Abstract
Growth of Saccharomyces cerevisiae in poor nitrogen sources or exposure to the Tor inhibitor rapamycin results in expression of the nitrogen catabolite repressed (NCR) genes whose products are involved in scavenging and metabolizing nitrogen. The NCR genes are regulated by the GATA-like transactivators Gln3 and Gat1, which are thought to be under control of the rapamycin-sensitive Tor complex 1 (TORC1). We have recently shown that Gln3 nuclear translocation in response to nitrogen source quality but not in response to rapamycin requires Golgi to endosome trafficking. These and previous findings that several TORC1 components localize to low density endomembranes are discussed in a model that underscores a prominent role for the vesicular trafficking system in facilitating molecular interactions in response to nitrogen source. In addition, these findings have important implications for Tor signaling and rapamycin mechanism of action, both in yeast and in metazoans.
In the yeast Saccharomyces cerevisiae the expression of genes required for uptake and metabolism of suboptimal nitrogen sources is regulated by the transactivators Gln3 and Gat1 and the repressor Ure2.Citation1 Activity of these transactivators is in large part modulated by the nutrient-responsive and rapamycin-sensitive TORC1 and its downstream effector the Tap42-Sit4 protein phosphatase.Citation2–Citation6 It has been proposed that, during growth under optimal nitrogen conditions (i.e., glutamine or ammonium), Tap42-Sit4 is anchored to light membranes via interaction with TORC1.Citation7 Rapamycin exposure or complete nitrogen starvation results in Tap42-Sit4 release into the cytosol and Gln3 dephosphorylation, which in turn leads to Ure2-Gln3 complex dissociation and Gln3 nuclear translocation.Citation7,Citation8 Intriguingly, this model does not operate for Gln3 activation in response to nitrogen source discrimination: transfer of cells from rich to poor nitrogen medium (proline) does not cause release of Tap42-Sit4 from membranesCitation8 and although Gln3 translocates into the nucleus in a Sit4-dependent fashion, it is apparently not dephosphorylated.Citation9,Citation10 Importantly, it has been suggested that Tap42-Sit4 activity is not influenced by the nitrogen source.Citation10 Collectively these results argue that, under poor nitrogen conditions, Tap42-Sit4 remains active and Gln3 is dephosphorylated at specific amino acid residues or to an extent not detectable by gel shift or, less likely, Tap42-Sit4 dephosphorylates another target that in turn regulates Gln3 activation.
The class C Vps protein complex mediates docking and membrane fusion of Golgi-derived vesicles at the endosome, and between endosomes and vacuoles, and thus, actively regulates protein and membrane traffic between these cellular compartments.Citation11,Citation12 The vacuole is a major amino acid reservoir and defects in class C VPS genes result in severely fragmented vacuoles, low amino acid levels, and defects in poor nitrogen source utilization and nitrogen starvation survival.Citation13–Citation16 Importantly, class C Vps complex function is required to provide amino acid homeostasis for efficient TORC1 signaling.Citation15
Recently, we demonstrated that the inability of class C vps mutants to utilize poor nitrogen sources is attributable to marked defects in Gln3 nuclear translocation and NCR gene expression.Citation16 Similar defects were also observed in class D vps mutants, which are impaired in Golgi to endosome transport, but not in mutants affected in other protein trafficking steps. Strikingly, Gln3 nuclear translocation elicited by rapamycin was independent of class C and D Vps function. A closer examination of Gln3 cytoplasmic distribution in wild-type cells revealed a significant fraction of Gln3 and Ure2 peripherally associated with low-density membranes and Gln3 colocalized with Golgi and endosomal markers. Other poor nitrogen source-induced TORC1 readouts including repression of ribosomal protein and induction of STRE genes, known to be regulated via Sch9 independently of Tap42-Sit4, were not altered by class C and D vps mutations.Citation16,Citation17 These findings indicate that Golgi to endosome trafficking is crucial for Gln3 nitrogen source regulation and have important implications for understanding TORC1 signaling and rapamycin mechanisms of action.
Our studies support a model in which, under poor nitrogen source conditions, Gln3-Ure2 associated with Golgi-derived vesicles is transported to endosomes or a later compartment where activated Tap42-Sit4 complexes normally reside and act to dephosphorylate Gln3 (). In this model rapamycin-induced membrane liberation of Tap42-Sit4 complexes bypasses the requirement of Gln3-Ure2 to undergo Golgi to endosome transport for activation (). Experimental avenues to test this model include: (1) examine if Ure2 colocalizes with Gln3 along with Golgi and endosomal markers, (2) identification of the light membranes on which Tap42-Sit4 resides in association with TORC1, (3) determine whether membrane TORC1-associated Tap42-Sit4 complexes are active, and (4) examine if other TORC1-Tap42 targets require Golgi to endosome transport for regulation. Further examination of this model is likely to reveal insights into the mechanisms of Tor signaling and rapamycin action, which presently are not well understood.
In summary these studies reveal an unanticipated role for the vesicle-endosomal trafficking system in facilitating molecular interactions for TORC1-regulated signal transduction events in response to nutritional cues. This model is well supported by studies demonstrating that TORC1 components are localized to endosomal and prevacuolar compartments (reviewed in ref. Citation18). Thus, TORC1 joins an emerging number of pathways that employ the vesicle-endosomal system as a staging platform for signaling.Citation19 These findings also reveal further insight into TORC1 signaling and rapamycin action and suggest analogous mechanisms might operate in more complex eukaryotic organisms, including humans, in which Vps proteins and TORC1 orthologs are conserved.
Figures and Tables
Figure 1 NCR gene expression in response to poor nitrogen source or rapamycin is governed by distinct signaling circuits. (A) Upon shift from a rich to a poor nitrogen source, free Gln3-Ure2 complexes associate with Golgi-derived vesicles to be transported to endosomes or a later compartment where Tap42-Sit4 complexes are membrane-tethered via TORC1. This trafficking step results in Gln3 dephosphorylation, Gln3-Ure2 complex dissociation, and Gln3 nuclear import. (B) Rapamycin-induced Gln3 nuclear translocation bypasses Golgi-to-endosome trafficking by liberating membrane-tethered Tap42-Sit4 complexes, which then dephosphorylate Gln3 in the cytosol or on Golgi-derived vesicles to enable nuclear import and activation of NCR gene expression.
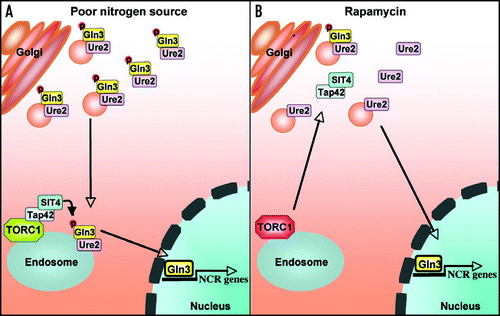
Acknowledgements
We thank Joseph Heitman, Robert Bastidas and Helena Morales-Johansson for comments. This work was supported by National Cancer Institute grant CA114107 (to Maria E. Cardenas).
Addendum to:
References
- Magasanik B, Kaiser CA. Nitrogen regulation in Saccharomyces cerevisiae. Gene 2002; 290:1 - 18
- Beck T, Hall MN. The TOR signalling pathway controls nuclear localization of nutrientregulated transcription factors. Nature 1999; 402:689 - 692
- Cardenas ME, Cutler NS, Lorenz MC, Di Como CJ, Heitman J. The TOR signaling cascade regulates gene expression in response to nutrients. Genes Dev 1999; 13:3271 - 3279
- Hardwick JS, Kuruvilla FG, Tong JK, Shamji AF, Schreiber SL. Rapamycin-modulated transcription defines the subset of nutrient-sensitive signaling pathways directly controlled by the Tor proteins. Proc Natl Acad Sci USA 1999; 96:14866 - 14870
- Bertram PG, Choi JH, Carvalho J, Ai W, Zeng C, Chan TF, Zheng XF. Tripartite regulation of Gln3p by TOR, Ure2p and phosphatases. J Biol Chem 2000; 275:35727 - 35733
- Loewith R, Jacinto E, Wullschleger S, Lorberg A, Crespo JL, Bonenfant D, Oppliger W, Jenoe P, Hall MN. Two TOR complexes, only one of which is rapamycin sensitive, have distinct roles in cell growth control. Mol Cell 2002; 10:457 - 468
- Yan G, Shen X, Jiang Y. Rapamycin activates Tap42-associated phosphatases by abrogating their association with Tor complex 1. EMBO J 2006; 25:3546 - 3555
- Di Como CJ, Jiang Y. The association of Tap42 phosphatase complexes with TORC1: another level of regulation in Tor signaling. Cell Cycle 2006; 5:2729 - 2732
- Cox KH, Kulkarni A, Tate JJ, Cooper TG. Gln3 phosphorylation and intracellular localization in nutrient limitation and starvation differ from those generated by rapamycin inhibition of Tor1/2 in Saccharomyces cerevisiae. J Biol Chem 2004; 279:10270 - 10278
- Tate JJ, Feller A, Dubois E, Cooper TG. Saccharomyces cerevisiae Sit4 phosphatase is active irrespective of the nitrogen source provided, and Gln3 phosphorylation levels become nitrogen source-responsive in a sit4-deleted strain. J Biol Chem 2006; 281:37980 - 37992
- Srivastava A, Woolford CA, Jones EW. Pep3p/Pep5p complex: a putative docking factor at multiple steps of vesicular transport to the vacuole of Saccharomyces cerevisiae. Genetics 2000; 156:105 - 122
- Peterson MR, Emr SD. The class C Vps complex functions at multiple stages of the vacuolar transport pathway. Traffic 2001; 2:476 - 486
- Kitamoto K, Yoshizawa K, Ohsumi Y, Anraku Y. Mutants of Saccharomyces cerevisiae with defective vacuolar function. J Bacteriol 1988; 170:2687 - 2691
- Raymond CK, Howald-Stevenson I, Vater CA, Stevens TH. Morphological classification of the yeast vacuolar protein sorting mutants: evidence for a prevacuolar compartment in class E vps mutants. Mol Biol Cell 1992; 3:1389 - 1402
- Zurita-Martinez SA, Puria R, Pan X, Boeke JD, Cardenas ME. Efficient Tor signaling requires a functional class C Vps protein complex in Saccharomyces cerevisiae. Genetics 2007; 176:2139 - 2150
- Puria R, Zurita-Martinez SA, Cardenas ME. Nuclear translocation of Gln3 in response to nutrient signals requires Golgi-to-endosome trafficking in Saccharomyces cerevisiae. Proc Natl Acad Sci USA 2008; 105:7194 - 7199
- Urban J, Soulard A, Huber A, Lippman S, Mukhopadhyay D, Deloche O, Wanke V, Anrather D, Ammerer G, Riezman H, et al. Sch9 is a major target of TORC1 in Saccharomyces cerevisiae. Mol Cell 2007; 26:663 - 674
- Rohde JR, Bastidas R, Puria R, Cardenas ME. Nutritional control via Tor signaling in Saccharomyces cerevisiae. Curr Opin Microbiol 2008; 11:153 - 160
- Mitchell AP. A VAST staging area for regulatory proteins. Proc Natl Acad Sci USA 2008; 105:7111 - 7112