Abstract
Symmetric cell division in Gram-negative bacteria requires the concerted action of three Min proteins that together ensure exclusive formation of the cell division septum at the mid-point of the cell. We have recently described the structure and dynamic properties of MinE, the protein responsible for directing the cell division inhibitor complex formed by the MinC and MinD proteins away from the middle of the cell. An unexpected feature of this structure was the location of MinD-binding residues at buried, non-accessible sites in the dimeric interface. Here we elaborate on the potential role of conformational changes that might be involved to allow access to these residues, along with the interesting questions raised by these features of the MinE structure.
In order for Gram-negative bacteria to divide symmetrically, the Min protein system forms a dynamic polymeric assembly that prevents the formation of cell division septa at the cell poles.Citation1–Citation4 This system is comprised of three proteins; MinC, inhibitor of the Z-ring formation that is required for septum formation,Citation5 MinD, the ATPase that localizes this inhibitor to the membrane,Citation6 and MinE, the regulator that confers site selectivity to the inhibitory MinCD complex.Citation7 This ability of MinE to maintain a low average concentration of the MinCD complex in the middle of the cell creates a zone that allows Z-ring formation to occur exclusively at this site. It achieves this function by stimulating the ATP-hydrolysis driven disassembly of membrane-associated subcellular structures formed by MinD.Citation8–Citation10 The molecular details of this disassembly process have yet to be elucidated, however, new insights into these events have been provided by our work characterizing the structure and dynamics of MinE.Citation11
We determined the first complete structure of a full-length MinE dimer (), revealing features that were not anticipated from the extensive biochemical literature available for this protein.Citation12–Citation17 In particular, a structure of the dimer previously determined for a C-terminal fragment of MinE (residues 31–88) showed residues corresponding to strand β3 centrally located in the dimer interface ().Citation18 However, in the structure of the full-length protein this interface is formed instead by residues preceding this C-terminal domain that form β1 (residues 20–30), a feature that was also observed in a recently released x-ray crystal structure.Citation19 This was particularly surprising given that residues in β1 are important for interactions with MinD; for example, a fragment containing the first 31 residues of MinE could bind MinD and displace MinC from the MinCD complex.Citation12 Our own data monitoring stimulation of MinD ATPase activity by MinE confirmed that residues within this region are directly involved in the interaction. Nonetheless, our structure showed that access to some of these residues should be highly restricted, making them largely unavailable for interactions with a macromolecular binding partner such as MinD.
This new data raises a number of interesting questions regarding how MinD might be able to bind residues that are buried in the dimeric interface of MinE. A clue was provided by solution NMR spin relaxation measurements that identified residues undergoing conformational dynamics.Citation11 The location of dynamic residues was consistent with a motional model where the N-terminal helix dissociates from the main body of the structure to increase accessibility for MinD-binding residues (). However, even in this more open state a critical residue for MinD activation (Leu-22) would still be expected to remain buried, suggesting that another mechanism for improving solvent accessibility must also be involved.
A straightforward mechanism that would increase the accessibility of these residues is one in which the MinE dimer dissociates into a monomeric state. However, this scenario is difficult to reconcile with the high affinity for MinE dimerization, particularly as suggested by the absence of a detectable monomer at low mM concentrations.Citation11 Moreover, the location of functionally important residues does not present a coherent protein-binding interface in the dimer structure. Specifically, residues participating in the MinD interaction lie on opposing sides of the β-strand, with critical residues being adjacent to others that do not participate in the interaction (). These structural features suggest that a significant change in MinE conformation may be required for these interactions, potentially brought about through loss of inter-subunit interactions in dimer dissociation, or induced through the interaction with MinD itself.
It is tempting to speculate that the conformational change required for interactions with MinD involves dissociation of the β1 strand from the main body of the MinE structure allowing it to adopt the a-helical structure that was previously proposed based on yeast two-hybrid assays using an N-terminal fragment of MinE (residues 1–31).Citation12 In this conformation, Ala-18 Arg-21 Leu-22 and Ile-25 would all localize to one face of this helix, with the less critical Lys-19 being adjacent to this core (). This binding conformation offers the advantage in that residues that were shown not to participate in the interaction (e.g., Ile-24) are directed away from the binding interface. In contrast, side chains from Leu-22 and Ile-24 are in close proximity in the β1 structure, making it difficult to envisage a binding pocket that could bind one residue, but not be inhibited by drastic mutations made at the other.
If MinD binding involves dissociation of the MinD-binding region from the rest of the MinE structure, this raises interesting questions regarding the fate of the remaining part of protein that is not binding MinD, also known as the topological specificity domain. For example, is it possible that this part of MinE could form a dimer resembling that formed by the C-terminal fragment of MinE, with inter-subunit interactions between β3? This could explain the biological activity that is associated with this domain (e.g., overexpression of the isolated TSD disrupts normal cell division in E. coli even though it cannot interact with MinD).Citation7,Citation14,Citation16 At this stage little is known about the oligomerization properties of MinE, particularly in the context of the MinD polymer. These are the features that will need to be elucidated in order to understand the molecular basis of MinE-mediated Min protein oscillation. However, our identification of MinE residues that are directly involved in the interaction with MinD should serve as a fundamental step toward this elusive goal.
Figures and Tables
Figure 1 (A) Two views of the solution NMR structure of MinE from Neisseria gonorrhoeae (PDB accession number 2KXO), with a model of the more open conformation suggested by NMR dynamics data on the right hand side. Each subunit in the dimer is shown in a different colour, and β-strands β1, β2 and β3 labeled for each subunit. Sidechain atoms for residues that directly participate in MinD interactions are also shown, with the inaccessible Leu-22 highlighted in red and the partially inaccessible residues (Arg-21 Ile-25) shown in orange. (B) The solution NMR structure of residues 31–88 from E. coli MinE (1EV0), showing the different dimer interface formed by residues corresponding to β3 in the full-length structure.
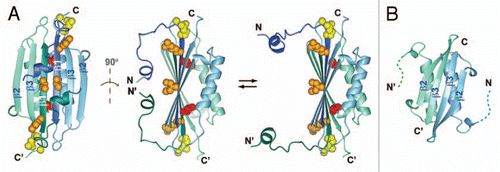
Figure 2 Magnified view of the region of MinE containing residues that bind to MinD, either in (A) the β1 structure as it appears in the dimer or (B) in a hypothetical α-helical structure. Sidechains that are critical for MinD binding are shown in red, those that play a smaller role in the interaction in orange and those that can be mutated and still give rise to wild-type function are in blue.
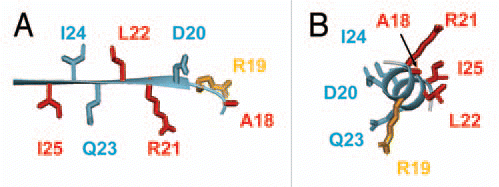
Addendum to:
References
- Lutkenhaus J. Min oscillation in bacteria. Adv Exp Med Biol 2008; 641:49 - 61
- Hu Z, Lutkenhaus J. Topological regulation of cell division in Escherichia coli involves rapid pole to pole oscillation of the division inhibitor MinC under the control of MinD and MinE. Mol Microbiol 1999; 34:82 - 90
- Raskin DM, de Boer PA. MinDE-dependent pole-to-pole oscillation of division inhibitor MinC in Escherichia coli. J Bacteriol 1999; 181:6419 - 6424
- Raskin DM, de Boer PA. Rapid pole-to-pole oscillation of a protein required for directing division to the middle of Escherichia coli. Proc Natl Acad Sci USA 1999; 96:4971 - 4976
- Hu Z, Mukherjee A, Pichoff S, Lutkenhaus J. The MinC component of the division site selection system in Escherichia coli interacts with FtsZ to prevent polymerization. Proc Natl Acad Sci USA 1999; 96:14819 - 14824
- Hu Z, Lutkenhaus J. A conserved sequence at the C-terminus of MinD is required for binding to the membrane and targeting MinC to the septum. Mol Microbiol 2003; 47:345 - 355
- Zhao CR, de Boer PA, Rothfield LI. Proper placement of the Escherichia coli division site requires two functions that are associated with different domains of the MinE protein. Proc Natl Acad Sci USA 1995; 92:4313 - 4317
- Fu X, Shih YL, Zhang Y, Rothfield LI. The MinE ring required for proper placement of the division site is a mobile structure that changes its cellular location during the Escherichia coli division cycle. Proc Natl Acad Sci USA 2001; 98:980 - 985
- Hale CA, Meinhardt H, de Boer PA. Dynamic localization cycle of the cell division regulator MinE in Escherichia coli. EMBO J 2001; 20:1563 - 1572
- Hu Z, Lutkenhaus J. Topological regulation of cell division in E. coli. Spatiotemporal oscillation of MinD requires stimulation of its ATPase by MinE and phospholipid. Mol Cell 2001; 7:1337 - 1343
- Ghasriani H, Ducat T, Hart CT, Hafizi F, Chang N, Al-Baldawi A, et al. Appropriation of the MinD protein-interaction motif by the dimeric interface of the bacterial cell division regulator MinE. Proc Natl Acad Sci USA 2010; 107:18416 - 18421
- Ma LY, King G, Rothfield L. Mapping the MinE site involved in interaction with the MinD division site selection protein of Escherichia coli. J Bacteriol 2003; 185:4948 - 4955
- King GF, Rowland SL, Pan B, Mackay JP, Mullen GP, Rothfield LI. The dimerization and topological specificity functions of MinE reside in a structurally autonomous C-terminal domain. Mol Microbiol 1999; 31:1161 - 1169
- Zhang Y, Rowland S, King G, Braswell E, Rothfield L. The relationship between hetero-oligomer formation and function of the topological specificity domain of the Escherichia coli MinE protein. Mol Microbiol 1998; 30:265 - 273
- Ramos D, Ducat T, Cheng J, Eng NF, Dillon JAR, Goto NK. Conformation of the cell division regulator MinE: evidence for interactions between the topological specificity and anti-MinCD domains. Biochemistry 2006; 45:4593 - 4601
- Pichoff S, Vollrath B, Touriol C, Bouché JP. Deletion analysis of gene minE which encodes the topological specificity factor of cell division in Escherichia coli. Mol Microbiol 1995; 18:321 - 329
- Eng NF, Szeto J, Acharya S, Tessier D, Dillon JA. The C-terminus of MinE from Neisseria gonorrhoeae acts as a topological specificity factor by modulating MinD activity in bacterial cell division. Res Microbiol 2006; 157:333 - 344
- King GF, Shih YL, Maciejewski MW, Bains NPS, Pan BL, Rowland SL, et al. Structural basis of topological specificity function of MinE. Nat Struct Biol 2000; 7:1013 - 1017
- Kang GB, Song HE, Kim MK, Youn HS, Lee JG, An JY, et al. Crystal structure of Helicobacter pylori MinE, a cell division topological specificity factor. Mol Microbiol 2010; 76:1222 - 1231