transcription, RNA processing, translation and protein translocation. RNA polymerase III transcription activity is tightly regulated during the cell cycle and coupled to growth control mechanisms. Furthermore, there are reports of changes in RNA polymerase III transcription activity during cellular differentiation, including the discovery of a novel isoform of human RNA polymerase III that has been shown to be specifically expressed in undifferentiated human H1 embryonic stem cells. Here, we review major regulatory mechanisms of RNA polymerase III transcription during the cell cycle, cell growth and cell differentiation.
Eukaryotes contain three distinct DNA-dependent RNA polymerases (Pol I, Pol II and Pol III)Citation1 that share the task of transcribing the information contained in genes into mobile RNA entities. In humans, Pol I transcribes the precursor of the large ribosomal 45S RNA, Pol II transcribes all messenger RNAs, most snRNAs, snoRNAs and micro RNAs and Pol III transcribes a diverse group of small untranslated RNAs that participate in the regulation of transcription, splicing and translation. After transcription, Pol III transcripts are either directly degraded or modified for participation in the regulation and execution of processes in the nucleus and cytoplasm (transcription regulation; RNA processing; ribosome assembly; translation) that ultimately lead to protein synthesis. In the past few years, in part due to the discovery of novel classes of regulatory RNAs such as micro (mi) RNAs and small interfering (si)RNAs, it has become clear that the three classical eukaryotic RNA polymerases have acquired additional layers of complexity during evolution from unicellular to multicellular eukaryotes. For instance, derivatives of Pol II that fulfill specific functions in transcription of siRNAs (Pol IV) or of noncoding RNAs at target loci (Pol V) have been found in Arabidopsis.Citation2,Citation3 More recently, an isoform of human Pol III has been described that is specifically expressed in embryonic stem cells and in certain tumor cell lines. This isoform of Pol III is able to contribute to cell transformation under tissue culture conditions.Citation4 In this review, we will describe our current understanding of how Pol III transcription is regulated during growth and differentiation, with emphasis on the mammalian Pol III system where appropriate.
Introduction to Pol III Transcription
Promoters.
Three types of promoters are recognized by RNA polymerase III in higher eukaryotes (). Type 1 and type 2 promoters are internal to the gene, whereas type 3 promoters are located entirely upstream of the transcription initiation site.Citation5 Type 1 (5S ribosomal RNA gene) and type 2 promoters [e.g., those in tRNA, VA1 RNA and VA2 RNA genes and in short interspersed nuclear elements (SINEs)] are composed of A- and C- or A- and B-boxes, respectively (). Transcription of type 1 and 2 genes is initiated about 8 to 50 nucleotides upstream of the 5′-end of Box A and terminates as soon as Pol III encounters four or more consecutive thymidines. Type 3 promoters (U6 RNA, 7SK RNA, RNAse P RNA, RNAse MRP RNA and Y RNA genes) are comprised of a distal sequence element (DSE) that is typically located about 200–250 nt upstream of the transcription initiation site, a proximal sequence element (PSE) at around −50 and a TATA box at −30. Type 3 promoters arose during evolution from unicellular to multicellular eukaryotes and are found in plants and animals, but not in yeast, which instead utilize a type 2 promoter for transcription of the U6 gene. Pol III-transcribed genes that contain both gene-internal and gene-external promoter elements have also been described and include the 7SL RNA, vault RNA, BC1 RNA, BC200 RNA, EBER1 RNA and EBER2 RNA genes.Citation5
Transcription factors.
The yeast and human transcription factors that recognize these promoters have been identified by genetic or biochemical means and their cognate subunits have been cloned (). The primary DNA-binding transcription factors that are required for the recognition of the evolutionary conserved type 1 and type 2 promoters have likewise been conserved from yeast to human. Type 2 genes are directly recognized by the six subunit transcription factor TFIIIC.Citation6,Citation7 The type 1 promoter of the 5S gene requires prior binding of TFIIIA, the prototype gene-specific transcriptional activator in eukaryotes,Citation8 for recruitment of TFIIIC to the gene.Citation6,Citation9 The type 3 promoters of U6 and 7SK genes appeared during the evolution of multicellular eukaryotes, along with transcription factors required for their expression. Type 3 promoters are directly bound by the multisubunit PSE-binding transcription factor (PTF; also referred to as SNAPc or PBP),Citation10–Citation12 for which no ortholog has been identified in yeast. The interaction of PTF/SNAPc/PBP with the PSE is reinforced by DSE binding proteins such as Oct-1,Citation13–Citation16 and Staf (ZNF143).Citation17 For transcription activation of type 3 promoter-containing genes, DSE and PSE sequences may be juxtaposed by a positioned nucleosome.Citation18,Citation19
In the model of a stepwise assembly of preinitiation complexes (PIC) on Pol III promoters, the DNA-binding proteins, once bound to their promoters, recruit the initiation factor TFIIIB to their respective genes. After its recruitment to the promoter, TFIIIB alone is able to direct multiple rounds of transcription in the absence of TFIIIC and TFIIIA,Citation20 demonstrating that interactions of Pol III with TFIIIC and TFIIIA are not essential for transcription initiation, at least in vitro, but rather serve for the recruitment of TFIIIB. Only a single isoform of TFIIIB has been described in yeast.Citation6 In contrast, human cells contain two isoforms of TFIIIB (TFIIIB-α and TFIIIB-β)Citation21 that are specifically recruited either to gene-internal type 1 and 2 (TFIIIB-β) promoters or to type 3 promoters (TFIIIB-α). As for the evolution of PTF/SNAPc/PBP, the appearance of a second isoform of TFIIIB may again have been necessary for allowing promoter type- or gene-specific regulation of Pol III transcription in higher eukaryotes. Human TFIIIB-α and TFIIIB-β both contain TATA-binding protein (TBP) and BDP1 but differ with respect to the presence of the TFIIB-related factors BRF1 (TFIIIB-β) and BRF2 (TFIIIB-α).Citation22,Citation23 Interestingly, it was demonstrated that purified S. cerevisiae BDP1 could replace its human ortholog in transcription of the human U6 gene reconstituted with human transcription factors, indicating that essential protein-protein-interactions with other components of the PIC at type 3 promoters could be established by S. cerevisiae BDP1.Citation24 An alternative model to the stepwise recruitment of the Pol III transcription machinery to promoters has been suggested by the discovery of an RNA polymerase III holoenzyme that contains TFIIIC and TFIIIB-β subunits in addition to Pol III.Citation25 This holoenzyme as an entity may directly recognize Pol III promoters and direct multiple rounds of transcription by mechanisms involving facilitated reinitiation of transcription.Citation26,Citation27
Accessory factors.
A variety of proteins have been described that, while not necessarily essential, are highly stimulatory for Pol III transcription in vitro. Complementation assays coupled with purification of factors from HeLa nuclear extracts showed that topoisomerase 1 and positive cofactor 4 (PC4) co-fractionate with TFIIIC (holo-TFIIIC) and that they activate Pol III transcription in a system reconstituted with highly purified components.Citation28 Similarly, NF1 was found to stimulate Pol III transcription from type 2 promoters and to extend the TFIIIC footprint over the transcription termination site.Citation29 A function similar to that of human PC4 was recently demonstrated for the S. cerevisiae ortholog Sub1.Citation30,Citation31 Although the mode of action for PC4 or Sub1 in Pol III transcription has remained a puzzle owing to the high (50–100 fold) molar excess of PC4 (relative to other Pol III transcription factors) required for in vitro transcription activation, it has been speculated that DNA-binding properties of PC4 may help to structure DNA for facilitating Pol III transcription in vitro. Furthermore, the transcription termination and recycling factor LaCitation32 co-purified with holo-TFIIIC preparations.Citation28 Although not absolutely required for in vitro transcription,Citation33 La was shown to associate with Pol III promoters in yeastCitation34 and human cells.Citation35 Moreover, the HMG-box-containing proteins NHP6A and NHP6B have been implicated in the activation of Pol III transcription in S. cerevisiae.Citation36,Citation37 Up to now, there has been no identification of a mammalian NHP6A or NHP6B ortholog that might fulfill a similar function Pol III transcription.
RNA polymerase III.
Along with the DNA-binding transcription factors, the distinct isoforms of human TFIIIB are necessary and sufficient to recruit Pol III to the transcription initiation site. Pol III is highly conserved from yeast to humans and is composed of 17 subunits (). Of these subunits, five (RPB5/POLR2E, RPB6/POLR2F, RPB8/POLR2H, RPB10/POLR2L and RPB12/POLR2K) are common to all three polymerases, two (RPAC40/POLR1C and RPAC19/POLR1D) are shared by Pol I and Pol III (with paralogous subunits in Pol II) and five (RPC1/POLR3A, RPC2/POLR3B, RPC8/POLR3H, RPC9 and RPC11/POLR3K) are paralogous to subunits found in Pol I and Pol II. The remaining five subunits (RPC5/POLR3E, RPC4/BN51/POLR3D, RPC3/RPC62/POLR3C, RPC6/RPC39/POLR3F and RPC7/RPC32/POLR3G) are specific to RNA polymerase III and no structural or functional counterparts have been identified in Pol I or Pol II.Citation38,Citation39 However, it has been suggested that some of these subunits may share structural homologies to basal transcription factors of the Pol II system.Citation40,Citation41
Three of the five Pol III-specific subunits (POLR3C, POLR3F, POLR3G) form a stable subcomplex (). Thus, it was shown in the yeast S. cerevisiae (Sc) that the ScRPC31, ScRPC34 and ScRPC82 subunits (orthologous to human (Hs) RPC32, HsRPC39 and HsRPC62, respectively) could be separated from the other subunits of Pol III by native gel electrophoresis.Citation42 In addition, a mutation in the zinc-binding domain of the largest subunit of Pol III (ScRPC160) led to an increased dissociation of subunits ScRPC31, ScRPC34 and ScRPC82 from the remainder of the enzyme,Citation43 indicating that ScRPC31, ScRPC34 and ScRPC82 may form a Pol III sub-complex that is less stably associated with the residual enzyme. The association of this sub-complex with the rest of Pol III may involve a direct ScRPC160-ScRPC31-interaction, since overexpression of ScRPC160 is able to suppress a conditional growth phenotype of a mutation in the C-terminal part of ScRPC31.Citation44 It was further shown that ScRPC31, ScRPC34 and ScRPC82 interact with each other in two hybrid assays.Citation45 The latter and subsequent experiments also revealed an interaction between ScRPC34 and ScBRF1.Citation45,Citation46 Altogether these results indicate that this sub-complex serves as a bridge between the enzymatic core of Pol III and its initiation factors. As a consequence, in pre-initiation complex formation, this subcomplex likely acts in a central position close to the transcription initiation site. Such a role was also supported by cross-linking experiments that projected ScRPC34 and ScRPC31 upstream of, and ScRPC82 also downstream of, the transcription initiation site.Citation47 By using the short distance crosslinker 4-S-dTMP, Bartholomew and colleaguesCitation47 further showed that photoaffinity labeling of ScRPC82 and ScRPC31 by 4-S-dTMP, but not by the 9 Å-crosslinker N3RdUMP, occurs in transcription bubbles of initiation complexes and stalled elongation complexes, indicating that these two subunits of Pol III may be in contact with single-stranded DNA.
Complementary data supporting a model in which HsRPC62, HsRPC39 and HsRPC32 likewise form a ternary complex were obtained with human Pol III. It was shown that the ternary complex can be removed from the other 14 subunits of Pol III by ultracentrifugation or by treatment with mild denaturants (2 M urea).Citation48 Human Pol III that was stripped of HsRPC32, HsRPC39 and HsRPC62 was capable of transcription elongation (following non-specific initiation), but was incapable of specifically initiating transcription from Pol III promoters, again indicating that this ternary sub-complex may be especially important for polymerase-transcription factor interactions during initiation. However, the residual elongation activity of the 14-subunit core Pol III complex, while significant, was nonetheless reduced, suggesting that the ternary complex may also function during transcription elongation.Citation48 Studies with recombinant proteins further showed that HsTFIIIC63 and HsRPC62, HsTFIIIC90 and HsRPC62 as well as HsTFIIIC90 and HsRPC39 interact in vitro,Citation49,Citation50 reinforcing the hypothesis that the ternary sub-complex of RNA polymerase III fulfils important functions for establishing protein-protein-contacts with transcription factors on the one hand and with Pol III on the other.
Purification of Pol III from mouse myeloma cells (MOPC 315) led to the identification of two chromatographically distinct enzymes (Pol IIIA and Pol IIIB) that were both active in transcription of class III genes.Citation51 Determination of the subunit compositions of these enzymes revealed that they are highly related and differ only in the presence of either a 32 kDa (IIIA) or a 33 kDa (IIIB) polypeptide.Citation52 Recently, we have been able to isolate two distinct isoforms of Pol III from human cells. The major physical difference in between these two isozymes is the presence of either HsRPC32α/POLR3G in Pol IIIα or of HsRPC32β/POLR3GL in Pol IIIβ.Citation4 Taking into account the migration of these two subunits on SDS-PAGE, it is very likely that the two isoforms isolated from human cells are orthologous to the previously reported isoforms of Pol III in mouse myeloma cells.
Regulation of Pol III Transcription—Lessons Learned from Yeast
Many factors and mechanisms that underlie the regulation of RNA polymerase III transcription have been identified by studying yeast cells. These unicellular organisms adapt their gene expression programs by all three RNA polymerases to environmental conditions. Favorable growth conditions lead to higher rates of transcription, whereas deprivation in nutrients results in the repression of transcription. Importantly, transcription of components of the translational apparatus, including rRNAs, tRNAs and mRNAs encoding ribosomal proteins consume about 75–80% of cellular nucleotides, mainly through transcription by Pols I and III,Citation53,Citation54 and this high energetic cost is restricted under unfavorable growth conditions.Citation55 Multiple pathways have been implicated in the regulation of Pol III transcription in S. cerevisiae. These include the secretory signaling pathway,Citation54 the TOR pathway,Citation56–Citation58 the DNA damage pathwayCitation59 and the PKA pathway (as a downstream effector of RAS).Citation60 Defects in the secretory pathway lead to the repression of 5S RNA and tRNA transcription. Temperature-sensitive mutations in two genes that function either in endoplasmic reticulum (ER) to Golgi complex transport (YPT6) or in protein and vesicle trafficking between ER and Golgi (SLY1) lead to repression of Pol III transcription at the non-permissive temperature. This repression can be partially rescued by deletion of protein kinase C (PKC1), the central effector kinase of the cell integrity pathway. Furthermore, deletions of either WSC1 or WSC2 genes, the farthest upstream components of the cell integrity pathway, also strongly reduce repression of Pol III transcription that is induced by secretory mutations.Citation54,Citation55 Deletion of MAF1 blocks the repression of Pol III transcription caused by defects in the secretory pathway,Citation59 possibly placing MAF1 as a downstream effector of PKC. Likewise, MAF1 was identified as a downstream effector of the repression of Pol III transcription by TOR,Citation61 suggesting that many, if not all, repressive signals for Pol III transcription in yeast converge on MAF1.
MAF1 was originally identified in a genetic screen that decreased the nonsense suppressor efficiency of SUP11 tRNACitation62 and the link to Pol III transcription was established by the isolation of fragments of the largest subunit of Pol III as multisuppressor copies of the conditional growth phenotype of the maf1-1 mutation.Citation63,Citation64 MAF1 was shown to inhibit S. cerevisiae Pol III transcription as a downstream effector of several nutrition and stress signaling pathways. Starvation, rapamycin treatment and oxidative or endoplasmic reticulum stress were shown to be dependent on MAF1 for the repression of Pol III transcription.Citation61 Under repressive growth conditions, MAF1 is dephosphorylated by protein phosphatase 2A (PP2A), leading to its translocation into the nucleus where it inhibits Pol III transcription mainly through interactions with BRF1 and Pol III itself.Citation65,Citation66 Favorable growth conditions lead to phosphorylation of MAF1 by SCH9 and PKA kinases, resulting in its retention in the cytoplasm.Citation60,Citation67–Citation69 Repression of Pol III transcription by MAF1, MAF1 regulation by phosphorylation and MAF1 interactions with BRF1 and Pol III have been conserved from yeast to humans.Citation70–Citation72 It was demonstrated that facilitated recycling of Pol III transcription prevents repression by MAF1 in HeLa cell extracts, suggesting that the regulation of Pol III recruitment to preinitiation complexes represents an important mechanistic component of MAF1 function.Citation27 Interestingly, overexpression of MAF1 in human glioblastoma cells led to the inhibition of colony formation in soft agar assays, thus demonstrating functional aspects that are usually attributed to tumor suppressor proteins.Citation73 Possibly, direct inhibition of TATA-binding protein expression and its effects on Pol I and Pol III transcription may contribute to tumor-suppressive activities of MAF1.Citation73 However, no MAF1 mutation causative for the development of tumors has hitherto been described in higher eukaryotes.
Regulation of Pol III Transcription in Higher Eukaryotes
Gene-specific cellular factor TFIIIA.
The prototypical example of Pol III-mediated transcriptional regulation in vertebrates is the regulation of 5S RNA transcription by TFIIIA during Xenopus laevis development. TFIIIA was the first eukaryotic transcription factor to be identified and purified to homogeneity,Citation8 allowing molecular cloning of the cognate cDNA encoding TFIIIACitation74 and deduction of zinc-finger motifs.Citation75 It was shown that TFIIIA regulates 5S RNA transcription through site-specific binding to the 5S gene promoterCitation8 and that it also binds to 5S RNA, forming a 7S ribonucleoprotein storage particle.Citation76 Two types of 5S RNA genes have been described in X. laevis, an oocyte-specific gene that is present in about 20,000 copies per haploid genome and a somatic gene present in about 400 copies per haploid genome.Citation77 The somatic 5S gene is expressed in both oocytes and in adult animals, whereas expression of the oocyte-specific 5S genes decreases dramatically after oogenesis. The reduction of oocyte 5S gene transcription has been attributed to multiple mechanisms involving both chromatin modificationsCitation78,Citation79 and regulation of the expression of TFIIIA itself.Citation74,Citation77,Citation80 Regarding the latter mechanism, there are high levels of TFIIIA mRNA and protein per cell during early oogenesis but dramatically reduced levels per cell during embryogenesis. Thus, the expression pattern of X. laevis TFIIIA suggests a regulatory role for TFIIIA in the developmental changes in 5S gene transcription. Differential expression of TFIIIA in oocyte and somatic cells has been attributed to the usage of distinct promoters. Interestingly, it has been reported that Pol III initiates transcription within the oocyte-specific promoter of TFIIIA, suggesting a Pol III-mediated downregulation of the oocyte-specific TFIIIA promoter in Xenopus laevis somatic cells.Citation81
Viral proteins.
In the late seventies and the eighties of the last millennium, after the discovery and partial characterization of general transcription initiation factors for Pol III,Citation82 Pol II,Citation83 and Pol I,Citation84 the focus of transcription research advanced to the identification of mechanisms that contribute to the regulation of the three transcription systems. The contribution of viral proteins in the process of transcription regulation was explored and, in particular, viral proteins with oncogenic functions were analyzed for their ability to enhance or repress transcription. We will restrict the description in this review to the regulation of mammalian Pol III transcription and refer to excellent publications that review the regulation of RNA polymerases I and II.Citation85–Citation87 Major breakthrough discoveries with respect to the regulation of Pol III transcription by viral proteins were obtained with the adenovirus E1A protein that activates transcription of immediate early viral genes. Transfection of cDNAs encoding E1A stimulated transcription of co-transfected VA1 and tRNA genes.Citation88–Citation90 The activation was attributed to indirect mechanisms that acted on the activity of TFIIIC, since depletion of E1A from HEK cell extracts did not abolish transcription activation.Citation91 The molecular cloning of TFIIIC110Citation92 showed that its expression could be regulated by transfection of adenoviral E1A or by serum stimulation of HeLa cells. However, it was also shown that recombinant E1A that was produced in baculoviral expression systems could likewise stimulate Pol III transcription,Citation93 pointing to the possibility that E1A may exert its function through alternative mechanisms. In addition to the regulation of Pol III transcription by E1A, it was demonstrated that the SV40 small t antigen also stimulates Pol III transcription.Citation94 The hepatitis B virus X (Hep B X) protein also was shown to activate transcription by Pol III.Citation95 It later was shown that the Hep B X protein acts, at least in part, through enhanced expression of the TATA-binding protein.Citation96
Link to cell cycle control.
Important mechanistic aspects of Pol III transcription regulation by viral proteins were revealed by the identification of cellular proteins that are regulated by these viral proteins. In particular, it was demonstrated that adenoviral E1A and E1B proteins, papillomaviral E6 and E7 proteins and SV40 large T antigen interacted with and inactivated p53 and pRb, thereby severely impacting on the regulation of the host cell's cell cycle.Citation97,Citation98 These findings led subsequently to the discoveries that major tumor suppressor proteins, such as pRb,Citation99,Citation100 p53,Citation101,Citation102 ARF,Citation103 PTENCitation104 and BRCA1,Citation105 negatively regulate Pol III transcription. For Rb it was demonstrated that only hypophosphorylated Rb was able to efficiently repress Pol III transcription.Citation99 The regulation of Pol III transcription by tumor suppressor proteins was shown to be exerted either through direct interactions with TFIIIBCitation102,Citation106 or PTF/SNAPc/PBPCitation107 subunits or by the activation of signal transduction cascades that ultimately lead to the phosphorylation and inactivation of TFIIIB subunits.Citation104 However, indirect effects of p53 on the stability of BRF1 have also been described. In this case, it was necessary to maintain ectopic expression of p53 in p53-/- Li-Fraumeni cells for at least 4 days in order to reveal an inhibitory effect on Pol III transcription activity; and this effect proved to be mediated by the disappearance of a hyperphosphorylated form of BRF1, which was degraded in a proteasome-dependent manner.Citation108 Moreover, pRb family members p107 and p130 were also reported to repress Pol III transcription through direct interaction with BRF1.Citation109,Citation110
It was further reported that Pol III transcription is repressed in mitosis and that the repression is conferred by a kinase that phosphorylates subunits of TFIIIB. In X. laevis it was shown that a 92 kDa protein in fractions with TFIIIB activity becomes phophorylated by p34cdc2 (CDK1).Citation111,Citation112 In HeLa cells, TBP-associated subunits of TFIIIB (presumably corresponding to BRF1 or BRF1 and BDP1) that were purified from cycling cells were shown to reconstitute transcription in mitotic extracts, suggesting that these components represent the target proteins of repression.Citation113 Subsequently, it was shown that BRF1 is phosphorylated and inactivated during mitosis by a kinase different from CDK1.Citation114 In addition, BDP1 within TFIIIB-α was shown to be phosphorylated by CK2 during mitosis, leading to the repression of Pol III transcription.Citation115 Apart from this inhibitory role of CK2, it was also reported that CK2 is able to stimulate transcription of the human U6 gene via phosphorylation of Pol III.Citation116 Regulatory roles for CK2 in Pol III transcription were also reported in the yeast S. cerevisiae.Citation117,Citation118 The phosphorylation of BRF1 and of BDP1 during mitosis has been confirmed by quantitative analysis of protein phosphorylation in HeLa cells.Citation119 This report also showed that subunits of Pol III (POLR3C, POLR3E and POLR3G), TFIIIB-α (BRF2), TFIIIC (TFIIIC220, TFIIIC110) and PTF (PTFα/SNAPc190) are likewise phosphorylated during mitosis, which could possibly contribute to mitotic repression of Pol III transcription in human cells. Repression of PTFα/SNAPc190 activity was shown to be mediated by CK2,Citation120 but it remains to be shown whether CK2 or another kinase is responsible for PTFα/SNAPc190 phosphorylation during mitosis.
Link to growth control.
In contrast to the repression exerted by tumor suppressor proteins, the proto-oncogene c-MYCCitation121 and components of the MAP kinase signal transduction pathway (ERK; JNK1)Citation122,Citation123 were found to activate Pol III transcription. c-Myc, ERK and JNK1, respectively, were shown to function through recruitment of TFIIIB to Pol III promoters (c-Myc), through phosphorylation of the BRF1 subunit of TFIIIB (ERK) or through enhanced expression of BRF1 (JNK1). Subsequently, it was shown that c-Myc induces the association of GCN5 and TRRAP with Pol III promoters (tRNALeu; 5S), resulting in acetylation of histone H3.Citation124 The association of c-Myc with TRRAP and GCN5, as well as Pol III transcription activation is negatively regulated upon nucleolar stress (induced by actinomycin D or serum starvation) by the ribosomal protein L11.Citation125 Myc functions in regulating Pol III transcription were demonstrated to be independent of its heterodimerization partner Max in Drosophila melanogaster.Citation126 Moreover, in an independent link of Myc to the Pol III transcription apparatus, it was shown that c-Myc activates transcription of the RPC53 (BN51) gene,Citation127 which encodes a Pol III subunit that has been reported to suppress the temperature-sensitive G1 cell cycle arrest in the hamster BHK-21 cell line.Citation128,Citation129 Interestingly, mutation of the gene encoding RPC53 in yeast also induced cell cycle arrest predominantly in G1, although no Myc ortholog has been described in this organism.Citation130 Collectively, these results strongly indicate that the regulation of growth and cell cycle control are intimately connected to the activity of Pol III transcription. Some of the regulatory mechanisms have been summarized in and .
Link to tumor development.
The above described discoveries that linked Pol III transcription to cellular processes such as cell cycle or growth suggested that Pol III transcription may be deregulated during cell transformation. In addition it was shown that the expression of five TFIIIC subunits (the sixth was not yet identified) is increased in ovarian carcinomas.Citation131 Importantly, the samples analyzed in this study were obtained from patients in clinics and not from cell lines grown under tissue culture conditions, demonstrating that the expression of Pol III transcription components is deregulated in tumors that have not been produced by experimental manipulations. It was nevertheless a surprise that the inducible overexpression of the BRF1 subunit of TFIIIB or of the tRNAiMet gene in mouse 3T3 fibroblasts was sufficient for transformation of these cells.Citation132 These data suggested that enhanced transcription from type 3 promoters was not required for tumor formation, but that only transcription by BRF1-containing TFIIIB-β, and in particular that of the tRNAi-Met gene, was crucial in this process. However, overexpression of BRF1 or tRNAiMet in rat1a fibroblasts proved insufficient for cellular transformation, indicating that cell type-specific differences may exist between these two model systems.Citation133 The analysis in rat1a cells has further shown that enhanced Pol III transcription is required for oncogenic transformation by c-Myc.Citation133 In summary, and even if the model of BRF1- or tRNAiMet-mediated cell transformation may not be applicable to all types of cells, these two studies clearly demonstrate the importance of Pol III transcription for cell transformation.
Regulation of Pol III Transcription during Cellular Differentiation
In addition to the mechanisms that coordinate the regulation of growth and Pol III transcription activity, multicellular organisms must have evolved the means to regulate transcription during differentiation in order to ensure that cells with high protein synthesis rates are capable of producing the components necessary for translation (i.e., ribosomal RNAs and proteins, as well as tRNAs)—even if they are resting and thus in the G0 phase of the cell cycle. Some tissues such as the exocrineCitation134 or endocrineCitation135 pancreas probably circumvent this problem in part by balancing cell growth and apoptosis, resulting in stable cell numbers whilst allowing cell growth and division. In these tissues, the required protein synthesis and thus active Pol III transcription, may presumably occur predominantly in dividing cells, although the presence of high amounts of endoplasmic reticulum suggests high protein synthesis rates in both dividing and resting cells. Other cells, including Schwann cells that induce myelin gene transcription after cell cycle exit,Citation136 require transcription of ribosomal components after entering the G0 phase of the cell cycle.
Only one study addresses the regulation of Pol III transcription in cells that have entered the G0 phase of the cell cycle. The model system for this study is the hypertrophic growth of cardiomyocytes that is induced by serum, endothelin-1 or phenylephrine. Even if these cells are in the G0 phase of the cell cycle, they are still able to increase cell mass (hypertrophy) upon serum stimulation and to a lesser extent by endothelin-1 or phenylephrine stimulation. Induction of hypertrophy is accompanied by enhanced BRF1 and c-Myc expression and by elevated rates of phosphorylated ERK, as well as phosphorylated Rb.Citation137 As a result, Pol III transcription is enhanced in cardiomyocytes by these stimuli. Except for this particular case, there is no detailed information on how mammalian Pol III transcription is regulated during differentiation and in terminally differentiated cells and tissues.
Little is known about the regulation of Pol III transcription during differentiation of mammalian cells. In particular, analyses of Pol III transcription at the level of protein expression and/or modification during early steps of differentiation (e.g., in undifferentiated embryonic stem cells and in the course of their differentiation) are limited by the amounts of cells and extracts that can be derived from these cells. To overcome these limits, embryonic carcinoma (EC) cells have been employed for analyzing the regulation of Pol III transcription during early steps of differentiation. Mouse F9 or human NTERA2 EC cells are tumor cell lines with certain stem cell-like features.Citation138 Suspension cultures of F9 EC cells can be differentiated into embryoid bodies by the addition of retinoic acid and monolayer cultures of F9 cells differentiate into parietal entoderm (PE) upon the addition of retinoic acid and cAMP (). This cell system has been employed for analyzing the activity of the Pol III transcription system during differentiation. It was shown that Pol III activity decreased for transcription of gene-internal promoters as well as of type 3 promoters. The reduced transcriptional activity at geneinternal promoters was attributed to altered TFIIIB activityCitation139 or to a decrease in TFIIIC1 activity.Citation140 The decrease in TFIIIB activity was shown to result from the combined reduction of the abundance of TFIIIB subunits (TBP, BRF1 and BDP1).Citation141,Citation142 Furthermore, the protein levels of c-MYC were also dramatically downregulated during differentiation of EC into PE cells, which may account in parts for the reduction of Pol III transcription.Citation142 Impaired transcription of type 3 genes was correlated with a decrease in binding of PTF/SNAPc/PBP to the PSECitation143 ().
These results, indicating that TFIIIB-, TFIIIC1- and PTF/SNAPs/PBP-activities are regulated during differentiation of F9 EC cells could be functionally linked to each other by the finding that the TFIIIB subunit BDP1 is essential for TFIIIC1-activity.Citation144 Originally, TFIIIC was separated by B-Box-based affinity purification or by chromatography over Mono Q into the TFIIIC1 and DNA-binding TFIIIC2 components, which were both required for Pol III transcription.Citation145,Citation146 TFIIIC1 was shown to stimulate binding of the six-subunit TFIIIC2 complex to gene-internal type 2 promoters,Citation145 as well as PTF/SNAPc/PBP binding to the PSE.Citation147 Extensive purification of TFIIIC1 in conjunction with analyses of recombinant TFIIIB150 (corresponding to amino acids 1–846 of BDP1) revealed that BDP1 and TFIIIC1 were functionally replaceable in complementation assays of transcription with extracts that were derived from differentiated F9 cells (and thus contained limiting amounts of TFIIIC1) or in transcription systems that were reconstituted with partially purified transcription factors.Citation144 Together, these data indicate that the activity of BDP1 is negatively regulated during differentiation of F9 teratocarcinoma cells.
The importance of Pol III transcription for proliferation and differentiation and thus for organ development, was further demonstrated in Danio rerio. Expression of a splice mutant of the second largest subunit of Pol III (POLR3B Δ239–279; slim jim) in zebrafish led to disruption of the development of digestive organs and to size reductions in the exocrine pancreas, liver, retina and terminal branchial arches. Although the strongest expression of POLR3B was reported in the nervous system 24 hours post fertilization, no neuronal phenotype other than that of the retina was reported for the slim jim mutant.Citation148 These data suggest that normal Pol III transcription rates may be crucial for the development of rapidly growing cells. In support of this notion, it was proposed that tissue-specific expression of tRNAs may be coordinated with the codon usage of individual cells, which may in turn be crucial for tissue or cell type-specific translation regulation.Citation149
Recent data indicate that expression of a subunit of Pol III itself is also subject to regulation during the process of differentiation. It was shown that RPC32α mRNA expression is negatively regulated during differentiation of human H1 embryonic stem cells and that RPC32α mRNA could not be detected in RNA blots from differentiated tissues (). In addition, RPC32α mRNA and protein expression were found to increase during transformation of human embryonic lung IMR90 fibroblasts by defined genetic elements.Citation4 These data collectively suggested that RPC32α mRNA and protein expression is negatively regulated during differentiation and upregulated during the malignant transformation of human cells. Furthermore, by employing siRNAs that specifically suppress RPC32α-expression, it was demonstrated that RPC32α is important for anchorage-independent growth of a HeLa S3 carcinoma cell line. Most importantly, overexpression of RPC32α in IMR90 human embryonic lung fibroblasts with altered functions of p53 and pRb (through the expression of papillomaviral E6 and E7 proteins) led to full transformation and immortalization of these cells. Altogether, these data indicated that the changes in expression of RPC32α were not only coincident with differentiation or cell transformation, but that RPC32α may play an active role in these processes.Citation4
In view of these results it is relevant to ask why studies that examined Pol III transcription during the differentiation of F9 teratocarcinoma cells did not identify the stem cell-specific isoform of RNA polymerase III. A possible explanation is that the genes analyzed in these studies were the well-known Pol III genes (transfer-, 5S-, U6- or VA1-RNA genes), that can be transcribed in vitro either by Pol IIIα or by Pol IIIβ.Citation4 As a consequence, the expression of these genes presumably does not change during the process of stem cell differentiation. If this supposition holds true, the downregulation of RPC32α during differentiation may affect the transcription of genes other than the 5S and tRNA genes—and these hypothetical yet-to-be-identified genes may turn out to be important for maintaining cells in an undifferentiated state. The possible existence of such Pol IIIα-specific genes also implies that the phenotypes observed in the zebrafish (which according to BLAST analyses contains orthologs of RPC32α and RPC32β) expressing the slim jim mutant may, at least in part, be attributable to a dysfunction of Pol IIIα. Thus, the identification of genes that are specifically transcribed by Pol IIIα and that cannot be transcribed by Pol IIIβ will be important for understanding the roles of Pol IIIα and Pol IIIβ in growth and development. A first step towards the identification of such genes that may fulfil important functions in growth and differentiation has been made by establishing a genome-wide map of RNA polymerase III DNA interactions.
The Human Pol III Transcriptome
Recently, the results from five independent genome-wide chromatin immunoprecipitation (ChIP) sequencing projects in human cells have been published.Citation150–Citation154 Antibodies against several distinct subunits of Pol III (RPC155/POLR3A; RPC53/POLR3D; RPC32/POLR3G) and its transcription factors (BRF1; BRF2; BDP1; TFIIIC220; TFIIIC110; TFIIIC63; PTFδ/SNAPc2/SNAP45) were employed for determining the genes that are in contact with these proteins and presumably transcribed in a variety of untransformed, immortalized or transformed cell lines (IMR90 fibroblasts/TERT; human foreskin fibroblasts/TERT; Jurkat; HEK; HeLa; K562; CD4+ cells). As expected, at least one copy of each multicopy gene (individual tRNA, 5S, 7SL and U6 genes) is associated with Pol III and BRF1 (TFIIIB-β) or BRF2 (TFIIIB-α).Citation151–Citation154 Single-copy Pol III-transcribed genes include the 7SK, RNAse MRP, RNAse P, BC200, U6atac and vault-1, -2 and -3 genes). Of these, the 7SK, U6atac, RNAse MRP, RNAse P and vault genes were reported to be associated with Pol III in each of the studies. BC200 was crosslinked to Pol III and identified in the ChIP-seq (K562 cells)Citation152 and microarray (HeLa cells)Citation153 analyses, but was not identified by the other studies. The differential detection of the BC200 gene in individual studies may be attributable to distinct cut-off values that were used to distinguish positive and negative results and/or a cell type-specific expression pattern of the BC200 gene.Citation155 A greater discrepancy between the different studies and distinct cell types was observed with respect to the occupancy of tRNA genes by Pol III and/or subunits of TFIIIC and TFIIIB. This variation may be attributable to the copy number of individual tRNA genes and cell type-specific nuclear organization of the genome. In general, the ChIP-sequencing results do not support earlier reportsCitation156,Citation157 indicating that micro RNAs may be transcribed by Pol III. In fact, the only miRNA loci that were found to be associated with Pol III are hsa-mir565 (a tRNA fragment),Citation150 hsa-mir-1975 (overlaps with the hy5 gene)Citation151,Citation152 and hsa-mir-886 (overlaps with a vault gene).Citation151–Citation154 Thus, all these micro RNA genes overlap genes that possess classical Pol III promoters. One single microRNA gene (has-mir-498) has been described to associate with Pol III in CD4+ cells but not in HeLa cells.Citation150 The number of novel Pol III genes is difficult to assess, although tens to hundreds of candidate genes, depending on the study, have been identified. These genes include SINEs, snaR loci, sn/snoRNA loci and unannotated genes.
Interestingly, two of the five studies employed antibodies directed against RPC32α for ChIPs.Citation153,Citation154 Some of the genes identified in these studies may represent Pol IIIα-specific genes, although the specificity of the antibodies for Pol IIIα versus Pol IIIβ in immunoprecipitation assays remains to be proven. At present, it cannot be excluded that the genomic loci identified in these studies also contain Pol IIIβ-transcribed genes, such that Pol IIIα-specific genes remain unequivocally to be determined.
Pol III, Chromatin and Higher Order Genome Organization
Chromatin modifications found near Pol III-bound genes share features with the histone modifications found at actively transcribed Pol II genes, including histone H3K4me3 or H3K9Ac.Citation150,Citation152,Citation153 However, other positively acting histone modifications described at Pol II transcribed genes, namely the RPB1 CTD-dependent, Set2-mediated H3K36me3 or H3K79me2 were absent at transcribed Pol III genes. Furthermore, H3K27me3 was rarely found at actively transcribed Pol III promoters.Citation150
In addition to the discovery of novel genes that may be transcribed by Pol III, two of the publications also described sites within the genome that are occupied by TFIIIC, but not by Pol III (extra TFIIIC [ETC] loci).Citation152,Citation153 These sites are often found in close vicinity to genes transcribed by Pol II and may represent boundary or insulator elements, similar to what was reported in yeast.Citation158 TFIIIC may bind to ETCs via a novel DNA recognition sequenceCitation152 and might also directly recruit histone modifying factors.Citation159 CCCTC-binding factor (CTFC) also was detected at these ETC sitesCitation152 and at a subset of tDNAs with the highest enrichment of Pol III.Citation153 The association of the cohesin-interacting protein CTCF with TFIIIC-bound ETCs or tDNA genes is reminiscent of a situation described in yeast wherein tDNAs and Pol III-associated proteins have been reported to contribute to the establishment of sister chromatid cohesion at the HMR locus.Citation160
c-Myc was crosslinked close to Pol III promoters, in support of a role for c-Myc in activating Pol III transcription.Citation154 Surprisingly, in addition to associations with Pol III transcription factors, c-Myc and Pol III itself, many of the transcribed Pol III genes were also in close proximity to Pol II transcription activators such as Fos and JunCitation154 or Ets1 and STAT1.Citation153 Moreover, basal Pol II transcription factors and the cyclin T1 subunit of elongation factor PTEFb could be crosslinked close to sites occupied by Pol III.Citation150 Most importantly, Pol II was similarly enriched at Pol III-transcribed genes,Citation151–Citation154 often upstream of the transcription initiation site and sometimes attributable to the presence of Pol II promoters within a distance of 2 kb.Citation153 Notably, in about two-thirds of the cases these Pol II promoters directed transcription by Pol II in the opposite direction of that of Pol III. The cooccupation of these sites by Pol II and Pol III has been interpreted as being advantageous for Pol III-mediated transcription as a result of Pol II-dependent chromatin modifications. However, this assumption has not been experimentally validated and alternative scenarios can be envisaged. In favor of direct interactions of Pol III components with chromatin-modifying enzymes, it has been shown that Pol III transcription of a chromatin template is facilitated by a direct TFIIIC-mediated recruitment of the histone acetyl transferase p300,Citation159 which could contribute to the histone acetylationCitation161 observed at these promoters in cells.
In addition to local chromatin modifications that are important for Pol III transcription, tRNA-induced and condensinmediated organization of chromatin domains results in the recruitment of tRNA genes to the nucleolus in S. cerevisiae.Citation162,Citation163 This could result in the co-recruitment of Pol II genes to these sites and placement of the tRNA genes into a chromatin context that may be favorable for their expression or repression. Moreover, perinucleolar domains (PNC) have been shown to form in tumor cells in a Pol III-dependent mannerCitation164 and may represent sites that are favorable for gene expression. With respect to PNCs, it remains to be established whether Pol IIIα contributes to the formation of these subcellular structures. If so, the ability of Pol IIIα to contribute to cell transformation may be attributable to repositioning Pol II genes involved in cell transformation or tumor suppression into actively transcribed or repressed domains, respectively. In summary, the genome-wide determination of Pol III-DNA associations provides important information about the genes that are in contact with Pol III in several distinct cell lines and a closer analysis of these genes may reveal the ones that contribute to essential functions of Pol III in cell growth and differentiation.
Conclusion
Research in recent years has contributed to an improved understanding of how RNA polymerase III-mediated transcription is regulated in yeast and mammals. There has been considerable progress with respect to an understanding of the molecular mechanisms that link cell growth and cell cycle control to Pol III transcription in lower and higher eukaryotes. This understanding has also allowed an appreciation of the importance of Pol III transcription for cell transformation and for sustaining tumor growth. Recent data have linked Pol III-mediated transcription to the differentiation of EC and of ES cells, indicating that the transcription of small untranslated RNAs by Pol III is essential not only for the regulation of growth, but also for the regulation of differentiation. Future research will provide a more detailed understanding of how Pol III contributes to these processes, including the specific functions of the two Pol III isoforms.
Figures and Tables
Figure 1 Mammalian RNA Polymerase III promoters and transcription factors. Promoter types 1–3. III A = TFIII A. TFIII B subunits are TBP, BDP1, BRF1 and BRF2; TFIIIC subunits are indicated as C35, C63, C90, C102, C110 and C220. PTFα, β, γ and δ subunits are also denoted according to the molecular weight of the respective SNAPc subunits (190, 50, 43 and 45). In addition, SNAP19 is shown, which has not been described in PTF. The ternary complexes composed of RPC39, RPC62 and RPC32α or RPC32β are high lightened within the symbolic representation of RNA polymerase III.
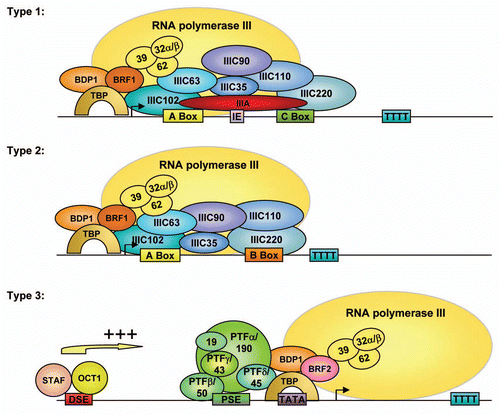
Figure 2 (A) Schematic representation of several regulatory pathways of mammalian RNA polymerase III transcription: Ras-dependent pathways. (B) Schematic representation of several regulatory pathways of mammalian RNA polymerase III transcription: Regulation of Pol III transcription by tumor suppressor and oncogene proteins.
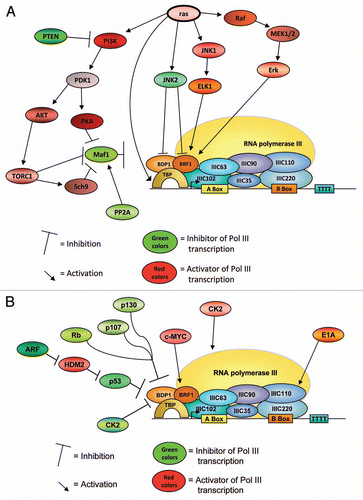
Figure 3 Regulation of RNA polymerase III transcription during differentiation of mouse F9 embryonic carcinoma cells.
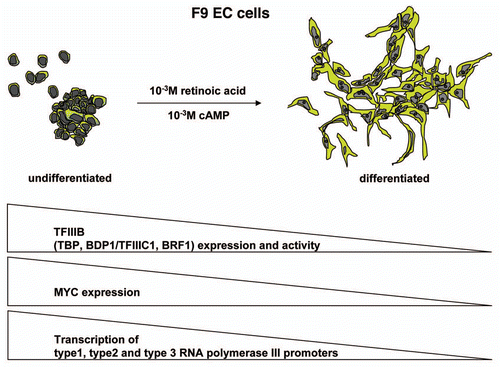
Figure 4 Regulation of RNA polymerase III transcription during differentiation of human H1 embryonic stem cells.
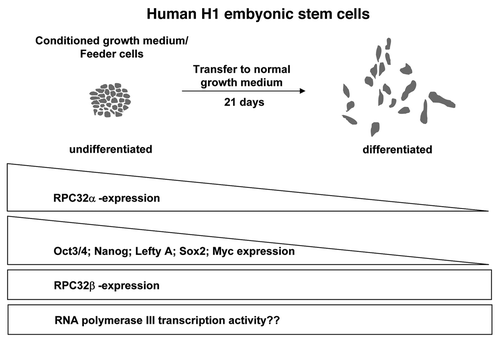
Table 1 The subunits of human RNA polymerase III
Acknowledgements
We thank Giorgio Dieci for critically reading the manuscript. This work has been supported by grants from the Conseil Régional d'Aquitaine and the European Regional Development Fund (to M.T.), from the Agence Nationale de la Recherche (ANR) “REGPOLSTRESS” (to M.T.) and from the Ligue Contre le Cancer-Comites Gironde and Dordogne (to M.T). R.G.R. was supported by grants (CA113872, DK071900 and CA113872) from the National Institutes of Health.
References
- Roeder RG, Rutter WJ. Multiple forms of DNA-dependent RNA polymerase in eukaryotic organisms. Nature 1969; 224:234 - 237
- Ream TS, Haag JR, Wierzbicki AT, Nicora CD, Norbeck AD, Zhu JK, et al. Subunit compositions of the RNA-silencing enzymes Pol IV and Pol V reveal their origins as specialized forms of RNA polymerase II. Mol Cell 2009; 33:192 - 203
- Wierzbicki AT, Haag JR, Pikaard CS. Noncoding transcription by RNA polymerase Pol IVb/Pol V mediates transcriptional silencing of overlapping and adjacent genes. Cell 2008; 135:635 - 648
- Haurie V, Durrieu-Gaillard S, Dumay-Odelot H, Da Silva D, Rey C, Prochazkova M, et al. Two isoforms of human RNA polymerase III with specific functions in cell growth and transformation. Proc Natl Acad Sci USA 2010; 107:4176 - 4181
- Dieci G, Fiorino G, Castelnuovo M, Teichmann M, Pagano A. The expanding RNA polymerase III transcriptome. Trends Genet 2007; 23:614 - 622
- Huang Y, Maraia RJ. Comparison of the RNA polymerase III transcription machinery in Schizosaccharomyces pombe, Saccharomyces cerevisiae and human. Nucleic Acids Res 2001; 29:2675 - 2690
- Dumay-Odelot H, Marck C, Durrieu-Gaillard S, Lefebvre O, Jourdain S, Prochazkova M, et al. Identification, molecular cloning and characterization of the sixth subunit of human transcription factor TFIIIC. J Biol Chem 2007; 282:17179 - 17189
- Engelke DR, Ng SY, Shastry BS, Roeder RG. Specific interaction of a purified transcription factor with an internal control region of 5S RNA genes. Cell 1980; 19:717 - 728
- Geiduschek EP, Kassavetis GA. The RNA polymerase III transcription apparatus. J Mol Biol 2001; 310:1 - 26
- Waldschmidt R, Wanandi I, Seifart KH. Identification of transcription factors required for the expression of mammalian U6 genes in vitro. EMBO J 1991; 10:2595 - 2603
- Murphy S, Yoon JB, Gerster T, Roeder RG. Oct-1 and Oct-2 potentiate functional interactions of a transcription factor with the proximal sequence element of small nuclear RNA genes. Mol Cell Biol 1992; 12:3247 - 3261
- Sadowski CL, Henry RW, Lobo SM, Hernandez N. Targeting TBP to a non-TATA box cis-regulatory element: a TBP-containing complex activates transcription from snRNA promoters through the PSE. Genes Dev 1993; 7:1535 - 1548
- Carbon P, Murgo S, Ebel JP, Krol A, Tebb G, Mattaj LW. A common octamer motif binding protein is involved in the transcription of U6 snRNA by RNA polymerase III and U2 snRNA by RNA polymerase II. Cell 1987; 51:71 - 79
- Bark C, Weller P, Zabielski J, Janson L, Pettersson U. A distant enhancer element is required for polymerase III transcription of a U6 RNA gene. Nature 1987; 328:356 - 359
- Murphy S, Pierani A, Scheidereit C, Melli M, Roeder RG. Purified octamer binding transcription factors stimulate RNA polymerase III—mediated transcription of the 7SK RNA gene. Cell 1989; 59:1071 - 1080
- Tanaka M, Lai JS, Herr W. Promoter-selective activation domains in Oct-1 and Oct-2 direct differential activation of an snRNA and mRNA promoter. Cell 1992; 68:755 - 767
- Schaub M, Myslinski E, Schuster C, Krol A, Carbon P. Staf, a promiscuous activator for enhanced transcription by RNA polymerases II and III. EMBO J 1997; 16:173 - 181
- Stünkel W, Kober I, Seifart KH. A nucleosome positioned in the distal promoter region activates transcription of the human U6 gene. Mol Cell Biol 1997; 17:4397 - 4405
- Zhao X, Pendergrast PS, Hernandez N. A positioned nucleosome on the human U6 promoter allows recruitment of SNAPc by the Oct-1 POU domain. Mol Cell 2001; 7:539 - 549
- Kassavetis GA, Braun BR, Nguyen LH, Geiduschek EP. S. cerevisiae TFIIIB is the transcription initiation factor proper of RNA polymerase III, while TFIIIA and TFIIIC are assembly factors. Cell 1990; 60:235 - 245
- Teichmann M, Seifart KH. Physical separation of two different forms of human TFIIIB active in the transcription of the U6 or the VAI gene in vitro. EMBO J 1995; 14:5974 - 5983
- Schramm L, Pendergrast PS, Sun Y, Hernandez N. Different human TFIIIB activities direct RNA polymerase III transcription from TATA-containing and TATA-less promoters. Genes Dev 2000; 14:2650 - 2663
- Teichmann M, Wang Z, Roeder RG. A stable complex of a novel transcription factor IIB- related factor, human TFIIIB50 and associated proteins mediate selective transcription by RNA polymerase III of genes with upstream promoter elements. Proc Natl Acad Sci USA 2000; 97:14200 - 14205
- Teichmann M, Dieci G, Huet J, Rüth J, Sentenac A, Seifart KH. Functional interchangeability of TFIIIB components from yeast and human cells in vitro. EMBO J 1997; 16:4708 - 4716
- Wang Z, Luo T, Roeder RG. Identification of an autonomously initiating RNA polymerase III holoenzyme containing a novel factor that is selectively inactivated during protein synthesis inhibition. Genes Dev 1997; 11:2371 - 2382
- Dieci G, Sentenac A. Facilitated recycling pathway for RNA polymerase III. Cell 1996; 84:245 - 252
- Cabart P, Lee J, Willis IM. Facilitated recycling protects human RNA polymerase III from repression by Maf1 in vitro. J Biol Chem 2008; 283:36108 - 36117
- Wang Z, Roeder RG. DNA topoisomerase I and PC4 can interact with human TFIIIC to promote both accurate termination and transcription reinitiation by RNA polymerase III. Mol Cell 1998; 1:749 - 757
- Wang Z, Bai L, Hsieh YJ, Roeder RG. Nuclear factor 1 (NF1) affects accurate termination and multiple-round transcription by human RNA polymerase III. EMBO J 2000; 19:6823 - 6832
- Tavenet A, Suleau A, Dubreuil G, Ferrari R, Ducrot C, Michaut M, et al. Genome-wide location analysis reveals a role for Sub1 in RNA polymerase III transcription. Proc Natl Acad Sci USA 2009; 106:14265 - 14270
- Rosonina E, Willis IM, Manley JL. Sub1 functions in osmoregulation and in transcription by both RNA polymerases II and III. Mol Cell Biol 2009; 29:2308 - 2321
- Maraia RJ. Transcription termination factor La is also an initiation factor for RNA polymerase III. Proc Natl Acad Sci USA 1996; 93:3383 - 3387
- Weser S, Bachmann M, Seifart KH, Meissner W. Transcription efficiency of human polymerase III genes in vitro does not depend on the RNP-forming autoantigen La. Nucleic Acids Res 2000; 28:3935 - 3942
- French SL, Osheim YN, Schneider DA, Sikes ML, Fernandez CF, Copela LA, et al. Visual analysis of the yeast 5S rRNA gene transcriptome: regulation and role of La protein. Mol Cell Biol 2008; 28:4576 - 4587
- Fairley JA, Kantidakis T, Kenneth NS, Intine RV, Maraia RJ, White RJ. Human La is found at RNA polymerase III-transcribed genes in vivo. Proc Natl Acad Sci USA 2005; 102:18350 - 18355
- Kruppa M, Moir RD, Kolodrubetz D, Willis IM. Nhp6, an HMG1 protein, functions in SNR6 transcription by RNA polymerase III in S. cerevisiae. Mol Cell 2001; 7:309 - 318
- Stillman DJ. Nhp6: a small but powerful effector of chromatin structure in Saccharomyces cerevisiae. Biochim Biophys Acta 2010; 1799:175 - 180
- Chédin S, Ferri ML, Peyroche G, Andrau JC, Jourdain S, Lefebvre O, et al. The yeast RNA polymerase III transcription machinery: a paradigm for eukaryotic gene activation. Cold Spring Harb Symp Quant Biol 1998; 63:381 - 389
- Hu P, Wu S, Sun Y, Yuan CC, Kobayashi R, Myers MP, et al. Characterization of human RNA polymerase III identifies orthologues for Saccharomyces cerevisiae RNA polymerase III subunits. Mol Cell Biol 2002; 22:8044 - 8055
- Kassavetis GA, Prakash P, Shim E. The C53/C37 subcomplex of RNA polymerase III lies near the active site and participates in promoter opening. J Biol Chem 2010; 285:2695 - 2706
- Carter R, Drouin G. The increase in the number of subunits in eukaryotic RNA polymerase III relative to RNA polymerase II is due to the permanent recruitment of general transcription factors. Mol Biol Evol 2010; 27:1035 - 1043
- Valenzuela P, Hager GL, Weinberg F, Rutter WJ. Molecular structure of yeast RNA polymerase III: demonstration of the tripartite transcriptive system in lower eukaryotes. Proc Natl Acad Sci USA 1976; 73:1024 - 1028
- Werner M, Hermann-Le Denmat S, Treich I, Sentenac A, Thuriaux P. Effect of mutations in a zinc-binding domain of yeast RNA polymerase C (III) on enzyme function and subunit association. Mol Cell Biol 1992; 12:1087 - 1095
- Thuillier V, Stettler S, Sentenac A, Thuriaux P, Werner M. A mutation in the C31 subunit of Saccharomyces cerevisiae RNA polymerase III affects transcription initiation. EMBO J 1995; 14:351 - 359
- Werner M, Chaussivert N, Willis IM, Sentenac A. Interaction between a complex of RNA polymerase III subunits and the 70 kDa component of transcription factor IIIB. J Biol Chem 1993; 268:20721 - 20724
- Brun I, Sentenac A, Werner M. Dual role of the C34 subunit of RNA polymerase III in transcription initiation. EMBO J 1997; 16:5730 - 5741
- Bartholomew B, Braun BR, Kassavetis GA, Geiduschek EP. Probing close DNA contacts of RNA polymerase III transcription complexes with the photoactive nucleoside 4-thiodeoxythymidine. J Biol Chem 1994; 269:18090 - 18095
- Wang Z, Roeder RG. Three human RNA polymerase III-specific subunits form a subcomplex with a selective function in specific transcription initiation. Genes Dev 1997; 11:1315 - 1326
- Hsieh YJ, Kundu TK, Wang Z, Kovelman R, Roeder RG. The TFIIIC90 subunit of TFIIIC interacts with multiple components of the RNA polymerase III machinery and contains a histone-specific acetyltransferase activity. Mol Cell Biol 1999; 19:7697 - 7704
- Hsieh YJ, Wang Z, Kovelman R, Roeder RG. Cloning and characterization of two evolutionarily conserved subunits (TFIIIC102 and TFIIIC63) of human TFIIIC and their involvement in functional interactions with TFIIIB and RNA polymerase III. Mol Cell Biol 1999; 19:4944 - 4952
- Schwartz LB, Sklar VE, Jaehning JA, Weinmann R, Roeder RG. Isolation and partial characterization of the multiple forms of deoxyribonucleic acid-dependent ribonucleic acid polymerase in the mouse myeloma, MOPC 315. J Biol Chem 1974; 249:5889 - 5897
- Sklar VE, Roeder RG. Purification and subunit structure of deoxyribonucleic acid-dependent ribonucleic acid polymerase III from the mouse plasmacytoma, MOPC 315. J Biol Chem 1976; 251:1064 - 1073
- Paule MR, White RJ. Survey and summary: transcription by RNA polymerases I and III. Nucleic Acids Res 2000; 28:1283 - 1298
- Li Y, Moir RD, Sethy-Coraci IK, Warner JR, Willis IM. Repression of ribosome and tRNA synthesis in secretion-defective cells is signaled by a novel branch of the cell integrity pathway. Mol Cell Biol 2000; 20:3843 - 3851
- Willis IM, Desai N, Upadhya R. Signaling repression of transcription by RNA polymerase III in yeast. Prog Nucleic Acid Res Mol Biol 2004; 77:323 - 353
- Zaragoza D, Ghavidel A, Heitman J, Schultz MC. Rapamycin induces the G0 program of transcriptional repression in yeast by interfering with the TOR signaling pathway. Mol Cell Biol 1998; 18:4463 - 4470
- Wei Y, Zheng XF. Sch9 partially mediates TORC1 signaling to control ribosomal RNA synthesis. Cell Cycle 2009; 8:4085 - 4090
- Tsang CK, Liu H, Zheng XF. mTOR binds to the promoters of RNA polymerase I- and III-transcribed genes. Cell Cycle 2010; 9:953 - 957
- Upadhya R, Lee J, Willis IM. Maf1 is an essential mediator of diverse signals that repress RNA polymerase III transcription. Mol Cell 2002; 10:1489 - 1494
- Moir RD, Lee J, Haeusler RA, Desai N, Engelke DR, Willis IM. Protein kinase A regulates RNA polymerase III transcription through the nuclear localization of Maf1. Proc Natl Acad Sci USA 2006; 103:15044 - 15049
- Ciesla M, Boguta M. Regulation of RNA polymerase III transcription by Maf1 protein. Acta Biochim Pol 2008; 55:215 - 225
- Murawski M, Szczesniak B, Zoładek T, Hopper AK, Martin NC, Boguta M. maf1 mutation alters the subcellular localization of the Mod5 protein in yeast. Acta Biochim Pol 1994; 41:441 - 448
- Boguta M, Czerska K, Zoładek T. Mutation in a new gene MAF1 affects tRNA suppressor efficiency in Saccharomyces cerevisiae. Gene 1997; 185:291 - 296
- Pluta K, Lefebvre O, Martin NC, Smagowicz WJ, Stanford DR, Ellis SR, et al. Maf1p, a negative effector of RNA polymerase III in Saccharomyces cerevisiae. Mol Cell Biol 2001; 21:5031 - 5040
- Oficjalska-Pham D, Harismendy O, Smagowicz WJ, Gonzalez de Peredo A, Boguta M, Sentenac A, Lefebvre O. General repression of RNA polymerase III transcription is triggered by protein phosphatase type 2A-mediated dephosphorylation of Maf1. Mol Cell 2006; 22:623 - 632
- Roberts DN, Wilson B, Huff JT, Stewart AJ, Cairns BR. Dephosphorylation and genome-wide association of Maf1 with Pol III-transcribed genes during repression. Mol Cell 2006; 22:633 - 644
- Willis IM, Moir RD. Integration of nutritional and stress signaling pathways by Maf1. Trends Biochem Sci 2007; 32:51 - 53
- Lee J, Moir RD, Willis IM. Regulation of RNA polymerase III transcription involves SCH9-dependent and SCH9-independent branches of the target of rapamycin (TOR) pathway. J Biol Chem 2009; 284:12604 - 12608
- Wei Y, Tsang CK, Zheng XF. Mechanisms of regulation of RNA polymerase III-dependent transcription by TORC1. EMBO J 2009; 28:2220 - 2230
- Reina JH, Azzouz TN, Hernandez N. Maf1, a new player in the regulation of human NA polymerase III transcription. PLoS ONE 2006; 1:134
- Rollins J, Veras I, Cabarcas S, Willis I, Schramm L. Human Maf1 negatively regulates NA polymerase III transcription via the TFIIB family members Brf1 and Brf2. Int J Biol Sci 2007; 3:292 - 302
- Goodfellow SJ, Graham EL, Kantidakis T, Marshall L, Coppins BA, Oficjalska-Pham D, et al. Regulation of RNA polymerase III transcription by Maf1 in mammalian cells. J Mol Biol 2008; 378:481 - 491
- Johnson SS, Zhang C, Fromm J, Willis IM, Johnson DL. Mammalian Maf1 is a negative regulator of transcription by all three nuclear RNA polymerases. Mol Cell 2007; 26:367 - 379
- Ginsberg AM, King BO, Roeder RG. Xenopus 5S gene transcription factor, TFIIIA: characterization of a cDNA clone and measurement of RNA levels throughout development. Cell 1984; 39:479 - 489
- Miller J, McLachlan AD, Klug A. Repetitive zinc-binding domains in the protein transcription factor IIIA from Xenopus oocytes. EMBO J 1985; 4:1609 - 1614
- Honda BM, Roeder RG. Association of a 5S gene transcription factor with 5S RNA and altered levels of the factor during cell differentiation. Cell 1980; 22:119 - 126
- Shastry BS, Honda BM, Roeder RG. Altered levels of a 5 S gene-specific transcription factor (TFIIIA) during oogenesis and embryonic development of Xenopus laevis. J Biol Chem 1984; 259:11373 - 11382
- Wolffe AP, Brown DD. Developmental regulation of two 5S ribosomal RNA genes. Science 1988; 241:1626 - 1632
- Wolffe AP. Dominant and specific repression of Xenopus oocyte 5S RNA genes and satellite I DNA by histone H1. EMBO J 1989; 8:527 - 537
- Taylor W, Jackson IJ, Siegel N, Kumar A, Brown DD. The developmental expression of the gene for TFIIIA in Xenopus laevis. Nucleic Acids Res 1986; 14:6185 - 6195
- Martinez E, Lagna G, Roeder RG. Overlapping transcription by RNA polymerases II and III of the Xenopus TFIIIA gene in somatic cells. J Biol Chem 1994; 269:25692 - 25698
- Segall J, Matsui T, Roeder RG. Multiple factors are required for the accurate transcription of purified genes by RNA polymerase III. J Biol Chem 1980; 255:11986 - 11991
- Matsui T, Segall J, Weil PA, Roeder RG. Multiple factors required for accurate initiation of transcription by purified RNA polymerase II. J Biol Chem 1980; 255:11992 - 11996
- Grummt I, Roth E, Paule MR. Ribosomal RNA transcription in vitro is species specific. Nature 1982; 296:173 - 174
- Roeder RG. Lasker Basic Medical Research Award. The eukaryotic transcriptional machinery: complexities and mechanisms unforeseen. Nat Med 2003; 9:1239 - 1244
- Juven-Gershon T, Kadonaga JT. Regulation of gene expression via the core promoter and the basal transcriptional machinery. Dev Biol 2010; 339:225 - 229
- Drygin D, Rice WG, Grummt I. The RNA polymerase I transcription machinery: an emerging target for the treatment of cancer. Annu Rev Pharmacol Toxicol 2010; 50:131 - 156
- Berger SL, Folk WR. Differential activation of RNA polymerase III-transcribed genes by the polyomavirus enhancer and the adenovirus E1A gene products. Nucleic Acids Res 1985; 13:1413 - 1428
- Hoeffler WK, Roeder RG. Enhancement of RNA polymerase III transcription by the E1A gene product of adenovirus. Cell 1985; 41:955 - 963
- Gaynor RB, Feldman LT, Berk AJ. Transcription of class III genes activated by viral immediate early proteins. Science 1985; 230:447 - 450
- Yoshinaga S, Dean N, Han M, Berk AJ. Adenovirus stimulation of transcription by RNA polymerase III: evidence for an E1A-dependent increase in transcription factor IIIC concentration. EMBO J 1986; 5:343 - 354
- Sinn E, Wang Z, Kovelman R, Roeder RG. Cloning and characterization of a TFIIIC2 subunit (TFIIICbeta) whose presence correlates with activation of RNA polymerase III-mediated transcription by adenovirus E1A expression and serum factors. Genes Dev 1995; 9:675 - 685
- Patel G, Jones NC. Activation in vitro of RNA polymerase II and III directed transcription by baculovirus produced E1A protein. Nucleic Acids Res 1990; 18:2909 - 2915
- Loeken M, Bikel I, Livingston DM, Brady J. Transactivation of RNA polymerase II and III promoters by SV40 small t antigen. Cell 1988; 55:1171 - 1177
- Aufiero B, Schneider RJ. The hepatitis B virus X-gene product trans-activates both RNA polymerase II and III promoters. EMBO J 1990; 9:497 - 504
- Wang HD, Yuh CH, Dang CV, Johnson DL. The hepatitis B virus X protein increases the cellular level of TATA-binding protein, which mediates transactivation of RNA polymerase III genes. Mol Cell Biol 1995; 15:6720 - 6728
- Levine AJ. The common mechanisms of transformation by the small DNA tumor viruses: The inactivation of tumor suppressor gene products: p53. Virology 2009; 384:285 - 293
- DeCaprio JA. How the Rb tumor suppressor structure and function was revealed by the study of Adenovirus and SV40. Virology 2009; 384:274 - 284
- White RJ, Trouche D, Martin K, Jackson SP, Kouzarides T. Repression of RNA polymerase III transcription by the retinoblastoma protein. Nature 1996; 382:88 - 90
- Chu WM, Wang Z, Roeder RG, Schmid CW. RNA polymerase III transcription repressed by Rb through its interactions with TFIIIB and TFIIIC2. J Biol Chem 1997; 272:14755 - 14761
- Chesnokov I, Chu WM, Botchan MR, Schmid CW. p53 inhibits RNA polymerase III-directed transcription in a promoter-dependent manner. Mol Cell Biol 1996; 16:7084 - 7088
- Cairns CA, White RJ. p53 is a general repressor of RNA polymerase III transcription. EMBO J 1998; 17:3112 - 3123
- Morton JP, Kantidakis T, White RJ. RNA polymerase III transcription is repressed in response to the tumour suppressor ARF. Nucleic Acids Res 2007; 35:3046 - 3052
- Woiwode A, Johnson SA, Zhong S, Zhang C, Roeder RG, Teichmann M, et al. PTEN represses RNA polymerase III-dependent transcription by targeting the TFIIIB complex. Mol Cell Biol 2008; 28:4204 - 4214
- Veras I, Rosen EM, Schramm L. Inhibition of RNA polymerase III transcription by BRCA1. J Mol Biol 2009; 387:523 - 531
- Larminie CG, Cairns CA, Mital R, Martin K, Kouzarides T, Jackson SP, White RJ. Mechanistic analysis of RNA polymerase III regulation by the retinoblastoma protein. EMBO J 1997; 16:2061 - 2071
- Hirsch HA, Jawdekar GW, Lee KA, Gu L, Henry RW. Distinct mechanisms for repression of RNA polymerase III transcription by the retinoblastoma tumor suppressor protein. Mol Cell Biol 2004; 24:5989 - 5999
- Eichhorn K, Jackson SP. A role for TAF3B2 in the repression of human RNA polymerase III transcription in nonproliferating cells. J Biol Chem 2001; 276:21158 - 21165
- Sutcliffe JE, Cairns CA, McLees A, Allison SJ, Tosh K, White RJ. RNA polymerase III transcription factor IIIB is a target for repression by pocket proteins p107 and p130. Mol Cell Biol 1999; 19:4255 - 4261
- White RJ. RNA polymerases I and III, growth control and cancer. Nat Rev Mol Cell Biol 2005; 6:69 - 78
- Gottesfeld JM, Wolf VJ, Dang T, Forbes DJ, Hartl P. Mitotic repression of RNA polymerase III transcription in vitro mediated by phosphorylation of a TFIIIB component. Science 1994; 263:81 - 84
- Leresche A, Wolf VJ, Gottesfeld JM. Repression of RNA polymerase II and III transcription during M phase of the cell cycle. Exp Cell Res 1996; 229:282 - 288
- White RJ, Gottlieb TM, Downes CS, Jackson SP. Mitotic regulation of a TATA-binding-protein-containing complex. Mol Cell Biol 1995; 15:1983 - 1992
- Fairley JA, Scott PH, White RJ. TFIIIB is phosphorylated, disrupted and selectively released from tRNA promoters during mitosis in vivo. EMBO J 2003; 22:5841 - 5850
- Hu P, Samudre K, Wu S, Sun Y, Hernandez N. CK2 phosphorylation of Bdp1 executes cell cycle-specific RNA polymerase III transcription repression. Mol Cell 2004; 16:81 - 92
- Hu P, Wu S, Hernandez N. A minimal RNA polymerase III transcription system from human cells reveals positive and negative regulatory roles for CK2. Mol Cell 2003; 12:699 - 709
- Ghavidel A, Hockman DJ, Schultz MC. A review of progress towards elucidating the role of protein kinase CK2 in polymerase III transcription: regulation of the TATA binding protein. Mol Cell Biochem 1999; 191:143 - 148
- Ghavidel A, Schultz MC. TATA binding protein-associated CK2 transduces DNA damage signals to the RNA polymerase III transcriptional machinery. Cell 2001; 106:575 - 584
- Dephoure N, Zhou C, Villén J, Beausoleil SA, Bakalarski CE, Elledge SJ, et al. A quantitative atlas of mitotic phosphorylation. Proc Natl Acad Sci USA 2008; 105:10762 - 10767
- Gu L, Husain-Ponnampalam R, Hoffmann-Benning S, Henry RW. The protein kinase CK2 phosphorylates SNAP190 to negatively regulate SNAPC DNA binding and human U6 transcription by RNA polymerase III. J Biol Chem 2007; 282:27887 - 27896
- Gomez-Roman N, Grandori C, Eisenman RN, White RJ. Direct activation of RNA polymerase III transcription by c-Myc. Nature 2003; 421:290 - 294
- Felton-Edkins ZA, Fairley JA, Graham EL, Johnston IM, White RJ, Scott PH. The mitogen-activated protein (MAP) kinase ERK induces tRNA synthesis by phosphorylating TFIIIB. EMBO J 2003; 22:2422 - 2432
- Zhong S, Johnson DL. The JNKs differentially regulate RNA polymerase III transcription by coordinately modulating the expression of all TFIIIB subunits. Proc Natl Acad Sci USA 2009; 106:12682 - 12687
- Kenneth NS, Ramsbottom BA, Gomez-Roman N, Marshall L, Cole PA, White RJ. TRRAP and GCN5 are used by c-Myc to activate RNA polymerase III transcription. Proc Natl Acad Sci USA 2007; 104:14917 - 14922
- Dai MS, Sun XX, Lu H. Ribosomal protein L11 associates with c-Myc at 5 S rRNA and tRNA genes and regulates their expression. J Biol Chem 2010; 285:12587 - 12594
- Steiger D, Furrer M, Schwinkendorf D, Gallant P. Max-independent functions of Myc in Drosophila melanogaster. Nat Genet 2008; 40:1084 - 1091
- Greasley PJ, Bonnard C, Amati B. Myc induces the nucleolin and BN51 genes: possible implications in ribosome biogenesis. Nucleic Acids Res 2000; 28:446 - 453
- Ittmann M, Greco A, Basilico C. Isolation of the human gene that complements a temperature-sensitive cell cycle mutation in BHK cells. Mol Cell Biol 1987; 7:3386 - 3393
- Ittmann M, Ali J, Greco A, Basilico C. The gene complementing a temperature-sensitive cell cycle mutant of BHK cells is the human homologue of the yeast RPC53 gene, which encodes a subunit of RNA polymerase C (III). Cell Growth Differ 1993; 4:503 - 511
- Mann C, Micouin JY, Chiannilkulchai N, Treich I, Buhler JM, Sentenac A. RPC53 encodes a subunit of Saccharomyces cerevisiae RNA polymerase C (III) whose inactivation leads to a predominantly G1 arrest. Mol Cell Biol 1992; 12:4314 - 4326
- Winter AG, Sourvinos G, Allison SJ, Tosh K, Scott PH, Spandidos DA, White RJ. RNA polymerase III transcription factor TFIIIC2 is overexpressed in ovarian tumors. Proc Natl Acad Sci USA 2000; 97:12619 - 12624
- Marshall L, Kenneth NS, White RJ. Elevated tRNA(iMet) synthesis can drive cell proliferation and oncogenic transformation. Cell 2008; 133:78 - 89
- Johnson SA, Dubeau L, Johnson DL. Enhanced RNA polymerase III-dependent transcription is required for oncogenic transformation. J Biol Chem 2008; 283:19184 - 19191
- Hinault C, Hu J, Maier BF, Mirmira RG, Kulkarni RN. Differential expression of cell cycle proteins during ageing of pancreatic islet cells. Diabetes Obes Metab 2008; 10:136 - 146
- Bouwens L, Rooman I. Regulation of pancreatic betacell mass. Physiol Rev 2005; 85:1255 - 1270
- Atanasoski S, Notterpek L, Lee HY, Castagner F, Young P, Ehrengruber MU, et al. The protooncogene Ski controls Schwann cell proliferation and myelination. Neuron 2004; 43:499 - 511
- Goodfellow SJ, Innes F, Derblay LE, MacLellan WR, Scott PH, White RJ. Regulation of RNA polymerase III transcription during hypertrophic growth. EMBO J 2006; 25:1522 - 1533
- Blum B, Benvenisty N. The tumorigenicity of diploid and aneuploid human pluripotent stem cells. Cell Cycle 2009; 8:3822 - 3830
- White RJ, Stott D, Rigby PW. Regulation of RNA polymerase III transcription in response to F9 embryonal carcinoma stem cell differentiation. Cell 1989; 59:1081 - 1092
- Meissner W, Thomae R, Seifart KH. The activity of transcription factor IIIC1 is impaired during differentiation of F9 cells. J Biol Chem 2002; 277:7148 - 7156
- Alzuherri HM, White RJ. Regulation of a TATA-binding protein-associated factor during cellular differentiation. J Biol Chem 1998; 273:17166 - 17171
- Athineos D, Marshall L, White RJ. Regulation of TFIIIB during F9 cell differentiation. BMC Mol Biol 2010; 11:21
- Meissner W, Ahlers A, Seifart KH. The activity of transcription factor PBP, which binds to the proximal sequence element of mammalian U6 genes, is regulated during differentiation of F9 cells. Mol Cell Biol 1995; 15:5888 - 5897
- Weser S, Gruber C, Hafner HM, Teichmann M, Roeder RG, Seifart KH, et al. Transcription factor (TF)-like nuclear regulator, the 250 kDa form of Homo sapiens TFIIIB”, is an essential component of human TFIIIC1 activity. J Biol Chem 2004; 279:27022 - 27029
- Dean N, Berk AJ. Separation of TFIIIC into two functional components by sequence specific DNA affinity chromatography. Nucleic Acids Res 1987; 15:9895 - 9907
- Yoshinaga SK, Boulanger PA, Berk AJ. Resolution of human transcription factor TFIIIC into two functional components. Proc Natl Acad Sci USA 1987; 84:3585 - 3589
- Oettel S, Härtel F, Kober I, Iben S, Seifart KH. Human transcription factors IIIC2, IIIC1 and a novel component IIIC0 fulfil different aspects of DNA binding to various pol III genes. Nucleic Acids Res 1997; 25:2440 - 2447
- Yee NS, Gong W, Huang Y, Lorent K, Dolan AC, Maraia RJ, Pack M. Mutation of RNA Pol III subunit rpc2/polr3b Leads to Deficiency of Subunit Rpc11 and disrupts zebrafish digestive development. PLoS Biol 2007; 5:312
- Dittmar KA, Goodenbour JM, Pan T. Tissue-specific differences in human transfer RNA expression. PLoS Genet 2006; 2:221
- Barski A, Chepelev I, Liko D, Cuddapah S, Fleming AB, Birch J, et al. Pol II and its associated epigenetic marks are present at Pol III-transcribed noncoding RNA genes. Nat Struct Mol Biol 2010; 17:629 - 634
- Canella D, Praz V, Reina JH, Cousin P, Hernandez N. Defining the RNA polymerase III transcriptome: Genome-wide localization of the RNA polymerase III transcription machinery in human cells. Genome Res 2010; 20:710 - 721
- Moqtaderi Z, Wang J, Raha D, White RJ, Snyder M, Weng Z, Struhl K. Genomic binding profiles of functionally distinct RNA polymerase III transcription complexes in human cells. Nat Struct Mol Biol 2010; 17:635 - 640
- Oler AJ, Alla RK, Roberts DN, Wong A, Hollenhorst PC, Chandler KJ, et al. Human RNA polymerase III transcriptomes and relationships to Pol II promoter chromatin and enhancer-binding factors. Nat Struct Mol Biol 2010; 17:620 - 628
- Raha D, Wang Z, Moqtaderi Z, Wu L, Zhong G, Gerstein M, et al. Close association of RNA polymerase II and many transcription factors with Pol III genes. Proc Natl Acad Sci USA 2010; 107:3639 - 3644
- Tiedge H, Chen W, Brosius J. Primary structure, neural-specific expression and dendritic location of human BC200 RNA. J Neurosci 1993; 13:2382 - 2390
- Ozsolak F, Poling LL, Wang Z, Liu H, Liu XS, Roeder RG, et al. Chromatin structure analyses identify miRNA promoters. Genes Dev 2008; 22:3172 - 3183
- Borchert GM, Lanier W, Davidson BL. RNA polymerase III transcribes human microRNAs. Nat Struct Mol Biol 2006; 13:1097 - 1101
- Noma K, Cam HP, Maraia RJ, Grewal SI. A role for TFIIIC transcription factor complex in genome organization. Cell 2006; 125:859 - 872
- Mertens C, Roeder RG. Different functional modes of p300 in activation of RNA polymerase III transcription from chromatin templates. Mol Cell Biol 2008; 28:5764 - 5776
- Dubey RN, Gartenberg MR. A tDNA establishes cohesion of a neighboring silent chromatin domain. Genes Dev 2007; 21:2150 - 2160
- Kalkhoven E. CBP and p300: HATs for different occasions. Biochem Pharmacol 2004; 68:1145 - 1155
- Haeusler RA, Engelke DR. Genome organization in three dimensions: thinking outside the line. Cell Cycle 2004; 3:273 - 275
- Haeusler RA, Pratt-Hyatt M, Good PD, Gipson TA, Engelke DR. Clustering of yeast tRNA genes is mediated by specific association of condensin with tRNA gene transcription complexes. Genes Dev 2008; 22:2204 - 2214
- Pollock C, Huang S. The perinucleolar compartment. Cold Spring Harb Perspect Biol 2010; 2:000679