Abstract
The larvae of aquatic vertebrates sometimes possess a transient, mucus-secreting gland on their heads. The most studied of these organs is the Xenopus cement gland. The tadpoles use it to attach to plants or to the water surface, supposedly to hide from predators and save energy before they can swim or feed. Moreover their gland, being innervated by trigeminal fibres, also mediates a locomotor stopping response when the larvae encounter an obstacle. We have described an equivalent organ on the head of the teleost Astyanax mexicanus, that we have called the casquette because of its shape and position on the larval head. The casquette is transient, sticky, secretes mucus, is innervated by the trigeminal ganglion, has an inhibitory function on larval swimming behaviour, and expresses Bmp4 and Pitx1/2 during embryogenesis. Here we further discuss the nature of the equivalence between the frog cement gland and the fish casquette, and highlight the usefulness of non-conventional model species to decipher developmental and evolutionary mechanisms of morphological variations.
A Question of Homology
The Astyanax casquette and the Tilapia adhesive glands we have describedCitation1 both possess multiple common features with the well-studied and described Xenopus cement gland ().Citation2–Citation5 Their developmental origin and specification gene network, their connectivity and their role in regulating larval swimming behavior provide evo-devo, hodological and functional types of arguments to propose equivalence between these organs, respectively.Citation1 But what type of equivalence are we reporting here? Homology? Analogy? Or else? The answer is important to discuss the ancestral state/nature of this gland in chordate larvae ().
Two organs that look similar are said to be homologous if they are inherited from a common ancestor, implying historical continuity that can be followed in the tree of life. Moreover, as defined by Owen (1843),Citation6 homologous organs show structural correspondence, i.e., the same connections with neighbor structures, as well as conserved topology within a comparable body plan of organization. By contrast, analogy depicts the relationship between two traits that look similar but do not share common ancestry. If such traits arise independently in two taxa or species, they are called homoplasic and likely result from evolutionary convergence. More recently, Shubin, Tabin and Carroll have coined the term “deep homology” to describe “the sharing of the genetic regulatory apparatus that is used to build morphologically and phylogenetically disparate animal features“.Citation7,Citation8 Below we develop an interpretation in favor of the former of these conditions: a true homology.
The Astyanax casquette secretes mucus, is innervated by the trigeminal nerve (although not by the exact same branch as the Xenopus cement gland), mediates larval attachment to support like its amphibian counterpart, and develops under the control of the same gene regulatory network, including Bmp4 and Pitx1/2, as the one used to specify the Xenopus cement gland. In fact they are both ectodermal derivatives and share a placodal-like common genetic developmental specification, and they contain the same secretory type of cells. Thus, we think that mere analogy does not faithfully reflect the fact that the two organs are fully comparable with both the connectivity and the developmental specification criteria. Actually, only the apparent dorsal position of the Astyanax casquette and the Tilapia adhesive glands seems different from the ventral position of the Xenopus cement gland, making it difficult at first sight to propose a homology hypothesis.
However, the weight of the criteria we have gathered appears heavy in favor of such a homology hypothesis. The adhesive organs we have studied vary in shape, size, number, structure and position, and are therefore morphologically disparate. Yet we do not think they are phylogenetically disparate. Instead, we suggest that the anterior head ectoderm (as a field) of chordates has the potential to express an “adhesive organ developmental module” in a versatile manner, in varied positions. Depending on where this module is recruited, the organ will not appear evidently homologous; however it is always an anterior ectodermal derivative. The developmental module itself is most probably ancestral, as adhesive organs are found in animals as diverse as ascidians, dipnoi, actinopterygians or amphibians (). The use of this module, driven by patterning and morphogenetic factors such as Otx, Pitx and Bmp, has been lost in a lot of species, but can nevertheless be virtually “traced back” in history at all nodes of the tree up to the common ancestor of all chordates. We think unlikely that such a developmental module has been re-invented at least 12 times (and probably much more as discussed below) in the chordate tree (). The most parsimonious hypothesis is therefore to propose that the organs generated by this ancestral developmental module are indeed homologous. The next challenge will be to understand how this module is specifically triggered on the head ectoderm, and perhaps to find an “adhesive organ regulatory cassette” in the non-coding sequences of the implicated genes.
A World of Adhesive Organs to Discover
Among teleosts, the two conventional and widely used laboratory models for developmental, physiological and behavioral studies are the zebrafish (Danio rerio, a cypriniform) and the medaka (Oryzias latipes, a beloniform). Strikingly, neither of them possesses a cement gland-like adhesive organ, in relation with the idea that often, the species chosen by researchers to become models show peculiarities and losses. Conversely, adhesive organs have long been described in lungfishesCitation9,Citation10 and in actinopterygians in “classical” studies on ganoids (non-teleostean actinopterygians with hard, bony scales)Citation11–Citation13 as well as mormyresCitation9 and several species of cichlids.Citation14–Citation17 In fact, it is to be expected that if the presence of attachment organs in aquatic larvae was systematically investigated, the number of asterisks on the chordate tree in would increase significantly, and adhesive glands would appear more widely shared than anticipated when considering exclusively laboratory models.
Then, in all the adhesive gland-equipped aquatic larvae, is the gland used exactly in the same manner, and does it serve exactly the same function? The global role of attachment organs is certainly the protection of the larvae, but this goal is achieved through diverse and specific adaptive strategies, well illustrated by the 3 types of larva we have studied.Citation1
As soon as they hatch, the larvae of Tilapia mariae (a perciform) form rosettes, in the aquarium as well as in a Petri dish. Inside the rosette, the larvae are attached heads to heads by their adhesive glands through filamentous mucus secretions mixed with sand and plant debris. This attachment is quite robust. The parents stay over the rosettes and guard them, so that there is no loss of larvae at this stage. Rosette formation is exclusive (meaning there are no freely-swimming larvae) during three days after hatching, and then tends to decrease quickly, in parallel with the decrease in size and rapid disappearance of the glands on the larval heads. Eight days after fertilization all the larvae swim freely, always under parental guard, but they are now much more prone to predation by other fishes in the aquarium and their number drops drastically. Concerning Astyanax (a characiform), there is no such formation of rosettes via the casquette. We hypothesized that surface fish larvae use their casquette to stick to plant leaves or rocks and hide from predators, whereas cavefish larvae use it to adhere to the water surface, where oxygen levels are higher in the still waters of the ponds, and where they are not prone to predation by adults who are exclusive bottom feeders. In sum, when comparing the attachment role of their adhesive glands, it appears that Astyanax larvae use their casquette to stick to substrate, whereas Tilapia larvae use it to stay grouped together. To precise the mechano-sensory role of their organs, more detailed studies and comparisons will be necessary. These emerging model species have a lot more to tell us about the evolution of their morphologies and behaviors.
Figures and Tables
Figure 1 Comparison of the Xenopus cement gland and the Astyanax casquette. Basic properties of the two organs (black) are compared: position, gene expression, trigeminal innervation and functional role. Only the former is different between the two species.
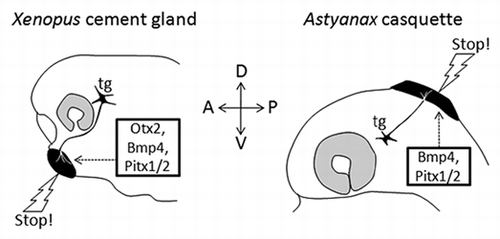
Figure 2 Adhesive organs in the chordate phylogenetic tree. A simplified chordate tree helps visualisation of the presence of adhesive organs in diverse taxa, as well as the extreme diversity of their morphology. Position, number, size, shape and structure vary greatly between examples shown. A star indicates that adhesive organs have also been described in these species; however they are not illustrated for the sake of clarity of the figure. Drawing credits: the Lepidosteus (gar) sucking disk is a detail taken from Alexander Agassiz, Planche II.Citation11 The Xenopus laevis cement gland is a detail from the stage 38 embryo of the Nieuwkoop and Faber staging table.Citation18 The salamander (Pleurodeles Waltl) balancer is a detail taken from Figure 5.12 of Duellman and Trueb.Citation19 The ascidian papilla, the Astyanax casquette and the Tilapia adhesive glands were drawn by SR. A blind cavefish is drawn for Astyanax, so that the eye is small and transparent, hence not visible.
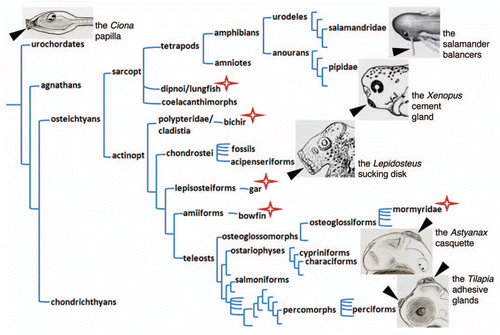
Addendum to:
References
- Pottin K, Hyacinthe C, Rétaux S. Conservation, development and function of a cement gland-like structure in the fish Astyanax mexicanus. Proc Natl Acad Sci USA 2010; 107:17256 - 17261
- Boothby KM, Roberts A. The stopping response of Xenopus laevis embryos: pharmacology and intracellular physiology of rhythmic spinal neurones and hindbrain neurones. J Exp Biol 1992; 169:65 - 86
- Honore E, Hemmati-Brivanlou A. In vivo evidence for trigeminal nerve guidance by the cement gland in Xenopus. Dev Biol 1996; 178:363 - 374
- Nokhbatolfoghahai M, Downie JR. Larval cement gland of frogs: comparative development and morphology. J Morphol 2005; 263:270 - 283
- Sive H, Bradley L. A sticky problem: the Xenopus cement gland as a paradigm for anteroposterior patterning. Dev Dyn 1996; 205:265 - 280
- Owen R. Lectures on the comparative anatomy and physiology of the invertebrate animals 1843; London Longman Brown Green and Longmans
- Shubin N, Tabin C, Carroll S. Fossils, genes and the evolution of animal limbs. Nature 1997; 388:639 - 648
- Shubin N, Tabin C, Carroll S. Deep homology and the origins of evolutionary novelty. Nature 2009; 457:818 - 823
- Budgett JS. On the breeding habits of West African fishes, with an account of the external features in the development of Protopterus annectans and a description of the larva of Polypterus lapradei. T Zoological Soc London 1901; 16:115 - 136
- Kerr JG. The external features in the development of Lepidosiren paradoxa, Fitz. Phil Trans Roy Soc London 1900; 46
- Agassiz A. The development of Lepidosteus. Amer Acad Arts and Sci Prc (NS) 1879; 14:65 - 85
- Balfour FM, Parker WN. The structure and development of Lepisdosteus. Phil Trans Roy Soc London 1882; 173:360 - 436
- Eycleshymer AC, Wilson JM. Adhesive organs of Amia. Biol Bull 1908; 14:134 - 149
- Jones S. On the origin and development of the cement glands in Etroplus maculatus (Bloch). Proc Ind Acad Sci Sect B 1937; 6:251 - 261
- Meijide FJ, Guerrero GA. Embryonic and larval development of a substrate-brooding cichlid Cichlasoma dimerus (Heckel, 1840) under laboratory conditions. J Zool 2000; 252:481 - 493
- Peters HM. Uber larvale Haftorgane bei Tilapia (Cichlidae, Teleostei) und ihre Rückbildung in der Evolution. Zool Jb Physiol 1965; 71:287 - 300
- Prusinska M, Mamcarz A, Kupren K. Early ontogeny of Tropheus Moorii Boulenger 1898 (Pisces, Cichlidae, Lake Tanganyika) in laboratory conditions. Polish J Nat Sci 2009; 23:888 - 903
- Nieuwkoop PD, Faber J. Normal table of Xenopus laevis. A systematical and chronological survey of the development from fertilized egg till the end of metamorphosis 1956; North Holland, Amsterdam Daudin
- Duellman WE, Trueb L. Biology of Amphibians 1994; Baltimore and London The Johns Hopkins University Press