Abstract
Oxidative stresses can induce rapid depolarization of inner mitochondrial membrane potential and subsequent impairment of oxidative phosphorylation. Damaged mitochondria produce more reactive oxygen species (ROS), particularly the superoxide anion (O2-) and hydrogen peroxide (H2O2), which potentiate mitochondria-driven ROS propagation, so-called ROS-induced ROS release (RIRR), via activation of an inter-mitochondrial signaling network. In this context, mitochondrial network dynamics, such as their density, number, and spatial distribution, can affect mitochondria-driven ROS propagation. To investigate this inter-mitochondrial communication, we developed a mathematical model using an agent-based modeling approach, and tested the effect of mitochondrial network dynamics on RIRR for mitochondria under various conditions. Simulation results show that mitochondrial network dynamics are critical determinants of inter-mitochondrial ROS signaling patterns and main messenger ROS molecules. We further elucidated the potential mechanism of these actions, which is conversion of major messenger molecules involved in ROS signaling. Collectively, we propose that mitochondrial network dynamics can determine cellular responses to oxidative stress by switching the molecular species involved in cellular signaling.
Among the many intracellular organelles, mitochondria are the powerhouse that provides energy for other cellular processes. During cellular respiration, reactive oxygen species (ROS) are inevitably produced, which can cause oxidative damage to many cellular components including proteins, nucleic acids, lipid membranes, and even the mitochondria themselves.Citation1-Citation3Damaged mitochondria produce more ROS, particularly the superoxide anion (O2-) and hydrogen peroxide (H2O2), which potentiate mitochondria-driven ROS propagation, called ROS-induced ROS release (RIRR), via activation of an inter-mitochondrial signaling network ().Citation4,Citation5 Several studies have reported on RIRR and how loss of function of a small number of mitochondria might influence overall cell functioning.Citation4,Citation6,Citation7 Specifically, Zhou et al. developed a mathematical model of RIRR based on reaction-diffusion and identified novel aspects of the inter-mitochondrial synchronization mechanisms.Citation8However, most previous RIRR studies were based on a specialized context of intracellular mitochondrial dynamics within cardiomyocytes, taking advantage of the electrically independent distribution of rigid, lattice-like mitochondria suitable for high precision confocal line-scan imaging.Citation6,Citation9 In the real world, mitochondrial network dynamics, such as their density, number, and spatial distribution, can affect the pattern of ROS propagation in cells. Thus, many unknowns regarding mitochondrial dynamics and mitochondria-driven ROS propagation remain, and some current experimental results do not fit the existing model. To resolve this problem, a more systemic approach to study inter-mitochondrial networks is required.
Figure 1. Schematic diagram of RIRR and mitochondria-driven ROS propagation. (A) Oxidative phosphorylation occurring in the mitochondrial electron transport chain is an ATP producing mechanism. During this respiration process, ROS are produced when high-energy electrons escape before they reach the final acceptor O2. Damaged mitochondria produce more ROS, which potentiates RIRR and mitochondria-driven ROS propagation. Therefore, loss of function in only a fraction of the mitochondria may eventually affect the viability of the whole cell through this positive feedback loop. (B) The effect of two macro-variables, which are mitochondrial distribution and the number of mitochondria, on inter-mitochondrial ROS messenger molecules (mitochondrial distribution is dichotomous variable, so tested for regular and irregular cases). When mitochondrial distribution is regular and the number of mitochondria is relatively high (cardiomyocyte model, yellow spot), inter-mitochondrial ROS propagation is O2- dependent. However, the main messenger ROS molecules are converted to H2O2 when mitochondrial distribution is irregular (blue spot) or the cytosolic density of mitochondria becomesrelatively low (red spot). (C) Mitochondria-driven ROS propagation pattern of the yellow spot case in (B) (D) RIRR pattern of the green spot case in (B).
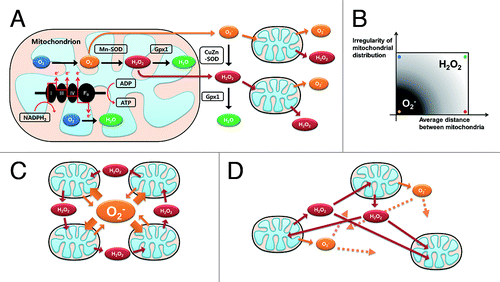
We created a mathematical model of inter-mitochondrial communication using the agent-based modeling (ABM)Citation10,Citation11 method, which allows controlling many variables of spatial information in molecular signaling systems. A mathematical and computational approach based on ABM is meaningful and reasonable in that it can simulate diverse scenarios by controlling variables independently, whereas design of in vitro/in vivo experiments incorporating such variables is practically impossible. In our model, local introduction of initial oxidative stress causes damage in that area, and ROS are propagated in every cycle. Simulation results revealed that the response to oxidative stress differed according to the status of mitochondrial network dynamics. The cardiomyocyte model was more dependent on the initial dose of oxidative stress compared with irregular distribution or low mitochondrial density models. Hence, an identical oxidative stress input can cause different responses, and the eventual fate of cells is related to mitochondrial network dynamics. We also elucidated conversion of main messenger ROS molecules from O2- to H2O2 in the irregularly distributed and low-number mitochondria models (). Our findings clearly suggest that mitochondrial network dynamics may determine the intracellular ROS propagation profile and cellular responses to oxidative stress by switching inter-mitochondrial ROS messengers.Citation12
In previous research, O2-, which is a more reactive ROS species than H2O2, has been considered important in the process of RIRRCitation4,Citation7,Citation8,Citation13,Citation14 Because such research was performed using only the cardiomyocyte model, which has a strictly regular distribution and high number of mitochondria, this explanation is reasonable only in that specific system. However, our simulation results revealed that mitochondrial network dynamics determine the identity of inter-mitochondrial ROS signaling and that H2O2-dependent ROS propagation occurs according to the distribution of mitochondria and distance between neighboring mitochondria. With this knowledge, several extraordinary findings in various cell types can be explained in that inhibition or reduction of CuZn-superoxide dismutase (SOD) increases resistance to oxidative stress,Citation15 and that treatment of cells with the SOD mimetic MnTBAP triggers abnormal hyperpolarization of mitochondria under oxidative stress (unpublished data). Our data also provide a plausible explanation for the correlation between oxidative stress vulnerability and the status of the cytoskeleton in cardiomyocytes. Colchicine is reported to disrupt microtubules by binding to tubulin subunits.Citation16 Because mitochondria are attached to and move along these microtubules, treatment with colchicine may break up the regular distribution of mitochondria into an irregular network. Therefore, our results also provide an explanation of how colchicine attenuates ischemic preconditioning-induced cardioprotection.Citation17
We showed that the degree of ROS propagation, effect of each antioxidant enzyme, and vulnerability to oxidative stress deciding the ultimate fate of a cell can be changed depending on the characteristics of mitochondrial network dynamics. What would be the cellular advantage of determining the profile of ROS signaling by mitochondrial network dynamics? Mitochondria have previously been shown to be highly dynamic organelles whose morphology, distribution, and activity can be regulated by fusion, fission, and migration.Citation18,Citation19 Thus, cells can modulate their own mitochondrial network dynamics to efficiently adapt to environmental oxidative stresses. By introducing ABM-based computational analysis, the complex dynamics of various cellular behaviors regarding RIRR was better understood and it may provide a rational background for development of mechanism-based therapeutic interventions.
Abbreviations: | ||
ABM | = | agent-based modeling |
RIRR | = | ROS-induced ROS release |
ROS | = | reactive oxygen species |
SOD | = | superoxide dismutase |
Acknowledgment
This study is supported by the Korea Science and Engineering Foundation (KOSEF) grant funded by the Korea government (MEST 2009–0080280) (to C.C.). The funders had no role in study design, data collection and analysis, decision to publish, or preparation of the manuscript.
References
- Orrenius S, Gogvadze V, Zhivotovsky B. Mitochondrial oxidative stress: implications for cell death. Annu Rev Pharmacol Toxicol 2007; 47:143 - 83; http://dx.doi.org/10.1146/annurev.pharmtox.47.120505.105122; PMID: 17029566
- Wallace DC. A mitochondrial paradigm of metabolic and degenerative diseases, aging, and cancer: a dawn for evolutionary medicine. Annu Rev Genet 2005; 39:359 - 407; http://dx.doi.org/10.1146/annurev.genet.39.110304.095751; PMID: 16285865
- Turrens JF. Mitochondrial formation of reactive oxygen species. J Physiol 2003; 552:335 - 44; http://dx.doi.org/10.1113/jphysiol.2003.049478; PMID: 14561818
- Aon MA, Cortassa S, Marban E, O'Rourke B. Synchronized whole cell oscillations in mitochondrial metabolism triggered by a local release of reactive oxygen species in cardiac myocytes. J Biol Chem 2003; 278:44735 - 44; http://dx.doi.org/10.1074/jbc.M302673200; PMID: 12930841
- Zorov DB, Juhaszova M, Sollott SJ. Mitochondrial ROS-induced ROS release: an update and review. Biochim Biophys Acta 2006; 1757:509-17.
- Zorov DB, Filburn CR, Klotz LO, Zweier JL, Sollott SJ. Reactive oxygen species (ROS)-induced ROS release: a new phenomenon accompanying induction of the mitochondrial permeability transition in cardiac myocytes. J Exp Med 2000; 192:1001 - 14; http://dx.doi.org/10.1084/jem.192.7.1001; PMID: 11015441
- Brady NR, Elmore SP, van Beek JJ, Krab K, Courtoy PJ, Hue L, et al. Coordinated behavior of mitochondria in both space and time: a reactive oxygen species-activated wave of mitochondrial depolarization. Biophys J 2004; 87:2022 - 34; http://dx.doi.org/10.1529/biophysj.103.035097; PMID: 15345578
- Zhou L, Aon MA, Almas T, Cortassa S, Winslow RL, O'Rourke B. A reaction-diffusion model of ROS-induced ROS release in a mitochondrial network. PLOS Comput Biol 2010; 6:e1000657; http://dx.doi.org/10.1371/journal.pcbi.1000657; PMID: 20126535
- Brady NR, Hamacher-Brady A, Westerhoff HV, Gottlieb RA. A wave of reactive oxygen species (ROS)-induced ROS release in a sea of excitable mitochondria. Antioxid Redox Signal 2006; 8:1651 - 65; http://dx.doi.org/10.1089/ars.2006.8.1651; PMID: 16987019
- Bankes SC. Agent-based modeling: a revolution?. Proc Natl Acad Sci USA 2002; 99:Suppl 3 7199 - 200; http://dx.doi.org/10.1073/pnas.072081299; PMID: 11997445
- Bonabeau E. Agent-based modeling: methods and techniques for simulating human systems. Proc Natl Acad Sci USA 2002; 99:Suppl 3 7280 - 7; http://dx.doi.org/10.1073/pnas.082080899; PMID: 12011407
- Park J, Lee J, Choi C. Mitochondrial network determines intracellular ROS dynamics and sensitivity to oxidative stress through switching inter-mitochondrial messengers. PLoS ONE 2011; 6:e23211; http://dx.doi.org/10.1371/journal.pone.0023211; PMID: 21829717
- Aon MA, Cortassa S, O'Rourke B. Percolation and criticality in a mitochondrial network. Proc Natl Acad Sci USA 2004; 101:4447 - 52; http://dx.doi.org/10.1073/pnas.0307156101; PMID: 15070738
- Aon MA, Cortassa S, Akar FG, O'Rourke B. Mitochondrial criticality: a new concept at the turning point of life or death. Biochim Biophys Acta 2006; 1762:232-40.
- Zaheer A, Yang B, Cao X, Lim R. Decreased copper-zinc superoxide dismutase activity and increased resistance to oxidative stress in glia maturation factor-null astrocytes. Neurochem Res 2004; 29:1473 - 80; http://dx.doi.org/10.1023/B:NERE.0000029558.82943.00; PMID: 15260123
- Sadoshima J, Takahashi T, Jahn L, Izumo S. Roles of mechano-sensitive ion channels, cytoskeleton, and contractile activity in stretch-induced immediate-early gene expression and hypertrophy of cardiac myocytes. Proc Natl Acad Sci USA 1992; 89:9905 - 9; http://dx.doi.org/10.1073/pnas.89.20.9905; PMID: 1384064
- Sharma A, Singh M. Possible mechanism of cardioprotective effect of ischaemic preconditioning in isolated rat heart. Pharmacol Res 2000; 41:635 - 40; http://dx.doi.org/10.1006/phrs.1999.0631; PMID: 10816332
- Chan DC. Mitochondrial fusion and fission in mammals. Annu Rev Cell Dev Biol 2006; 22:79 - 99; http://dx.doi.org/10.1146/annurev.cellbio.22.010305.104638; PMID: 16704336
- Frederick RL, Shaw JM. Moving mitochondria: establishing distribution of an essential organelle. Traffic 2007; 8:1668 - 75; http://dx.doi.org/10.1111/j.1600-0854.2007.00644.x; PMID: 17944806