Abstract
We recently reported evidence implicating fatty-acid binding protein (Fabp) in the control of sleep and memory formation. We used Drosophila melanogaster to examine the relationship between sleep and memory through transgenic overexpression of mouse brain-Fabp, Fabp7, or the Drosophila Fabp homologue, (dFabp). The key findings are that, (1) a genetically induced increase in daytime consolidated sleep (naps) correlates with an increase in cognitive performance, and (2) a late “window” of memory consolidation occurs days after the traditionally understood “synaptic” consolidation. Exactly how Fabp-signaling may be involved in converting normal to enhanced long-term memory (LTM) is not known. Here we describe additional data which support relative subcellular compartmental localization of Fabp in regulating stage associations of different forms of memory in Drosophila. Anesthesia resistant memory (ARM) is a longer lasting memory that is produced by massed training, but unlike LTM produced by spaced training, it is insensitive to protein synthesis inhibitors and does not persist as long. We observed that the ratio of ARM to LTM performance index of Fabp7-transgenic flies is proportional to the relative cytoplasmic to nuclear Fabp7 expression level. These data suggest a common lipid-signaling cascade exists between phases of memory formation previously thought to be molecularly distinct.
Distinguishing various forms of memory and the cellular and molecular processes that underlie the conversion of short- to long-term memory are active areas of research. The Drosophila model offers a unique and powerful genetic tool in order to evaluate these processes. The tractability of Drosophila for the study of complex behavior becomes even more critical when considering the relationship of memory in the context of circadian rhythms and sleep.Citation1,Citation2 Previous studies have shown important behavioral and genetic features involved in plasticity and learning-related processes with sleep/wake regulation using the Drosophila model.Citation3–Citation6 However, molecular mechanisms responsible for a relay between various memory consolidation stages, particularly in the context of sleep behavior, remain largely unknown.Citation7
In our previous study, we showed that transgenic flies expressing the mouse cycling gene Fabp7 can regulate sleep, and later periods of long-term memory consolidation using the olfactory avoidance conditioning paradigm.Citation8 This learning paradigm is an example of classical conditioning used in Drosophila, and has been critical in discriminating genetic correlates for distinct phases of memory.Citation9 For example, anesthesia-resistant memory (ARM) is a longer lasting memory that, as its name implies, is insensitive to disruption via anesthesia, but is a form of memory that is resistant to protein synthesis inhibitors. In Drosophila olfactory avoidance conditioning, ARM is produced by massed training, and can last for multiple days with 10 consecutive training sessions repeated sequentially.Citation10 By comparison, long-term memory (LTM), which persists at least one week, differs from ARM since it is sensitive to protein synthesis inhibitors. This longer-lasting memory is generated by dispersing 10 training sessions with 15 min inter-trial interval rest periods. ARM and LTM are believed to be generated independently by distinct genetic pathways, and therefore are thought to coexist as two unique and disparate phases of long-lasting memory.Citation10,Citation11
Here, we were interested in determining whether Fabp7 expression could also influence the consolidation period of ARM as it does for LTM. Fabp7-expressing and wild-type w(isoCJ1) background flies were trained in the olfactory conditioning paradigm to elicit ARM following 10 sessions of massed training (10XM). Flies maintained at 20°C and 30°C, but not at 25°C, for 4 days following 10XM training elicited a statistically significant enhancement of ARM compared to w(isoCJ1) (). A comparison of the amount of Fabp7 protein expression at each of these three temperatures shows a differential level of relative subcellular localization of protein (). Our previous findings suggested that an increase in Fabp7 nuclear protein expression (shown again here in ) correlated with LTM formation following training.Citation8 We therefore compared the relative performance of ARM to the LTM we previously described at each of these temperatures in order to determine whether a relationship exists in these two forms of memory. The w(isoCJ1) flies show a progressive increase in the performance ratio of ARM:LTM with temperature, while Fabp7 expressing flies show an enhanced ratio of performance at the temperature extremes (). We next compared the relative Fabp7 subcellular localization with this performance ratio of memory. Plotting the ratio of ARM:LTM performance against the ratio of cytoplasmic:nuclear Fabp7 expression (from ) revealed a proportionate correlation of memory and subcellular localization with temperature (). Therefore, increasing the relative level of Fabp7 between the cytoplasm and the nucleus during the consolidation period following training can enhance both ARM and LTM, respectively. Together, these data suggest that ARM and LTM may share some common lipid-mediated pathways of regulation.
It has previously been shown that ARM and LTM in Drosophila memory formation are genetically distinct, since disruptions of the transcription factors dCREB2, Adf1, or Notch block LTM without affecting ARM,Citation12–Citation16 and LTM remains intact in radish mutants, which disrupt ARM.Citation13,Citation17 Since these studies primarily focused on disruption of memory rather than enhancement, the conclusion that ARM and LTM are genetically distinct may not be completely unequivocal. Our evidence presented here suggests that the relative abundance of a small lipid transport molecule between the cytoplasmic and nuclear compartments can regulate the formation of different types of longer-lasting memory after training. A relative increase in Fabp7 expression in the cytoplasm during the consolidation period following massed training produces ARM enhancement (), while a relative increase in the nucleus produces an enhancement in LTM.Citation8 Since Fabp7 flies have reduced sleep at lower temperatures,Citation8 this suggests cytoplasmic lipidbinding processes may reduce the need for sleep, which could be beneficial for the memory enhancement observed during ARM consolidation (). Further, the enhancement in LTM consolidation generated by an increase in Fabp7 in the nucleusCitation8 could reflect lipid-signaling in transcriptional processes important for sleep and long-term memory (). Our data suggest that cytoplasmic processes modulate ARM, while nuclear processes are important for regulating LTM, and that lipid-signaling is involved in both ARM and LTM during the consolidation period post-training.
Genetic evidence for lipid metabolism in the regulation of learning impairments and the homeostatic response to sleep loss was recently shown.Citation18 Using cDNA microarrays, changes in gene expression of another lipid-binding protein, the Retinoid Fatty-acid binding protein, Rfabg, have been observed in Drosophila following ARM and LTM trainingCitation19 and following changes in sleep/wake homeostasis.Citation20 Whether altering Fabp levels can regulate changes in sleep induced by experience-dependent plasticity, cognitive impairments induced by sleep deprivation, or the homeostatic response to sleep loss, remain open questions. The identification of specific lipid species involved in the regulation of sleep/wake and during memory consolidation will be important avenues of future research. Our data suggest Fabp signaling is able to modulate memory consolidation in two previously understood to be distinct forms of longerlasting memory in Drosophila. While our data present the novel finding that a common protein can regulate consolidation of both ARM and LTM, the difference in relative Fabp7 levels in the cytoplasm versus the nucleus still suggest distinct cellular functions, and therefore may not necessarily support the “exclusive memory hypothesis.”Citation10,Citation21 However it is equally unclear whether these Fabp-mediated events coexist, or instead retain independent cellular mechanisms by parallel processes.Citation10 Further determination of the cells and circuits that differentiate ARM and LTM, particularly in the context of Fabp signaling and relative subcellular localization will be required before any conclusions are made on the degree of exclusivity between these two forms of memory. What appears evident is that a role of nuclear Fabp localization may serve as a “gatekeeper” during later consolidation phases of LTM. Thus, discovery of specific lipids bound by Fabps which signal to nuclear orphan receptors, and subsequent changes in transcription of genes during the consolidation period are critical for our understanding of LTM stability. These active areas of research will undoubtedly yield novel targets involved in the cell signaling processes important for neural fitness and overall cognitive health.
Materials and Methods
Transgenic flies, olfactory avoidance conditioning, and protein gel blot analysis were performed with methods and reagents as previously described.Citation8 Anesthesia resistant memory by 10X massed training was performed according to previously published methods.Citation11
Figures and Tables
Figure 1 Fabp7 flies have enhanced ARM formation. Fabp7 flies elicit Anesthesia Resistant Memory (ARM) enhancement following 10X Massed (10XM) training when maintained at lower (20°C) and higher temperatures (30°C) for 4 days prior to testing, compared to controls. Grey bars, Fabp7, open bars, w(isoCJ1) background strain. Results are mean ± s.e.m.; n = 8 groups. * P < 0.05, t-test Fabp7 vs. w(isoCJ1).
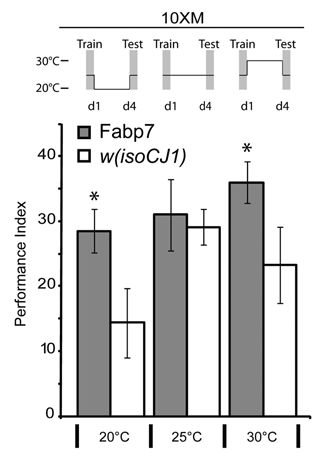
Figure 2 Subcellular localization of Fabp7 correlates with temperature. Protein gel blots of Fabp7 protein expression in Fabp7 fly heads staged at 20°, 25°, and 30°C for seven days, compared to immunoreactive background, in cytoplasmic and nuclear fractions previously publishedCitation8 (lysate sample: ∼50–100 fly heads per lane). Image contrast has been inverted for comparison.
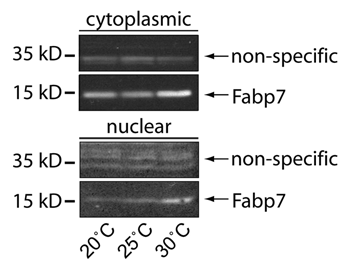
Figure 3 Relative subcellular localization of Fabp7 correlates with memory formation. (A) Ratio of Performance Index (PI) scores from ARM data (), to Long-Term Memory following (10X Spaced) training previously described.Citation8 Grey bars, Fabp7, open bars, w(isoCJ1) background strain. Results are mean ± s.e.m.; n = 8–10 groups. * P < 0.05, t-test, vs. Fabp7 at 25°C. (B) Ratio of Performance Index (PI) scores in () for Fabp7 flies (diamonds; y-axis left) shows a correlation of performance with ratio of cytoplasmic to nuclear (Cyto/Nuc) Fabp7 protein expression (black circles; y-axis right; from ).
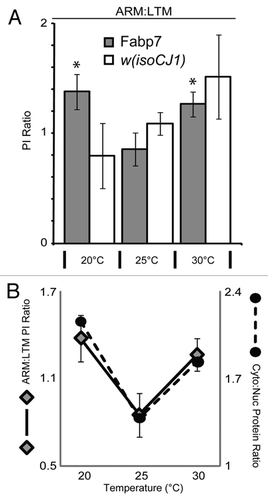
Figure 4 Proposed model for Fabp cellular processes regulating sleep and memory formation. Upon neuronal activation, wakefulness, or learning events (such as repeated stimulation during ARM training), free fatty-acids (FFA) are liberated from the cell membrane or other lipid stores, and can modulate sleep/wake. Increases in the relative amount of cytoplasmic Fabps bind more FFA and thereby regulate cellular responses to lipid signals. Following LTM training, additional FFA are liberated, to which Fabp will sequester and transport to the nucleus in order to activate fatty-acid nuclear receptors (FA-NR) and regulate transcription of downstream fatty-acid responsive genes (FA-rgs). This altered transcription will generate a change in protein synthesis of FA-rgs (FA-rps), which in turn modulates sleep/wake behavior.
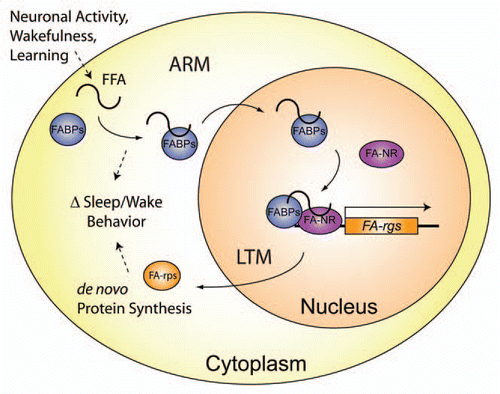
Acknowledgments
We would like to thank H. Zhou for expert technical assistance with Westerns, and A. Pack and the Center for Sleep and Circadian Neurobiology for support on this manuscript. J.R.G. is currently supported by NIH T32 HL07713.
Addendum to:
References
- Gerstner J R, Yin JC. Circadian rhythms and memory formation. Nat Rev Neurosci 2010; 11:577 - 588
- Gerstner JR, Lyons LC, Wright KP Jr, Loh DH, Rawashdeh O, Eckel-Mahan KL, et al. Cycling behavior and memory formation. J Neurosci 2009; 29:12824 - 12830
- Ganguly-Fitzgerald I, Donlea J, Shaw PJ. Waking experience affects sleep need in Drosophila. Science 2006; 313:1775 - 1781
- Donlea JM, Ramanan N, Shaw PJ. Use-dependent plasticity in clock neurons regulates sleep need in Drosophila. Science 2009; 324:105 - 108
- Gilestro GF, Tononi G, Cirelli C. Widespread changes in synaptic markers as a function of sleep and wakefulness in Drosophila. Science 2009; 324:109 - 112
- Li X, Yu F, Guo A. Sleep deprivation specifically impairs short-term olfactory memory in Drosophila. Sleep 2009; 32:1417 - 1424
- Hernandez PJ, Abel T. A molecular basis for interactions between sleep and memory. Sleep Med Clin 2011; 6:71 - 84
- Gerstner JR, Vanderheyden WM, Shaw PJ, Landry CF, Yin JC. Fatty-Acid binding proteins modulate sleep and enhance long-term memory consolidation in Drosophila. PLoS One 2011; 6:e15890
- Tully T, Quinn WG. Classical conditioning and retention in normal and mutant Drosophila melanogaster. J Comp Physiol A 1985; 157:263 - 277
- Margulies C, Tully T, Dubnau J. Deconstructing memory in Drosophila. Curr Biol 2005; 15:R700 - R713
- Tully T, Preat T, Boynton SC, Del Vecchio M. Genetic dissection of consolidated memory in Drosophila. Cell 1994; 79:35 - 47
- DeZazzo J, Tully T. Dissection of memory formation: from behavioral pharmacology to molecular genetics. Trends Neurosci 1995; 18:212 - 218
- Yin JC, Wallach JS, Del Vecchio M, Wilder EL, Zhou H, Quinn WG, et al. Induction of a dominant negative CREB transgene specifically blocks long-term memory in Drosophila. Cell 1994; 79:49 - 58
- DeZazzo J, Sandstrom D, de Belle S, Velinzon K, Smith P, Grady L, et al. nalyot, a mutation of the Drosophila myb-related Adf1 transcription factor, disrupts synapse formation and olfactory memory. Neuron 2000; 27:145 - 158
- Ge X, Hannan F, Xie Z, Feng C, Tully T, Zhou H, et al. Notch signaling in Drosophila long-term memory formation. Proc Natl Acad Sci USA 2004; 101:10172 - 10176
- Presente A, Boyles RS, Serway CN, de Belle JS, Andres AJ. Notch is required for long-term memory in Drosophila. Proc Natl Acad Sci USA 2004; 101:1764 - 1768
- Dudai Y, Jan YN, Byers D, Quinn WG, Benzer S. dunce, a mutant of Drosophila deficient in learning. Proc Natl Acad Sci USA 1976; 73:1684 - 1688
- Thimgan MS, Suzuki Y, Seugnet L, Gottschalk L, Shaw PJ. The perilipin homologue, lipid storage droplet 2, regulates sleep homeostasis and prevents learning impairments following sleep loss. PLoS Biol 2010; 8:e1000466
- Dubnau J, et al. The staufen/pumilio pathway is involved in Drosophila long-term memory. Curr Biol 2003; 13:286 - 296
- Zimmerman JE, Rizzo W, Shockley KR, Raizen DM, Naidoo N, Mackiewicz M, et al. Multiple mechanisms limit the duration of wakefulness in Drosophila brain. Physiol Genomics 2006; 27:337 - 350
- Isabel G, Pascual A, Preat T. Exclusive consolidated memory phases in Drosophila. Science 2004; 304:1024 - 1027