Abstract
Apoptosis is essential for normal development. Large numbers of cells are eliminated by apoptosis in early neural development and during the formation of neural connections. However, our understanding of this life-or-death decision is incomplete, because it is difficult to identify dying cells by conventional strategies. Live imaging is powerful for studying apoptosis, because it can trace a death-fated cell throughout its lifetime. The Drosophila sensory organ development is a convenient system for studying neural-cell selection via lateral inhibition. We recently showed that about 20% of the differentiating neuronal cells die during sensory organ development, which results in the characteristic spatial patterning of the sensory organs. The eliminated differentiating neurons expressed neurogenic genes and high levels of activated Notch. Thus, live imaging allowed us to document the role of apoptosis in neural progenitor selection, and revealed that Notch activation is the mechanism determining which cells die during sensory organ development.
Apoptosis is essential for normal development. The molecular mechanisms underlying apoptosis induction are highly conserved in worms, flies and mammals.Citation1,Citation2 In addition, the physiological significance of apoptosis has been revealed, especially in neural development.Citation3 Severe brain malformation results when apoptosis is inhibited in flies or mammals,Citation4–Citation9 suggesting that apoptosis regulates the proper cell number by eliminating excess or aberrant cells during neural development. There must be a rule for determining which cells survive, and which die, but how the life-or-death fate is determined in neural development is still unclear. For example, it is uncertain whether the cells that die are selected randomly or whether the dying cells have specific characteristics. Whether a cell lives or dies is probably determined by multiple factors, including the cell's differentiation state, intercellular communications and microenvironment.
In neural-circuit formation, excess innervating post-mitotic neurons are eliminated by programmed cell death. This event is well accounted for by the neurotrophic theory, first proposed in the 1940s, in which limiting amounts of nerve growth factor (NGF) released by target tissues determines the fate of innervating neurons according to their connectivity; poorly connected neurons die from a lack of NGFCitation10–Citation12 (). However, apoptosis is also critical during the early phase of neural-cell development, during which it regulates the number of neural progenitorsCitation13 and limits the amount of proliferation in the neuroblast lineages.Citation14 However, because dying cells are mostly identified by the features of late-stage apoptosis, like nuclear fragmentation, destruction of the membrane structure and caspase activation, the properties of the cells that are fated to die have not been well defined. To elucidate the mechanisms determining the life-or-death fate in early neural development, we need to know the history of the dying cells, including their time of birth, state of differentiation and their interactions with surrounding cells. For such studies, live-imaging analysis has become a powerful tool for tracing the entire lifespan of cells eliminated through apoptosis. Moreover, the recent development of various fluorescent indicatorsCitation15–Citation18 has made it possible to detect the spatiotemporal dynamics of apoptotic signaling in live animals.
The developing Drosophila sensory organ is a convenient system for studying the process of neural cell-fate determination. The molecular mechanisms by which the sensory organ precursors (SOPs) develop from proneural cell clusters have been well studied. Notch/Delta-mediated lateral inhibition functions to generate a pattern of uniformly spaced SOPs.Citation19,Citation20 Through time-lapse imaging, we traced the development of sensory organ pattern formation. SOPs could be distinguished by their expression of the neurogenic gene neuralized. We observed the appearance of neuralized-positive cells, and the beginning of their differentiation into sensory organs. About 20% of the neuralized-positive cells died accompanied by high caspase activation and nuclear fragmentation, and were ultimately eliminated (). We then characterized the neuralized-positive cells in detail, to understand how their life-ordeath fate is determined.
Surprisingly, we found that the properties of the dying neuralized-positive cells are different from those of the surviving ones. They have characteristics intermediate between those of SOPs and epithelial cells; therefore, we named them, “SOP-like cells.” At their first division, the SOP-like cells divided symmetrically, and their daughter cells did not express other SOP markers. In contrast, normal SOPs divide asymmetrically, accompanied by the expression of neural markers like senseless or prospero. Importantly, the SOP-like cells showed high Notch activation, which was never observed in SOPs. Since Notch signaling functions to determine the non-neural cell fate in the surrounding epithelial cells, we speculated that Notch signaling also determines the celldeath fate of the SOP-like cells. In Notch heterozygous mutant flies, the proportion of SOP-like cells was decreased, and the number of adult bristles increased, indicating that the Notch activation level is a determinant for whether the neuralized-positive cells become SOPs. If a neuralized-positive cell fails to complete its neural differentiation, accompanied by inappropriate Notch activation, it is specifically eliminated via caspase-dependent cell death.
We also asked why excess neuralizedpositive cells are produced in the normal developmental context. We examined whether the SOP-like cells could function as a reserve for SOPs when neighboring SOPs are lost. Contrary to our expectation, the fate of the SOP-like cells was irreversible, even when SOPs were ablated. Instead, an alternative SOP arose from the surrounding epithelial cells. This suggested that once Notch is activated in a neuralized-positive cell, the cell's fate is invariant, and it must die to achieve the proper pattern of neural progenitors. We have not yet determined the detailed mechanisms underlying Notch activation in the SOP-like cells. One possibility is that the surrounding SOPs express Delta and activate Notch signaling in the SOP-like cells.Citation21 Another is that a cis-interaction of Notch and its ligands determines Notch's activation level in the SOP-like cells.Citation22,Citation23 A Notch indicator that allows to monitor Notch activity with high temporal resolution, which has already been applied to mammalian systems,Citation24,Citation25 would be useful for examining this unsolved issue.
Live imaging first made it possible to confirm the existence of SOP-like cells that never develop into sensory organs, by revealing the transient cell fate of neuralized-positive cells. Furthermore, by reversing the imaging movie, we could examine the detailed characteristics of these aberrant cells, like their birth timing, manner of cell division and gene-expression profile. From these analyses, we found that the excess neural progenitors do not die in a random manner during sensory organ development; instead, which neuralized-positive cells die follows a rule and depends on the level of Notch activation. Our recent report showed that apoptosis mediates the fine-tuning of SOP selection. Apoptosis-mediated cell selection has been proposed to eliminate harmful autoreactive lymphocytes in primary lymphoid tissues,Citation26 suggesting that mammals are also equipped with an apoptosis-mediated error-correction system for eliminating aberrant cells, achieving normal development and maintaining homeostasis.
Figures and Tables
Figure 1 Fine-tuning of neural cell number via programmed cell death. Neural cell number is adjusted via programmed cell death during both early neural cell-fate determination and post-mitotic neural wiring. (A) Our recent study revealed the function of programmed cell death in the selection of neural progenitors. Mis-specified SOP-like cells have characteristics intermediate between SOPs and epithelial cells. They are specifically eliminated by caspase-dependent cell death to ensure the correct sensory organ patterning. (B) Neurotrophic theory proposed the function of programmed cell death in neuronal wiring. Excess neurons compete for a limited amount of NGF provided by target tissues. Neurons that fail to bind enough of the survival factor die by programmed cell death, resulting in the proper innervation of targets.
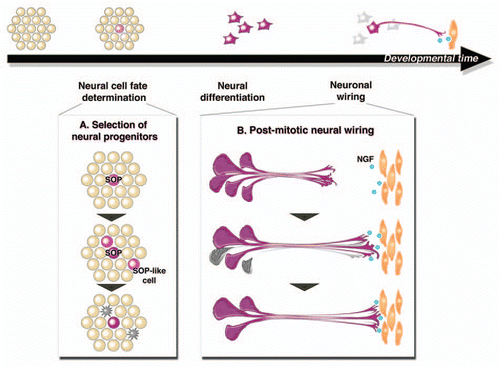
Acknowledgments
We thank E. Kuranaga for great contributions for this study, and all members of the Miura laboratory for technical support and helpful advice. We thank the University of Tokyo and Leica Microsystems Imaging Center (TLI) for imaging analysis.
Addendum to:
References
- Riedl SJ, Shi Y. Molecular mechanisms of caspase regulation during apoptosis. Nat Rev Mol Cell Biol 2004; 5:897 - 907
- Degterev A, Yuan J. Expansion and evolution of cell death programmes. Nat Rev Mol Cell Biol 2008; 9:378 - 390
- Buss RR, Sun W, Oppenheim RW. Adaptive roles of programmed cell death during nervous system development. Annu Rev Neurosci 2006; 29:1 - 35
- Hakem R, Hakem A, Duncan GS, Henderson JT, Woo M, Soengas MS, et al. Differential requirement for caspase 9 in apoptotic pathways in vivo. Cell 1998; 94:339 - 352
- Kanuka H, Sawamoto K, Inohara N, Matsuno K, Okano H, Miura M. Control of the cell death pathway by Dapaf-1, a Drosophila Apaf-1/CED-4-related caspase activator. Mol Cell 1999; 4:757 - 769
- Kuida K, Haydar TF, Kuan CY, Gu Y, Taya C, Karasuyama H, et al. Reduced apoptosis and cytochrome c-mediated caspase activation in mice lacking caspase 9. Cell 1998; 94:325 - 337
- Kuida K, Zheng TS, Na S, Kuan C, Yang D, Karasuyama H, et al. Decreased apoptosis in the brain and premature lethality in CPP32-deficient mice. Nature 1996; 384:368 - 372
- Rodriguez A, Oliver H, Zou H, Chen P, Wang X, Abrams JM. Dark is a Drosophila homologue of Apaf-1/CED-4 and functions in an evolutionarily conserved death pathway. Nat Cell Biol 1999; 1:272 - 279
- Zhou L, Song Z, Tittel J, Steller H. HAC-1, a Drosophila homolog of APAF-1 and CED-4 functions in developmental and radiation-induced apoptosis. Mol Cell 1999; 4:745 - 755
- Cowan WM. Viktor Hamburger and Rita Levi-Montalcini: the path to the discovery of nerve growth factor. Annu Rev Neurosci 2001; 24:551 - 600
- Oppenheim RW. Cell death during development of the nervous system. Annu Rev Neurosci 1991; 14:453 - 501
- Raff MC. Social controls on cell survival and cell death. Nature 1992; 356:397 - 400
- Depaepe V, Suarez-Gonzalez N, Dufour A, Passante L, Gorski JA, Jones KR, et al. Ephrin signalling controls brain size by regulating apoptosis of neural progenitors. Nature 2005; 435:1244 - 1250
- Bello BC, Hirth F, Gould AP. A pulse of the Drosophila Hox protein Abdominal-A schedules the end of neural proliferation via neuroblast apoptosis. Neuron 2003; 37:209 - 219
- Bardet PL, Kolahgar G, Mynett A, Miguel-Aliaga I, Briscoe J, Meier P, Vincent JP. A fluorescent reporter of caspase activity for live imaging. Proc Natl Acad Sci USA 2008; 105:13901 - 13905
- Koto A, Kuranaga E, Miura M. Temporal regulation of Drosophila IAP1 determines caspase functions in sensory organ development. J Cell Biol 2009; 187:219 - 231
- Takemoto K, Nagai T, Miyawaki A, Miura M. Spatio-temporal activation of caspase revealed by indicator that is insensitive to environmental effects. J Cell Biol 2003; 160:235 - 243
- Williams DW, Kondo S, Krzyzanowska A, Hiromi Y, Truman JW. Local caspase activity directs engulfment of dendrites during pruning. Nat Neurosci 2006; 9:1234 - 1236
- Heitzler P, Simpson P. The choice of cell fate in the epidermis of Drosophila. Cell 1991; 64:1083 - 1092
- Simpson P. Lateral inhibition and the development of the sensory bristles of the adult peripheral nervous system of Drosophila. Development 1990; 109:509 - 519
- Cohen M, Georgiou M, Stevenson NL, Miodownik M, Baum B. Dynamic filopodia transmit intermittent Delta-Notch signaling to drive pattern refinement during lateral inhibition. Dev Cell 2010; 19:78 - 89
- Barad O, Rosin D, Hornstein E, Barkai N. Error minimization in lateral inhibition circuits. Sci Signal 2010; 3:51
- del Alamo D, Rouault H, Schweisguth F. Mechanism and significance of cis-inhibition in Notch signalling. Curr Biol 2011; 21:40 - 47
- Masamizu Y, Ohtsuka T, Takashima Y, Nagahara H, Takenaka Y, Yoshikawa K, et al. Real-time imaging of the somite segmentation clock: revelation of unstable oscillators in the individual presomitic mesoderm cells. Proc Natl Acad Sci USA 2006; 103:1313 - 1318
- Shimojo H, Ohtsuka T, Kageyama R. Oscillations in notch signaling regulate maintenance of neural progenitors. Neuron 2008; 58:52 - 64
- Strasser A, Puthalakath H, O'Reilly LA, Bouillet P. What do we know about the mechanisms of elimination of autoreactive T and B cells and what challenges remain. Immunol Cell Biol 2008; 86:57 - 66