Abstract
Pannexin 1 forms ion and metabolite permeable hexameric channels with abundant expression in the central nervous system and elsewhere. Although pannexin 1 does not form intercellular channels, a common channel topology and oligomerization state, as well as involvement of the intracellular carboxyl terminal (CT) domain in channel gating, is shared with connexins. In this study, we characterized the secondary structure of the mouse pannexin 1 cytoplasmic domains to complement structural studies of the transmembrane segments and compare with similar domains from connexins. A combination of structural prediction tools and circular dichroism revealed that, unlike connexins (predominately intrinsically disordered), cytosolic regions of pannexin 1 contain approximately 50% secondary structure, a majority being α-helical. Moreover, prediction of transmembrane domains uncovered a potential membrane interacting region (I360-G370) located upstream of the caspase cleavage site (D375-D378) within the pannexin 1 CT domain. The α-helical content of a peptide containing these domains (G357-S384) increased in the presence of detergent micelles providing evidence of membrane association. We also purified a pannexin 1 CT construct containing the caspase cleavage site (M374-C426), assigned the resonances by NMR, and confirmed cleavage by Caspase-3 in vitro. On the basis of these structural studies of the cytoplasmic domains of pannexin 1, we propose a mechanism for the opening of pannexin 1 channels upon apoptosis, involving structural changes within the CT domain.
The core proteins of chordate gap junctions are connexins and of invertebrates are innexins. Although connexin homologs have not been found in invertebrates, a BLAST search for innexin homologs in chordate genomes identified the pannexin gene family.Citation1 The family has 3 members, pannexin 1 (Panx1), pannexin 2 (Panx2), and pannexin 3 (Panx3). Panx1 is the most ubiquitously expressed and the most widely studied, particularly with respect to functional properties.
There is no sequence homology between pannexins and connexins, although there is topological similarity, both families encoding proteins with four transmembrane domains and both carboxyl and amino termini within the cytoplasm. Similarly to connexins, Panx1 oligomerizes as a hexamer and traffics to the plasma membrane to form channel-like structures, as visualized with electron microscopy.Citation2,Citation3 Unlike connexins, which connect cell interiors without access to extracellular space, Panx1 channels are non-junctional, allowing movement of ions and small molecules between inside and outside of cells.Citation4-Citation6 Diameter of the Panx1 pore was estimated to be between ~17 and 21 Å, which is significantly larger than the well-studied 43 kDa connexin (Cx43), ~15 Å, consistent with early electron micrographs showing larger particles in invertebrate than vertebrate gap junctions.Citation3,Citation7,Citation8
Other similarities between connexins and Panx1 are the involvement of the carboxyl terminus in opening or closing the channel and the potential for phosphorylation of cytoplasmic serine, threonine or tyrosine residues to modify such gating. For Cx43, channel closure in response to acidification and transjunctional voltage gradients has been attributed to binding of carboxyl terminus (CT) to the cytoplasmic loop (CL) domain.Citation9,Citation10 In the case of pH gating, channel closure appears to result from acquisition of α-helicity within cytoplasmic Cx43 domains, giving rise to increased inter-domain affinity.Citation11 Phosphorylation of a specific serine residue on the cytoplasmic CT of Cx43 (S365) also changes the structure of the region, inhibiting phosphorylation of adjacent residues that would otherwise induce channel closure.Citation12 Inter-domain interactions are also hypothesized to underlie gating of Panx1. Release of ATP has been attributed to caspase cleavage of the CT of Panx1 at residue D378,Citation13 and the co-expression of hPanx1CT299–426 or hPanx1CT299–391 with the constitutively active truncated hPanx1Δ371 restored the basal inhibition of the channel.Citation13,Citation14 Phosphorylation of residues in the cytoplasmic domains of Panx1 is predicted from its amino acid sequence.Citation15,Citation16 Src family kinases have been shown to be involved in rPanx1 channel activation in CA1 neurons in response to anoxia, suggesting the phosphorylation of tyrosine(s), although phosphorylation of the putative Src consensus site was not demonstrated.Citation17 P2X7 receptor activation of Panx1 channels in J774 cells was also suspected to involve a Src tyrosine kinase, but likewise, Panx1 tyrosine phosphorylation was not detected.Citation18 More recently, during skeletal muscle potentiation, release of ATP through Panx1 channels was shown to be accompanied by increased serine and threonine phosphorylation, although specific phosphorylated residues were not identified.Citation19
Altogether, these data demonstrate the importance of Panx1 cytoplasmic domains and the need to further elucidate the mechanism of pore closure. In this study, we used a combination of structural prediction tools and circular dichroism (CD) to characterize the cytosolic domains of mPanx1. Unlike the cytoplasmic domains of connexins that are mainly intrinsically disordered, significant portions of the mPanx1 N-terminus (NT), CL, and CT adopt diverse secondary structures. We also provide evidence that a potential membrane interacting region located within the mPanx1 CT interacts with lipids and this association affects secondary structure. Finally, NMR was used to verify that Caspase-3 can interact and cleave the mPanx1 CT in vitro. These data provide the first structural information of the cytoplasmic domains of mPanx1, groundwork that will allow further clarification of the function and regulation of Panx1 channels.
Results
Structural prediction of mPanx1
To assign cytoplasmic and transmembrane (TM) domains of mPanx1, four TM prediction programs based on distinct algorithms were used (): (1) ΔG prediction server v1.0, which uses the apparent free energy difference necessary for insertion into a membrane;Citation20 (2) TMpred program, which utilizes the statistical analysis of the sequence against Tmbase (database of naturally occurring TM proteins);Citation21 (3) Dense Alignment Surface (DAS), which calculates hydrophobicity profiles based on low-stringency dot-plots of the sequence against a library;Citation22 and (4) TopPred program, which generates a hydrophobicity profile.Citation23 All algorithms predicted four TM domains, with limits similar to previously described studies: TM1, 37–56; TM2, 107–127; TM3, 217–235; TM4, 277–296.Citation15,Citation24 A unique domain, however, composed of about 10 mainly hydrophobic residues in the CT domain (~I360-G370) also emerged from all 4 algorithms (indicated with black arrows in ). The prediction values did not meet the criteria for an actual TM domain (below cutoff) but suggests a potential membrane interacting region. A similar domain was also identified in the hPanx1, which shares 86.2% identity with mPanx1, indicating a similar feature across species (data not shown).
Figure 1. Output of transmembrane (TM) domain prediction servers. Results of mPanx1 (A), mPanx2 (B), and mPanx3 (C) TM prediction of the following programs are displayed: ΔG prediction server v1.0, TMpred program, Dense Alignment Surface (DAS), and TopPred. The potential membrane interacting region of mPanx1 and mPanx3 is indicated with black arrows.
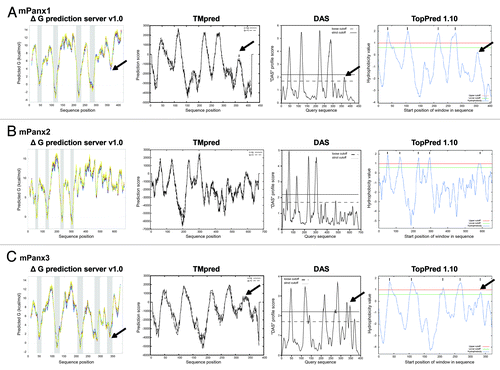
To investigate the secondary structure of the mPanx1 cytoplasmic domains, the primary sequence of the NT, CL, and CT (delineated by the TM prediction calculations) were submitted to 4 different secondary structure prediction algorithms: (1) GOR4,Citation25 based on information theory; (2) JpredCitation26 and (3) Predict (PROF),Citation27 both established from database set comparison; and (4) PSIPRED,Citation28 obtained by analysis of output from PSI-BLAST (). All 4 sets of predictions largely overlapped. Surprisingly, unlike connexins whose cytoplasmic domains are mainly intrinsically disordered, mPanx1 counterparts are predicted to present an assortment of α-helices linked by random coiled segments and some β-strands, especially in the mPanx1 NT and CT domains (). It is noteworthy that the secondary structure prediction for the suspected membrane interacting domain (~I360-G370) is mainly α-helical, followed by β-strand structure in the putative caspase cleavage site. Based on the secondary structure prediction consensus, we designed peptides of 14 to 33 amino acids to preserve the integrity of domains with predicted structure and to validate their structural predictions by CD. Peptides used for the rest of the study are indicated under the sequences in .
Figure 2. Secondary structure prediction of the mPanx1 cytoplasmic domains. Display of the predicted secondary structure of (A) mPanx1 NT, (B) mPanx1 CL, and (C) mPanx1 CT obtained by utilizing four different prediction algorithms: GOR4, Jpred, Predict (PROF), and PSIPRED. Prediction per residue is labeled under the sequence as follow: H, α-helix; E, β-strand; black dash, random coil. Peptides used for the rest of the study are indicated under the sequences. The segment expected to interact with the membrane and the caspase cleavage site are respectively indicated with a wavy and solid black line.
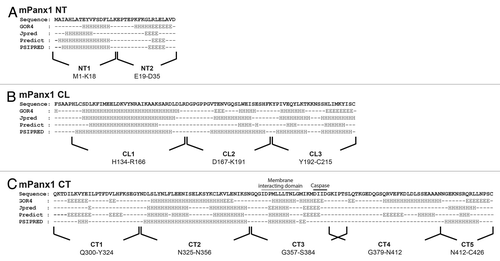
Secondary structure studies of mPanx1 cytoplasmic domain peptides by CD
Peptides are commonly used to study the secondary structure of proteins; however, caution is needed when interpreting implications for domain structure of membrane proteins as peptides lack long-range constraints that may impact their folding (e.g., being tethered to a TM domain). Therefore, the co-solvent, 2, 2, 2-trifluoroethanol (TFE) was used as a tool to probe the secondary structure propensity of the mPanx1 peptides.Citation29 Although TFE is primarily known as an α-helix-stabilizing co-solvent,Citation30,Citation31 some studies have also shown efficiency of TFE to stabilize β-strands.Citation32,Citation33 CD spectra were collected for each peptide in the absence and presence of increasing amount of TFE, however only up to 30% to ascertain intrinsic secondary structure without inducing any non-native conformation ().Citation34 As a general indication, CD spectra of α-helices present double minima at 208 nm and 222 nm and a positive peak at 193 nm; β-strands show a minimum at 217 nm and positive peak at 195 nm, and intrinsically disordered peptides are marked by a minimum near 195 nm and very low ellipticity above 210 nm.
Figure 3. Circular Dichroism (CD) analysis of mPanx1 peptides. CD spectra of (A) mPanx1 NT1 and NT2, (B) mPanx1 CL1, CL2, and CL3, (C) mPanx1 CT1, CT2, CT3, CT4, and CT5 in 1x PBS in the presence of 0 (solid line), 5 (dashed line), 15 (dotted line) and 30% (dashed and dotted line) TFE (2,2,2-trifluoroethanol).
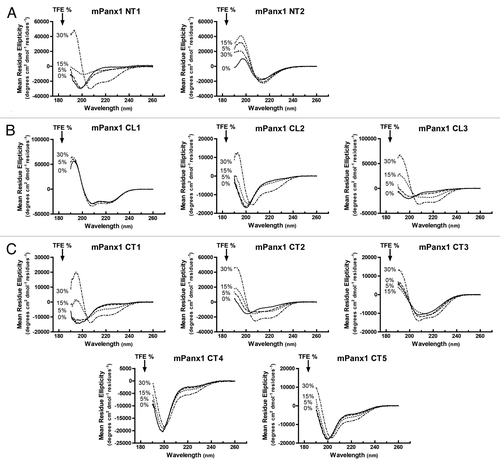
Overall, most of the CD findings were consistent with the secondary structure predictions. The C-terminus of the mPanx1 CT (CT4 and CT5) was mainly random coiled as was the middle of the mPanx1 CL (CL2) (spectra presenting minimum at 198 nm with only a weak peak at 222 nm).Citation35 The addition of 30% TFE only slightly increased the amount of α-helical structure in these peptides. mPanx1 NT1 and CT1 showed spectra consistent with random coiled structure; however, they have a propensity to form an α-helix at 15% TFE. Similarly, mPanx1 CL3 and CT2 were mainly random coiled in absence of TFE but exhibited α-helical structure with as little as 5% TFE, suggesting a higher propensity to form an α-helix than the mPanx1 NT1 and CT1 domains. Finally, the C-terminus of the NT and the N-terminus of the CL were inherently structured in absence of TFE. NT2 presented a spectrum characteristic of a β-strand with a minimum at 217 nm, while the CD spectrum of CL1 exhibited 2 minima at 208 and 222 nm typical of an α-helix. Increasing the amount of TFE did not impact the structure of these peptides. Interestingly, the mPanx1 CT3 encompassing the membrane interacting domain (~I360-G370) and caspase cleavage site (D375-D378) displayed a spectrum suggesting a mixture of α-helix and β-strand, consistent with the secondary structure prediction (). In presence of 30% of TFE though, the peptide preferred an α-helical structure as shown by the spectrum with a double minima around 208 and 220 nm. In summary, out of the 10 peptides analyzed, 5 of them were disordered or presented a low propensity to form secondary structures (NT1, CL2, CT1, CT4, and CT5), 2 showed a very high propensity to form α-helices (CL3 and CT2), and 3 of them were inherently structured (α-helix and/or β-strand; NT2, CL1 and CT3). Significantly, unlike the predominantly unstructured nature of most connexin intracellular domains, about 50% of the cytoplasmic residues of mPanx1 are expected to be involved in some sort of secondary structure in their native state.
Secondary structure change of mPanx1 CT3 in presence of lipid
The TM prediction programs suggested that the mPanx1 CT segment comprising residues I360-G370 interacts with the membrane. To test this possibly, we collected CD spectra for the mPanx1 CT3 peptide in the presence and absence of 1-palmitoyl-2-hydroxy-sn-glycero-3-[phospho-RAC-(1-glycerol)] (LPPG), a membrane mimetic detergent (). Upon addition of 8% LPPG, the spectrum exhibited a second minimum at 222 nm and an increased positive maximum at 190 nm, indicating an increase in α-helix structure. Interestingly, CD revealed that the CT3 peptide alone (no LPPG) contained a mixture of α-helix and β-strand structure; however, an α-helical conformation was more prominent in presence of 30% TFE. Altogether, the data support the possibility that the central portion of the mPanx1 CT domain interacts with the membrane, and the association increases the α-helical content of this domain.
Assignment of resonances for amino acids comprising the mPanx1 CT374–426
To provide a global perspective to complement the peptide CD data, NMR was used to investigate the secondary structure on a residue-by-residue basis as well as determine the location of molecular partner interactions. We initially focused on the mPanx1 CT because of the unique membrane interacting domain (~I360-G370), reported cleavage by Caspase-3, and the presence of predicted sites of phosphorylation. The full-length mPanx1 CT domain (Q300-C426) was insoluble. While it is conceivable that solubility might have been improved if reconstituted in a lipid environment, we attempted to bypass this problem by cloning 2 separate mPanx1 CT domains (mPanx1 CT300–356 and CT374–426), thereby excluding the highly hydrophobic segment. Unfortunately, although both polypeptides overexpressed, only the mPanx1 CT374–426 was present in the soluble fraction after cell lysis (data not shown). Therefore, mPanx1 CT374–426 was purified in 1x PBS (pH 7.4) to a final concentration of 3 mM. All 1H, 15N, 13C backbone resonances and most side chains were assigned at 7 °C (BioMag ResBank database, accession number 19814). Data were collected at a low temperature (7 °C vs. 25 °C) to reduce the molecular tumbling and increase the spectral resolution. Presented in is the assigned 15N-HSQC spectrum of the mPanx1 CT374–426 domain. The 15N-HSQC spectrum, in which each amino acid (except Pro) gives a discrete signal, displays a narrow chemical shift dispersion in the 1H dimension typical of intrinsically disordered domain. This observation is in agreement with CD data and analysis of the chemical shifts. CD shows the mPanx1 CT374–426 is predominantly unstructured even in the presence of increasing concentration of TFE (). Meanwhile, evaluation of the chemical shift deviations with respect to the random coil value (compared with the Wishart databaseCitation36), which is used to predict secondary structure, indicates the lack thereof for the mPanx1 CT374–426 domain ().
Figure 5. Structural study of the mPanx1 CT374–426 domain. (A) 15N-HSQC spectrum of the mPanx1 CT374–426. Each resonance peak has been labeled. (B) CD spectra of mPanx1 CT374–426 in 1x PBS in the presence of 0 (solid line), 5 (dashed line), 15 (dotted line) and 30% (dashed and dotted line) TFE. (C) Chemical shift index analysis for 1Hα, 13Cα, 13C, and 15N obtained by comparison to the Wishart database.
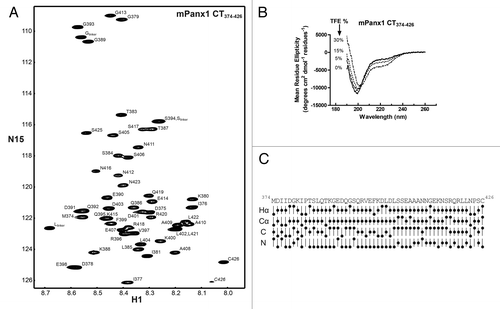
In vitro Caspase-3 cleavage assay of mPanx1 CT374–426
When studying a soluble domain from a membrane protein, a critical question is whether it retains the same biological function as when in the native condition. Therefore, the ability was assessed of the mPanx1 CT374–426 construct to be cleaved by the known protein partner, Caspase-3.Citation13 Active recombinant Caspase-3 (25U) was added to purified labeled mPanx1 CT374–426 and the cleavage reaction was monitored by NMR. After 1 h incubation at 37 °C, a decrease of intensity of the peaks surrounding the caspase cleavage site (I376-I381) as well as the appearance of 4 new peaks was observed (, new peaks indicated with black arrows). An additional 25U of Caspase-3 followed by 2 more hours at 37 °C led to complete cleavage of the mPanx1 CT374–426 construct after residue D378. This is evident by the 3 residues on each side of the cleavage site disappearing congruent with an increase in the intensity of the 4 new peaks. Interestingly, 3 of the 4 new peaks presented chemical shift close to the 3 residues preceding the cleavage site, I376, I377, and D378, suggesting that they represent the chemical shift of these 3 residues in the GPLGSMDIID peptide released upon cleavage (, inset). Meanwhile, the signals of G379, K380, and I381 residues, localized on the C-terminal of the cleavage site disappeared and only one of them, most likely I381, emerged as a new peak. This is not surprising since G379 and K380 constitute the new N-terminus of the cleaved peptide G379-C426, and the dynamic nature of the N-terminal residues of a soluble protein usually prevents their detection in the 15N-HSQC spectrum.
Figure 6. In vitro Caspase-3 cleavage assay. 15N-HSQC spectrum of mPanx1 CT374–426 alone (black) has been overlaid with spectra obtained in the presence of 25U of Caspase-3 after 1 h at 37 °C (red) and after 25U more of Caspase-3 and an additional 2 h of incubation at 37 °C (green). Residues affected by the cleavage reaction have been labeled and the new chemical shifts are indicated with black arrows. Sequence of the mPanx1 CT374–426 region involved in the cleavage (cloning linker attached to residues M374-L385) is displayed (inset). Residues affected by the cleavage are colored in red. Caspase recognition sequence and cleavage site are respectively indicated by a solid black line and a purple arrow. Residues colored in gray are not visible on the spectra due to their N-terminal location.
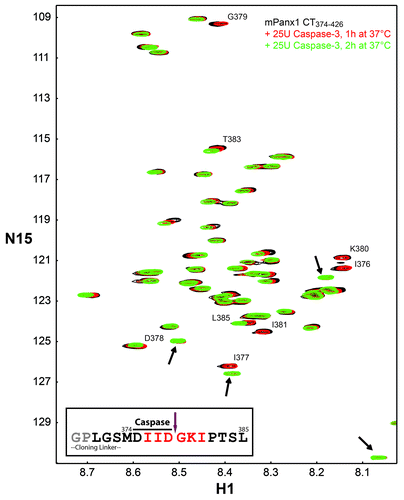
Discussion
Here we provide the first structural information regarding the cytoplasmic domains of mPanx1. These studies complement the data of rPanx1 obtained by electron microscopy that defined the general architecture of the channel and the pore, but were unable to give insight regarding the soluble domains.Citation2,Citation3 CD and secondary structural prediction data revealed that the mPanx1 cytosolic domains present a significant amount of secondary structures, estimated at up to 50% of the primary sequence. mPanx1 regions NT2, CL1, and CT3 were inherently structured, respectively β-strand, α-helix and a mixture of both β-strand and α-helix, and the CL3 and CT2 domains displayed a high propensity for α-helix conformation ().Citation37 Our laboratory has also performed similar structural studies with several connexin cytoplasmic domains and while they are predominately disordered, they do contain small domains with inherent propensity and susceptibility to adopt secondary structure.Citation29,Citation38,Citation39 Interestingly, the structured segments of mPanx1 and some of the connexins somewhat overlap when comparing their cytoplasmic domains and proposed distance from the membrane. For example, the second half of the CL domains of Cx43, Cx36 and Cx32 exhibit a high propensity to form α-helical structure, similarly to mPanx1 CL3. Meanwhile, there is a correlation between the centrally located α-helices of the mPanx1, Cx40, Cx43, and Cx45 CT domains.Citation38-Citation40
Figure 7. Model of the mPanx1 topology. Overall topology of mPanx1 is depicted. Each amino acid is represented by a black circle. mPanx1 CT segment predicted to interact with the membrane has been modeled (CT3). Domains shown to have a secondary structure by CD are cartooned in green hexagon for α-helix and magenta up-arrows for β-strand. mPanx1 CT Caspase-3 site (DIID) is labeled in black. Extracellular cysteines and the N-glycosylation site are indicated with orange circles and a blue diamond, respectively.
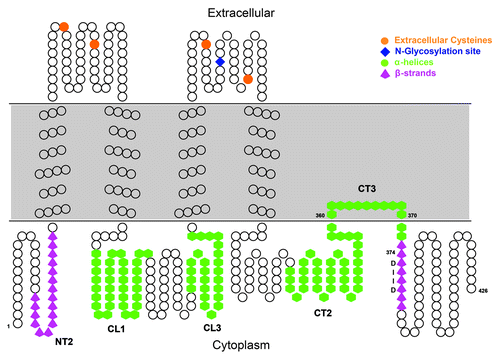
Secondary structural predictions for the cytosolic domains of mPanx2 and mPanx3 were also calculated employing the algorithms used for mPanx1 (). Similarly to mPanx1, mPanx3 NT contains some β-strand structure in its second half, while mPanx2 NT is mainly α-helical. The most similarities are found in the CL domains with α-helices linked by random coiled portions. Finally, like the mPanx1 CT, the mPanx3 CT presents a central α-helical segment and the second half of Panx2 CT is mainly intrinsically disordered. Noticeably, the mPanx2 CT shows only a structural consensus for three small α-helices in its first half.
Figure 8. Secondary structure prediction of the mPanx2 and mPanx3 cytoplasmic domains. Display of the predicted secondary structure of (A) mPanx1 and (B) mPanx2 NT, CL, and CT domains obtained by utilizing three different prediction algorithms: GOR4, Jpred, and PSIPRED. Prediction per residue is labeled under the sequence as follow: H, α-helix; E, β-strand; black dash, random coil. The mPanx3 segment expected to interact with the membrane is indicated with a wavy black line.
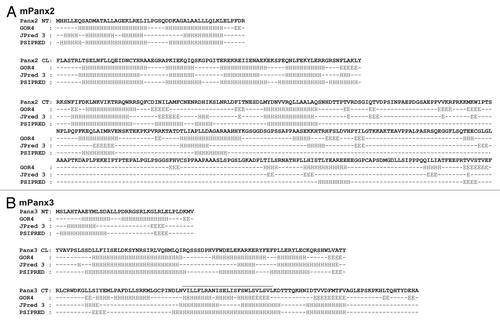
Another interesting feature detected by TM prediction algorithms is a potential membrane interacting segment spanning I360-G370 in the mPanx1 CT; residues that present high hydrophobicity and α-helical content. Here, we provide the first evidence that this mPanx1 CT domain could interact with the membrane as its secondary structure composition was modified (i.e., increase in α-helical content) in presence of membrane mimetic lipids. mPanx2 and mPanx3 sequences were also subjected to the same TM prediction servers (). There was no consensus for such a membrane interacting domain in the CT of mPanx2; however, the results for mPanx3 converged to identify a segment of ~20 residues modeled as a fifth TM domain. The secondary structure of this region, like mPanx1, is predicted to be α-helical (). Although the definitive topology of mPanx3 remains to be clarified, the existence of a real fifth TM is less likely and this domain is expected to interact with the membrane similarly to mPanx1 CT360–370. A “reentrant” loop, or half-membrane spanning domain located between 2 TM domains is commonly found in ion channels, such as the Kcsa potassium ion channel and the glutamate receptor ion channel.Citation41,Citation42 However, to our knowledge, a membrane interacting domain localized in the cytosolic C-terminal of an integral protein is less frequent. Moreover, the location of this segment is of importance as cleavage of residues situated just downstream by Caspase-3 and -7 (after D378) is a mechanism to open Panx1 channels to release ATP into the extracellular space during apoptosis.Citation13,Citation43,Citation44
While generally found in a closed state, in addition to apoptosis, Panx1 channels can become active (high conductance pores that are non-selectively permeable to ions and small molecules of up to 1.5 kDa) by mechanical stimulation, elevation of extracellular [K+] or through purinergic receptors (for review see ref. Citation45). The exact molecular details that maintain Panx1 channels in the closed state are still not clear, but a “particle-receptor” model between the CT and the pore, comparable to connexins, has been suggested. CT residues involved in this mechanism have emerged from studies involving hPanx1 deletion constructs and peptides. The hPanx1Δ371 construct is constitutionally active while hPanx1Δ391 resembles that of the wild type.Citation13,Citation14 Additionally, the hPanx1 peptide CT380–391 had no inhibitory effect on activated hPanx1 channels, as opposed to the co-expression of hPanx1 CT299–391 that closed the pore.Citation14 Together, these data indicate involvement of residues I372-D379 in pore closure. Of note, these residues overlap with the caspase cleavage site and are in close proximity to the membrane interacting domain.
Based upon the structural analysis provided herein and data from the literature, we propose a model where the mPanx1 CT372–379 domain participates in channel gating: under normal physiological conditions (closed state), association of residues I360-G370 with the membrane enables residues 372–379 to interact with the pore forming domain as an α-helix. Mechanical stimulation, apoptosis, elevation of extracellular [K+], and purinergic receptors cause, by yet to be determined mechanism(s), release of residues 372–379 from the pore forming domain. Residues 372–379 then switch secondary structure to a β-strand. In the case of apoptosis, this forms a high affinity binding site for caspases to facilitate cleavage. This succession of events, helped by the tethering of this domain to the membrane interacting segment, leads to the activation of the channel and the release of ATP outside of the cell. Further investigation will have to be performed to confirm or refute this model.
Materials and Methods
Peptide synthesis, and expression and purification of Panx1 CT374–426
Panx1 peptides described in and were synthesized by LifeTein (95% purity). The mPanx1 CT374–426 polypeptide was subcloned into the bacterial expression vector pGEX-6P-2 (GST-tagged; http://www.gelifesciences.com/catalogue/item/28-9546-50) by standard PCR methods and transformed for overexpression in BL21(DE3) competent cells (http://www.genomics.agilent.com/catalogue/item/200131). Growth and purification of the 15N13C-labeled polypeptide was conducted as previously described for recombinant GST-tagged protein, the tag being removed by Turbo3C protease digestion (http://www.accelagen.com/catalog/item/H0101S).Citation10,Citation11,Citation46
Circular Dichroïsm (CD)
CD experiments were performed on a JASCO J-815 CD spectrometer at 25 °C in the far UV (260–190 nm) with a 0.1 mm path length quartz cell, using a bandwidth of 1 nm, an integration time of 1 s, and a scan rate of 50 nm/min. Each spectrum is the average of 5 scans. All spectra were corrected by subtracting the solvent spectrum acquired under identical conditions. The synthetic or recombinant peptides were in solution in 1x PBS, in the presence of various amount of TFE or 1-palmitoyl-2-hydroxy-sn-glycero-3-[phospho-RAC-(1-glycerol)] (LPPG), at final concentrations between 0.3–0.9 mM depending on the peptide CD signal. All CD data were processed and converted to mean residue ellipticity to account for the peptide size and concentration differences using Spectra Analysis from the Jasco Spectra Manager software, Version 2.05.01.
Nuclear Magnetic Resonance (NMR)
NMR data were acquired at 7 °C using a 600 MHz Varian INOVA NMR spectrometer outfitted with a cryo-probe. Purified 15N13C-labeled Panx1 CT374–426 was used to collect NMR experiments necessary to determine the backbone sequential assignments (3 mM in 1x PBS), as previously described.Citation47 NMR spectra were processed using NMRPipe and analyzed using NMRView.Citation48,Citation49
In vitro Caspase-3 cleavage assay
15N13C-labeled Panx1 CT374–426 (0.1 mM in 1x PBS, 10 mM DTT, and 5 mM EDTA) was incubated at 37 °C for one hour in presence of 25U of recombinant Caspase-3 (http://www.enzolifesciences.com/catalogue/item/ALX-201-059). To bring the cleavage to completion, an additional 25U of Caspase-3 was added followed by incubation for 2 more hours at 37 °C. Progression of the reaction was followed by collecting 15N-HSQC experiments.
Abbreviations: | ||
Panx1 | = | pannexin 1 |
Panx2 | = | pannexin 2 |
Panx3 | = | pannexin 3 |
Cx43 | = | connexin 43 |
CD | = | circular dichroism |
NMR | = | nuclear magnetic resonance |
TM | = | transmembrane |
NT | = | N-terminus |
CL | = | cytoplasmic loop |
CT | = | C-terminus |
TFE | = | 2,2,2-trifluoroethanol |
Disclosure of Potential Conflicts of Interest
No potential conflicts of interest were disclosed.
Acknowledgments
This work was supported, in whole or in part, by the National Institutes of Health grants GM07263 (PS) and NS041282 (DS). The authors would like to thank Marcia Urban-Maldonado and Sydney Zack for their technical assistance with cloning and protein purification respectively.
References
- Panchin Y, Kelmanson I, Matz M, Lukyanov K, Usman N, Lukyanov S. A ubiquitous family of putative gap junction molecules. Curr Biol 2000; 10:R473 - 4; http://dx.doi.org/10.1016/S0960-9822(00)00576-5; PMID: 10898987
- Boassa D, Ambrosi C, Qiu F, Dahl G, Gaietta G, Sosinsky G. Pannexin1 channels contain a glycosylation site that targets the hexamer to the plasma membrane. J Biol Chem 2007; 282:31733 - 43; http://dx.doi.org/10.1074/jbc.M702422200; PMID: 17715132
- Ambrosi C, Gassmann O, Pranskevich JN, Boassa D, Smock A, Wang J, Dahl G, Steinem C, Sosinsky GE. Pannexin1 and Pannexin2 channels show quaternary similarities to connexons and different oligomerization numbers from each other. J Biol Chem 2010; 285:24420 - 31; http://dx.doi.org/10.1074/jbc.M110.115444; PMID: 20516070
- Vanden Abeele F, Bidaux G, Gordienko D, Beck B, Panchin YV, Baranova AV, Ivanov DV, Skryma R, Prevarskaya N. Functional implications of calcium permeability of the channel formed by pannexin 1. J Cell Biol 2006; 174:535 - 46; http://dx.doi.org/10.1083/jcb.200601115; PMID: 16908669
- Iglesias R, Dahl G, Qiu F, Spray DC, Scemes E. Pannexin 1: the molecular substrate of astrocyte “hemichannels”. J Neurosci 2009; 29:7092 - 7; http://dx.doi.org/10.1523/JNEUROSCI.6062-08.2009; PMID: 19474335
- Bao L, Locovei S, Dahl G. Pannexin membrane channels are mechanosensitive conduits for ATP. FEBS Lett 2004; 572:65 - 8; http://dx.doi.org/10.1016/j.febslet.2004.07.009; PMID: 15304325
- Unger VM, Kumar NM, Gilula NB, Yeager M. Three-dimensional structure of a recombinant gap junction membrane channel. Science 1999; 283:1176 - 80; http://dx.doi.org/10.1126/science.283.5405.1176; PMID: 10024245
- Epstein ML, Gilula NB. A study of communication specificity between cells in culture. J Cell Biol 1977; 75:769 - 87; http://dx.doi.org/10.1083/jcb.75.3.769; PMID: 562887
- Moreno AP, Chanson M, Elenes S, Anumonwo J, Scerri I, Gu H, Taffet SM, Delmar M. Role of the carboxyl terminal of connexin43 in transjunctional fast voltage gating. Circ Res 2002; 90:450 - 7; http://dx.doi.org/10.1161/hh0402.105667; PMID: 11884375
- Hirst-Jensen BJ, Sahoo P, Kieken F, Delmar M, Sorgen PL. Characterization of the pH-dependent interaction between the gap junction protein connexin43 carboxyl terminus and cytoplasmic loop domains. J Biol Chem 2007; 282:5801 - 13; http://dx.doi.org/10.1074/jbc.M605233200; PMID: 17178730
- Duffy HS, Sorgen PL, Girvin ME, O’Donnell P, Coombs W, Taffet SM, Delmar M, Spray DC. pH-dependent intramolecular binding and structure involving Cx43 cytoplasmic domains. J Biol Chem 2002; 277:36706 - 14; http://dx.doi.org/10.1074/jbc.M207016200; PMID: 12151412
- Solan JL, Marquez-Rosado L, Sorgen PL, Thornton PJ, Gafken PR, Lampe PD. Phosphorylation at S365 is a gatekeeper event that changes the structure of Cx43 and prevents down-regulation by PKC. J Cell Biol 2007; 179:1301 - 9; http://dx.doi.org/10.1083/jcb.200707060; PMID: 18086922
- Chekeni FB, Elliott MR, Sandilos JK, Walk SF, Kinchen JM, Lazarowski ER, Armstrong AJ, Penuela S, Laird DW, Salvesen GS, et al. Pannexin 1 channels mediate ‘find-me’ signal release and membrane permeability during apoptosis. Nature 2010; 467:863 - 7; http://dx.doi.org/10.1038/nature09413; PMID: 20944749
- Sandilos JK, Chiu YH, Chekeni FB, Armstrong AJ, Walk SF, Ravichandran KS, Bayliss DA. Pannexin 1, an ATP release channel, is activated by caspase cleavage of its pore-associated C-terminal autoinhibitory region. J Biol Chem 2012; 287:11303 - 11; http://dx.doi.org/10.1074/jbc.M111.323378; PMID: 22311983
- Penuela S, Bhalla R, Gong XQ, Cowan KN, Celetti SJ, Cowan BJ, Bai D, Shao Q, Laird DW. Pannexin 1 and pannexin 3 are glycoproteins that exhibit many distinct characteristics from the connexin family of gap junction proteins. J Cell Sci 2007; 120:3772 - 83; http://dx.doi.org/10.1242/jcs.009514; PMID: 17925379
- Barbe MT, Monyer H, Bruzzone R. Cell-cell communication beyond connexins: the pannexin channels. Physiology (Bethesda) 2006; 21:103 - 14; http://dx.doi.org/10.1152/physiol.00048.2005; PMID: 16565476
- Weilinger NL, Tang PL, Thompson RJ. Anoxia-induced NMDA receptor activation opens pannexin channels via Src family kinases. J Neurosci 2012; 32:12579 - 88; http://dx.doi.org/10.1523/JNEUROSCI.1267-12.2012; PMID: 22956847
- Iglesias R, Locovei S, Roque A, Alberto AP, Dahl G, Spray DC, Scemes E. P2X7 receptor-Pannexin1 complex: pharmacology and signaling. Am J Physiol Cell Physiol 2008; 295:C752 - 60; http://dx.doi.org/10.1152/ajpcell.00228.2008; PMID: 18596211
- Riquelme MA, Cea LA, Vega JL, Boric MP, Monyer H, Bennett MV, Frank M, Willecke K, Sáez JC. The ATP required for potentiation of skeletal muscle contraction is released via pannexin hemichannels. Neuropharmacology 2013; 75:594 - 603; http://dx.doi.org/10.1016/j.neuropharm.2013.03.022; PMID: 23583931
- Hessa T, Meindl-Beinker NM, Bernsel A, Kim H, Sato Y, Lerch-Bader M, Nilsson I, White SH, von Heijne G. Molecular code for transmembrane-helix recognition by the Sec61 translocon. Nature 2007; 450:1026 - 30; http://dx.doi.org/10.1038/nature06387; PMID: 18075582
- Hofmann K, Stoffel W. TMBASE - A database of membrane spanning protein segments. Biol Chem Hoppe Seyler 1993; 374:166
- Cserzö M, Wallin E, Simon I, von Heijne G, Elofsson A. Prediction of transmembrane alpha-helices in prokaryotic membrane proteins: the dense alignment surface method. Protein Eng 1997; 10:673 - 6; http://dx.doi.org/10.1093/protein/10.6.673; PMID: 9278280
- von Heijne G. Membrane protein structure prediction. Hydrophobicity analysis and the positive-inside rule. J Mol Biol 1992; 225:487 - 94; http://dx.doi.org/10.1016/0022-2836(92)90934-C; PMID: 1593632
- Wang J, Dahl G. SCAM analysis of Panx1 suggests a peculiar pore structure. J Gen Physiol 2010; 136:515 - 27; http://dx.doi.org/10.1085/jgp.201010440; PMID: 20937692
- Garnier J, Gibrat JF, Robson B. GOR method for predicting protein secondary structure from amino acid sequence. Methods Enzymol 1996; 266:540 - 53; http://dx.doi.org/10.1016/S0076-6879(96)66034-0; PMID: 8743705
- Cole C, Barber JD, Barton GJ. The Jpred 3 secondary structure prediction server. Nucleic Acids Res 2008; 36:W197-201; http://dx.doi.org/10.1093/nar/gkn238; PMID: 18463136
- Rost B, Yachdav G, Liu J. The PredictProtein server. Nucleic Acids Res 2004; 32:W321-6; http://dx.doi.org/10.1093/nar/gkh377; PMID: 15215403
- Jones DT. Protein secondary structure prediction based on position-specific scoring matrices. J Mol Biol 1999; 292:195 - 202; http://dx.doi.org/10.1006/jmbi.1999.3091; PMID: 10493868
- Fort AG, Spray DC. Trifluoroethanol reveals helical propensity at analogous positions in cytoplasmic domains of three connexins. Biopolymers 2009; 92:173 - 82; http://dx.doi.org/10.1002/bip.21166; PMID: 19226516
- Sönnichsen FD, Van Eyk JE, Hodges RS, Sykes BD. Effect of trifluoroethanol on protein secondary structure: an NMR and CD study using a synthetic actin peptide. Biochemistry 1992; 31:8790 - 8; http://dx.doi.org/10.1021/bi00152a015; PMID: 1390666
- Kentsis A, Sosnick TR. Trifluoroethanol promotes helix formation by destabilizing backbone exposure: desolvation rather than native hydrogen bonding defines the kinetic pathway of dimeric coiled coil folding. Biochemistry 1998; 37:14613 - 22; http://dx.doi.org/10.1021/bi981641y; PMID: 9772190
- Ramírez-Alvarado M, Kortemme T, Blanco FJ, Serrano L. Beta-hairpin and beta-sheet formation in designed linear peptides. Bioorg Med Chem 1999; 7:93 - 103; http://dx.doi.org/10.1016/S0968-0896(98)00215-6; PMID: 10199660
- Blanco FJ, Serrano L. Folding of protein G B1 domain studied by the conformational characterization of fragments comprising its secondary structure elements. Eur J Biochem 1995; 230:634 - 49; http://dx.doi.org/10.1111/j.1432-1033.1995.tb20605.x; PMID: 7607238
- Reiersen H, Rees AR. Trifluoroethanol may form a solvent matrix for assisted hydrophobic interactions between peptide side chains. Protein Eng 2000; 13:739 - 43; http://dx.doi.org/10.1093/protein/13.11.739; PMID: 11161104
- Brahms S, Brahms J. Determination of protein secondary structure in solution by vacuum ultraviolet circular dichroism. J Mol Biol 1980; 138:149 - 78; http://dx.doi.org/10.1016/0022-2836(80)90282-X; PMID: 7411608
- Wishart DS, Sykes BD. The 13C chemical-shift index: a simple method for the identification of protein secondary structure using 13C chemical-shift data. J Biomol NMR 1994; 4:171 - 80; http://dx.doi.org/10.1007/BF00175245; PMID: 8019132
- Johns SJ. TOPO2, transmembrane protein display software, http://www.sacs.ucsf.edu/TOPO2/
- Sorgen PL, Duffy HS, Sahoo P, Coombs W, Delmar M, Spray DC. Structural changes in the carboxyl terminus of the gap junction protein connexin43 indicates signaling between binding domains for c-Src and zonula occludens-1. J Biol Chem 2004; 279:54695 - 701; http://dx.doi.org/10.1074/jbc.M409552200; PMID: 15492000
- Bouvier D, Spagnol G, Chenavas S, Kieken F, Vitrac H, Brownell S, Kellezi A, Forge V, Sorgen PL. Characterization of the structure and intermolecular interactions between the connexin40 and connexin43 carboxyl-terminal and cytoplasmic loop domains. J Biol Chem 2009; 284:34257 - 71; http://dx.doi.org/10.1074/jbc.M109.039594; PMID: 19808665
- Kopanic JL, Sorgen PL. Chemical shift assignments of the connexin45 carboxyl terminal domain: monomer and dimer conformations. Biomol NMR Assign 2013; 7:293 - 7; http://dx.doi.org/10.1007/s12104-012-9431-9; PMID: 23070843
- Jiang Y, Lee A, Chen J, Cadene M, Chait BT, MacKinnon R. The open pore conformation of potassium channels. Nature 2002; 417:523 - 6; http://dx.doi.org/10.1038/417523a; PMID: 12037560
- Traynelis SF, Wollmuth LP, McBain CJ, Menniti FS, Vance KM, Ogden KK, Hansen KB, Yuan H, Myers SJ, Dingledine R. Glutamate receptor ion channels: structure, regulation, and function. Pharmacol Rev 2010; 62:405 - 96; http://dx.doi.org/10.1124/pr.109.002451; PMID: 20716669
- Bao L, Locovei S, Dahl G. Pannexin membrane channels are mechanosensitive conduits for ATP. FEBS Lett 2004; 572:65 - 8; http://dx.doi.org/10.1016/j.febslet.2004.07.009; PMID: 15304325
- Locovei S, Wang J, Dahl G. Activation of pannexin 1 channels by ATP through P2Y receptors and by cytoplasmic calcium. FEBS Lett 2006; 580:239 - 44; http://dx.doi.org/10.1016/j.febslet.2005.12.004; PMID: 16364313
- Sandilos JK, Bayliss DA. Physiological mechanisms for the modulation of pannexin 1 channel activity. J Physiol 2012; 590:6257 - 66; http://dx.doi.org/10.1113/jphysiol.2012.240911; PMID: 23070703
- Kieken F, Spagnol G, Su V, Lau AF, Sorgen PL. NMR structure note: UBA domain of CIP75. J Biomol NMR 2010; 46:245 - 50; http://dx.doi.org/10.1007/s10858-010-9397-9; PMID: 20127391
- Sorgen PL, Duffy HS, Cahill SM, Coombs W, Spray DC, Delmar M, Girvin ME. Sequence-specific resonance assignment of the carboxyl terminal domain of Connexin43. J Biomol NMR 2002; 23:245 - 6; http://dx.doi.org/10.1023/A:1019892719979; PMID: 12238598
- Delaglio F, Grzesiek S, Vuister GW, Zhu G, Pfeifer J, Bax A. NMRPipe: a multidimensional spectral processing system based on UNIX pipes. J Biomol NMR 1995; 6:277 - 93; http://dx.doi.org/10.1007/BF00197809; PMID: 8520220
- Johnson BA, Blevins RA. NMR View: A computer program for the visualization and analysis of NMR data. J Biomol NMR 1994; 4:603 - 14; http://dx.doi.org/10.1007/BF00404272; PMID: 22911360