Abstract
The formation of axon collateral branches is a fundamental aspect of the development of neuronal circuits. Emergence of axonal filopodia from the axon is the first step in the formation of axon collateral branches and pre-synaptic structures. Using embryonic sensory axons as a model system, we have determined that axonal filopodia are formed from transient accumulations of F-actin within the axon, termed actin patches. We found that the branch-inducing factor NGF induces the formation of axonal actin patches and filopodia. NGF signaling, through PI3K, promotes the formation of localized axonal microdomains of PIP3 accumulation. The microdomains in turn drive formation of actin patches. Under basal conditions, only a subset of actin patches gives rise to filopodia, and many patches dissipate without forming a filopodium. Neither NGF nor direct activation of PI3K affects the probability that an actin patch will give rise to a filopodium. Thus, NGF increases formation of axonal filopodia through localized PI3K signaling that promotes the initiation of actin patch precursors to the formation of axonal filopodia. The promotion of actin patch formation by NGF may be mediated through a PI3K-TOR pathway driving intra-axonal protein synthesis. We propose the hypothesis that NGF signaling “turns up the volume” on the mechanism of filopodial formation by increasing axonal levels of the cytoskeletal proteins required for the orchestration of actin patch formation by PIP3 microdomains.
Neurons are amongst the most morphologically complex metazoan cells. The morphological complexity of neurons is directly related to their function. During development, axons are guided to their synaptic target cells. In order to make synaptic contacts with multiple target cells axons establish complex arbors. Axonal arbors are formed by the generation of axon collateral branches.Citation1 Collateral branches form de novo from the shaft of the main axon.Citation2 The first step in the emergence of a collateral branch is the initiation of F-actin based protrusive activity in the form of a filopodium or a lamellipodium.Citation2,Citation3 The axon shaft, unlike the dynamic growth cone, contains sparse F-actin and does not normally exhibit high levels of protrusive activity. The initiation of protrusive activity, and in turn formation of collateral branches, is regulated by extracellular signals. Neurotrophins (e.g., NGF, BDNF) and Slit proteins are signals that induce formation of axonal collateral branches.Citation1,Citation3,Citation4 The signaling and cytoskeletal mechanisms used by branch-inducing factors to stimulate axonal protrusive activity and collateral branch formation are not well understood.
Our laboratory has focused on understanding how F-actin based protrusive activity is initiated along the axon shaft of embryonic sensory DRG neurons. We initially demonstrated that axonal filopodia emerge from precursor F-actin based structures which we termed axonal F-actin patches (actin patches, ).Citation5 As revealed by live imaging of fluorescently labeled actin (e.g., eYFP-actin), actin patches form spontaneously along the axon shaft. The patches then grow in size and intensity, and eventually dissipate. These observations are consistent with other reports that protrusive axonal activity is initiated from segments of the axon that exhibit increased F-actin content relative to the adjacent segments.Citation6–Citation8 The formation of filopodia from precursor actin patches has also been observed in vivo along Drosophila dendrites.Citation9 Thus, the localized regulation of the cytoskeleton giving rise to focal accumulations of F-actin is a major determinant of the protrusive activity of axons and dendrites.
In our most recent publication,Citation10 we demonstrate that PI3K-dependent localized microdomains of PIP3 accumulation drive the initiation and development of axonal actin patches (see for an example of a microdomain). Microdomains of PIP3 are thus orchestrators of the spatio-temporal aspects of the initiation and development of axonal actin patches. When neurons are growing on laminin, in the absence of NGF, they generate low numbers of axonal PIP3 microdomains, actin patches and filopodia. NGF promotes the formation of filopodia and branches from the axons of sensory neurons. Treatment with NGF increases the rate of formation of axonal microdomains of PIP3, and in turn the associated PI3K-dependent actin patches. However, NGF treatment does not alter the probability that a patch will give rise to a filopodium. These observations demonstrate that NGF-PI3K signaling increases the formation of axonal filopodia by inducing the formation of actin patch precursors to the emergence of filopodia, but does not promote the formation of filopodia from actin patches ( and ).
The cytoskeletal proteins downstream of NGF-PI3K signaling that regulate the formation of axonal filopodia, and in turn axon branches, are not characterized. The axon shaft contains low levels of F-actin.Citation11 Thus, localized nucleation of actin filaments is likely a major determinant of the formation of axonal actin patches. Experimental interference with the activity of the Arp2/3 complexCitation12 and cordon bleu,Citation13 two actin nucleators, decreases formation of axon collateral branches. It will be of interest to determine whether these two nucleators of actin filaments are under regulation by NGF-PI3K in the mechanism that initiates axonal actin patches.
Although NGF does not promote the emergence of filopodia from actin patches, it will be necessary to elucidate the mechanism that determines the emergence of filopodia from actin patches. Preliminary platinum replica electron microscopic observations (collaborative work with Dr. T. Svitkina, University of Pennsylvania) reveal that filaments in actin patches are organized into a meshwork, similar to that observed in lamellipodia. Filopodia can arise from lamellipodial actin meshworks through the convergent elongation mechanism.Citation14 In the context of this mechanism, the actin bundling protein fascin is a major determinant of the reorganization of filaments into a filopodial bundle.Citation15 We have localized fascin to axonal filopodia (data not shown), suggesting that it may play a role in the formation of filopodia from actin patches. Fascin is negatively regulated by the activity of PKC and inhibition of PKC in retinal axons promotes collateral branch formation.Citation16 It will be interesting to determine if PKC, through its regulation of the actin bundling activity of fascin, is involved in the reorganization of actin filaments in patches leading to the formation of axonal filopodia. Additionally, Eps8 is an actin filament barbed end capping protein that negatively regulates the formation of filopodia from the axons of hippocampal neurons and is inactivated by BDNF-MAPK signaling.Citation17 Thus, concerted increases in PI3K and MAPK activity induced by neurotrophins could serve to match the increase in the formation of actin patches with the rate of emergence of filopodia from patches. In this case, inhibition of MAPK would not affect the PI3K-dependent increase in the rate of actin patch formation induced by NGF, but would decrease the probability that a patch gives rise to a filopodium.
PTEN is a phosphatase that antagonizes the activity of PI3K. Decreased levels of PTEN promote axon branching and correlate with autism-like behaviors.Citation18,Citation19 Furthermore, PTEN signaling has been shown to be a potential target for promoting axon regeneration and sprouting in the injured spinal cord.Citation20 Intra-axonal protein synthesis is involved in axon regeneration and guidance.Citation21 TOR is a positive regulator of protein synthesis and it is involved in the promotion of axon regeneration resulting from loss of PTEN.Citation22 Treatment of embryonic DRG sensory neurons with NGF activates intra-axonal protein synthesis.Citation23 Localized application of neurotrophins to sensory axons induces formation of filopodia and branches,Citation3 and also locally recruits axonal mRNAs (e.g., β-actin mRNA) and promotes their translation.Citation24 Therefore, it would be of interest to determine if NGF promotes the formation of axonal actin patches and filopodia through intra-axonal protein synthesis driven by the PI3K-TOR pathway.
We are currently testing the hypothesis that NGF signaling through PI3K-TOR induces localized synthesis of cytoskeletal proteins required for NGF to promote the formation of axonal actin patches. We hypothesize that PI3K signaling induced by NGF results in two cellular events (): (1) increasing the formation of PIP3 microdomains which locally orchestrate the assembly of axonal actin patches and (2) the intra-axonal synthesis of cytoskeletal proteins required for actin patch formation. We propose that NGF “turns up the volume” on the axonal levels of the cytoskeletal proteins involved in the formation of actin patches in order to “keep up” with the NGF-induced increase in the numbers of PIP3 microdomains. Continued investigation into the cellular mechanisms of the formation of axonal filopodia and branches may reveal specific targets for therapeutic interventions aimed at the manipulation of axon branching in disease states and recovery from injury.
Abbreviations
NGF | = | nerve growth factor |
BDNF | = | brain derived neurotrophic factor |
DRG | = | dorsal root ganglion |
MAPK | = | mitogen activated protein kinase |
PI3K | = | phosphoinositide 3-kinase |
PIP3 | = | phosphatidylinositol (3,4,5)-trisphosphate |
F-actin | = | actin filaments |
PH | = | plekstrin homology domain |
PKC | = | protein kinase C |
PTEN | = | phosphatase and tensin homolog |
TOR | = | target of rapamycin |
Figures and Tables
Figure 1 Axonal actin patches and PIP3 microdomains. (A) Example of the development of an axonal actin patch along the axon of a cultured sensory neuron as revealed by time-lapse imaging of eYFP-β-actin. The numbers in the panels reflect seconds elapsed. At 0 sec, the patch becomes detectable as a localized accumulation of eYFP-actin (arrowhead) amongst the dim background signal of the axon. Over the next 24 sec the patch grows in intensity. Between 12–18 sec the patch gives rise to a filopodium (arrow). (B) Example of the formation and development of an axonal microdomain of PIP3 accumulation as revealed by PH-GFP imaging. The microdomain becomes detectable above background at 6 sec (arrowhead) and by 24 sec it has almost returned to background levels. (A and B) are from different time-lapse sequences. Dual-channel imaging of mCherry-actin and PH-GFP in the same axon is reported elsewhere in reference Citation10. (C) Schematic summarizing the effects of NGF on the number of axonal actin patches and filopodia. The axon is shown as a black bar. NGF treatment (Post NGF) doubles the numbers of axonal actin patches and filopodia. However, the percentage of patches that give rise to a filopodium is not affected by NGF.
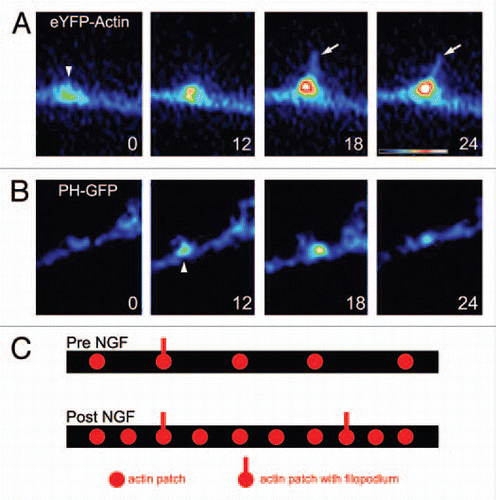
Figure 2 (A) Schematic summarizing the role of PI3K signaling in laminin (LN) and NGF mediated formation of axonal actin patches.Citation10 In the absence of NGF treatment (top part), LN signaling through integrins promotes the formation of PI3K-dependent PIP3 axonal microdomains (region shaded green). Axonal actin patches form in the vicinity, of integrin clusters. Following NGF treatment, TrkA signaling induces formation of additional PI3K-dependent PIP3 axonal microdomains, and actin patch formation colocalizes with clusters of TrkA receptors. Thus, localized PIP3 microdomains act to orchestrate the focal assembly of actin patches at discrete foci along the axon. (B) Schematic summarizing the hypothesis that NGF-induced intra-axonal protein synthesis of cytoskeletal proteins involved in the formation of actin patches is required to maintain a balance between the increase in the number of PIP3 microdomains (µ domains) and the availability of proteins utilized by microdomains to drive formation of actin patches. PIP3 microdomains regulate the spatio-temporal aspects of actin patch initiation and development along axons, while increases in intra-axonal protein synthesis provide the molecular “building blocks” required for the coordinated assembly of actin patches by PIP3 microdomains. Inhibition of intra-axonal protein synthesis induced by NGF is predicted to have no effect on the formation of PIP3 microdomains while blocking the ability of the NGF-induced microdomains to assemble actin patches due to insufficient supply of cytoskeletal proteins.
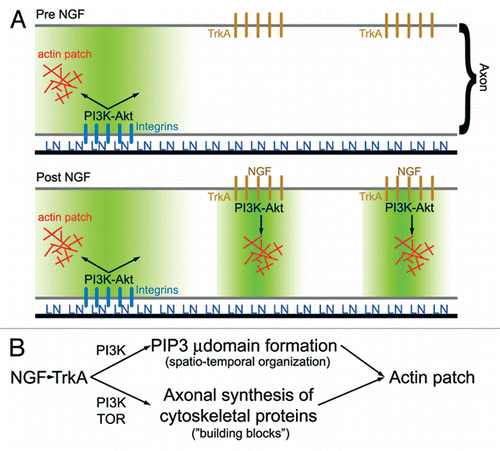
Acknowledgements
The work was supported by an NIH grant to G.G. (NS048090).
Addendum to:
References
- Cohen-Cory S, Kidane AH, Shirkey NJ, Marshak S. Brain-derived neurotrophic factor and the development of structural neuronal connectivity. Dev Neurobiol 2010; 70:271 - 288
- Bastmeyer M, O'Leary DD. Dynamics of target recognition by interstitial axon branching along developing cortical axons. J Neurosci 1996; 16:1450 - 1459
- Gallo G, Letourneau PC. Localized sources of neurotrophins initiate axon collateral sprouting. J Neurosci 1998; 18:5403 - 5414
- Wang KH, Brose K, Arnott D, Kidd T, Goodman CS, Henzel W, et al. Biochemical purification of a mammalian slit protein as a positive regulator of sensory axon elongation and branching. Cell 1999; 96:771 - 784
- Loudon RP, Silver LD, Yee HF Jr, Gallo G. RhoA-kinase and myosin II are required for the maintenance of growth cone polarity and guidance by nerve growth factor. J Neurobiol 2006; 66:847 - 867
- Lau PM, Zucker RS, Bentley D. Induction of filopodia by direct local elevation of intracellular calcium ion concentration. J Cell Biol 1999; 145:1265 - 1275
- Mingorance-Le Meur A, O'Connor TP. Neurite consolidation is an active process requiring constant repression of protrusive activity. EMBO J 2009; 28:248 - 260
- Korobova F, Svitkina T. Arp2/3 complex is important for filopodia formation, growth cone motility and neuritogenesis in neuronal cells. Mol Biol Cell 2008; 19:1561 - 1574
- Andersen R, Li Y, Resseguie M, Brenman JE. Calcium/calmodulin-dependent protein kinase II alters structural plasticity and cytoskeletal dynamics in Drosophila. J Neurosci 2005; 25:8878 - 8888
- Ketschek A, Gallo G. Nerve growth factor induces axonal filopodia through localized microdomains of phosphoinositide 3-Kinase Activity that drive the formation of cytoskeletal precursors to filopodia. J Neurosci 2010; 30:12185 - 12197
- Letourneau PC. Actin in axons: stable scaffolds and dynamic filaments. Results Probl Cell Differ 2009; 48:65 - 90
- Strasser GA, Rahim NA, VanderWaal KE, Gertler FB, Lanier LM. Arp2/3 is a negative regulator of growth cone translocation. Neuron 2004; 43:81 - 94
- Ahuja R, Pinyol R, Reichenbach N, Custer L, Klingensmith J, Kessels MM, et al. Cordon-bleu is an actin nucleation factor and controls neuronal morphology. Cell 2007; 131:337 - 350
- Svitkina TM, Bulanova EA, Chaga OY, Vignjevic DM, Kojima S, Vasiliev JM, et al. Mechanism of filopodia initiation by reorganization of a dendritic network. J Cell Biol 2003; 160:409 - 421
- Vignjevic D, Kojima S, Aratyn Y, Danciu O, Svitkina T, Borisy GG. Role of fascin in filopodial protrusion. J Cell Biol 2006; 174:863 - 875
- Schmidt JT, Fleming MR, Leu B. Presynaptic protein kinase C controls maturation and branch dynamics of developing retinotectal arbors: possible role in activity-driven sharpening. J Neurobiol 2004; 58:328 - 340
- Menna E, Disanza A, Cagnoli C, Schenk U, Gelsomino G, Frittoli E, et al. Eps8 regulates axonal filopodia in hippocampal neurons in response to brain-derived neurotrophic factor (BDNF). PLoS Biol 2009; 7:1000138
- Drinjakovic J, Jung H, Campbell DS, Strochlic L, Dwivedy A, Holt CE. E3 ligase Nedd4 promotes axon branching by downregulating PTEN. Neuron 2010; 65:341 - 357
- Kwon CH, Luikart BW, Powell CM, Zhou J, Matheny SA, Zhang W, et al. Pten regulates neuronal arborization and social interaction in mice. Neuron 2006; 50:377 - 388
- Liu K, Lu Y, Lee JK, Samara R, Willenberg R, Sears-Kraxberger I, et al. PTEN deletion enhances the regenerative ability of adult corticospinal neurons. Nat Neurosci 2010; 13:1075 - 1081
- Gumy LF, Tan CL, Fawcett JW. The role of local protein synthesis and degradation in axon regeneration. Exp Neurol 2010; 223:28 - 37
- Park KK, Liu K, Hu Y, Smith PD, Wang C, Cai B, et al. Promoting axon regeneration in the adult CNS by modulation of the PTEN/mTOR pathway. Science 2008; 322:963 - 966
- Roche FK, Marsick BM, Letourneau PC. Protein synthesis in distal axons is not required for growth cone responses to guidance cues. J Neurosci 2009; 29:638 - 652
- Willis DE, van Niekerk EA, Sasaki Y, Mesngon M, Merianda TT, Williams GG, et al. Extracellular stimuli specifically regulate localized levels of individual neuronal mRNAs. J Cell Biol 2007; 178:965 - 980