Abstract
Vesicle-mediated cargo transport within the endomembrane system requires precise coordination between adaptor molecules, which recognize sorting signals on substrates, and factors that promote changes in membrane architecture. At endosomal compartments, a set of protein complexes collectively known as the ESCRT machinery sequesters transmembrane cargoes that harbor a ubiquitin modification and packages them into vesicles that bud into the endosome lumen. Several models have been postulated to describe this process. However, consensus in the field remains elusive. Here, we discuss recent findings regarding the structure and function of the ESCRT machinery, highlighting specific roles for ESCRT-0 and ESCRT-III in regulating cargo selection and vesicle formation.
The biogenesis of transport vesicles requires a series of membrane remodeling events that typically involve cytoplasmic proteins that peripherally associate with lipid bilayers. Additionally, changes in membrane composition also play critical roles in this process, stabilizing energetically unfavorable intermediates necessary to form a budded structure. In particular, endosomal compartments undergo a wide variety of remodeling events. Membrane tubulation at endosomes, mediated by BAR domain proteins and members of the EHD family of ATPases, has been shown to promote endocytic recycling to the cell surface.Citation1-Citation4 In contrast, components of the ESCRT (Endosomal Sorting Complex Required for Transport) machinery have been implicated in the formation of intralumenal vesicles (ILVs), which exhibit negative membrane curvature and bud away from the cytoplasm toward the endosome interior.Citation5-Citation7 In topologically similar processes, the ESCRT machinery also participates in membrane abscission during cytokinesis and the formation of retroviral particles that bud from the cell surface during infection.Citation8-Citation11 Furthermore, specific components of the ESCRT machinery function to select cargo molecules for deposition into ILVs, thereby coupling protein sorting with vesicle formation at endosomes. In this review, we focus on recent advances that highlight how the ESCRT machinery assembles on the endosomal membrane and discuss mechanisms by which it may function to spatially and temporally regulate the biogenesis of ILVs that specifically sequester ubiquitinylated cargos. For a more in depth examination of the individual ESCRT complexes, we refer the reader to more comprehensive reviews.Citation12-Citation16
Membrane Targeting and Assembly of the ESCRT Machinery
The ESCRT machinery is composed of five multi-subunit complexes (ESCRT-0, ESCRT-I, ESCRT-II, ESCRT-III, and the Vps4-Vta1 complex) that each exhibits unique membrane binding properties. ESCRT-0 is composed of two subunits (Hrs and STAM1/2) and associates constitutively with endosomes through a combination of electrostatic, hydrophobic and lipid-specific interactions. In particular, the Hrs FYVE domain binds to the headgroup of endosomally enriched phosphatidylinositol 3-phosphate (PI3P), while simultaneously penetrating the lipid bilayer to achieve a high affinity membrane association (~10−8 M).Citation17,Citation18 Crystal structures of two FYVE domains derived from yeast and metazoan Hrs isoforms have been solved. Although their overall architectures are similar, the metazoan FYVE domain forms a dimer while the yeast homolog is monomeric.Citation19,Citation20 These data initially raised the possibility that metazoan ESCRT-0 harbors multiple copies of Hrs. Consistent with this idea, Hrs purified in the absence of STAM assembles as a cylindrical hexamer when overexpressed in insect cells.Citation21 Based on a three-dimensional cryo-electron microscopy structure, the central core of hexameric Hrs consists of six anti-parallel coiled-coil domains. In contrast, hydrodynamic studies examining native human Hrs have failed to identify such a oligomer. In vivo, Hrs appears to be constitutively bound to STAM, and loss of STAM dramatically impairs the stability of Hrs.Citation22,Citation23 By co-purifying recombinant forms of both proteins, multiple groups have now reconstituted intact ESCRT-0 in vitro, demonstrating that Hrs and STAM co-exist as a 1:1 heterodimer in solution.Citation22,Citation23 However, in the presence of physiological or synthetic membranes, ESCRT-0 self-associates to form 2:2 heterotetramers.Citation23 Although it remains unclear whether dimerization of the Hrs FYVE domain may mediate formation of the heterotetramer, the association between Hrs and STAM has been demonstrated to involve two domain-swapped GAT domains as well as the coiled-coil motifs in each protein.Citation22 Thus, in the presence of STAM, the formation of Hrs hexamers is highly improbable. Instead, ESCRT-0 likely assembles as heterotetramers on endosomal membranes in vivo.
ESCRT-I is composed of four subunits (Tsg101, Vps28, Vps37A/B/C/D, and Mvb12A/B/UBAP1), which associate as a 1:1:1:1 heterotetramer in solution that interacts weakly with acidic phospholipids in vitro.Citation24-Citation27 Consistent with its poor affinity for membranes, the majority of ESCRT-I is distributed throughout the cytoplasm and is only recruited to lipid bilayers through additional protein-protein interactions.Citation28 For example, at the endosome, ESCRT-0 (via PTAP-like motifs in Hrs) binds to ESCRT-I (via the Tsg101 UEV domain), to promote the formation of intralumenal vesicles. Similarly, an interaction between Tsg101 and Cep55 is necessary to place ESCRT-I at the intracellular bridge during late stages of cytokinesis.Citation9,Citation29,Citation30 Notably, as homologs of Hrs and Cep55 are absent in many species that exhibit ESCRT-I function during multivesicular endosome (MVE) biogenesis and abscission, additional regulators of ESCRT-I membrane association likely exist.Citation31
ESCRT-II is also a heterotetramer and is composed of three subunits (Vps22, Vps25, and Vps36) in a 1:2:1 configuration.Citation32,Citation33 Unlike ESCRT-I, the ESCRT-II complex binds well to acidic phospholipids including PI3P, which are abundant in endosomal membranes.Citation33-Citation36 Nonetheless, endogenous ESCRT-II is found mostly in the cytoplasm and only undergoes transient recruitment to membranes.Citation37-Citation39 Studies using the yeast isoforms of ESCRT-I and ESCRT-II indicate they interact directly in vitro (through an association between the Vps28 carboxyl-terminus and the Vps36 NZF motif) with nanomolar affinity (10−8 M), which may play a key role in ESCRT-II recruitment to endosomes in cells.Citation36,Citation40,Citation41 However, an interaction between native ESCRT-I and ESCRT-II is not detectable in cytosolic yeast extracts, indicating that the association is under tight regulation in vivo. In higher eukaryotes, the NZF motif within yeast Vps36 is absent. Instead, a region immediately downstream of the human Vps36 GLUE domain has been shown to be important, though not sufficient, for ESCRT-I binding in vitro.Citation33 Thus, multiple new binding surfaces between metazoan ESCRT-I and ESCRT-II have likely evolved, but await rigorous characterization.
In vitro, a mixture of recombinant yeast ESCRT-I and ESCRT-II is sufficient to bind and deform giant unilamellar vesicles (GUVs) to create stable, inward budded structures.Citation42 These data suggest that ESCRT-I and ESCRT-II are key components of the ESCRT machinery that direct membrane bending. Consistent with this finding, mutations in Drosophila ESCRT-I or ESCRT-II essentially block ILV formation, while mutants lacking Hrs or ESCRT-III subunits remain capable of MVE biogenesis, though the process is significantly curtailed.Citation43,Citation44 Three biologically relevant mechanisms by which the ESCRT complexes may induce membrane curvature have been postulated.Citation45-Citation47 First, ESCRT-I and ESCRT-II may generate a scaffold with intrinsic curvature that forces the lipid bilayer to bend. In this case, the energy of the protein-membrane association must exceed the membrane bending energy. Although co-sedimentation assays indicate that yeast ESCRT-II can recruit ESCRT-I onto liposomes in vitro, the affinity of ESCRT-II for membranes is not significantly increased by ESCRT-I, arguing against a model in which these ESCRT complexes co-assemble to form a scaffold that alters membrane curvature.Citation36 However, a structure of ESCRT-I bound to ESCRT-II will be necessary to ultimately resolve whether they can adopt a configuration capable of membrane bending.
A second possibility is that components of the ESCRT machinery alter the lipid composition within subdomains of the endosomal membrane to promote bending. With the exception of the Vps4-Vta1 complex, all ESCRT complexes directly associate with acidic phospholipids such as phosphatidylserine (PS). Since PS exhibits a positive spontaneous curvature, its aggregation in a single monolayer would generate a local asymmetry in the membrane and potentially reduce the energy requirement for invagination.Citation48 Although it is improbable that lipid demixing alone would be sufficient to induce significant membrane curvature, it may play an important role in concert with other mechanisms to enable ESCRT-mediated vesicle biogenesis.
Third, amphipathic regions of ESCRT-I and/or ESCRT-II may be exposed upon their co-assembly on membranes, resulting in membrane penetration. Insertion of an amphiphatic helix into the bilayer would perturb lipid packing and cause membrane deformation. However, regions of ESCRT-I and ESCRT-II capable of membrane insertion have yet to be defined. Alternatively, the interpretation of membrane deformations observed following ESCRT-I and ESCRT-II incubation with GUVs might require revision. High-speed confocal imaging of GUVs indicates that they undergo spontaneous and highly dynamic membrane bending events, most of which are unstable and relax shortly after formation.Citation49-Citation51 The addition of membrane binding proteins, such as a complex of ESCRT-I and ESCRT-II, may stabilize some of these deformations to create structures resembling budded vesicles. This interpretation would help to explain how high concentrations of ESCRT-III components alone are sufficient to promote vesicle formation and budding from GUVs.Citation49 It will be important to develop methods to distinguish this possibility from a direct role of ESCRT-I and ESCRT-II in membrane bending.
Distinct from the other ESCRT complexes, subunits of ESCRT-III are believed to exist in an autoinhibited state in solution and only assemble into an oligomeric complex when recruited onto membranes and activated by other ESCRT components.Citation52,Citation53 The major function of ESCRT-III is to act as the scission machinery necessary for vesicle release into the endosome lumen and daughter cell separation following cytokinesis.Citation11,Citation16,Citation49,Citation54 During MVE biogenesis, ESCRT-II initially binds to the ESCRT-III subunit Vps20, creating a curvature sensitive complex that exhibits intrinsic curvature. Importantly, the membrane binding energy of the complex was shown to vary with membrane curvature, indicating that ESCRT-II/Vps20 can associate more tightly with a lipid bilayer as it becomes increasingly bent.Citation51 These findings support a model in which Vps20 promotes the localization of ESCRT-II specifically to regions of elevated membrane curvature such as the neck of a budding vesicle. Consistent with this idea, the addition of Vps20 to GUVs coated with ESCRT-I and ESCRT-II dramatically enhances the localization of the ESCRT complexes to membrane bud necks.Citation42 By sensing membrane curvature, the ESCRT-II:Vps20 complex restricts ESCRT-III polymerization to highly curved membranes, thereby spatially regulating ESCRT-III scission activity.
Recombinant Vps20 binds membranes weakly, likely via electrostatic interactions with acidic phospholipids. In vivo, Vps20 is myristoylated, which enhances its ability to associate with endosomal membranes. Nonetheless, based on cell fractionation studies using yeast extracts, the majority of native Vps20 is cytosolic, similar to other ESCRT-III subunits.Citation52 In contrast, polymerized ESCRT-III filaments bind tightly to membranes and require energy in the form of ATP hydrolysis for dissociation and disassembly.Citation14,Citation51,Citation52,Citation55,Citation56 Although the precise composition of native ESCRT-III filaments remains unknown and may be highly heterogeneous, several ESCRT-III subunits (Vps32, Vps24, and Vps2) exhibit the ability to homo- or hetero-polymerize.Citation51,Citation56-Citation58 However, the variety of analyzed filaments all show significant differences in their sizes and characteristics. For example, human Vps32 has been shown to assemble into ~5 nm filaments that self-associate to form circular arrays and are capable of deforming the plasma membrane in cultured cells.Citation57 In contrast, a mixture of human Vps24 and Vps2 isoforms, lacking their inhibitory carboxyl-termini, form helical tubules in vitro with a diameter of ~40 nm.Citation56 Importantly, in neither case is filament formation initiated by other components of the ESCRT machinery, raising concerns regarding their physiological relevance. Recently, filaments of recombinant Vps32 nucleated by ESCRT-II and Vps20 were imaged by atomic force microscopy on supported lipid bilayers. These polymers exhibited an average diameter of 13.4 nm, similar to endogenous ESCRT-III filaments visualized within the intercellular bridge of dividing human cells (~17 nm) by electron tomography.Citation11,Citation51 Moreover, the Vps32 filaments bound specifically to highly curved membranes and promoted membrane remodeling in a manner akin to membrane fission. Consistent with these findings, release of membrane buds on GUVs exhibited a strong dependence on Vps32, and yeast Vps32 has been shown to be the most abundant ESCRT-III component that functions during MVE biogenesis.Citation42,Citation59 These data collectively argue that Vps32 polymerization, triggered by ESCRT-II and Vps20 at MVEs, plays a significant role in the scission of ILVs. Based on depletion studies showing that ESCRT-II is not required for cytokinesis or retroviral budding, alternative mechanisms to promote Vps32 filament assembly must exist and may involve the ESCRT-related protein Alix, which can bind directly to Vps32.Citation3,Citation38,Citation60
ESCRT-III disassembly and membrane dissociation require the input of free energy in the form of ATP hydrolysis, a process mediated by the Vps4 ATPase and its cofactor Vta1, which co-assemble and bind to ESCRT-III filaments present on membranes.Citation56,Citation58,Citation61-Citation63 Although the precise mechanism by which Vps4 functions in ESCRT-III disassembly remains unknown, studies using yeast proteins indicate that Vps4 oligomerizes to form two stacked hexameric rings.Citation64 Current models propose that ESCRT-III subunits may be inserted through the central pore of the rings in an ATP-dependent fashion, resulting in their unfolding and release from the membrane.Citation61 The presence of Vta1 stabilizes the Vps4 dodecamer and can stimulate its ATPase activity.Citation65,Citation66 Additionally, ESCRT-III subunits have also been shown to modulate Vps4-mediated ATP hydrolysis in vitro.Citation66,Citation67 Although the yeast Vps4-Vta1 complex has been extensively characterized, relatively little is known about its metazoan counterparts, mainly due to difficulties in their isolation and the absence of facile functional assays.
Cargo Sorting and Deposition into Intralumenal Vesicles
Modification of integral membrane proteins by ubiquitin is a key step in their sorting and transport to the lysosome lumen for degradation. Ubiquitin conjugation can occur at the cell surface, which acts as a signal for endocytosis.Citation6,Citation68,Citation69 A number of distinct endocytic adaptor proteins, including Eps15 and epsin, harbor ubiquitin interacting motifs (UIMs) that may engage cargo at this early stage of internalization.Citation70-Citation72 At the early endosome, ESCRT-0 functions in cargo recognition, as both of its subunits exhibit ubiquitin-binding properties.Citation73 ESCRT-0 also recruits flat clathrin lattices onto endosomes, generating discrete subdomains, which ultimately recruit other components of the ESCRT machinery that promote membrane curvature and scission.Citation7,Citation74,Citation75 These data suggest that ESCRT-0 marks the region of the endosomal membrane that will be internalized. Consistent with this idea, ESCRT-0 was shown to generate large domains (> 1 μm) on GUVs with a surface area similar to that of internalized vesicles that formed following the addition of other ESCRT complexes.Citation42 Although the dimensions of the ESCRT-0 footprint were far from physiological in the GUV-based assay, it is worth examining a model in which the ESCRT complexes define the size of lumenal vesicles in vivo.
Based on hydrodynamic studies and analysis by atomic force microscopy, metazoan ESCRT-0 assembles as a heterotetramer on membranes with a diameter of ~29 nm.Citation23 ESCRT-I binds to ESCRT-0 through an association between PXXP motifs in Hrs and the Tsg101 UEV domain.Citation29,Citation30 Although the stoichiometry of this interaction remains unknown, the presence of two Hrs molecules in ESCRT-0 predicts the recruitment of at least two ESCRT-I complexes. The structure of metazoan ESCRT-I has not been solved, but the related yeast complex exhibits an elongated shape similar to ESCRT-0, with a length of ~25 nm in solution.Citation26 Potential changes in ESCRT-I conformation following its recruitment onto membranes by ESCRT-0 await characterization. Each ESCRT-I complex recruits an ESCRT-II heterotetramer, which exhibits a compact structure in solution, but may undergo remodeling upon membrane binding. Using atomic force microscopy, an individual metazoan ESCRT-II complex was found to possess an average diameter of ~26 nm.Citation51 Thus, each early-acting ESCRT complex exhibits a similar overall size and would generate a combined footprint between 80–130 nm in diameter, depending on their arrangement on the membrane, corresponding to a surface area of ~104 nm2. A spherical vesicle derived from such a surface would exhibit a diameter of 40–65 nm, which corresponds well to the size range of metazoan lumenal vesicles within endosomes.Citation76 Efficient scission of the membrane is also likely to play an important role in determining ILV size, as defects in ESCRT-III function have been linked to the formation of vesicles with abnormal morphologies.Citation77,Citation78
Although the ESCRT complexes likely define the membrane area that will be internalized, they are typically not consumed during the reaction. How ESCRT-0 is released from the invaginating membrane is unclear. One possibility is that the interaction with ESCRT-I causes a conformational change in ESCRT-0, resulting in its redistribution away from the site. Consistent with this idea, ESCRT-0 and ESCRT-I only transiently co-localize on endosomal membranes in vivo.Citation30 Importantly, the weak affinity between ESCRT-0 and ubiquitin allows for ESCRT-0 to be released from the membrane without affecting the distribution of its sequestered transmembrane cargoes. Moreover, lateral diffusion of cargoes would be restricted by ESCRT-I and incoming ESCRT-II, which both harbor ubiquitin-binding domains that are important for cargo sorting.Citation27,Citation79-Citation82 Importantly, this model does not depend on the directed transfer of cargoes between ESCRT complexes, which does not appear to be thermodynamically favorable. Instead, ESCRT-0 functions as the critical cargo recognition and clustering complex, while ESCRT-I and ESCRT-II block cargo dispersion until ESCRT-III is assembled. Ultimately, the formation of an ESCRT-III polymer further restricts cargo within the forming ILV ().
Figure 1. A model highlighting the multiple functions of the ESCRT machinery during cargo sorting and vesicle biogenesis. Step 1: The membrane bound ESCRT-0 complex captures ubiquitin-modified transmembrane cargoes and subsequently recruits ESCRT-I onto the endosomal membrane. A combination of protein-lipid and protein-protein interactions leads to ESCRT-II accumulation on the membrane, establishing an ESCRT footprint that is ~80–130 nm in diameter. Step 2: The ESCRT-0 complex is released from the membrane, potentially due to conformational changes following ESCRT-I binding, and both ESCRT-I and ESCRT-II prevent the lateral diffusion of cargoes. Additionally, ESCRT-I and ESCRT-II may initiate membrane bending. Step 3: ESCRT-II nucleates filaments of ESCRT-III that associate tightly with membrane. In particular, the association of ESCRT-II with Vps20 generates a curvature-sensitive complex that may further bend the membrane to generate a highly curved vesicle bud neck. Polymerized ESCRT-III filaments ultimately drive the vesicle scission process through additional membrane remodeling events, which may include lipid demixing.
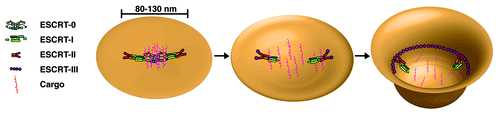
Mechanisms underlying the release of ESCRT-I and ESCRT-II from the endosomal membrane have yet to be determined. Unlike ESCRT-0, the other ESCRT complexes co-localize with late endosomal markers, suggesting they function together during the final stages of lumenal vesicle biogenesis.Citation30 However, Vps4 appears only to regulate the disassembly of ESCRT-III.Citation78 Recent work has shown that the affinity of ESCRT-II for flat lipid bilayers decreases upon binding to Vps20.Citation51 Therefore, when the membrane flattens out following vesicle scission, the ESCRT-II:Vps20 complex may be released. In the absence of other ESCRT machinery, the weak affinity of ESCRT-I for membranes would be insufficient to maintain its localization, resulting in its loss from the endosome surface ().
Conclusions and Perspectives
Although initially recognized for its roles in endosomal protein sorting, the ESCRT machinery has proven to exhibit diverse functions in several topologically similar membrane remodeling events.Citation83 In most cases, ESCRT-III orthologs play a critical role in membrane fission or fusion, while other components of the ESCRT machinery act as regulatory molecules that target ESCRT-III activity to different cellular locations. For example, during the formation of lumenal vesicles within endosomes, ESCRT-0 initially directs the assembly of other ESCRT components.Citation84 In a similar fashion, Cep55 targets the ESCRT machinery to the intracellular bridge during the final stages of cell division.Citation9 However, unlike Cep55, ESCRT-0 also harbors a number of ubiquitin binding sites, which can engage ubiquitin-modified cargoes in the endocytic pathway and sequester them for deposition into lumenal vesicles.Citation73 Interestingly, the components of ESCRT-0 are found in relatively few eukaryotic species, suggesting that other ubiquitin-binding proteins participate in ESCRT-mediated cargo sorting. Consistent with this idea, orthologs of the ubiquitin-interacting protein Tom1 have been shown to interact with clathrin and Tsg101 and may participate in lysosomal protein transport in several systems.Citation85-Citation87 However, a major difference between Tom1 and ESCRT-0 is the number of ubiquitin binding domains present. When assembled on the endosomal membrane, ESCRT-0 can associate with at least eight individual ubiquitin molecules simultaneously, far more than Tom1 (which has one), even when it forms a complex with other ubiquitin-binding proteins such as Tollip.Citation23,Citation87,Citation88 Thus, unlike Tom1, ESCRT-0 exhibits the unique ability to cluster cargoes, a function that may have arisen in fungi and metazoans to enable the rapid downregulation of ubiquitin-modified cargoes. Additional forms of specialization have evolved in other components of the ESCRT machinery.Citation31 How these changes impact ESCRT structure and function will continue to be a focus of intense study.
Likely the most ancient function of the ESCRT machinery lies in its ability to promote membrane scission.Citation89 The mechanisms underlying this process are far from clear, but several studies highlight the importance of ESCRT-III polymerization in promoting homotypic membrane fusion.Citation11,Citation51,Citation56,Citation58 Other work further suggests that ESCRT-III may also regulate the membrane bending process.Citation57,Citation77 A striking example of this was observed following the overexpression of Vps32 in human cells. Initially, polymers of the ESCRT-III subunit assembled into self-associating circular arrays on the plasma membrane, which could generate curvature following co-expression of dominant negative Vps4.Citation57 It remains unclear whether similar flat spirals of ESCRT-III actually form under normal conditions. For example, electron tomographic reconstructions of endogenous ESCRT-III polymers suggest they do not self-associate along their entire length.Citation11 Additionally, ESCRT-III filaments that form following Vps32 overexpression exhibit a diameter dramatically smaller than that observed during cytokinesis in control cells, raising further concerns regarding the physiological relevance of the flat spirals. Instead, ESCRT-I and ESCRT-II, potentially in concert with Vps20, are more likely candidates for generating membrane curvature prior to ESCRT-III mediated scission, although mechanistic details for this process remain to be solved.
Unlike other budding events such as dynamin-mediated endocytosis from the plasma membrane and COPII vesicle biogenesis at endoplasmic reticulum exit sites, nucleotide hydrolysis is not absolutely necessary for ESCRT-III dependent scission.Citation42,Citation90 Instead, Vps4-mediated ATP turnover likely plays a critical role in the removal and recycling of ESCRT-III subunits from the membrane, subsequent to their function. However, one model postulates that Vps4 activity may actually promote scission through its role in ESCRT-III disassembly. In this model, circular filaments of ESCRT-III steadily depolymerize and decrease in radius, exerting force on the underlying membrane to promote fusion.Citation77 The generation of force would require one end of the ESCRT-III filament to be anchored to the constricting lipid bilayer, while the other is free to interact with Vps4 and depolymerize. Supporting evidence for such a disassembly pathway is lacking, and it remains unclear whether Vps4 could selectively associate with a specific region of the ESCRT-III complex, as multiple subunits harbor Vps4 interacting motifs. Moreover, ESCRT-mediated vesicle budding does not require Vps4 activity in vitro.
Several other models exist to describe how a nucleotide-independent membrane scission event may proceed. One is based on the in vitro analysis of helical filaments composed of Vps24 and Vps2, which terminate in a dome-like structure.Citation56,Citation91 Interactions between the surface of the dome and membrane could draw opposing lipid bilayers together to promote scission. This model relies on the formation of ESCRT-III filaments that self-associate, similar to a circular array of Vps32, but significantly more compacted. Interestingly, recent evidence suggests that human Vps2 can form helical tubes capable of deforming the plasma membrane.Citation92 However, their assembly requires the overexpression of Vps2, which may promote the formation of structures with limited physiological relevance. An alternative model that we favor posits that individual ESCRT-III filaments are sufficient to promote membrane scission. This idea is supported by work showing that ESCRT-III filaments nucleated by ESCRT-II do not self-associate, but instead bind specifically to highly curved regions of lipid bilayers.Citation51 The data suggest that ESCRT-III assembly in vivo is restricted to membranes of elevated curvature such as those present at the neck of a budding vesicle. If the binding energy of ESCRT-III for the membrane is sufficient to exceed the energy barrier to scission, membrane fusion and vesicle release would likely occur. Strikingly, the association of individual ESCRT-III filaments with the edges of supported lipid bilayers was sufficient to promote their mobility, potentially in a manner akin to scission. Ultimately, electron microscopy-based approaches will need to be employed to measure the thickness of ESCRT-III filaments that assemble during lumenal vesicle biogenesis in vivo. These data will likely resolve whether self-interacting helices or individual filaments of ESCRT-III form to promote membrane scission.
Abbreviations: | ||
BAR | = | Bin-Amphiphysin-Rvs |
EHD | = | Eps15 homology domain |
ESCRT | = | endosomal sorting complex required for transport |
FYVE | = | Fab1-YOTB-Vac1-EEA1 |
GAT | = | GGA and Tom1 |
GLUE | = | Gram-like ubiquitin-binding |
GUV | = | giant unilamellar vesicle |
ILV | = | intralumenal vesicle |
MVE | = | multivesicular endosome |
NZF | = | Npl4 zinc finger |
PI3P | = | phosphatidylinositol 3-phosphate |
PS | = | phosphatidylserine |
UEV | = | ubiquitin E2 variant |
UIM | = | ubiquitin interacting motif |
Acknowledgments
We thank members of the Audhya lab for critically reading the manuscript. This work was supported by a grant from the NIH (1R01GM088151–01A1) to A.A.
REFERENCES
- Peter BJ, Kent HM, Mills IG, Vallis Y, Butler PJ, Evans PR, et al. BAR domains as sensors of membrane curvature: the amphiphysin BAR structure. Science 2004; 303:495 - 9; http://dx.doi.org/10.1126/science.1092586; PMID: 14645856
- Daumke O, Lundmark R, Vallis Y, Martens S, Butler PJ, McMahon HT. Architectural and mechanistic insights into an EHD ATPase involved in membrane remodeling. Nature 2007; 449:923 - 7; http://dx.doi.org/10.1038/nature06173; PMID: 17914359
- Grant BD, Caplan S. Mechanisms of EHD/RME-1 protein function in endocytic transport. Traffic 2008; 9:2043 - 52; http://dx.doi.org/10.1111/j.1600-0854.2008.00834.x; PMID: 18801062
- Pant S, Sharma M, Patel K, Caplan S, Carr CM, Grant BD. A novel requirement for C. elegans Alix/ALX-1 in RME-1 mediated membrane transport. Nat Cell Biol 2009; 11:1399 - 410; http://dx.doi.org/10.1038/ncb1986
- McMahon HT, Gallop JL. Membrane curvature and mechanisms of dynamic cell membrane remodeling. Nature 2005; 438:590 - 6; http://dx.doi.org/10.1038/nature04396; PMID: 16319878
- Piper RC, Katzmann DJ. Biogenesis and function of multivesicular bodies. Annu Rev Cell Dev Biol 2007; 23:519 - 47; http://dx.doi.org/10.1146/annurev.cellbio.23.090506.123319; PMID: 17506697
- Henne WM, Buchkovich NJ, Emr SD. The ESCRT pathway. Dev Cell 2011; 21:77 - 91; http://dx.doi.org/10.1016/j.devcel.2011.05.015; PMID: 21763610
- Garrus JE, von Schwedler UK, Pornillos OW, Morham SG, Zavitz KH, Wang HE, et al. Tsg101 and the vacuolar protein sorting pathway are essential for HIV-1 budding. Cell 2001; 107:55 - 65; http://dx.doi.org/10.1016/S0092-8674(01)00506-2; PMID: 11595185
- Carlton JG, Martin-Serrano J. Parallels between cytokinesis and retroviral budding: a role for the ESCRT machinery. Science 2007; 316:1908 - 12; http://dx.doi.org/10.1126/science.1143422; PMID: 17556548
- Martin-Serrano J, Neil SJ. Host factors involved in retroviral budding and release. Nat Rev Microbiol 2011; 9:519 - 31; http://dx.doi.org/10.1038/nrmicro2596; PMID: 21677686
- Guizetti J, Schermelleh L, Mantler J, Maar S, Poser I, Leohardt H, et al. Cortical constriction during abscission involves helices of ESCRT-III-dependent filaments. Science 2011; 331:1616 - 20; http://dx.doi.org/10.1126/science.1201847; PMID: 21310966
- Hurley JH, Emr SD. The ESCRT complexes: structure and mechanism of a membrane-trafficking network. Annu Rev Biophys Biomol Struct 2006; 35:277 - 98; http://dx.doi.org/10.1146/annurev.biophys.35.040405.102126; PMID: 16689637
- Williams RL, Urbe S. The emerging shape of the ESCRT machinery. Nat Rev Mol Cell Biol 2007; 8:355 - 68; http://dx.doi.org/10.1038/nrm2162; PMID: 17450176
- Raiborg C, Stenmark H. The ESCRT machinery in endosomal sorting of ubiquitylated membrane proteins. Nature 2009; 458:445 - 52; http://dx.doi.org/10.1038/nature07961; PMID: 19325624
- Hurley JH, Hanson PI. Membrane budding and scission by the ESCRT machinery: it’s all in the neck. Nat Rev Mol Cell Biol 2010; 11:556 - 66; http://dx.doi.org/10.1038/nrm2937; PMID: 20588296
- Hurley JH. The ESCRT complexes. Crit Rev Biochem Mol Biol 2010; 45:463 - 87; http://dx.doi.org/10.3109/10409238.2010.502516; PMID: 20653365
- Raiborg C, Bremnes B, Mehlum A, Gillooly DJ, D’Arrigo A, Stang E, et al. Hrs recruits clathrin to early endosomes. J Cell Sci 2001; 114:2255 - 63; PMID: 11493665
- Stahelin RV, Long F, Diaviyam K, Bruzik KS, Murray D, Cho W. Phosphatidylinositol 3-phosphate induces the membrane penetration of the FYVE domains of Vps27p and Hrs. J Biol Chem 2002; 277:26379 - 88; http://dx.doi.org/10.1074/jbc.M201106200; PMID: 12006563
- Misra S, Hurley JH. Crystal structure of a phosphatidylinositol 3-phosphate-specific membrane-targeting motif, the FYVE domain of Vps27p. Cell 1999; 97:657 - 66; http://dx.doi.org/10.1016/S0092-8674(00)80776-X; PMID: 10367894
- Mao Y, Nickitenko A, Duan X, Lloyd TE, Wu MN, Bellen H, et al. Crystal structure of the VHS and FYVE tandem domains of Hrs, a protein involved in membrane trafficking and signal. Cell 2000; 100:447 - 56; http://dx.doi.org/10.1016/S0092-8674(00)80680-7; PMID: 10693761
- Pullan L, Mullapudi S, Huang Z, Baldwin PR, Chic C, Sun W, et al. The endosome-associated protein Hrs is hexameric and controls cargo sorting as a “master molecule”. Structure 2006; 14:661 - 71; http://dx.doi.org/10.1016/j.str.2006.01.012; PMID: 16615908
- Ren X, Kloer DP, Kim YC, Ghirlando R, Saidi LF, Hummer G, et al. Hybrid structural model of the complete human ESCRT-0 complex. Structure 2009; 17:406 - 16; http://dx.doi.org/10.1016/j.str.2009.01.012; PMID: 19278655
- Mayers JR, Fyfe I, Schuh AL, Chapman ER, Edwardson JM, Audhya A. ESCRT-0 assembles as a heterotetrameric complex on membranes and binds multiple ubiquitinylated cargos simultaneously. J Biol Chem 2011; 286:9636 - 45; http://dx.doi.org/10.1074/jbc.M110.185363
- Morita E, Sandrin V, Alam SL, Eckert DM, Gygi SP, Sundquist WI. Identification of human MVB12 proteins as ESCRT-I subunits that function in HIV budding. Cell Host Microbe 2007; 2:41 - 53; http://dx.doi.org/10.1016/j.chom.2007.06.003; PMID: 18005716
- Audhya A, McLeod IX, Yates JR, Oegema K. MVB-12, a fourth subunit of metazoan ESCRT-I, functions in receptor downregulation. PLoS ONE 2007; 2:e956; http://dx.doi.org/10.1371/journal.pone.0000956; PMID: 17895996
- Kostelansky MS, Schluter C, Tam YY, Lee S, Ghirlando R, Conibear E, et al. Molecular architecture and functional model of the complete yeast ESCRT-I heterotetramer. Cell 2007; 129:485 - 98; http://dx.doi.org/10.1016/j.cell.2007.03.016; PMID: 17442384
- Stefani F, Zhang L, Taylor S, Donovan J, Rollinson S, Doyotte A, et al. UBAP1 is a component of an endosome-specific ESCRT-I complex that is essential for MVB sorting. Curr Biol 2011; 21:1245 - 50; http://dx.doi.org/10.1016/j.cub.2011.06.028; PMID: 21757351
- Xie W, Li L, Cohen SN. Cell cycle-dependent subcellular localization of the TSG101 protein and mitotic and nuclear abnormalities associated with TSG-101 deficiency. Proc Natl Acad Sci USA 1998; 95:1595 - 600; http://dx.doi.org/10.1073/pnas.95.4.1595; PMID: 9465061
- Katzmann DJ, Stefan CJ, Babst M, Emr SD. Vps27 recruits ESCRT machinery to endosomes during MVB sorting. J Cell Biol 2003; 162:413 - 23; http://dx.doi.org/10.1083/jcb.200302136; PMID: 12900393
- Bache KG, Brech A, Mehlum A, Stenmark H. Hrs regulates multivesicular body formation via ESCRT recruitment to endosomes. J Cell Biol 2003; 162:435 - 42; http://dx.doi.org/10.1083/jcb.200302131; PMID: 12900395
- Leung KF, Dacks JB, Field MC. Evolution of the multivesicular body ESCRT machinery; retention across the eukaryotic lineage. Traffic 2008; 9:1698 - 716; http://dx.doi.org/10.1111/j.1600-0854.2008.00797.x; PMID: 18637903
- Hierro A, Sun J, Runsnak AS, Kim J, Prag G, Emr SD, et al. Structure of the ESCRT-II endosomal trafficking complex. Nature 2004; 431:221 - 5; http://dx.doi.org/10.1038/nature02914; PMID: 15329733
- Im YJ, Hurley JH. Integrated structural model and membrane targeting mechanism of the human ESCRT-II complex. Dev Cell 2008; 14:902 - 13; http://dx.doi.org/10.1016/j.devcel.2008.04.004; PMID: 18539118
- Teo H, Perisic O, Gonzalez B, Williams RL. ESCRT-II, an endosome-associated complex required for protein sorting: crystal structure and interactions with ESCRT-III and membranes. Dev Cell 2004; 7:559 - 69; http://dx.doi.org/10.1016/j.devcel.2004.09.003; PMID: 15469844
- Slagsvold T, Aasland R, Hirano S, Bache KG, Raiborg C, Trambaiolo D, et al. Eap45 in mammalian ESCRT-II binds ubiquitin via a phosphoinositide-interacting GLUE domain. J Biol Chem 2005; 280:19600 - 6; http://dx.doi.org/10.1074/jbc.M501510200; PMID: 15755741
- Teo H, Perisic O, Gonzalez B, Williams RL. ESCRT-II, an endosome-associated complex required for protein sorting: crystal structure and interactions with ESCRT-III and membranes. Dev Cell 2004; 7:559 - 69; http://dx.doi.org/10.1016/j.devcel.2004.09.003; PMID: 15469844
- Babst M, Katzmann DJ, Snyder WB, Wendland B, Emr SD. Endosome-associated complex, ESCRT-II, recruits transport machinery for protein sorting at the multivesicular body. Dev Cell 2002; 3:283 - 9; http://dx.doi.org/10.1016/S1534-5807(02)00219-8; PMID: 12194858
- Langelier C, von Schwedler UK, Fisher RD, De Domenico I, White PL, Hill CP, et al. Human ESCRT-II complex and its role in human immunodeficiency virus type 1 release. J Virol 2006; 80:9465 - 80; http://dx.doi.org/10.1128/JVI.01049-06; PMID: 16973552
- Irion U, St. Johnston D. Bicoid RNA localization requires specific binding of an endosomal sorting complex. Nature 2007; 445:554 - 8; http://dx.doi.org/10.1038/nature05503; PMID: 17268469
- Kostelansky MS, Sun J, Lee S, Kim J, Ghirlando R, Hierro A, et al. Structural and functional organization of the ESCRT-I trafficking complex. Cell 2006; 125:113 - 26; http://dx.doi.org/10.1016/j.cell.2006.01.049; PMID: 16615894
- Gill DJ, Teo H, Sun J, Perisic O, Veprintsev DB, Emr SD, et al. Structural insight into the ESCRT-I/-II link and its role in MVB trafficking. EMBO J 2007; 26:600 - 12; http://dx.doi.org/10.1038/sj.emboj.7601501; PMID: 17215868
- Wollert T, Hurley JH. Molecular mechanism of multivesicular body biogenesis by ESCRT complexes. Nature 2010; 464:864 - 9; http://dx.doi.org/10.1038/nature08849; PMID: 20305637
- Lloyd TE, Atkinson R, Wu MN, Zhou Y, Penetta G, Bellen HJ. Hrs regulates endosome membrane ivagination and tyrosine kinase receptor signalling in Drosophila. Cell 2002; 108:261 - 9; http://dx.doi.org/10.1016/S0092-8674(02)00611-6; PMID: 11832215
- Vaccari T, Rusten TE, Menut L, Nezis IP, Brech A, Stenmark H, et al. Comparative analysis of ESCRT-I, ESCRT-II and ESCRT-III function in Drosophila by efficient isolation of ESCRT mutants. J Cell Sci 2009; 122:2413 - 23; http://dx.doi.org/10.1242/jcs.046391; PMID: 19571114
- Rand RP, Fuller NL, Gruner SM, Parsegian VA. Membrane curvature, lipid segragation, and structural transitions for phospholipids under dual-solvent stress. Biochemistry 1990; 29:76 - 87; http://dx.doi.org/10.1021/bi00453a010; PMID: 2322550
- McMahon HT, Gallop JL. Membrane curvature and mechanisms of dynamic cell membrane remodeling. Nature 2005; 438:590 - 6; http://dx.doi.org/10.1038/nature04396; PMID: 16319878
- Khelashvili G, Harries D, Weinstein H. Modeling membrane deformations and lipid demixing upon protein-membrane interaction: the BAR dimmer adsorption. Biophys J 2009; 97:1626 - 35; http://dx.doi.org/10.1016/j.bpj.2009.07.006; PMID: 19751667
- Fuller N, Benatti CR, Rand RP. Curvature and bending constants for phosphatidylserine-containing membranes. Biophys J 2003; 85:1667 - 74; http://dx.doi.org/10.1016/S0006-3495(03)74596-2; PMID: 12944281
- Wollert T, Wunder C, Lippincott-Schwartz J, Hurley JH. Membrane scission by the ESCRT-III complex. Nature 2009; 458:172 - 7; http://dx.doi.org/10.1038/nature07836; PMID: 19234443
- Rossman JS, Jing X, Leser GP, Lamb RA. Influenza virus M2 protein mediates ESCRT-independent membrane scission. Cell 2010; 142:902 - 13; http://dx.doi.org/10.1016/j.cell.2010.08.029; PMID: 20850012
- Fyfe I, Schuh AL, Edwardson JM, Audhya A. Association of ESCRT-II with VPS20 generates a curvature sensitive protein complex capable of nucleating filaments of ESCRT-III. J Biol Chem 2011; 286:34262 - 70; http://dx.doi.org/10.1074/jbc.M111.266411; PMID: 21835927
- Babst M, Katzmann DJ, Estepa-Sabal EJ, Meerloo T, Emr SD. Escrt-III: an endosome-associated heterooligomeric protein complex required for mvb sorting. Dev Cell 2002; 3:271 - 82; http://dx.doi.org/10.1016/S1534-5807(02)00220-4; PMID: 12194857
- Zamborlini A, Usami Y, Radoshitzky SR, Popova E, Palu G, Gottlinger H. Release of autoinhibition converts ESCRT-III components into potent inhibitors of HIV-1 budding. Proc Natl Acad Sci USA 2006; 103:19140 - 5; http://dx.doi.org/10.1073/pnas.0603788103; PMID: 17146056
- Roxrud I, Stenmark H, Malerod L. ESCRT & Co. Biol Cell 2010; 102:293 - 318; http://dx.doi.org/10.1042/BC20090161; PMID: 20222872
- Babst M, Wendland B, Estepa EJ, Emr SD. The Vps4p AAA ATPase regulates membrane association of a Vps protein complex required for normal endosome function. EMBO J 1998; 17:2982 - 3; http://dx.doi.org/10.1093/emboj/17.11.2982; PMID: 9606181
- Lata S, Schoehn G, Jain A, Pires R, Piehler J, Gottlinger HG, et al. Helical structures of ESCRT-III are disassembled by VPS4. Science 2008; 321:1354 - 7; http://dx.doi.org/10.1126/science.1161070; PMID: 18687924
- Hanson PI, Roth R, Lin Y, Heuser JE. Plasma membrane deformation by circular arrays of ESCRT-III protein filaments. J Cell Biol 2008; 180:389 - 402; http://dx.doi.org/10.1083/jcb.200707031; PMID: 18209100
- Ghazi-Tabatabai S, Saksena S, Short JM, Pobbati AV, Veprintsev DB, Crowther RA, et al. Structure and disassembly of filaments formed by the ESCRT-III subunit Vps24. Structure 2008; 16:1345 - 56; http://dx.doi.org/10.1016/j.str.2008.06.010; PMID: 18786397
- Teis D, Saksena S, Emr SD. Ordered assembly of the ESCRT-III complex on endosmes is required to sequester cargo during MVB formation. Dev Cell 2008; 15:578 - 89; http://dx.doi.org/10.1016/j.devcel.2008.08.013; PMID: 18854142
- Kim J, Sitaraman S, Hierro A, Beach BM, Odorizzi G, Hurley JH. Structural basis for endosomal targeting by the Bro1 domain. Dev Cell 2005; 8:937 - 47; http://dx.doi.org/10.1016/j.devcel.2005.04.001; PMID: 15935782
- Scott A, Chung HY, Gonciarz-Swiatek M, Hill CP, Whitby FG, Gaspar J, et al. Structural and mechanistic studies of VPS4 proteins. EMBO J 2005; 24:3658 - 69; http://dx.doi.org/10.1038/sj.emboj.7600818; PMID: 16193069
- Azmi I, Davies B, Dimaano C, Payne M, Eckert D, Babst M, et al. Recycling of ESCRTs by the AAA-ATPase Vps4 is regulated by a conserved VSL region in Vta1. J Cell Biol 2006; 172:705 - 17; http://dx.doi.org/10.1083/jcb.200508166; PMID: 16505166
- Shestakova A, Hanono A, Drosner S, Curtiss M, Davies BA, Katzmann DJ, et al. Assembly of the AAA ATPase Vps4 on ESCRT-III. Mol Biol Cell 2010; 21:1059 - 71; http://dx.doi.org/10.1091/mbc.E09-07-0572; PMID: 20110351
- Yu Z, Gonciarz MD, Sundquist WI, Hill CP, Jensen GJ. Cryo-EM structure of dodecameric Vps4p and its 2:1 complex with Vta1p. J Mol Biol 2008; 377:364 - 77; http://dx.doi.org/10.1016/j.jmb.2008.01.009; PMID: 18280501
- Yang D, Hurley JH. Structural role of the Vps4-Vta1 interface in ESCRT-III recycling. Structure 2010; 18:976 - 84; http://dx.doi.org/10.1016/j.str.2010.04.014; PMID: 20696398
- Azmi IF, Davies B, Xiao J, Babst M, Xu Z, Katzmann DJ. ESCRT-III family members stimulate Vps4 ATPase activity directly or via Vta1. Dev Cell 2008; 14:50 - 61; http://dx.doi.org/10.1016/j.devcel.2007.10.021; PMID: 18194652
- Merrill SA, Hanson PI. Activation of human VPS4A by ESCRT-III proteins reveals ability of substrates to relieve enzyme autoinhibition. J Biol Chem 2010; 285:35428 - 38; http://dx.doi.org/10.1074/jbc.M110.126318; PMID: 20805225
- Hicke L. Protein regulation by monoubiquitin. Nat Rev Mol Cell Biol 2001; 2:195 - 201; http://dx.doi.org/10.1038/35056583; PMID: 11265249
- Clague MJ, Urbe S. Endocytosis: the DUB version. Trends Cell Biol 2006; 16:551 - 9; http://dx.doi.org/10.1016/j.tcb.2006.09.002; PMID: 16996268
- Polo S, Sigismund S, Faretta M, Guidi M, Capua MR, Bossi G, et al. A single motif responsible for ubiquitin recognition and monoubiquitination in endocytic proteins. Nature 2002; 416:451 - 5; http://dx.doi.org/10.1038/416451a; PMID: 11919637
- Shih SC, Katzmann DJ, Schnell JD, Sutanto M, Emr SD, Hicke L. Epsins and Vps27p/Hrs contain ubiquitin-binding domains that function in receptor endocytosis. Nat Cell Biol 2002; 4:389 - 93; http://dx.doi.org/10.1038/ncb790; PMID: 11988742
- Madshus IH. Ubiquitin binding in endocytosis—how tight should it be and where does it happen?. Traffic 2006; 7:258 - 61; http://dx.doi.org/10.1111/j.1600-0854.2006.00393.x; PMID: 16497221
- Bilodeau PS, Urbanowski JL, Winistorfer SC, Piper RC. The Vps27p Hse1p complex binds uqiquitin and mediates endosomal protein sorting. Nat Cell Biol 2002; 4:534 - 9; PMID: 12055639
- Raiborg C, Bache KG, Mehlum A, Stang E, Stenmark H. Function of Hrs in endocytic trafficking and signaling. EMBO J 2001; 20:5008 - 21; http://dx.doi.org/10.1093/emboj/20.17.5008; PMID: 11532964
- Raiborg C, Bache KG, Gillooly DJ, Madshus IH, Stang E, Stenmark H. Hrs sorts ubiquitinated proteins into clathrin-coated microdomains of early endosomes. Nat Cell Biol 2002; 4:394 - 8; http://dx.doi.org/10.1038/ncb791; PMID: 11988743
- Bassereau P. Division of labour in ESCRT complexes. Nat Cell Biol 2010; 12:422 - 3; http://dx.doi.org/10.1038/ncb0510-422; PMID: 20442701
- Saksena S, Wahlman J, Teis D, Johnson AE, Emr SD. Functional reconstitution of ESCRT-III assembly and disassembly. Cell 2009; 136:97 - 109; http://dx.doi.org/10.1016/j.cell.2008.11.013; PMID: 19135892
- Nickerson DP, West M, Henry R, Odorizzi G. Regulators of Vps4 ATPase activity at endosomes differentially influence the size and rate of formation of intralumenal vesicles. Mol Biol Cell 2010; 21:1023 - 32; http://dx.doi.org/10.1091/mbc.E09-09-0776; PMID: 20089837
- Katzmann DJ, Babst M, Emr SD. Ubiquitin-dependent sorting into the multivesicular body pathway requires the function of a conserved endosomal protein sorting complex, ESCRT-I. Cell 2001; 106:145 - 55; http://dx.doi.org/10.1016/S0092-8674(01)00434-2; PMID: 11511343
- Pornillos O, Alam SL, Rich RL, Myszka DG, Davis DR, Sundquist WI. Structure and functional interactions of the Tsg101 UEV domain. EMBO J 2002; 21:2397 - 406; http://dx.doi.org/10.1093/emboj/21.10.2397; PMID: 12006492
- Alam SL, Sun J, Payne M, Welch BD, Blake BK, Davis DR, et al. Ubiquitin interactions of NZF zinc fingers. EMBO J 2004; 23:1411 - 21; http://dx.doi.org/10.1038/sj.emboj.7600114; PMID: 15029239
- Slagsvold T, Aasland R, Hirano S, Bache KG, Raiborg C, Trambaiolo D, et al. Eap45 in mammalian ESCRT-II binds ubiquitin via a phosphoinositide-interacting GLUE domain. J Biol Chem 2005; 280:19600 - 6; http://dx.doi.org/10.1074/jbc.M501510200; PMID: 15755741
- Carlton JG, Martin-Serrano J. The ESCRT machinery: new functions in viral and cellular biology. Biochem Soc Trans 2009; 37:195 - 9; http://dx.doi.org/10.1042/BST0370195; PMID: 19143630
- Hurley JH. ESCRT complexes and the biogenesis of multivesicular bodies. Curr Opin Cell Biol 2008; 20:4 - 11; http://dx.doi.org/10.1016/j.ceb.2007.12.002; PMID: 18222686
- Seet LF, Hong W. Endofin recruits clathrin to early endosomes via TOM1. J Cell Sci 2005; 118:575 - 87; http://dx.doi.org/10.1242/jcs.01628; PMID: 15657082
- Puertollano R. Interactions of TOM1L1 with the multivesicular body sorting machinery. J Biol Chem 2005; 280:9258 - 64; http://dx.doi.org/10.1074/jbc.M412481200; PMID: 15611048
- Blanc C, Charette SJ, Mattei S, Aubry L, Smith EW, Cosson P, et al. Dictyostelium Tom1 participates to an ancestral ESCRT-0 complex. Traffic 2009; 10:161 - 71; http://dx.doi.org/10.1111/j.1600-0854.2008.00855.x; PMID: 19054384
- Yamakami M, Yoshimori T, Yokosawa H. Tom1; a VHS domain-containing protein, interacts with tollip, ubiquitin, and clathrin. J Biol Chem 2003; 278:52865 - 72; http://dx.doi.org/10.1074/jbc.M306740200; PMID: 14563850
- Samson RY, Bell SD. Ancient ESCRTs and the evolution of binary fission. Trends Microbiol 2009; 17:507 - 13; http://dx.doi.org/10.1016/j.tim.2009.08.003; PMID: 19783442
- Pucadyil TJ, Schmid SL. Conserved functions of membrane active GTPases in coated vesicle formation. Science 2009; 325:1217 - 20; http://dx.doi.org/10.1126/science.1171004; PMID: 19729648
- Fabrikant G, Lata S, Riches JD, Briggs JA, Weissenhorn W, Kozlov MM. Computational model of membrane fission catalyzed by ESCRT-III. PLOS Comput Biol 2009; 5:e1000575; http://dx.doi.org/10.1371/journal.pcbi.1000575; PMID: 19936052
- Bodon G, Chassefeyre R, Pernet-Gallay K, Martinelli N, Effantin G, Hulsik DJ, et al. Charged multivesicular body protein-2B (CHMP2B) of the endosomal sorting complex required for transport-III (ESCRT-III) polymerizes into helical structures deforming the plasma membrane. J Biol Chem 2011; In press http://dx.doi.org/10.1074/jbc.M111.283671; PMID: 21926173