Abstract
A temporal study was conducted to evaluate the dynamic complementary chromatic adaptation (CCA) response of two Fremyella diplosiphon strains—wild-type pigmentation strain SF33 and an RcaE-deficient (ΔrcaE) strain, which lacks the photosensor that regulates CCA. SF33 and ΔrcaE cultures were monitored for 15 days after transition of green-light (GL) acclimated cultures to red light (RL) and vice versa. SF33 showed similar growth irrespective of the external light quality; however, a ΔrcaE strain grew slower than SF33 under both RL and GL. Chlorophyll a (chla) content increased in both strains over time and was not much different under RL and GL indicating that chla biosynthesis is not affected significantly by light quality or RcaE function. Phycoerythrin is the sole pigment to absorb GL, whereas several pigments, i.e., allophycocyanin, phycocyanin and chla, function under RL to drive photosynthesis. SF33 compensates for this by synthesizing a higher percentage of PE under GL. The final pigment distribution in the ΔrcaE mutant was found to be more different from SF33 under GL than under RL indicating that RcaE is needed for a transitional response to RL and RL-dependent repression of PE accumulation, yet RcaE is virtually critical for both transitioning to and a full adaptation to GL.
Introduction
Cyanobacteria are prokaryotes that have an inherent ability to use solar energy to split water during photosynthesis, producing oxygen as bi-product and biomass to support themselves, as well as other organisms. These organisms are widely distributed in both terrestrial and aquatic ecosystems and possess a central position in regulating the stability of an ecosystem by performing two very important ecological functions, i.e., photosynthesis and nitrogen fixation.Citation1 Cyanobacteria absorb energy from the visible region of the solar spectrum (i.e., 400–700 nm) to perform photosynthesis; however, in aquatic ecosystems both light quality and quantity change significantly at different depth levels and affect photosynthesis.Citation2 Thus, in benthic cyanobacteria, i.e., those living at lower depths of lakes or other bodies of water, light is a limiting factor due to light absorption by chlorophyll (chl) molecules and other light-absorbing pigments in organisms found higher in the water column, as well as a reduction in light penetration to lower depths. However, cyanobacteria have evolved other pigments to absorb the available light to support photosynthesis.Citation2 Apart from chl, cyanobacteria have an additional light-harvesting complex called a phycobilisome (PBS), which transfers absorbed light energy to chl-containing reaction centers within thylakoid membranes to drive photosynthesis.Citation3
PBSs consist of pigmented phycobiliproteins (PBPs) and largely non-pigmented linker proteins.Citation4,Citation5 There are three major classes of water-soluble PBPs: red-light (RL) absorbing allophycocyanin (AP; λmax ∼650 nm), RL-absorbing phycocyanin (PC; λmax ∼620 nm), and green-light (GL) absorbing phycoerythrin (PE; λ max ∼565 nm). AP makes up the core of PBSs while PC and PE make up the outward-radiating rods of PBSs (reviewed in ref. Citation6). Linker proteins interconnect hexameric discs made up of PBPs and thus help in assembly, stability and efficient transfer of energy within PBSs, as well as energy transfer from PBSs to the photosynthetic reaction centers (reviewed in ref. Citation7). The ratio of PE and PC in PBSs depends on the ambient light quality, i.e., GL promotes the biosynthesis of red-colored, GL-absorbing PE, whereas RL promotes the biosynthesis of blue-colored, RL-absorbing PC (reviewed in ref. Citation6).
Several benthic cyanobacteria can alter PE and PC levels in their PBSs depending upon the prevalent wavelengths of light in which they are growing to maximize photosynthesis—this ecologically important phenomenon is called complementary chromatic adaptation (CCA).Citation8 However, not all cyanobacteria having PE and PC alter PE/PC ratios in response to changes in external light quality. Based on the response of these organisms during variable light exposure they have been divided into three groups.Citation8 Group I strains change neither PE nor PC in response to changes in external GL or RL and are thus incapable of chromatic adaptation.Citation8 Group II strains increase PE levels in response to GL, but do not vary PC levels in response to GL to RL shifts; however, group III strains can alter both PE and PC levels, GL exposure results in increased levels of PE and RL in higher PC levels.Citation8 Recently, group IV strains, which alter the chromophores attached to PBPs in response to changes in blue and green wavelengths, also have been described in reference Citation9 and Citation10. The freshwater cyanobacterium Fremyella diplosiphon (also called Calothrix sp. PCC 7601) belongs to group III and has been widely used to study the biochemistry and molecular biology of CCA (reviewed in ref. Citation6 and Citation7).
Based on the isolation of CCA mutants and complementation experiments, a plant phytochrome-related photoreceptor RcaE was identified in F. diplosiphon and was shown to regulate CCA under RL and GL.Citation11,Citation12 The N-terminal part of RcaE was found to be similar to the chromophore attachment domain of phytochromes and to plant ethylene receptors; however, its C-terminus resembles histidine kinase domains of two-component sensor kinases.Citation11 Biochemical and genetic evidence for the role of RcaE as a photoreceptor in CCA demonstrated that it requires a conserved cysteine residue at position 198 to covalently attach a bilin chromophore, similar to chromophore attachment in plant phytochromes.Citation11,Citation12 Based on several molecular genetic studies, it has been proposed that RcaE controls CCA by acting as a kinase under RL and a phosphatase under GL.Citation12,Citation13 In RL-grown cultures, RcaE is proposed to phosphorylate its response regulator (RR) RcaF, which further activates DNA-binding RR RcaC by transfer of a phosphate group (reviewed in ref. Citation6). Phosphorylated RcaC in turn acts as an activator for transcription of genes involved in PC biosynthesis, while acting as a repressor for the transcription of PE-encoding genes (reviewed in ref. Citation6). By contrast, in GL-grown cultures, RcaE is presumed to act as a phosphatase to remove a phosphate group from RcaF in turn leaving RcaC in an unphosphorylated state which results in a downregulation of the biosynthesis of PC and upregulation of PE biosynthesis (reviewed in ref. Citation6). In support of this model, RcaC has been shown to bind to a conserved promoter element in light-regulated genes that would account for its function as a light-regulated transcription factor.Citation14
So far nearly all studies on CCA in F. diplosiphon have been conducted on GL- or RL-acclimated cultures. Few studies have been conducted on a temporal scale and/or with light transitions apart from studies by Oelmüller et al.Citation15,Citation16 that were performed to investigate light-dependent transcript accumulation for PC-, AP- and PE-encoding genes under transitions between different light conditions, and two studies at the protein level.Citation17,Citation18 The first was a very early study by Bennett and BogoradCitation17 that examined protein synthesis under transitions from green-enriched to red-enriched light and vice versa, whereas the second reported accumulation of PE/AP and PC/AP ratios during switches of RL-acclimated cells to GL and vice versa.Citation18 The latter studied provided evidence that light-dependent regulation of RcaC levels, i.e., higher levels under RL, is required for a normal CCA response.Citation18 Thus, information about how chromatically-adapting cyanobacteria respond temporally at the pigment level during exposure to GL or RL and detailed insight into how this temporal response is regulated at the molecular level is limited. In the present study, we investigated the temporal response of CCA in wild-type (WT) pigmentation strain SF33 and RcaE-deficient mutant strain ΔrcaE of F. diplosiphon to understand the differences in their behavior during transitions from GL-to-RL and RL-to-GL over 15 days. We found that RcaE deficiency results in lower growth rates and impairments in transitioning between GL and RL growth compared to a WT pigmentation background. Thus, RcaE is required for temporal and long-term adaptive responses to changes in light quality in the chromatically-adapting F. diplosiphon.
Results
We used time-courses analyses over 15 days to study the responses of SF33 and ΔrcaE strains of F. diplosiphon under transitions between red and green light. The results from growth experiments estimated as scattering of light at 750 nm (O.D.750) showed that there was no significant difference in the growth of either strain under RL or GL conditions during the first 6 days of light exposure (). However, after 7 days whereas there was no significant difference between RL- and GL-grown cultures of the SF33 strain, growth of SF33 was significantly higher than either RL- or GL-grown cultures of the ΔrcaE strain. At this point, no significant difference was observed for ΔrcaE grown under RL vs. GL after 7 days of exposure. By 15 days, the growth was highest in the GL-grown SF33 strain followed by RL-grown SF33. However, growth of the ΔrcaE strain was significantly lower than the SF33 strain at 15 days; nevertheless, GL supported slightly higher growth of ΔrcaE than RL ().
The growth of both strains under RL and GL was also estimated by total cellular protein content and all cultures showed similar growth during the first 8 days of exposure (). Protein content was highest in the GL-grown ΔrcaE strain and lowest in RL-grown ΔrcaE; however, there was no significant difference between protein levels of GL- and RL-grown SF33 strain after 9 days of growth. After 12 and 15 days, no significant difference was noticed in protein content of SF33 and ΔrcaE strains under GL, which were slightly higher than RL-grown SF33. The protein content of ΔrcaE under RL was significantly lower than the others at 15 days ().
The effect of RL and GL on chla content in SF33 and ΔrcaE strains was determined. The results obtained showed that chla content increased over time in all cells. However, chla content did not vary much under either light condition between the two strains at each time interval ().
PBP concentrations in the SF33 strain during 15 days after transition to GL were determined. On day 0, as cells had been acclimated in RL, the PC level was highest followed by AP and then PE, which was significantly lower than the PC and AP (). As cells began to adapt to GL, there was no significant difference observed between PC, AP and PE levels after 2 or 3 days of growth under GL ( and data not shown); however, by 6 days PE was significantly higher than PC and AP levels (). During the time course examining the adaptation of SF33 to GL, PC levels were relatively stable, indicating a cessation of PC synthesis under GL; whereas PE levels increased greatly ().
The PE concentration in the SF33 strain shifted from GL to RL was significantly higher than PC and AP levels at the start of the experiment as expected for SF33 cells that were earlier adapted to GL (). There was no significant difference between PC and AP concentration at the start of the experiment (). After 4 and 5 days of growth, there was no significant difference between PC and PE concentration, and the AP level was significantly lower than both PC and PE (data not shown), indicating that PC levels were increasing in SF33 cells in response to RL exposure. At 6 days after shifting to RL, all PBPs were present at nearly equal levels (). By 7 days, the PC concentration was significantly higher than the PE and AP, which were not significantly different (data not shown). The growth of SF33 under RL for 9 days resulted in a significantly higher concentration of PC than AP, which was also higher than PE (). During the course of the transition of SF33 from GL to RL, PE levels decreased, AP showed a slight increase until day 6 at which time AP levels became relatively stable, and PC levels increased, reaching a maximum at 9 days ().
The results for PBP contents of the ΔrcaE strain on transitioning between light conditions differed from SF33. At the start of experiment, i.e., day 0, the PC level was higher than AP or PE ( and G). After 1 day of exposure of RL-acclimated cells to GL, PC levels decreased and there was no significant difference between PC and PE levels, which were both higher than AP (data not shown). No significant difference was observed between any of the three PBPs after 3 days of growth under GL (). By 15 days of growth, the concentrations of PC, AP and PE were not significantly different in the GL-grown ΔrcaE strain (). Although, minor fluctuations occurred in relative PC, PE and AP levels during the time course, ΔrcaE showed a very minor response to a switch from RL to GL as compared to SF33 (compare and ), as expected for a strain deficient in the CCA photoreceptor RcaE.
PBP concentrations in the ΔrcaE strain during its growth under RL for 15 days were similarly distinct from SF33. At the start of the time course (day 0), the PC concentration was significantly higher than both AP and PE, which were not significantly different (). This general trend was observed for growth of the ΔrcaE strain under RL throughout the 15 days observation period ().
The percentage distribution of PC, AP and PE in the total PBP pool in SF33 and ΔrcaE strains during their growth for 15 days under GL or RL was calculated (). In the SF33 strain shifted from RL to GL (day 0), PC contributed 59.5% of the total PBPs; however, at the same time PE and AP contributed 12.2% and 28.3%, respectively. Over the course of the experiment the contribution of PC to total PBP levels in GL-grown SF33 cells decreased to 17.3%, whereas PE increased to 59.7% and AP decreased slightly to 23%. By comparison, the ΔrcaE strain shifted from RL to GL exhibited 40.7% PC, 31.8% PE and 27.5% AP at the start of the transition and 36.1% PC, 31.1% PE and 32.7% AP after 15 days in GL conditions (). Thus, the ΔrcaE strain exhibited only minor differences in the relative contributions of the PBPs after transitioning from RL to GL.
At the time of shifting from GL to RL growth condition (day 0), PE contributed 66.9% of total PBPs in SF33; whereas, 17.9% and 15.3% of total PBPs consisted of AP and PC, respectively (). The percentage distribution of PE decreased to 19.6% of total PBPs in contrast with the percentage of AP and PC which increased to 35.5% and 44.9%, respectively, during the next 15 days of exposure to RL (). By comparison, in the ΔrcaE strain 44.7% of total PBPs was contributed by PC at the start of experiment (day 0), whereas 28.8% and 26.6% were contributed by AP and PE, respectively. The percentage distribution of PC, AP and PE in ΔrcaE strain did not vary much during the next 15 days of growth under RL and after 15 days of growth PC, AP and PE contributed 44.0%, 29.6% and 26.4% of total PBPs, respectively.
Discussion
In benthic environments, several cyanobacteria possess the ability to use ambient GL or RL to maximize light absorption for photosynthesis by changing the composition of pigments in their PBSs.Citation2 F. diplosiphon is a well-established model to study CCA as it responds to both RL and GL by adjusting its PBP content accordingly (reviewed in ref. Citation6 and Citation19). The growth of F. diplosiphon WT pigmentation strain SF33 was similar under both RL and GL as represented by optical density or total cellular protein content () and this can be explained by the fact that this strain shows CCA. In contrast, the ΔrcaE strain showed lower growth than the SF33 strain under GL and RL (), which supports a significant contribution of the RcaE photoreceptor to the optimization of normal growth depending on the light quality in benthic environments. The chla content was not different between SF33 and ΔrcaE strains under either light condition indicating that neither RcaE nor light quality significantly influence chla biosynthesis (). The PBPs, therefore, and not chla, are the major contributors to fine-tuning light absorption and energy production for optimized growth. The RcaE photoreceptor regulates the biosynthesis of PE or PC under GL or RL, respectively,Citation11,Citation12 to fuel optimal photosynthesis and growth under the prevalent light condition.Citation20 In RL conditions, light is absorbed by a pool of photosynthetic pigments, including PC, AP and chla; however, under GL, PE is the sole pigment that can absorb available light photons.Citation20 F. diplosiphon compensates for this by synthesizing higher levels of PE under GL conditions to fully utilize the available photons.Citation20 It has been also shown that in F. diplosiphon, cell shape changes during CCA, followed by pigment changes.Citation21 It has been hypothesized that the larger brick-shaped cells observed under GL can allow for the accumulation of more total PBPs, and thus sufficient PE, than spherical-shaped cells observed under RL.Citation7
Several studies have been conducted to study pigment changes and signal transduction during CCA (reviewed in ref. Citation6 and Citation7). However, prior studies nearly exclusively reported assessment of GL- or RL-acclimated cultures and studies of the temporal response to changes in light at the pigment level have been limited to an early study by Bennett and BogoradCitation17 and a recent study by Li and Kehoe.Citation18 In the former study, assessment of changes in pigment accumulation for cultures shifted from red-enriched growth to green-enriched growth and vice versa were limited to ∼100 h (or ∼4 days) after switching from RL to GL and ∼150 hours (or ∼6 days) after switching for GL to RL;Citation17 whereas in the latter study cultures at mid-logarithmic phase were assessed for ∼180 h (or ∼7.5 days) after switching between light conditions.Citation18 Studies investigating the impact of light-quality-dependent temporal changes at the level of the accumulation of transcripts encoding PBPs have been reported in reference Citation15 and Citation16. These studies revealed that transcript levels for genes encoding constitutive PC, AP and the core linker polypeptide were virtually constant when GL- or RL-acclimated cultures were shifted to RL or GL, respectively.Citation15,Citation16 However, under different light conditions, the transcript that encodes constitutive PC was found to be higher by a factor of three in RL-acclimated cells and thus it was concluded that constitutive PC can also be induced by RL but to a lesser degree than the inducible PC.Citation22 In contrast, the transcripts that encode inducible PC were abundant in RL-acclimated cells and were scarcely present in GL-acclimated cells; however, PE-encoding transcripts were present in both RL- and GL-acclimated cells but were 10-fold higher under GL.Citation15 The results from shifting RL-acclimated cultures to GL and vice versa revealed that the cessation of transcription of PC-encoding transcripts is more rapid than that of PE-encoding genes—the change in PC-encoding transcripts is completed within 2 h of transfer after transition from RL to GL or vice versa.Citation15 By comparison, PE-encoding transcripts accumulated to their maximum after 3 h of transfer from RL to GL, yet even after 15 h of transfer from GL to RL, PE transcripts were higher than RL-acclimatized cells.Citation15 Thus, transcription of PE-encoding genes continue for some time after transfer to RL.Citation15
In the present study, we observed the same trend at the PBP level in SF33 cultures that were grown from very early logarithmic phase when shifted from GL to RL and vice versa (). However, changes were noticed after several days as expected rather than the hours observed for the mRNA.Citation15 PE accumulation increased slightly after transfer from GL to RL (data not shown), reflecting the slower shut down of the transcription of genes encoding PE.Citation15 Under these conditions, PC levels increased greatly, resulting in an overall decrease in PE/PC ratio over the 15 day acclimation to RL (see ). When shifted from RL to GL, PC levels were not altered significantly in SF33, whereas PE levels increased greatly (). These changes result in an overall increased in the PE/PC ratios over the time course (), and are in agreement with the observations reported during shorter time courses.Citation17
The response of the ΔrcaE mutant strain was different from SF33, under both light conditions. After 15 days of growth under GL, the ΔrcaE strain exhibited equal levels of both PE and PC, indicating a requirement for RcaE in regulating the relative amounts of PBPs that accumulate during CCA under GL ().Citation11,Citation12 Notably, under RL at 15 days, the ΔrcaE strain had higher levels of PC similar to SF33, yet had a higher percentage of PE than SF33 (). This indicated that the RcaE-deficient mutant is more different from WT under GL than RL when considering the transitory and long-term response of cells to light shifts. In this regard, though lacking any temporal response to RL, the ΔrcaE mutant appears more similar to the SF33 strain by 15 days. This may be explained by the fact that RcaE purportedly acts as a kinase under RL and activates expression of PC by phosphorylating RR RcaC; however, cyanobacterial genomes possess several kinasesCitation23,Citation24 and it may be possible that even in the absence of RcaE some other kinases unspecifically activate RcaC by phosphorylation under RL and thus maintain PC biosynthesis even in the absence of RcaE and largely independently of RL as PC levels were already elevated at the start of the transition to RL.
In conclusion, WT pigmentation strain SF33 that exhibits a normal CCA response shows similar growth as measured by optical density or total cellular protein content irrespective of the light conditions. However, a mutant lacking functional RcaE and thus disrupted in CCA grows slower confirming the contribution of RcaE-dependent regulation of CCA in determining organismal fitness in benthic environments where both light quality and quantity play a significant role. RcaE-deficient cells still exhibit a response to GL early in the transition from RL to GL. Notably, prior studies demonstrated a response to GL in an rcaE mutant at the level of cpeCDE transcription,Citation25 which was the first evidence of an independent green-light responsive system. This system has been named Cgi for control of green-light induction of genes (reviewed in ref. Citation6). Here we see evidence of such a system at the level of regulation of protein accumulation—PE levels in RcaE-deficient cells shift in response to GL up to 3 days after a light transition. After 3 days, the pigment composition of the RcaE-deficient mutant was much different from SF33 under transition to GL indicating that RcaE is essential for full adaptation to GL conditions.
Materials and methods
Experimental organisms and growth conditions.
Fremyella diplosiphon WT pigmentation strain SF33,Citation26 and RcaE-deficient mutant strain (ΔrcaE or Bk14 Citation11) were used in this study. Both strains were grown in BG-11 mediumCitation27 containing 10 mM HEPES at pH 8.0 (hereafter BG-11/HEPES) with continuous shaking at 175 rpm at 28°C as previously described in reference 21. The cultures were subjected to growth under either GL or RL at an intensity of ∼10–15 µmolm−2s−1. GL and RL sources were those previously reported in reference Citation21. The intensity of the light used in this study was measured with LI-250A light meter (LI-COR, Lincoln, NE) equipped with a quantum sensor. To study the temporal adaptation of F. diplosiphon cells to RL and GL, cultures of SF33 and ΔrcaE strains grown in RL and GL to an optical density at 750 nm (O.D.750) of ∼1.0, were diluted and transferred to GL and RL, respectively. Time-course experiments were started at an O.D.750 of ∼0.2 and different growth parameters and pigment profiles assessed over 15 days. In each replicate, samples were collected immediately after the light transitions and daily for the first 9 days and thereafter at 12 and 15 days. Growth results are shown for all the days; however, pigment concentrations and percentage distribution of PBPs are shown for 0, 3, 6, 9, 12 and 15 days.
Growth measurements.
The growth of SF33 and ΔrcaE strains under RL and GL was estimated by O.D.750 measured using a SpectraMax M2 microplate reader (Molecular Devices, Sunnyvale, CA). For spectrophotometer measurement, 500 µl of cell cultures were collected and diluted with an equal volume of BG-11/HEPES medium to measure O.D.750. The final values were multiplied by dilution factor.
Total protein content measurements.
Total soluble protein was extracted by CelLytic™ B cell lysis reagent (Sigma, Cat. No. B7435) according to the manufacturer's protocol. Total protein content was determined from 1 ml of culture using the BCA™ protein assay kit following the manufacturer's instructions (Pierce, Cat. No. 23227).
Chlorophyll a measurements.
The concentration of chlorophyll a (chla) was estimated from 1 ml of culture according to the method detailed by Tandeau de Marsac and Houmard.Citation28 In brief, after centrifugation at 13,000x g for 5 min, cell pellets were dissolved in 500 µl of 90% methanol (v/v) and chla extraction performed with constant shaking at 4°C for 1 h. Afterwards, supernatants were collected and extractions repeated with the remaining pellet. Pooled supernatants were used to measure absorbance at 665 nm (A665) and A665 values used to calculate the chla concentration.Citation28
Phycobiliprotein extraction and quantification.
PBPs were extracted from 1 ml of cell culture according to Kahn et al. Briefly, cells were pelleted by centrifugation at 13,000x g for 5 min and immediately frozen in liquid nitrogen. Cell pellets were stored at −80°C until further processing. PBPs were extracted from thawed cell pellets in 1 ml of ice cold STES buffer (50 mM Tris-HCL, pH 8.0; 50 mM NaCl; 10 mM EDTA, pH 8.0; 250 mM Sucrose) containing 5 mgml−1 lysozyme with constant shaking at room temperature for 30 min in darkness. After centrifugation at 13,000x g for 5 min, supernatants were used to determine A565, A620 and A650. Absorbance values were used to quantify AP, PC and PE according to the formulas given by Tandeau de Marsac and Houmard.Citation28 PBPs values were normalized to chla as described in reference Citation21.
Statistical analysis.
Time-course analysis was repeated twice to confirm the reproducibility of the results and all results are presented as a mean value of three replicates. Results were analyzed using one-way ANOVA and once significant differences were detected post hoc multiple comparisons were performed using the Tukey test. The significance level was set at 0.05 for all analyses and all tests were performed using OpenStat statistical software [version 10.01.08; W.G. Miller (statpages.org/miller/openstat)].
Abbreviations
AP | = | allophycocyanin |
CCA | = | complementary chromatic adaptation |
chl | = | chlorophyll |
GL | = | green light |
PBP | = | phycobiliprotein |
PBS | = | phycobilisomes |
PC | = | phycocyanin |
PE | = | phycoerythrin |
RL | = | red light |
Figures and Tables
Figure 1 Growth of wild-type pigmentation strain SF33 and RcaE-deficient mutant (ΔrcaE) strain of F. diplosiphon under shifts between green and red light. (A) Cell growth was estimated as scattering of light (i.e., optical density or O.D.) at 750 nm and (B) total cellular protein content (µg ml−1) during 15 days of exposure to green light (GL) and red light (RL) after transition of RL-acclimated cultures to GL and vice versa.
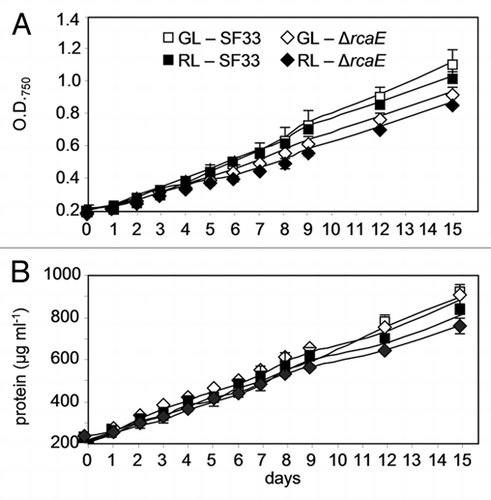
Figure 2 Chlorophyll content in wild-type pigmentation strain SF33 and RcaE-deficient mutant (ΔrcaE) strain of F. diplosiphon under shifts between green and red light. Chlorophyll a (chla) concentration was determined during 15 days of exposure to green light (GL) and red light (RL) after transition of RL-acclimated cultures to GL and vice versa. Asterisks represent significant difference from 0 day (*p < 0.05), similar alphabets over the bars indicate homogenous mean group while a line over the bars show no significant difference (p > 0.05) between treatment at each time interval.
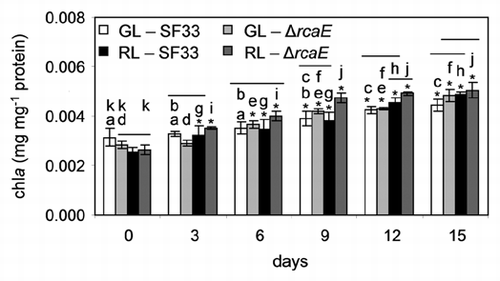
Figure 3 Phycobiliprotein content in SF33 wild-type pigmentation strain of F. diplosiphon under shifts between green and red light. Concentration of phycobiliproteins, including phycocyanin (PC), allophycocyanin (AP) and phycoerythrin (PE) were determined during 15 days of exposure to green light (GL) and red light (RL) after transition of RL-acclimated cultures to GL and vice versa. Similar alphabets over the bars represent no significant difference between pigment levels (p > 0.05).
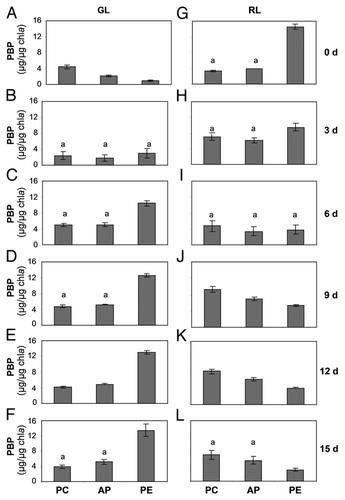
Figure 4 Phycobiliprotein content in RcaE-deficient mutant (ΔrcaE) strain of F. diplosiphon under shifts between green and red light. Concentration of phycobiliproteins, including phycocyanin (PC), allophycocyanin (AP) and phycoerythrin (PE) were determined during 15 days of exposure to green light (GL) and red light (RL) after transition of RL-acclimated cultures to GL and vice versa. Similar alphabets over the bars represent no significant difference between pigment levels (p > 0.05).
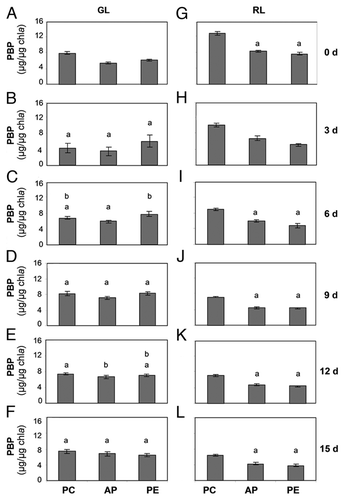
Figure 5 Phycobiliprotein distributions in wild-type pigmentation strain SF33 and RcaE-deficient mutant (ΔrcaE) strain under shifts between green and red light. Percentage distribution of phycocyanin (PC), allophycocyanin (AP) and phycoerythrin (PE) were calculated for strains during 15 days of exposure to green light (GL) and red light (RL) after transition of RL-acclimated cultures to GL and vice versa.
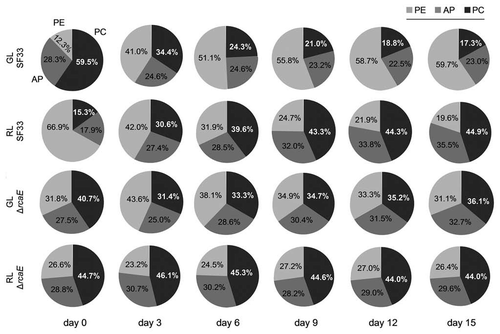
Acknowledgments
We thank Dr. Bagmi Pattanaik for critically reading and commenting on the manuscript. This research was supported by the USDepartment of Energy (Chemical Sciences, Geosciences and Biosciences Division, Office of Basic Energy Sciences, Office of Science, grant no. DE-FG02-91ER20021 to B.L.M.) and a CAREER award from the National Science Foundation (grant no. MCB-0643516 to B.L.M.).
References
- Berman-Frank I, Lundgren P, Falkowski P. Nitrogen fixation and photosynthetic oxygen evolution in cyanobacteria. Res Microbiol 2003; 154:157 - 164
- Postius C, Neuschaefer-Rube O, Haid V, Böger P. N2-fixation and complementary chromatic adaptation in non-heterocystous cyanobacteria from Lake Constance. FEMS Microbiol Ecol 2001; 37:117 - 125
- Bogorad L. Phycobiliproteins and complementary chromatic adaptation. Annu Rev Plant Physiol 1975; 26:369 - 401
- Gantt E. Phycobilisomes. Annu Rev Plant Physiol 1981; 32:327 - 347
- Glazer AN. Phycobilisomes: structure and dynamics. Annu Rev Microbiol 1982; 36:173 - 198
- Kehoe DM, Gutu A. Responding to color: The regulation of complementary chromatic adaptation. Annu Rev Plant Biol 2006; 57:127 - 150
- Montgomery BL. Shedding new light on the regulation of complementary chromatic adaptation. Cent Eur J Biol 2008; 3:351 - 358
- Tandeau de Marsac N. Bogorad L, Vasil IK. Chromatic adaptation by cyanobacteria. Cell Culture and Somatic Cell Genetics of Plants 1991; New York Academic Press Inc 417 - 446
- Everroad C, Six C, Partensky F, Thomas JC, Holtzendorff J, Wood AM. Biochemical bases of type IV chromatic adaptation in marine Synechococcus spp. J Bacteriol 2006; 188:3345 - 3356
- Palenik B. Chromatic adaptation in marine Synechococcus strains. Appl Environ Microbiol 2001; 67:991 - 994
- Kehoe DM, Grossman AR. Similarity of a chromatic adaptation sensor to phytochrome and ethylene receptors. Science 1996; 273:1409 - 1412
- Terauchi K, Montgomery BL, Grossman AR, Lagarias JC, Kehoe DM. RcaE is a complementary chromatic adaptation photoreceptor required for green and red light responsiveness. Mol Microbiol 2004; 51:567 - 577
- Kehoe DM, Grossman AR. New classes of mutants in complementary chromatic adaptation provide evidence for a novel four-step phosphorelay system. J Bacteriol 1997; 179:3914 - 3921
- Li L, Alvey RM, Bezy RP, Kehoe DM. Inverse transcriptional activities during complementary chromatic adaptation are controlled by the response regulator RcaC binding to red and green light-responsive promoters. Mol Microbiol 2008; 68:286 - 297
- Oelmüller R, Conley PB, Federspiel NA, Briggs WR, Grossman AR. Changes in accumulation and synthesis of transcripts encoding phycobilisome components during acclimation of Fremyella diplosiphon to different light qualities. Plant Physiol 1988; 88:1077 - 1083
- Oelmüller R, Grossman AR, Briggs WR. Photoreversibility of the effect of red and green light pulses on the accumulation in darkness of mRNAs coding for phycocyanin and phycoerythrin in Fremyella diplosiphon. Plant Physiol 1988; 88:1084 - 1091
- Bennett A, Bogorad L. Complementary chromatic adaptation in a filamentous blue-green alga. J Cell Biol 1973; 58:419 - 435
- Li L, Kehoe DM. Abundance changes of the response regulator RcaC require specific aspartate and histidine residues and are necessary for normal light color responsiveness. J Bacteriol 2008; 190:7241 - 7250
- Kehoe DM. Chromatic adaptation and the evolution of light color sensing in cyanobacteria. Proc Natl Acad Sci USA 2010; 107:9029 - 9030
- Campbell D. Complementary chromatic adaptation alters photosynthetic strategies in the cyanobacterium Calothrix. Microbiology 1996; 142:1255 - 1263
- Bordowitz JR, Montgomery BL. Photoregulation of cellular morphology during complementary chromatic adaptation requires sensor-kinase-class protein RcaE in Fremyella diplosiphon. J Bacteriol 2008; 190:4069 - 4074
- Conley PB, Lemaux PG, Lomax TL, Grossman AR. Genes encoding major light-harvesting polypeptides are clustered on the genome of the cyanobacterium Fremyella diplosiphon. Proc Natl Acad Sci USA 1986; 83:3924 - 3928
- Zhang X, Zhao F, Guan X, Yang Y, Liang C, Qin S. Genome-wide survey of putative serine/threonine protein kinases in cyanobacteria. BMC genomics 2007; 8:395 - 410
- Wang L, Sun YP, Chen WL, Li JH, Zhang CC. Genomic analysis of protein kinases, protein phosphatases and two-component regulatory systems of the cyanobacterium Anabaena sp. strain PCC 7120. FEMS Microbiol Lett 2002; 217:155 - 165
- Seib LO, Kehoe DM. A turquoise mutant genetically separates expression of genes encoding phycoerythrin and its associated linker peptides. J Bacteriol 2002; 184:962 - 970
- Cobley JG, Zerweck E, Reyes R, Mody A, Seludo-Unson JR, Jaeger H, et al. Construction of shuttle plasmids which can be efficiently mobilized from Escherichia coli into the chromatically adapting cyanobacterium, Fremyella diplosiphon. Plasmid 1993; 30:90 - 105
- Allen MM. Simple conditions for growth of unicellular blue-green algae on plates. J Phycol 1968; 4:1 - 4
- Tandeau de Marsac N, Houmard J. Complementary chromatic adaptation: physiological conditions and action spectra. Methods Enzymol 1988; 167:318 - 328
- Kahn K, Mazel D, Houmard J, Tandeau de Marsac N, Schaefer MR. A role for cpeYZ in cyanobacterial phycoerythrin biosynthesis. J Bacteriol 1997; 179:998 - 1006