Abstract
The mechanism for nucleo-cytoplasmic transport of integral membrane proteins is poorly understood compared to transport of soluble molecules. We recently demonstrated that at least four distinct mechanisms can contribute to transport of integral proteins through the peripheral channels of the nuclear pore complex. One of these requires having multiple phenylalanine-glycine (FG) pairings on the integral protein. It also requires the nuclear pore complex protein Nup35, which separately contains FG repeats. FG-repeats on nuclear pore complex proteins in the central channel have been proposed to interact with FGs on transport receptors to facilitate transport of soluble proteins. Here we show that FG repeats occur quite frequently in both transmembrane and soluble proteins identified in multiple separate proteomic analyses of nuclear envelopes. We postulate that the FG repeats enable these proteins to function as their own transport receptors.
The nuclear envelope (NE) is a double-membrane system that shields the genome from cytoplasmic activities. Nonetheless, interdependence between the nucleus and the rest of the cell requires the directed exchange of material between the two compartments. Transport of soluble proteins >40–60 kDa in and out of the nucleus is thought to occur through a receptor-mediated mechanism. A nuclear localization signal (NLS) on the cargo (the protein to be transported) is recognized by the receptor (importins or exportins). The receptor-cargo complex then moves through the central channel of nuclear pore complexes (NPCs) that are inserted into the NE at perforations where the outer and inner nuclear membranes (INM) join.Citation1,Citation2 NPCs are >60 MDa structures containing multiple copies of over 30 nucleoporins.Citation3 Although mechanistic detail remains elusive, movement through the central channel is generally thought to involve iterative interactions of the transport receptors with phenylalanineglycine (FG) repeats that occur on several nucleoporins.Citation4 It is generally accepted that the FG-repeat regions of nucleoporins are unstructured and form some kind of entropic barrier to diffusion,Citation5,Citation6 but there is considerable contention as to the precise nature of the barrier and how transport receptors interact with it. An early study argued that FGs on different nucleoporins interact with FGs that occur on several transport receptors (e.g., importin ß has four FGs) to support processive movement through the channel,7 but removal of 50% of the FGs on nucleoporins did not block transport thus raising the possibility that they are not essential for transport.Citation8
Despite these controversies, transport of soluble proteins through the central channel is comparatively well characterized compared to the transport of integral membrane proteins. After their synthesis in the endoplasmic reticulum integral proteins of the INM must translocate into the nucleus. The endoplasmic reticulum is continuous with the outer membrane of the NE and so it was initially thought that proteins diffused in the membrane to where NPCs are inserted and then continue diffusing in the membrane on the outer face of the NPC to get to the INM. This idea was based on cryo-electron microscopy observation of ∼100 Å peripheral channels between the membrane and the NPC that could allow passage for transmembrane proteins with a nucleoplasmic/cytoplasmic mass <60 kDa.Citation9 Retention in the INM was suggested to depend on binding to nuclear proteins.Citation10,Citation11 Subsequent studies argued that transport is more complex including steps for licensing in the ER,Citation12 an ATP-dependent possible conformational change in the NPC,Citation13 and the functioning of several different NPC components including nucleoporins gp210, Nup188, Nup155 and transport receptors/exchange factors such as importin alpha and the Ran GTPase.Citation13–Citation16
By directly comparing 15 different integral proteins of the INM, we recently demonstrated that these transport mechanisms are independent of one another and in fact different proteins use different mechanisms for translocation.Citation17 We additionally found evidence for a translocation mechanism whereby addition of FGs to a transmembrane protein facilitated its accumulation in the INM. This facilitation was dependent upon the FG repeat-containing nucleoporin Nup35. Previous studies indicated that this nucleoporin was not likely to be positioned in the central channel of the NPC, but rather on the nucleoplasmic sideCitation18 or in the peripheral channelCitation3 where it could come in contact with integral proteins moving to the INM. Analysis of transmembrane proteins identified in a proteomic analysis of liver NEs revealed enrichment in proteins containing FGs. FGs occur infrequently in proteins and are more commonly found in the hydrophobic membrane spans of integral proteins, yet this enrichment compared to other integral proteins encoded in the genome was still observed when removing all predicted membrane spans from consideration.Citation17
Here we show further that this enrichment for FG-containing proteins is observed in separate NE fractions isolated from different tissues,Citation19,Citation20 demonstrating the universality of the observation and that it does not reflect an artifact of a particular NE purification approach (). Even more intriguingly enrichment for FG-containing soluble proteins is also observed in the isolated NEs (). In the case of the transmembrane proteins it makes sense that the small size of the peripheral channels would preclude the binding of transport receptors that tend to be themselves greater in mass than the ∼60 kDa limit these 100 Å channels could accommodate. Conventional dogma has always suggested that soluble proteins require transport receptors; however, only a miniscule percentage of nuclear proteins have been directly tested and our observation of enrichment in FG-containing soluble proteins suggests that, provided the FGnucleoporin:FGreceptor interaction model is correct, some nuclear proteins could use these FGs to similarly serve as their own transport receptors. Intriguingly, the DEAD-box helicase Dbp5 that binds RNA was also found to play an important role in RNA exportCitation21 and Dbp5 has five FGs. We observe that several RNA-binding proteins that were found in the NE datasets have 6–9 FGs,Citation22 consistent with the idea that these proteins could essentially coat mRNAs and facilitate their export by effectively acting as additional transport receptors. Their identification in NE preparations would indicate a population caught in the act of transport. Thus we propose that the appearance of FGs in proteins might serve as a backup and/or an alternative to the standard NLS and receptor-mediated mechanisms for transport.
Figures and Tables
Figure 1 Occurrence of FGs in NE proteins isolated from blood or muscle compared to other proteins encoded in the genome. As the number of FGs per protein increases on the x-axis, an enrichment of FGs in proteins from the NE fractions is observed. (A) When considering transmembrane proteins (determined with TM HMM v2.0 Citation23) with 14 or more FGs the percentage among NETs was more than 40-fold higher than in the set of transmembrane proteins from the rest of the genome. (B) Surprisingly the same analysis done on soluble proteins revealed an even greater enrichment.
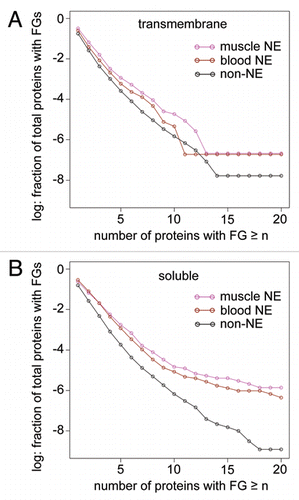
Addendum to:
References
- Prunuske AJ, Ullman KS. The nuclear envelope: form and reformation. Curr Opin Cell Biol 2006; 18:108 - 116
- Suntharalingam M, Wente SR. Peering through the pore: nuclear pore complex structure, assembly and function. Dev Cell 2003; 4:775 - 789
- Alber F, Dokudovskaya S, Veenhoff LM, Zhang W, Kipper J, Devos D, et al. The molecular architecture of the nuclear pore complex. Nature 2007; 450:695 - 701
- Wente SR, Rout MP, Blobel G. A new family of yeast nuclear pore complex proteins. J Cell Biol 1992; 119:705 - 723
- Denning DP, Patel SS, Uversky V, Fink AL, Rexach M. Disorder in the nuclear pore complex: the FG repeat regions of nucleoporins are natively unfolded. Proc Natl Acad Sci USA 2003; 100:2450 - 2455
- Lim RY, Huang NP, Köser J, Deng J, Lau KH, Schwarz-Herion K, et al. Flexible phenylalanineglycine nucleoporins as entropic barriers to nucleocytoplasmic transport. Proc Natl Acad Sci USA 2006; 103:9512 - 9517
- Rexach M, Blobel G. Protein import into nuclei: association and dissociation reactions involving transport substrate, transport factors and nucleoporins. Cell 1995; 83:683 - 692
- Strawn LA, Shen T, Shulga N, Goldfarb DS, Wente SR. Minimal nuclear pore complexes define FG repeat domains essential for transport. Nat Cell Biol 2004; 6:197 - 206
- Reichelt R, Holzenburg A, Buhle EL Jr, Jarnik M, Engel A, Aebi U. Correlation between structure and mass distribution of the nuclear pore complex and of distinct pore complex components. J Cell Biol 1990; 110:883 - 894
- Soullam B, Worman HJ. The amino-terminal domain of the lamin B receptor is a nuclear envelope targeting signal. J Cell Biol 1993; 120:1093 - 1100
- Soullam B, Worman HJ. Signals and structural features involved in integral membrane protein targeting to the inner nuclear membrane. J Cell Biol 1995; 130:15 - 27
- Saksena S, Shao Y, Braunagel SC, Summers MD, Johnson AE. Cotranslational integration and initial sorting at the endoplasmic reticulum translocon of proteins destined for the inner nuclear membrane. Proc Natl Acad Sci USA 2004; 101:12537 - 12542
- Ohba T, Schirmer EC, Nishimoto T, Gerace L. Energy- and temperature-dependent transport of integral proteins to the inner nuclear membrane via the nuclear pore. J Cell Biol 2004; 167:1051 - 1062
- Theerthagiri G, Eisenhardt N, Schwarz H, Antonin W. The nucleoporin Nup188 controls passage of membrane proteins across the nuclear pore complex. J Cell Biol 2010; 189:1129 - 1142
- Mitchell JM, Mansfeld J, Capitanio J, Kutay U, Wozniak RW. Pom121 links two essential subcomplexes of the nuclear pore complex core to the membrane. J Cell Biol 2010; 191:505 - 521
- King MC, Lusk CP, Blobel G. Karyopherin-mediated import of integral inner nuclear membrane proteins. Nature 2006; 442:1003 - 1007
- Zuleger N, Kelly DA, Richardson AC, Kerr AR, Goldberg MW, Goryachev AB, et al. System analysis shows distinct mechanisms and common principles of nuclear envelope protein dynamics. J Cell Biol 2011; 193:109 - 123
- Hawryluk-Gara LA, Shibuya EK, Wozniak RW. Vertebrate Nup53 interacts with the nuclear lamina and is required for the assembly of a Nup93-containing complex. Mol Biol Cell 2005; 16:2382 - 2394
- Korfali N, Wilkie GS, Swanson SK, Srsen V, Batrakou DG, Fairley EA, et al. The leukocyte nuclear envelope proteome varies with cell activation and contains novel transmembrane proteins that affect genome architecture. Mol Cell Proteomics 2010; 9:2571 - 2585
- Wilkie GS, Korfali N, Swanson SK, Malik P, Srsen V, Batrakou DG, et al. Several novel nuclear envelope transmembrane proteins identified in skeletal muscle have cytoskeletal associations. Mol Cell Proteomics 2011; 10:110.003129
- Tseng SS, Weaver PL, Liu Y, Hitomi M, Tartakoff AM, Chang TH. Dbp5p, a cytosolic RNA helicase, is required for poly(A)+ RNA export. EMBO J 1998; 17:2651 - 2662
- Batrakou DG, Kerr AR, Schirmer EC. Comparative proteomic analyses of the nuclear envelope and pore complex suggests a wide range of heretofore unexpected functions. J Proteomics 2008; 72:56 - 70
- Krogh A, Larsson B, von Heijne G, Sonnhammer EL. Predicting transmembrane protein topology with a hidden Markov model: application to complete genomes. J Mol Biol 2001; 305:567 - 580