Abstract
The Devonian appearance of 1-10 meter long armored fish (placoderms) coincides with geochemical evidence recording a transition into fully oxygenated oceans.1 A comparison of extant fish shows that the large individuals are less tolerant to hypoxia than their smaller cousins. This leads us to hypothesize that Early Paleozoic O2 saturation levels were too low to support >1 meter size marine, predatory fish. According to a simple model, both oxygen uptake and oxygen demand scale positively with size, but the demand exceeds supply for the largest fish with an active, predatory life style. Therefore, the largest individuals may lead us to a lower limit on oceanic O2 concentrations. Our presented model suggests 2-10 meter long predators require >30-50% PAL while smaller fish would survive at <25% PAL. This is consistent with the hypothesis that low atmospheric oxygen pressure acted as an evolutionary barrier for fish to grow much above ~1 meter before the Devonian oxygenation.
Geochemical evidence from sedimentary rocks based on stable molybdenum isotopes indicate rising O2 concentrations in the oceans and atmosphere ∼400 million years ago, and that the earliest animals lived from 550–400 Ma under lower oxygen levels than today.Citation1 This final rise in ocean oxygenation is roughly correlated to a dramatic increase in size maxima among predatory fish. We review the paleontological data and physiological O2 requirements in modern fish and use a simple model for fish metabolism to evaluate the relationship between fish size and early Paleozoic oxygen levels.
The History of Fish
Fossil chordates are known from all Phanerozoic periods, but exploration of pre-Silurian vertebrates builds on sketchy remains and often depends on soft-tissue preservation.Citation2 The most primitive chordates are Cambrian, jawless fish (agnathans)Citation3 resembling early ancestors of lampreys and hagfish. Jawed vertebrates (gnathostomata) are also an ancient lineage dating back to the Ordovician. They include cartilaginous fishes (chondrichthyans), bony fish and tetrapods (osteichthyans) and two extinct groups armored fish (placoderms) and spiny sharks (acanthodians).Citation2 Today, the vast majority of fish diversity is found within the osteichtyans including ray-finned fish (actinopterygii) and lobe-finned fish (sarcopterygii), most of which diversified in the Early Devonian.Citation4
A major transition in the size maxima of marine vertebrates took place in the Devonian. The appearance of more than 1 meter long jawed placoderms marks a 3–6 order of magnitude increase in body weight compared to centimeter sized, jawless fish of the earlier Paleozoic ().Citation5
However, the Cambrian ocean also hosted large marine swimmers like the ∼1 meter long segmented AnomalocarisCitation6 and the Ordovician, more than 1 meter-sized, shelled nautiloid cephalopods.Citation7 Lifestyle and swimming mode for the Anomalocaris is not fully understood. It has been suggested to be an agile predator,Citation8 but its mouth might have been adapted to a soft tissue diet,Citation9 meaning it was not necessarily energetic and versatile like predatory fish. Cephalopods work hard to move at the same speed as fish,Citation10 but their indolent life style requires less oxygen. Modern cephalopods consume oxygen at slower rate (0.5–8 µmol O2 g−1hr−1)Citation11 than active predatory fish (20–40 µmol O2 g−1 hr−1).Citation12,Citation13 Perhaps, this also explains why cephalopods demonstrate high hypoxia tolerance.Citation11 For these reasons we disregard the Ordovician cephalopods as more oxygen demanding than predatory fish.
Oxygen Tolerance in Modern Fish
Fish are among the most sensitive marine animals towards hypoxia. At low O2 concentrations fishes respond by increasing breathing frequency and breath amplitude, but modern fish are largely unaffected above 70% of today's surface levels.Citation14 The magnitude of the hypoxic ventilatory response depends on gill surface area, thickness of the gill membrane, body weight, environmental temperature and activity level.Citation15 Hypoxia tolerance levels are here summarized in fractions of oxygen saturation in 15°C seawater [7.9 mg/L = 248 µM at atmospheric pO2= 0.21 atm = 100% present atmospheric level (PAL) corresponding to pO2 = 156 torr in surface water when corrected for steam pressure]. We focus on whole body oxygen uptake in marine, pelagic predatory fish and their ability to spend their entire life under lower “normoxic” conditions than today.Citation1 In osteichthyans mortality is observed at 7–25% [O2], active swimmers stop growing and metabolizing below 57–75% and 25–41%, respectively.Citation16 This is consistent with LC50 levels showing 50% of the measured populations die at 18–20%.Citation17 These studies did not explicitly investigate the dependence of body weight, gill area, temperature and activity levels, so we have compared the tolerance levels with maximal length of the studied fish species (Fig. S7 and review in ref. Citation1). A statistical κ-test shows moderate agreement between larger fish and low hypoxia tolerance (Appendix Table 1 and 2 in Sup. Material). That is, large fish require higher O2 concentration than small fish. This is because large fish have a higher total O2 consumption despite using less oxygen per unit mass.
Oxygen Uptake and Requirement in Fish
We explore how oxygen tension (pO2) in the marine environment affect oxygen uptake in gill fish and use a calibration based on modern osteichthyans to evaluate possible placoderm physiological needs.Citation18 Our main point is that oxygen supply through diffusion to any individual is a slower function of size (e.g., mass0.82) than the oxygen demand in active predatory fish (demand ∼ massaA, aA ∼ 0.98) implying that the demand exceeds supply above a certain size. As maximal oxygen tension in seawater increases, so does oxygen uptake, which, in turn, allows for larger predatory fish.
Fish consume oxygen to fuel both basal and active metabolic needs. The demand depends on body mass, water temperature, and activity level. O2 is supplied by diffusion across the gill membrane. The total body uptake is a function of gill surface area, gill thickness, oxygen pressure in the blood (e.g., blood capacity and oxygen recharge potential inside the gill), and pO2 outside the gill.
In our simple model predatory fish of 2–10 meter length require >30–50% PAL while smaller fish would survive at 20–30% PAL (; derivation in the appendix in Sup. Material). The calculations of metabolic demand and oxygen uptake are based on fish with same geometry (here tuna), with gill area increasing as a function of mass to the power of 0.82,Citation19 and the gill membrane is fixed at typical thickness of 10 µm, so that the function of size maxima can be explored explicitly. Of course, these physiological parameters are subject to selection and there are alternative ways of improving oxygen uptake. Our model fish comparison assumes the chosen parameters are realistic values in fish with optimal oxygen uptake.
Scaling laws of size dependency of basal and maximal (active) metabolic rates exist for fish up to 1 meter, and there is no data for largest fish, e.g., sharks. The scope defines the ratio between maximal and resting metabolic rate and the highest measured scope is ∼15 in modern Picasso fish.Citation20 The scaling laws predict a positive correlation between scope and fish size with higher values in our placoderm-models. We calibrated the model to give zero oxygen uptake at 19% PAL that we assume is representative of minimal required O2 pressure, e.g., minimal venous oxygen tension (further details are stored in the appendix).
To improve these model predictions, future studies could investigate the values of the governing parameters for oxygen uptake in the largest, agile predatory fish, including consumption rate experiments with sharks and approach an evaluation of placoderm activity levels. The combination of paleontological examinations with plausible respiratory function may provide useful information about the environmentCitation23 and elucidate if intensified predation resulted from a physiological enabling increase in ocean oxygenation.
Figures and Tables
Figure 1 The length of 84 fish species found in each of the Paleozoic periods from the Cambrian through the Carboniferous. In this compilation the Carboniferous stage is clearly under-sampled, while the older periods are better represented in this study.
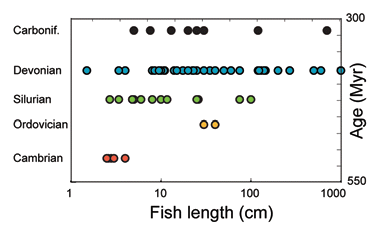
Figure 2 Model fish size maxima are shown as a function of ambient oxygen pressure for tuna shaped fish (See appendix in Sup. Material for details). The active metabolic rate in the model fish determines its high oxygen demand, which is so high that larger gill area is unable to accommodate the required supply. Therefore, there is a limit to how big predatory fish can grow in a low oxygen environment.
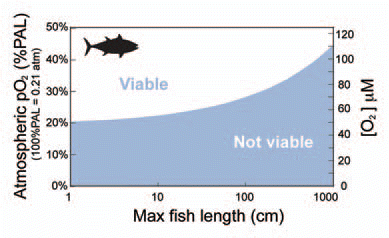
Additional material
Download Zip (361.5 KB)Acknowledgements
We thank John F. Steffensen, Frank B. Jensen, Per E. Ahlberg, Jan Bergström, Donald Canfield, and Andrew Knoll for thoughtful comments, additions and reviews. Villum Kann Rasmussen Foundation (T.W.D.), the Swedish Research Council (E.U.H.), and Danish National Research Foundation (NordCEE) supported this work.
Addendum to:
References
- Dahl TW, Hammarlund EU, Anbar AD, Bond DPG, Gill BC, Gordon GW, et al. A Devonian rise of atmospheric oxygen correlated to the radiations of terrestrial plants and predatory fish. Proc Natl Acad Sci USA 2010; 107:17911 - 17915
- Janvier P. Catching the first fish. Nature 1999; 402:21 - 22
- Shu D-G, Luo H-L, Conway Morris S, Zhang X-L, Hu S-X, Chen L, et al. Lower cambrian vertebrates from south China. Nature 1999; 402:42 - 46
- Botella H, Blom H, Dorka M, Ahlberg PE, Janvier P. Jaws and teeth of the earliest bony fishes. Nature 2007; 448:583 - 586
- Payne J, Boyer AG, Brown JH, Finnegan S, Kowalewski M, Krause RA Jr, et al. Two-phase increase in the maximum size of life over 3.5 billion years reflects biological innovation and environmental opportunity. Proc Natl Acad Sci USA 2009; 106:24 - 27
- Xianguang H, Bergstrom J, Ahlberg P. Anomalocaris and other large animals in the lower cambrian Chengjiang fauna of southwest China. Gff 1995; 117:163 - 183
- Kröger B, Yun-Bai Z. Anomalocaris and other large animals in the lower cambrian chengjiang fauna of southwest China. Palaeogeo Palaeoclim Palaeoecol 2009; 273:174 - 183
- Whittington H, Briggs D. The largest Cambrian animal, Anomalocaris Burgess Shale, British Columbia. Philosoph Transact B 1985; 309:569
- Hagadorn J. Putting Anomalocaris on a Soft-Food Diet?. 2010 GSA Denver Annual Meeting
- O'Dor R. Telemetered cephalopod energetics: swimming, soaring, and blimping. Integr Comp Biol 2002; 42:1065 - 1070
- Seibel B, Thuesen E, Childress J, Gorodezky L. Decline in pelagic cephalopod metabolism with habitat depth reflects differences in locomotory efficiency. Biol Bull 1997; 192:262
- Brett J. The metabolic demand for oxygen in fish, particularly salmonids, and a comparison with other vertebrates. Resp Physiol 1972; 14:151 - 170
- Clarke A, Johnston N. Scaling of metabolic rate with body mass and temperature in teleost fish. J Anim Ecol 1999; 68:893 - 905
- Steffensen JF. Copenhagen
- Almeida-Val V, Randall D. The Physiology of Tropical Fishes 2006; Academic Press
- Gray J, Wu R, Or Y. Effects of hypoxia and organic enrichment on the coastal marine environment. Mar Ecol Prog 2002; 238:249 - 279
- Vaquer-Sunyer R, Duarte C. Thresholds of hypoxia for marine biodiversity. Proc Natl Acad Sci USA 2008; 105:15452 - 15457
- Young GC. Placoderms (Armored Fish): Dominant vertebrates of the devonian period. Annu Rev Earth Planet Sci 2010; 38:523 - 550
- Schmidt-Nielsen K. Scaling, why is animal size so important? 1984; Cambridge Univ Press
- Korsmeyer K, Steffensen JF, Herskin J. Energetics of rigid-body swimming, undulatory swimming, and gait transition in parrotfish (Scarus schlegeli) and triggerfish (Rhinecanthus aculeatus). J Exp Biol 2002; 205:1253 - 1263
- Piiper J, Scheid P, Perry S, Hughes G. Effective and morphometric oxygen-diffusing capacity of the gills of the elasmobranch Sciliorhinus stellaris. J Exp Biol 1986; 123:27 - 41
- Parks W, Bard F, Cayré P, Kume S, Guerra A. Length-weight relations for bigeye tuna captured in the eastern atlantic ocean. Col Vol Sci Pap ICCAT 1982; 17:214 - 225
- Knoll AH, Bambach RK, Payne JL, Pruss S, Fischer WW. Paleophysiology and end-Permian mass extinction. Earth and Planetary Science Letters 256; 4:295 - 313