Abstract
Cytokinesis, the final step of cell division, normally proceeds to completion in living organisms, so that daughter cells physically separate by abscission. In certain tissues and developmental stages, on the other hand, the cytokinesis process is incomplete, giving rise to cells interconnected in syncytia by stable intercellular bridges. This evolutionarily conserved physiological process occurs in the female and male germline in species ranging from insects to humans, and has also been observed in some somatic tissues in invertebrates. Stable intercellular bridges have fascinated cell biologists ever since they were first described more than 50 years ago, and even though substantial progress has been made concerning their ultrastructure and molecular composition, much remains to be understood about their biological functions. Another major question is by which mechanisms complete versus incomplete cytokinesis is determined. In this mini-review we will try to give an overview of the current knowledge about the structure, composition and functions of stable intercellular bridges, and discuss recent insights into the molecular control of the incomplete cytokinesis process.
Introduction
Cytokinesis is the final step of cell division that leads to the physical separation of the two daughter cells.Citation1–Citation3 Cytokinesis normally proceeds through sequential steps of cleavage plane specification between the segregated chromosomes, formation and constriction of an actomyosin-based contractile ring, stabilization of the intercellular bridge and finally completion of the cytokinesis process by abscission.Citation1,Citation2,Citation4–Citation7 These processes are governed by a dynamic interplay between the actomyosin cytoskeleton, the mitotic microtubule spindle, membrane trafficking and fusion.Citation1–Citation4,Citation8
During the development of certain tissues, on the other hand, the cytokinesis process is incomplete, manifested by arrest of cleavage furrows and their transformation into stable intercellular bridges that interconnect cells in syncytia.Citation9–Citation12 Stable intercellular bridges are found in the female and male germline in metazoans ranging from insects to humans and have also been described in certain somatic tissues in invertebrates ().Citation9–Citation15 Plasmodesmata in plants and the septal pore in fungi also represent intercellular bridges formed by incomplete cytokinesis.Citation11,Citation16 The cytoplasmic bridges that form by incomplete cytokinesis are large enough (diameter range of ∼0.2–10 µm, see ) to allow organelles and/or macromolecules to pass.Citation11 This thus represents a very intimate and direct type of intercellular communication and complements the multitude of ways by which cells communicate during the development of multi-cellular organisms, e.g., by signaling via diffusible ligands, cell-cell adhesion, gap junctions or by other types of cytoplasmic connections (such as tunneling nanotubes in animal cells and epithelial bridges between human bronchial epithelial cells that form via projections of filopodia-like extensions between neighboring cells that are remodeled to intercellular tubes).Citation17,Citation18
Since the first descriptions of stable intercellular bridges significant progress has been made concerning their ultrastructure and molecular composition. However, their biological functions and the molecular mechanisms that determine complete versus incomplete cytokinesis remain largely unknown. Here, we will give an overview of the current understanding of these aspects of metazoan stable intercellular bridges and provide insight into recent advances in understanding how incomplete cytokinesis is achieved. We will focus on stable intercellular bridges in Drosophila melanogaster, since they have yet been most thoroughly characterized, but also cover important aspects of mammalian stable intercellular bridges.
Germline Intercellular Bridges
During gametogenesis in both females and males in diverse invertebrate and vertebrate species, gametes undergo incomplete cytokinesis to form clusters of germ cells interconnected by stable intercellular bridges (). The evolutionary conservation of incomplete cytokinesis in the germline suggests that this germ cell interconnection is important, and indeed stable intercellular bridges have been shown to be required for fertility in insects and mammals (see details below).Citation11,Citation12,Citation19,Citation20
Female germline intercellular bridges.
Stable intercellular bridges that interconnect germ cells in female germline cysts form during oogenesis in species ranging from insects to mammals ( and ).Citation12,Citation15,Citation21 The functions of the syncytial interconnection of germ cells in the mammalian female germline are not well understood, but has been proposed to be important for synchronization of mitotic divisions and entry into meiosis, and possibly coordination of programmed cell death or selective degeneration of oocytes.Citation22–Citation25 Strikingly, however, recent studies in mouse have indicated that the bridges are not required for female fertility.Citation26 In many insects, the germ cell intercellular bridges allow the directional transport of nutrients between cells in a syncytium to promote the growth of one of the cells that will develop into the oocyte, whereas the others, after contributing their cytoplasmic contents to the oocyte, retract and die ().Citation12,Citation27
The functions, formation and composition of stable intercellular bridges in the female germline are especially well characterized in Drosophila melanogaster, where the intercellular bridges are called ring canals (). In order to illustrate the processes of incomplete cytokinesis, ring canal formation and ring canal functions in the Drosophila female ovary we will first give an overview of Drosophila oogenesis and then summarize the key steps of incomplete cytokinesis and ring canal formation. More detailed descriptions are found in a number of excellent reviews and original articles ().Citation12,Citation28
Oogenesis is a continuous process in Drosophila femalesCitation29 (). Each ovary consists of 16–20 ovarioles, each of which is a single egg assembly line with egg chambers of increasing age and size from the anterior towards the posterior (). The egg chamber is the structural and functional unit of the ovary, and consists of 16 germ cells; 1 oocyte and 15 nutritive cells called nurse cells that are surrounded by a single layer of somatically derived epithelial follicle cells ( and B). Egg chambers are classified into 14 developmental stages based on their morphology. Stage 1 represents the 16-cell syncytium immediately after encapsulation by the follicle cells while stage 14 refers to the mature egg where eggshell is completely formed and the nurse cells have degenerated. As described above, the nurse cells provide the developing oocyte with organelles, proteins and RNA through the ring canals, a process required for normal oocyte development and female fertilityCitation12 (). The epithelial follicle cells, on the other hand, participate in the oocyte polarity specification and in the secretion of the egg shell during the late stages of oogenesis, a process enhancing survival of the embryo.Citation30
The Drosophila female germ stem cells, located in the germarium at the anterior tip of each ovariole, divide asymmetrically by complete cytokinesis to give rise to another stem cell and a cystoblast ().Citation12,Citation29 The cystoblasts in turn undergo four synchronized rounds of mitosis via incomplete cytokinesis, giving rise to a cluster of 16 germ cells interconnected by 15 arrested cleavage furrows ().Citation28,Citation31 The cleavage furrow arrest is accompanied by the appearance of an electron dense outer rim linked to the plasma membrane.Citation28,Citation32–Citation34 Another marker of constriction arrest is the formation of a plug of the fusome inside the ring.Citation28 The fusome is a vesiculated organelle that forms a branching network reaching through each intercellular bridge of dividing germ cell cysts and is involved in oocyte specification.Citation35 After completion of the fourth mitotic division, the fusome starts degrading and the intercellular bridges acquire a less electron dense inner rim consisting of actin filaments.Citation32,Citation36,Citation37 Well after the fourth division, ring canals start expanding in size and grow from a diameter of about 0.5–1.5 µm in the germarium to approximately 10 µm at late stages of oogenesis.Citation12,Citation28 Thus, it is only after completion of cleavage furrow arrest and the fourth mitotic division that ring canal maturation begins.Citation28 Therefore, incomplete cytokinesis and ring canal growth are two separate processes, both temporally and spatially, and the recent detailed mapping of these processes will allow detailed deciphering of the incomplete cytokinesis process in the Drosophila female germline by genetic means.Citation28
Cleavage furrow arrest, ring canal assembly and growth occur by sequential recruitment and removal of a number of identified components, the timing of which can be estimated from the defined regions of the germarium (regions 1, 2a, 2b and 3) (). Region 1 contains germline stem cells and mitotically active 2-, 4- and 8-cell cysts (). In this region, the cleavage furrows contain contractile ring actin, the actomyosin-binding protein Anillin, the centralspindlin component Pav-Klp, the glycoprotein mucin-D and the multi-adaptor protein Cindr.Citation12,Citation14,Citation38–Citation40 In late region 1, after the third mitotic division, phosphotyrosine epitopes appear in the cleavage furrows.Citation34 Region 2a contains the 16-cell clusters, and here, upon cleavage furrow arrest, contractile ring actin and Cindr disappear.Citation12,Citation14 At this point Anillin, Pav-Klp, phosphotyrosine epitopes, and possibly further components, build up the outer rim of the nascent ring canal.Citation12 After completion of all four mitotic divisions, the inner rim of the maturing ring canals recruit non-contractile actin filaments, the adducin-like protein Ovhts-RC and the filamin Cheerio.Citation12,Citation36 Phosphotyrosine epitopes, mainly originating from phosphorylation by the Src64 and Tec29 kinases, also become detected in the inner rim at this point.Citation12 In region 2b the cysts flatten, become lens-shaped and surrounded by follicle cells. Region 3 contains spherical stage 1 egg chambers that will leave the germarium and enter the vitellarium for maturation. At this step, Anillin disappears and the actin-bundling protein Kelch begins to localize.Citation12,Citation38 Throughout the continued ring canal growth, Ovhts-RC, Cheerio and Kelch play key roles in promoting bundling of the actin filaments at the inner rim of the ring canal.Citation12 Thus, mature ring canals in the female germline are built of both components of the cleavage furrow (Pav-Klp) and further structural components added after cleavage furrow arrest (e.g., F-actin, Ovhts-RC, Cheerio, Kelch).
Male germline intercellular bridges.
Spermatogenesis continues throughout life in the male germline and depends on the continuous production of germline stem cells.Citation41,Citation42 Male germline stem cells divide asymmetrically to give rise to another stem cell and a spermatogonial cell that undergoes mitotic proliferation to form primary spermatocytes. Each spermatocyte in turn undergoes two rounds of meiosis to produce four sperm. During both the mitotic and meiotic cell divisions, cytokinesis is incomplete, giving rise to syncytia of cells interconnected by stable intercellular bridges (). Interestingly, the mitotic and meiotic cell divisions occur synchronously. In mammals, germ cells form syncytia of hundreds of germ cells interconnected by intercellular bridges and in Drosophila melanogaster each cyst consists of 64 haploid spermatids.Citation42–Citation44
Functionally, it has been proposed that male germline intercellular bridges promote germ cell communication and sharing of cytoplasmic constituents, thereby synchronizing e.g., mitotic cell divisions and entry into meiosis ().Citation10,Citation45–Citation48 Studies of the mouse male germline have shown that the cytoplasmic bridges may facilitate the sharing of gene products between post-meiotic haploid spermatids so that genetically distinct spermatids remain phenotypically diploid.Citation47,Citation48 Interestingly, transport of mRNA and organelles has been observed between haploid spermatids.Citation48–Citation50 Specifically, the chromatoid body, an organelle controlling RNA processing in haploid spermatids has been shown to move across male germline intercellular bridges in a microtubule-dependent manner.Citation50 Even though a deeper understanding of their physiological roles is still required,Citation46 the importance of male germ cell intercellular bridges is emphasized by the fact that they are required for fertility of insects and mammals.Citation19,Citation20 Female germline intercellular bridges, on the other hand, are required for fertility in Drosophila, but not in mice.Citation12,Citation26
The molecular composition of male germline intercellular bridges has been most thoroughly studied in Drosophila and mouse. Drosophila male germline intercellular bridges contain phosphotyrosine epitopes, Anillin, Pav-Klp and at least three septins (Peanut, Septin 1 and Septin 2).Citation13 Male ring canals do not, on the other hand, contain a thick inner rim of filamentous actin, nor Ovhts-RC or Kelch, major components of female ring canals, nor do they grow in size, but rather remain at a diameter of 1–1.5 µm.Citation13,Citation51 Interestingly, in contrast to the Drosophila female germline, the fusome persists and develops further even after the cease of the mitotic divisions in the male germ cell clusters.Citation13,Citation35
In the mouse male germline, Anillin and several septins (at least 2, 7 and 9) are transiently present in the forming intercellular bridges, but are eventually absent from the mature bridges.Citation52 The mature intercellular bridges contain the testis-expressed gene 14 (TEX14, that shows similarity to Drosophila Src64 and Tec29 in its kinase domain), the two centralspindlin components MKLP1 (Pav-Klp in Drosophila) and MgcRacGAP, the centrosomal proteins CEP55 and pericentrin, as well as e.g., actin, plectin and δ-tubulin.Citation52–Citation54 Thus, Drosophila and mouse male germline intercellular bridges, as well as female and male ring canals in Drosophila are, at least partially, compositionally different, suggesting that there may be a variation in the ways they form.
Somatic Intercellular Bridges
Somatic stable intercellular bridges have been described in Caenorhabditis elegans, hydra and insects ().Citation14,Citation45,Citation55–Citation59 Several insects have apically localized stable intercellular bridges in the ovarian follicle cell epithelium ( and ).Citation14,Citation56–Citation60 In Drosophila, somatic stable intercellular bridges have also been observed in the larval imaginal disc epithelia ( and )Citation14,Citation40,Citation58 and in the larval brain.Citation14,Citation40 Cytoplasmic bridges of a more transient nature are found in the cellular blastoderm and extended germ band embryo.Citation11
To date, the functions of somatic intercellular bridges remain unknown. It is believed, by analogy to the functions of germline intercellular bridges, that they promote exchange of cytoplasm and maybe even organelles, thereby facilitating intercellular communication, synchronization of cell division or differentiation, and coordination of cell behavior.Citation11,Citation14,Citation40,Citation56–Citation58 Studies of the Drosophila follicle cell epithelium by injections of a fluorescent dye into a single follicle cell indeed revealed the presence of follicle cell clusters, in line with the cytoplasmic continuity observed by electron microscopy.Citation57 Many such independent clusters build up the follicle cell epithelium. Interestingly, these clusters do not show a uniform morphology, sometimes occurring in linear arrays or with asymmetric branching, suggesting that cell divisions by incomplete cytokinesis in the follicle cell epithelium are not as tightly synchronized as in the germline.Citation57,Citation60 It will be essential to elucidate the roles of somatic stable intercellular bridges during development and the identity of the signals that may be shared within somatic cell clusters, as well as whether stable somatic intercellular bridges exist in mammals.
Only a handful of proteins have yet been identified as constituents of somatic stable intercellular bridges in Drosophila. These include Anillin, Pav-Klp, Cindr and mucin-D, all of which are found in intercellular bridges in the egg chamber follicle cell and larval imaginal disc epithelia, as well as in the larval brain ().Citation14,Citation35,Citation39,Citation40
Ultrastructure of Intercellular Bridges
Female and male germline.
Detailed analyses of the ultrastructure of the ring canals have been performed only in the female germline in Drosophila melanogaster.Citation32,Citation61 The ring canals consist of two rims: An outer rim that appears electron dense in electron micrographs and an inner thicker rim that is less electron denseCitation61 (similar in the olive fruit fly Dacus oleae; ). Horizontal thin sections through the ring canal and decoration with subfragment 1 of myosin reveal that the inner rim consists of a layer of circumferentially oriented actin filaments attached to the plasma membrane at the periphery of each canal. The actin filaments in the ring are of mixed polarities, similar to those found in the contractile ring formed during cytokinesis. In vertical sections through the canal the actin filaments appear as dense dots.Citation61 As the ring canal diameter increases during oogenesis (from about 0.5 µm at stage 2 to about 10 µm at late stages), the thickness of the ring canal wall increases (from 0.06 µm at stage 2 to 0.37 µm at stage 9) and the ring canal length, too (from 0.22 µm at stage 2 to 1.87 µm at stage 9). At developmental stage 2 there are 82 actin filaments in the ring, by stage 6 there are 717 and by stage 10 there are 726. Taking into account the diameter, this indicates that there are 170 µm of actin filaments/canal at stage 2, 14,000 µm at stage 9 and approximately 23,000 µm at stage 11 or one inch of actin filament.Citation61 The density of actin filaments remains unchanged throughout development. Studies in mutant flies that lack the ring canal component Kelch revealed that actin filaments form normally but individual bundles that comprise the fibers of the net are not bound tightly together. This results in bundles to enter into the ring canal lumen, partially blocking the canal.Citation61
In vertebrates, the ultrastructure of ring canals in the female germline has been described in frog, chicken, rabbit, mouse, rat, hamster and human ovaries ().Citation21–Citation23,Citation62–Citation64 Mammalian female ring canals consist mainly of one rim that appears electron dense in electron micrographs. A second less electron dense inner rim, as evident in Drosophila, is not clearly seen in electron micrographs of mammalian female germline ring canals. Their diameter is on average 0.5 to 1 µm.Citation21,Citation23
The ultrastructure of stable intercellular bridges in the male germline has not yet been described in as much detail as for the bridges found in female germline. However, Drosophila male germline intercellular bridges consist mainly of one rim that appears electron dense in electron micrographs and they have a diameter of 1–1.5 µm ().Citation51 In mammals, the ultrastructural morphology of male ring canals has been described in mouse, rat, guinea pig, Chinese hamster, squirrel, rabbit, cat, goat, monkey and human testis.Citation9,Citation19,Citation44,Citation45 Interestingly, they also consist mainly of one electron dense rim and have a diameter of µ1–1.5 m.Citation9,Citation19,Citation44,Citation45
Somatic intercellular bridges.
Most information about the detailed morphology of somatic stable intercellular bridges comes from studies in insects and especially from Drosophila.Citation14,Citation56–Citation60 Somatic stable intercellular bridges found in the ovarian follicle cell epithelia and the epithelial cells of the larval imaginal discs all have a stable diameter of about 0.25 to 1 µm and they do not grow during development ().Citation14,Citation57,Citation58 In the follicular epithelium of Drosophila ovarian follicles, the bridges have a cylinder shape and an open lumen filled with cytoplasm that is continuous with both cells and they have an average luminal diameter of 0.25–0.3 µm and a length of 0.40 µm, which is smaller than that of ring canals in the female germline (). Their wall consists of an outer and an inner rim. The outer rim is more electrondense compared to the inner rim that appears filamentous.Citation57 By decoration with the S1 fragment of myosin, these filaments have been confirmed as actin filaments.Citation57 Stable intercellular bridges observed in the epithelial cells of the Drosophila imaginal discs exhibit a very similar ultrastructural morphology ().Citation14,Citation58
Molecular Mechanisms of Incomplete Cytokinesis
By which molecular mechanisms does the cytokinesis process halt during incomplete cytokinesis as opposed to conventional cytokinesis that proceeds to abscission? In the next paragraphs we will give an overview of specific features of stable intercellular bridges and some recent advances in the field that have shed light on the molecular control of the incomplete cytokinesis process.
Specific morphological features of stable intercellular bridges.
As evident from the ultrastructural studies of both germline and somatic intercellular bridges, the formation of a stable intercellular bridge is accompanied by the appearance of an electron dense rim just beneath the plasma membrane.Citation14,Citation19,Citation32,Citation44,Citation57,Citation61 One possibility is that this rim protects the plasma membrane of the intercellular bridge from membrane remodeling and vesicle fusion events that normally promote cytokinesis completion and abscission, thereby stabilizing the bridge.Citation2,Citation8 Interestingly, stable intercellular bridges indeed have smooth bridge walls, as opposed to the transient intercellular bridges during conventional cytokinesis which display membrane foldings and protrusions.Citation14,Citation57 The protrusions indicate active membrane remodeling prior to abscission of these transient intercellular bridges.Citation14,Citation65
Another characteristic of mature stable intercellular bridges is the absence of mitotic microtubule spindle bundles in their lumen.Citation14,Citation44,Citation52,Citation57,Citation66 The mitotic microtubule spindle is required for cytokinesis progression during conventional cytokinesis.Citation2 Electron microscopy studies of mammalian male germ cell intercellular bridges show that germ cell cytokinesis initially appears identical to conventional cytokinesis, but eventually the microtubule spindle and midbody break down.Citation44,Citation66 Similarly, stable somatic intercellular bridges in the Drosophila follicle cell and larval imaginal disc epithelia, as well as in the larval brain, do not contain visible microtubule bundles in their bridge lumen.Citation14,Citation56–Citation58 Interestingly, actively dividing cells in the same tissues, on the other hand, such as larval neuroblasts that will eventually undergo abscission, display very prominent microtubule spindles.Citation2,Citation14 Moreover, ovarian follicle cells that undergo complete cytokinesis until developmental stage 6 of oogenesis display mitotic spindles in their lumen.Citation57 In contrast, after stage 6, when stable intercellular bridges have formed, and until the end of oogenesis, microtubule bundles are not any more detected within their lumen.Citation14,Citation57 Of note is that microtubules may still be involved in intercellular transport, as evident in the female and male germline in insects and mammals.Citation12,Citation50 A deeper understanding of the formation, composition and functions of the electron dense bridge rim, the mechanism by which the mitotic spindle and midbody break down and their possible cross-talk may give important clues to the control of the incomplete cytokinesis process.
Halting constriction of the contractile ring.
Evidence for arrest of the actomyosin ring constriction as a mechanism of incomplete cytokinesis has recently been described in the Drosophila female germline. An essential motor for contractile ring constriction is non-muscle myosin II (hereafter referred to as myosin II). Phosphorylation of the regulatory myosin II light chains activates myosin II and thereby contractile ring constriction, whereas their dephosphorylation by myosin light chain phosphatase (MLCP) negatively regulates myosin II-mediated contraction.Citation67 Recent studies of the incomplete cytokinesis process in the germarium of the Drosophila female germline have shown that inactivation of MLCP phosphatase activity by mutation of its myosin-binding subunit MYPT leads to contractile ring over-constriction during incomplete cytokinesis in the germarium and thereby formation of small ring canals.Citation68,Citation69 These data suggest that dephosphorylation of myosin II is a prerequisite for contractile ring arrest and thereby incomplete cytokinesis in the Drosophila female germline. Whether similar mechanisms apply during the formation of other stable intercellular bridge types remains to be determined.
Stabilization of the intercellular bridge.
Clearly, stabilization of the intercellular bridge contributes to the maintenance of incomplete cytokinesis. In the Drosophila female germline, one molecular explanation of the cleavage furrow arrest was described above.Citation69 Subsequently, during ring canal assembly and growth, their structural burden is carried largely by the thick actin bundles, Ov-htsRC and Kelch at the inner rim of the bridge.Citation12,Citation61 In the Drosophila male germline ring canals, on the other hand, thick actin bundles are not evident.Citation13,Citation57 Here, members of the septin family may contribute to stabilizing the intercellular bridge.Citation13 Septins are components of the stable bud neck in yeast and form filamentous oligomeric ring-like arrays beneath the plasma mem- brane.Citation70,Citation71 Septins are missing from ring canals in the Drosophila female germline, but have been proposed to be components of somatic intercellular bridges.Citation11,Citation72 Moreover, somatic intercellular bridges have a monolayer of actin filaments at their inner rim that by analogy to female germline intercellular bridges may contribute to their structural stability.Citation14,Citation57 Interestingly, during conventional cytokinesis, actin disassembly is a prerequisite for abscission,Citation4 and in human cells with chromosome bridges, actin has been shown to accumulate to stabilize intercellular bridges in order to delay abscission until chromosome segregation has been completed.Citation73
Other components that may play a role in intercellular bridge stabilization include Anillin, Cindr and MKLP1/Pav-Klp. Anillin, a stable component of both Drosophila male germline and somatic stable intercellular bridges,Citation13,Citation14,Citation35,Citation38,Citation74 acts at several steps of the cytokinesis process,Citation75 and has been proposed to be involved in intercellular bridge stabilization during conventional cell divisions and incomplete cytokinesis.Citation14,Citation76,Citation77 Drosophila Anillin was recently found to interact with Cindr which is a component of cleavage furrows during female germline cell divisions and of stable somatic intercellular bridges.Citation14 Together, Anillin and Cindr may play a role in stabilizing actin filaments at the inner rim of somatic intercellular bridges.Citation14 Moreover, the fact that MKLP1/Pav-Klp represents a conserved component of all stable intercellular bridge types with known molecular composition ()Citation14,Citation26,Citation39,Citation52,Citation78 may suggest that it plays a role in stabilizing them. Interestingly, MKLP1/Pav-Klp is required for the formation of the electron dense midbody matrix during cytokinesis, and electron microscopy studies implicate that there may be a relationship between the midbody matrix and the electron dense rim of stable intercellular bridges.Citation65,Citation79 It will be exciting to learn more about the mode of action of these proteins, other intercellular bridge components, and their effectors during incomplete cytokinesis.
Inhibition of abscission.
A further mechanism that may contribute to promoting incomplete cytokinesis is controlled inhibition of the abscission process. Even though much still needs to be understood about the detailed molecular requirements for cytokinetic abscission, some important aspects have been discovered.Citation1,Citation2,Citation4,Citation6 Targeted fusion of vesicles derived from the Golgi, endosomes or recycling endosomes to the intercellular bridge, and membrane docking factors, are required.Citation4,Citation8,Citation80–Citation82 Centrosomal proteins, such as centriolin, CEP55, FYVE-CENT and TTC19, also participate in cytokinesis completion.Citation80,Citation83–Citation85 Centriolin and CEP55 recruit factors required for abscission, including SNAREs and components of the exocyst complex (recruited by centriolin),Citation80 the endosomal sorting complex required for transport (ESCRT) (recruited by CEP55) and ALIX (recruited by CEP55).Citation83,Citation84,Citation86 Specifically, CEP55 has been shown to recruit the ESCRT-I component TSG101, and ALIX, to the midbody via interactions between its hinge region and GPPX3Y motifs present in TSG101 and ALIX, to promote recruitment of ESCRT-III components and thereby abscission.Citation83,Citation84 It can be speculated that compartmentalized absence of active abscission factors, e.g., by developmentally regulated expression, their inactivation or by inhibition of their recruitment, may contribute to incomplete cytokinesis. Interestingly, such a case was recently described in the mouse male germline. These recent studies have shown that the intercellular bridge component TEX14 also contains a GPPX3Y motif, with which CEP55 strongly interacts, thereby inhibiting the interactions of CEP55 with TSG101 and ALIX.Citation53 This suggests that TEX14 prevents the completion of cytokinesis, including the localization of ALIX, by altering the fate of CEP55, from a midbody organizer that recruits proteins for abscission, to a major component of the stable intercellular bridge.Citation53 Thus, when TEX14 is bound to CEP55, TSG101 and ALIX are not recruited to the midbody to complete cytokinesis.Citation53 In this way, TEX14 plays an important role in promoting incomplete cytokinesis in the mouse male germline.
Hence, incomplete cytokinesis may be accomplished by mechanisms acting at multiple steps, including arrest of the actomyosin ring, structural stabilization of the intercellular bridge, disassembly of the microtubule spindle, inhibition of membrane fusion events, as well as of abscission. Given some morphological and compositional differences between female germline, male germline and somatic stable intercellular bridges, it will be interesting to learn what may be the conserved and specific features of the incomplete cytokinesis process when comparing tissue types and species.
Concluding Remarks and Perspectives
Since the first ultrastructural studies of stable intercellular bridges, we now have a greater understanding of their molecular composition and some insight into their functions and the molecular control of complete versus incomplete cytokinesis. However, questions still remain as to the physiological and developmental roles of stable intercellular bridges, to the identity of factors and signals that are exchanged, to how they can coordinate cell behavior in a syncytium, as well as to the temporal, spatial and molecular control of the incomplete cytokinesis process. Future challenges in the field include answering these questions. The available knowledge and scientific interest in the topic as well as the rapid development of advanced molecular genetics and imaging techniques ensure that the understanding of these issues will grow at a fast pace. A deeper understanding of the incomplete cytokinesis process may also give further insights into the control of abscission initiation during conventional cytokinesis.
Abbreviations
ESCRT | = | endosomal sorting complex required for transport |
TEX14 | = | testis-expressed gene 14 |
Drosophila | = | Drosophila melanogaster |
Figures and Tables
Figure 1 Stable intercellular bridges in the Drosophila melanogaster female germline. (A) Schematic overview of Drosophila oogenesis, the germarium and incomplete cytokinesis of germ cells in the germarium. Cleavage furrows and ring canals are schematically shown in black. Details are described in the text. (B) Confocal micrograph of an early stage 9 wild-type Drosophila melanogaster egg chamber stained with phalloidin-FITC (fluorescein isothiocyanate) (green) and propidium iodide (red) for visualizing filamentous actin and nuclei, respectively. Scale bar: 35 µm. (C) Confocal micrograph of a late stage 9 wild-type Drosophila melanogaster egg chamber stained with phalloidin-FITC for visualizing filamentous actin. The image is a projection of a z-stack of 18 serial sections of 2 µm thickness each. Scale bar: 15 µm. (D) Light micrograph of a 1 µm thick resin section stained with toluidine blue. It illustrates the anterior pole of a wild-type stage 11 Drosophila melanogaster egg chamber. Bipolar arrows: Ring canals, (F) follicle cell, (N) nurse cell. Scale bar: 10 µm. (E–G) Transmission electron micrographs of a stage 6 wild-type olive fruit fly Dacus oleae egg chamber. (E) illustrates a ring canal in longitudinal section. Arrows point to the ring canal edges. (F and G) are higher magnifications of the ring canal edges shown in (E). Arrows in (F and G) point to ring canal rims. Scale bars: (E) 1 µm; (F and G) 0.5 µm.
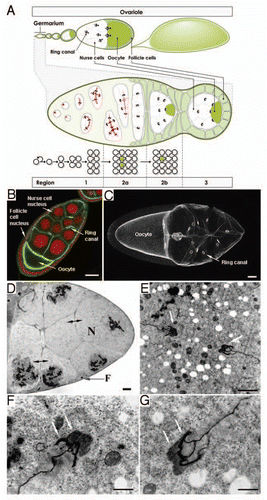
Figure 2 Stable intercellular bridges in somatic Drosophila tissues. (A) Stable intercellular bridges in the Drosophila melanogaster follicle cell epithelium. Left and middle: Cross section through a stage 8 egg chamber (left) and a superficial section through the follicle cell epithelium of the same egg chamber (middle) showing stable intercellular bridges (arrowheads) between cells detected with antibodies against the bridge component Cindr (green). Rhodamine-phalloidin was used to visualize F-actin (red). Scale bars: 10 µm (left) and 5 µm (middle). Right: Electron micrograph showing a longitudinal section through a stable intercellular bridge of a stage 8 egg chamber. The electron dense outer rim is evident (arrowhead) and a less electron dense inner rim (arrow) is also seen. Scale bar: 250 nm. (B) Stable intercellular bridges in the Drosophila melanogaster wing disc epithelium. Left: Stable intercellular bridges (arrowheads) in a wing disc epithelium are labeled with antibodies against the bridge component Cindr. Scale bar: 20 µm. Middle: Stable intercellular bridges (arrowheads) containing Cindr (green) are present at cell borders labeled by Dlg (red). Nuclear DNA was labeled with Hoechst (pseudo-colored blue). Scale bar: 5 µm. Right: Electron micrograph showing a longitudinal section through an intercellular bridge in the wing disc epithelium. The electron dense outer rim (arrowhead) and the less electron dense inner rim (arrow) are evident. Scale bar: 250 nm.
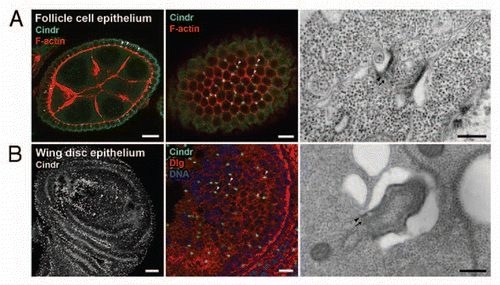
Table 1 Stable intercellular bridges in the germline and in somatic tissues in diverse metazoans
Acknowledgements
K.H. acknowledges support from the International Human Frontier Science Program Organization and The Research Council of Norway. I.P.N. acknowledges support from the European Research Council and H.S. from the Norwegian Cancer Society.
References
- Eggert US, Mitchison TJ, Field CM. Animal cytokinesis: from parts list to mechanisms. Annu Rev Biochem 2006; 75:543 - 566
- Barr FA, Gruneberg U. Cytokinesis: placing and making the final cut. Cell 2007; 131:847 - 860
- Glotzer M. Animal cell cytokinesis. Annu Rev Cell Dev Biol 2001; 17:351 - 386
- Steigemann P, Gerlich DW. Cytokinetic abscission: cellular dynamics at the midbody. Trends Cell Biol 2009; 19:606 - 616
- Schweitzer JK, D'Souza-Schorey C. Finishing the job: cytoskeletal and membrane events bring cytokinesis to an end. Exp Cell Res 2004; 295:1 - 8
- Guizetti J, Gerlich DW. Cytokinetic abscission in animal cells. Semin Cell Dev Biol 2010;
- Pollard TD. Mechanics of cytokinesis in eukaryotes. Curr Opin Cell Biol 2010; 22:50 - 56
- Montagnac G, Echard A, Chavrier P. Endocytic traffic in animal cell cytokinesis. Curr Opin Cell Biol 2008; 20:454 - 461
- Burgos MH, Fawcett DW. Studies on the fine structure of the mammalian testis. I. Differentiation of the spermatids in the cat (Felis domestica). J Biophys Biochem Cytol 1955; 1:287 - 300
- Fawcett DW. Intercellular bridges. Exp Cell Res 1961; 8:174 - 187
- Robinson DN, Cooley L. Stable intercellular bridges in development: the cytoskeleton lining the tunnel. Trends Cell Biol 1996; 6:474 - 479
- Robinson DN, Cooley L. Genetic analysis of the actin cytoskeleton in the Drosophila ovary. Annu Rev Cell Dev Biol 1997; 13:147 - 170
- Hime GR, Brill JA, Fuller MT. Assembly of ring canals in the male germ line from structural components of the contractile ring. J Cell Sci 1996; 109:2779 - 2788
- Haglund K, Nezis IP, Lemus D, Grabbe C, Wesche J, Liestol K, et al. Cindr interacts with anillin to control cytokinesis in Drosophila melanogaster. Curr Biol 2010; 20:944 - 950
- Pepling ME, de Cuevas M, Spradling AC. Germline cysts: a conserved phase of germ cell development?. Trends Cell Biol 1999; 9:257 - 262
- Lucas WJ, Wolf S. Plasmodesmata: the intercellular organelles of green plants. Trends Cell Biol 1993; 3:308 - 315
- Baluska F, Hlavacka A, Volkmann D, Menzel D. Getting connected: actin-based cell-to-cell channels in plants and animals. Trends Cell Biol 2004; 14:404 - 408
- Zani BG, Edelman ER. Cellular bridges: Routes for intercellular communication and cell migration. Commun Integr Biol 2010; 3:215 - 220
- Greenbaum MP, Yan W, Wu MH, Lin YN, Agno JE, Sharma M, et al. TEX14 is essential for intercellular bridges and fertility in male mice. Proc Natl Acad Sci USA 2006; 103:4982 - 4987
- Brill JA, Hime GR, Scharer-Schuksz M, Fuller MT. A phospholipid kinase regulates actin organization and intercellular bridge formation during germline cytokinesis. Development 2000; 127:3855 - 3864
- Pepling ME, Spradling AC. Female mouse germ cells form synchronously dividing cysts. Development 1998; 125:3323 - 3328
- Zamboni L, Gondos B. Intercellular bridges and synchronization of germ cell differentiation during oogenesis in the rabbit. J Cell Biol 1968; 36:276 - 282
- Gondos B. Germ cell degeneration and intercellular bridges in the human fetal ovary. Z Zellforsch Mikrosk Anat 1973; 138:23 - 30
- Franchi LL, Mandl AM. The ultrastructure of oogonia and oocytes in the foetal and neonatal rat. Proc R Soc Lond B Biol Sci 1962; 99 - 114
- Gondos B. Intercellular bridges and mammalian germ cell differentiation. Differentiation 1973; 1:177 - 182
- Greenbaum MP, Iwamori N, Agno JE, Matzuk MM. Mouse TEX14 is required for embryonic germ cell intercellular bridges but not female fertility. Biol Reprod 2009; 80:449 - 457
- King RC, Mills RP. Oogenesis in adult Drosophila XI. Studies of some organelles of the nutrient stream in egg chambers of D. melanogaster and D. willistoni. Growth 1962; 26:235 - 253
- Ong S, Tan C. Germline cyst formation and incomplete cytokinesis during Drosophila melanogaster oogenesis. Dev Biol 2010; 337:84 - 98
- Horne-Badovinac S, Bilder D. Mass transit: epithelial morphogenesis in the Drosophila egg chamber. Dev Dyn 2005; 232:559 - 574
- Trougakos IP, Margaritis LH. Hilker M, Meiners T. Novel morphological and physiological aspects of insect eggs. Chemoecology of Insect Eggs and Egg Deposition 2002; Berlin Blackwell Wissenschaftsverlag
- de Cuevas M, Lilly MA, Spradling AC. Germline cyst formation in Drosophila. Annu Rev Genet 1997; 31:405 - 428
- Yue L, Spradling AC. hu-li tai shao, a gene required for ring canal formation during Drosophila oogenesis, encodes a homolog of adducin. Genes Dev 1992; 6:2443 - 2454
- Mahowald AP. The formation of ring canals by cell furrows in Drosophila. Z Zellforsch Mikrosk Anat 1971; 118:162 - 167
- Robinson DN, Cant K, Cooley L. Morphogenesis of Drosophila ovarian ring canals. Development 1994; 120:2015 - 2025
- de Cuevas M, Spradling AC. Morphogenesis of the Drosophila fusome and its implications for oocyte specification. Development 1998; 125:2781 - 2789
- Warn RM, Gutzeit HO, Smith L, Warn A. F-actin rings are associated with the ring canals of the Drosophila egg chamber. Exp Cell Res 1985; 157:355 - 363
- Theurkauf WE, Alberts BM, Jan YN, Jongens TA. A central role for microtubules in the differentiation of Drosophila oocytes. Development 1993; 118:1169 - 1180
- Field CM, Alberts BM. Anillin, a contractile ring protein that cycles from the nucleus to the cell cortex. J Cell Biol 1995; 131:165 - 178
- Minestrini G, Mathe E, Glover DM. Domains of the Pavarotti kinesin-like protein that direct its subcellular distribution: effects of mislocalisation on the tubulin and actin cytoskeleton during Drosophila oogenesis. J Cell Sci 2002; 115:725 - 736
- Kramerova IA, Kramerov AA. Mucinoprotein is a universal constituent of stable intercellular bridges in Drosophila melanogaster germ line and somatic cells. Dev Dyn 1999; 216:349 - 360
- Brinster RL. Male germline stem cells: from mice to men. Science 2007; 316:404 - 405
- Fuller M. Spermatogenesis. The Development of Drosophila melanogaster 1993; 71 - 147
- Huckins C. The spermatogonial stem cell population in adult rats. I. Their morphology, proliferation and maturation. Anat Rec 1971; 169:533 - 557
- Dym M, Fawcett DW. Further observations on the numbers of spermatogonia, spermatocytes and spermatids connected by intercellular bridges in the mammalian testis. Biol Reprod 1971; 4:195 - 215
- Fawcett DW, Ito S, Slautterback D. The occurrence of intercellular bridges in groups of cells exhibiting synchronous differentiation. J Biophys Biochem Cytol 1959; 5:453 - 460
- Guo GQ, Zheng GC. Hypotheses for the functions of intercellular bridges in male germ cell development and its cellular mechanisms. J Theor Biol 2004; 229:139 - 146
- Caldwell KA, Handel MA. Protamine transcript sharing among postmeiotic spermatids. Proc Natl Acad Sci USA 1991; 88:2407 - 2411
- Braun RE, Behringer RR, Peschon JJ, Brinster RL, Palmiter RD. Genetically haploid spermatids are phenotypically diploid. Nature 1989; 337:373 - 376
- Morales CR, Lefrancois S, Chennathukuzhi V, El-Alfy M, Wu X, Yang J, et al. A TB-RBP and Ter ATPase complex accompanies specific mRNAs from nuclei through the nuclear pores and into intercellular bridges in mouse male germ cells. Dev Biol 2002; 246:480 - 494
- Ventela S, Toppari J, Parvinen M. Intercellular organelle traffic through cytoplasmic bridges in early spermatids of the rat: mechanisms of haploid gene product sharing. Mol Biol Cell 2003; 14:2768 - 2780
- Rasmussen SW. Ultrastructural studies of spermatogenesis in Drosophila melanogaster Meigen. Z Zellforsch Mikrosk Anat 1973; 140:125 - 144
- Greenbaum MP, Ma L, Matzuk MM. Conversion of midbodies into germ cell intercellular bridges. Dev Biol 2007; 305:389 - 396
- Iwamori T, Iwamori N, Ma L, Edson MA, Greenbaum MP, Matzuk MM. TEX14 interacts with CEP55 to block cell abscission. Mol Cell Biol 2010; 30:2280 - 2292
- Chang YC, Chen YJ, Wu CH, Wu YC, Yen TC, Ouyang P. Characterization of centrosomal proteins Cep55 and pericentrin in intercellular bridges of mouse testes. J Cell Biochem 2010; 109:1274 - 1285
- Waddle JA, Cooper JA, Waterston RH. Transient localized accumulation of actin in Caenorhabditis elegans blastomeres with oriented asymmetric divisions. Development 1994; 120:2317 - 2328
- Giorgi F. Intercellular bridges in ovarian follicle cells of Drosophila melanogaster. Cell Tissue Res 1978; 186:413 - 422
- Woodruff RI, Tilney LG. Intercellular bridges between epithelial cells in the Drosophila ovarian follicle: a possible aid to localized signaling. Dev Biol 1998; 200:82 - 91
- Poodry CA, Schneiderman HA. Ultrastructure of the developing leg of Drosophila melanogaster. Wilhelm Roux' Archiv 1970; 166:1 - 44
- Ramamurty PS, Engels W. Occurrence of intercellular bridges between follicle epithelial cells in the ovary of Apis mellifica queens. J Cell Sci 1977; 24:195 - 202
- Fiil A. Follicle cell bridges in the mosquito ovary: syncytia formation and bridge morphology. J Cell Sci 1978; 31:137 - 143
- Tilney LG, Tilney MS, Guild GM. Formation of actin filament bundles in the ring canals of developing Drosophila follicles. J Cell Biol 1996; 133:61 - 74
- Franchi LL, Mandl AM. The ultrastructure of germ cells in foetal and neonatal male rats. J Embryol Exp Morphol 1964; 12:289 - 308
- Ukeshima A, Fujimoto T. A fine morphological study of germ cells in asymmetrically developing right and left ovaries of the chick. Anat Rec 1991; 230:378 - 386
- Kloc M, Bilinski S, Dougherty MT, Brey EM, Etkin LD. Formation, architecture and polarity of female germline cyst in Xenopus. Dev Biol 2004; 266:43 - 61
- Mullins JM, Biesele JJ. Terminal phase of cytokinesis in D-98s cells. J Cell Biol 1977; 73:672 - 684
- Weber JE, Russell LD. A study of intercellular bridges during spermatogenesis in the rat. Am J Anat 1987; 180:1 - 24
- Asano S, Hamao K, Hosoya H. Direct evidence for roles of phosphorylated regulatory light chain of myosin II in furrow ingression during cytokinesis in HeLa cells. Genes Cells 2009; 14:555 - 568
- Tan C, Stronach B, Perrimon N. Roles of myosin phosphatase during Drosophila development. Development 2003; 130:671 - 681
- Ong S, Foote C, Tan C. Mutations of DMYPT cause over constriction of contractile rings and ring canals during Drosophila germline cyst formation. Dev Biol 2010; In press
- Field CM, al-Awar O, Rosenblatt J, Wong ML, Alberts B, Mitchison TJ. A purified Drosophila septin complex forms filaments and exhibits GTPase activity. J Cell Biol 1996; 133:605 - 616
- Chant J. Septin scaffolds and cleavage planes in Saccharomyces. Cell 1996; 84:187 - 190
- Neufeld TP, Rubin GM. The Drosophila peanut gene is required for cytokinesis and encodes a protein similar to yeast putative bud neck filament proteins. Cell 1994; 77:371 - 379
- Steigemann P, Wurzenberger C, Schmitz MH, Held M, Guizetti J, Maar S, Gerlich DW. Aurora B-mediated abscission checkpoint protects against tetraploidization. Cell 2009; 136:473 - 484
- Giansanti MG, Bonaccorsi S, Gatti M. The role of anillin in meiotic cytokinesis of Drosophila males. J Cell Sci 1999; 112:2323 - 2334
- D'Avino P. How to scaffold the contractile ring for a safe cytokinesis—lessons from Anillin-related proteins. J Cell Sci 2009; 122:1071 - 1079
- Echard A, Hickson GR, Foley E, O'Farrell PH. Terminal cytokinesis events uncovered after an RNAi screen. Curr Biol 2004; 14:1685 - 1693
- Field CM, Coughlin M, Doberstein S, Marty T, Sullivan W. Characterization of anillin mutants reveals essential roles in septin localization and plasma membrane integrity. Development 2005; 132:2849 - 2860
- Carmena M, Riparbelli MG, Minestrini G, Tavares AM, Adams R, Callaini G, et al. Drosophila polo kinase is required for cytokinesis. J Cell Biol 1998; 143:659 - 671
- Matuliene J, Kuriyama R. Kinesin-like protein CHO1 is required for the formation of midbody matrix and the completion of cytokinesis in mammalian cells. Mol Biol Cell 2002; 13:1832 - 1845
- Gromley A, Yeaman C, Rosa J, Redick S, Chen CT, Mirabelle S, et al. Centriolin anchoring of exocyst and SNARE complexes at the midbody is required for secretory-vesicle-mediated abscission. Cell 2005; 123:75 - 87
- Skop AR, Bergmann D, Mohler WA, White JG. Completion of cytokinesis in C. elegans requires a brefeldin A-sensitive membrane accumulation at the cleavage furrow apex. Curr Biol 2001; 11:735 - 746
- Boucrot E, Kirchhausen T. Endosomal recycling controls plasma membrane area during mitosis. Proc Natl Acad Sci USA 2007; 104:7939 - 7944
- Carlton JG, Martin-Serrano J. Parallels between cytokinesis and retroviral budding: a role for the ESCRT machinery. Science 2007; 316:1908 - 1912
- Morita E, Sandrin V, Chung HY, Morham SG, Gygi SP, Rodesch CK, Sundquist WI. Human ESCRT and ALIX proteins interact with proteins of the midbody and function in cytokinesis. EMBO J 2007; 26:4215 - 4227
- Sagona AP, Nezis IP, Pedersen NM, Liestol K, Poulton J, Rusten TE, et al. PtdIns(3)P controls cytokinesis through KIF13A-mediated recruitment of FYVE-CENT to the midbody. Nat Cell Biol 2010; 12:362 - 371
- Raiborg C, Stenmark H. The ESCRT machinery in endosomal sorting of ubiquitylated membrane proteins. Nature 2009; 458:445 - 452
- Gondos B, Bhiraleus P, Hobel CJ. Ultrastructural observations on germ cells in human fetal ovaries. Am J Obstet Gynecol 1971; 110:644 - 652
- Gondos B. Comparative studies of normal and neoplastic ovarian germ cells: 1. Ultrastructure of oogonia and intercellular bridges in the fetal ovary. Int J Gynecol Pathol 1987; 6:114 - 123
- Ruby JR, Dyer RF, Skalko RG. The occurence of intercellular bridges during oogenesis in the mouse. J Morph 1969; 127:307 - 340
- Sokol NS, Cooley L. Drosophila filamin encoded by the cheerio locus is a component of ovarian ring canals. Curr Biol 1999; 9:1221 - 1230
- Roulier EM, Panzer S, Beckendorf SK. The Tec29 tyrosine kinase is required during Drosophila embryogenesis and interacts with Src64 in ring canal development. Mol Cell 1998; 1:819 - 829
- Lu N, Guarnieri DJ, Simon MA. Localization of Tec29 to ring canals is mediated by Src64 and PtdIns(3,4,5)P3-dependent mechanisms. EMBO J 2004; 23:1089 - 1100
- Pyka-Fosciak G, Szklarzewicz T. Germ cell cluster formation and ovariole structure in viviparous and oviparous generations of the aphid Stomaphis quercus. Int J Dev Biol 2008; 52:259 - 265
- Patricio K, Cruz-Landim C. Ultrastructural aspects of the intercellular bridges between female bee germ cells. Braz J Biol = Revista brasleira de biologia 2006; 66:309 - 315
- Gondos B, Hobel CJ. Ultrastructure of germ cell development in the human fetal testis. Z Zellforsch Mikrosk Anat 1971; 119:1 - 20
- Miething A. Intercellular bridges between germ cells in the immature golden hamster testis: evidence for clonal and non-clonal mode of proliferation. Cell Tissue Res 1990; 262:559 - 567
- Kojima Y. Ultrastructure of goat testes: intercellular bridge between germ cells. J Vet Med Sci 1992; 54:213 - 219
- Russell LD, Vogl AW, Weber JE. Actin localization in male germ cell intercellular bridges in the rat and ground squirrel and disruption of bridges by cytochalasin D. Am J Anat 1987; 180:25 - 40
- Meola SM, Mollenhauer HH, Thompson JM. Cytoplasmic bridges within the follicular epithelium of the ovarioles of two diptera, Aedes aegypti and Stomoxys calcitrans. J Morphol 1977; 153:81 - 85