Abstract
Tight control of intracellular signaling is essential for developmental processes such as cell differentiation, migration but also for maintaining tissue homeostasis. Disruption in the control of these signaling pathways can result in cell death (apoptosis), anergy or uncontrolled cell proliferation and growth leading to cancer. In multicellular organisms, timely termination of signaling is thus equally important as initiation. Known pathways for downregulating membrane receptor-mediated signaling are mediated via specialized endosomal organelles known as lysosomes and proteosomes that degrade such proteins in the cytoplasm. An alternative pathway for attenuating receptor-mediated signaling was recently discovered independently by the group of M. Caplan and our own group.1,2 It appears that apart from the classical protein degradation machineries, the release of signaling proteins also effectively restricts signaling of at least two major signal transduction routes; the canonical Wnt/β-catenin and NFκB pathways. Expelling proteins from the cell, rather than coordinated degradation in lysosomes may involve defined protein modifications, such as ubiquitination, myristyolation, and/or palmitoylation, but little experimental data are currently available. Although the secretion of proteins via exosomes starts by accumulation within multivesicular bodies (MVBs), a key distinction with degredatory MVBs is that exosome-producing MVBs seem to preferentially fuse with the plasmamembrane (Fig. 1). Here we discuss the latest developments in the biology of exosomes and their unexpected effect on intracellular signal transduction.
Figure 1. Model for LMP1 trafficking and signaling as compared with EGFR. Model that highlights the distinction between latent membrane protein 1 (LMP1, in blue) trafficking via multivesicular bodies (MVB) into exosomes and Endothelial Growth Factor Receptor (EGFR, in red) trafficking and degradation in lysosomes. (1) LMP1 is synthesized in the ER and traffics through the Golgi (G). Subsequently, LMP1 traffics to and accumulates in microdomains of the limiting membrane (LM) of multivesicular bodies (MVBs). Inward budding of microdomains that contain LMP1 form intraluminal vesicles (ILVs). (2) ILVs that contain LMP1 are secreted as exosomes upon fusion of the LM with the plasma membrane (PM). In all steps from Golgi to LM the C-terminus of LMP1 that mediates NF-kB signaling is exposed to the cytoplasm. EGFR in contrast traffics to the plasma membrane (A), is ubiquitinated and internalized in early endosomes (B). A fraction is re-expressed at the PM via recycling endosomes (C), the remainder is incorporated in MVBs (D) and targeted for degradation (E) by fusion with lysosomes (L). Both PM associated and internalized EGFR signal after EGF binding.
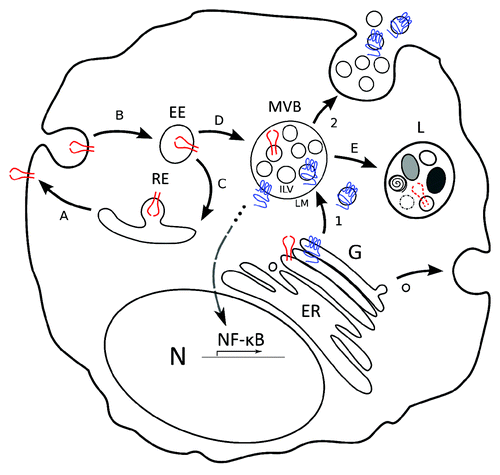
Exosomal discharge as a novel mechanism for signaling attenuation
Latent membrane protein-1 (LMP1) is considered the main oncoprotein encoded by the human tumor virus Epstein-Barr (EBV) as it has classic transforming activity in rodent fibroblasts.Citation3 LMP1 is a constant firing signaling-homolog of human CD40, which biological function is to ensure efficient maturation of naive B-cells into long-lived memory B-cells. Antibody affinity maturation is driven primarily by competition for T cell help, indicating that interactions between CD40-ligand expressed on T cell and CD40 on B cells is crucial for memory B cell development.Citation4 Interestingly, these memory B cells are the ultimate hiding-place for latent EBV infection in humans when no viral genes are expressed except occasionally EBNA-1. LMP1 contains six transmembrane regions that mediate trafficking and intermolecular (self)aggregation which are required for initiating downstream signaling via recruitment of TNF receptor-associated factors (TRAF) at the cytoplasmic C-terminus. In vitro, LMP1 expression is critical for efficient transformation of naive B-cellsCitation5 by inducing constitutive NFκB activation upon infection. Chronic NFκB activation may have oncogenic consequences and drives the continuous proliferation of EBV-infected lymphoma cells.Citation6 The oncogenic and growth-promoting properties of LMP1 are directly associated with its ability to signal without a ligand.Citation7 Forced mono-ubiquitylation at the N-terminus of LMP1 leads to a strong increase in LMP1 breakdown, but does not result in strikingly attenuated NFκB activation, suggesting alternative regulatory pathways that are functional apart from classic proteasomal and lysosomal degradation.Citation1 Indeed we recently discovered that endosome driven release of LMP1 via exosomes, chaperoned by the tetraspanin family member CD63, strongly reduces LMP1-mediated NFκB signaling. Knock-down of CD63 by short hairpin RNAs (RNAi) against CD63, lead to sequestering of LMP1 protein in intracellular compartments and reduced LMP1 release through exosomes that strongly increased NFκB activation. CD63 belongs to the evolutionary conserved family of tetraspanins proteins that assemble in tiny membranous platforms that guide intracellular trafficking of their associated-partners. Thus EBV encoded LMP1 may have co-opted the physiological role of CD63 in human B cells for its own benefit.
In a conceptually similar study, Chairoungdua et al.Citation2 reported on the crucial involvement of tetraspanins in the Wnt signal-transduction pathway. Wnt proteins are a group of secreted, lipid-modified, signaling glycoproteins ranging between 350–400 amino acids in length.Citation8 Wnt binds co-receptors Frizzled (FRZ) and low-density lipoprotein receptor-related protein (LRP) 5/6, resulting in the activation of the Dishevelled (Dvl) protein, which then prevents Glycogen Synthase Kinase 3β (GSK3β)–mediated phosphorylation and subsequent degradation of β-catenin. As a result, β-catenin accumulates in the cytosol and translocates to the nucleus where it binds to the transcription factors T-cell factor (TCF) and lymphoid enhancer factor (LEF). Activation of TCF/LEF results in direct binding to Wnt responsive elements (WREs) and the activation of target gene expression, including Met, a proto-oncogene encoding the tyrosine kinase receptor for HGF.Citation9 Gene expression resulting from Wnt signaling and cross-talk with other factors ultimately drive cell fate decisions, including proliferation/survival activities and changes in cell shape.Citation10 Therefore, it is not surprising that there is a clear link between aberrant Wnt-signaling and cancer, mediated by stabilized β–catenin in the cytoplasm and increased translocation to the nucleus.Citation11 Thus, exosomal secretion of the β-catenin via the E-cadherin complex to inhibit Wnt signaling, may be an effective alternative method to prevent overstimulation. Provocatively, this effect was enhanced by exogenous expression of the tetraspanins CD82 and CD9 (but not CD63) suggesting that tetraspanins are important for the proper (down) regulation of Wnt signaling by mediating endosomal trafficking and secretion via exosomes,Citation2 similar to the role of CD63 in LMP1-mediated NFκB activation as we described in B cells.
Tetraspanins
Tetraspanins in exosomes
Tetraspanins are a major class of proteins in exosomes secreted by multiple cell-types;Citation12 indicating a tight link between the exosomal pathway and this family of scaffolding proteins. Tetraspanins may function by selectively collecting partner proteins for incorporation into exosomes at endolysosomal membranes. Indeed, apart from LMP1 and β-catenin, other proteins that are secreted via exosomes also seem closely associated with tetraspanins such as the common HLA-DR molecule expressed in immune cells.Citation13 Whether tetraspanins have a role in the actual biogenesis of exosomes at the limiting membrane of endosomes is unclear. Possibly, inward budding of the limiting membrane of MVBs is potentiated by tetraspanins microdomains (TEMs) to form ILVs. TEMs may vary in size ranging from micro- to nanometer scale, depending on the techniques used. Such detailed distinctions are possibly important for our understanding on the biology of endosome-derived exosomes. Neither is it clear whether a specificity for targeting of (lipid) microdomains to different “classes” of MVBs exist. Despite a lack of experimental evidence, distinct mechanisms for ILV biogenesis have been proposed.Citation14 These could also play a role in the diversification between MVBs that are destined to fuse with lysosomes for degradation or those that preferentially fuse with the plasma membrane for release of cargo via exosomes. Whether the latter “class” of MVBs will contain mixed populations of ILVs or whether there might be a further diversification into “sub-classes” of MVBs remains uncertain. However, if one assumes that all secreted exosomes are biologically functional, simultaneous release of mixed ILV populations from a single MVB, seems incompatible with the presumed targeted effects of exosomes in local microenvironments.Citation15,Citation16 Thus, increasing selectivity of exosome function in vivo maybe more efficient when creating similar ILV in distinct subclasses of MVBs. Possibly pathogens such as EBV may disturb such coordinated release of functional exosomes by insertion of its own viral gene products.Citation1,Citation17
Tetraspanin modifications
Members of the tetraspanin superfamily are characterized by four transmembrane spanning domains, a small (EC1) and a large (EC2) extracellular loop with a highly conserved ‘CCG’ motif. CD9, CD63, and CD82 are glycosylated and can be palmitoylated at their juxtamembrane cysteine residues. CD63 is differentially glycosylated during maturation steps of immune cells.Citation18 Glycosylation and most myristoylation modifications are irreversible and function by promoting protein integrity and guiding proper intracellular trafficking. Palmitoylation is distinct as it is reversible in nature and may specifically contribute to the organization and flexibility of the tetraspanin-web.Citation19 This web of tetraspanin scaffolds facilitates proteins in temporal as well as spatial engagement into a diversity of microdomains, and is thereby of structurally importance for proper protein trafficking and signaling.
Late Endosomes in Signaling
From a signaling perspective, late endosomes/MVBs are convenient intracellular compartments for regulatory purposes. These compartments may allow “back-fusion” of ILVs with the limiting membrane such that presumably inactive signaling molecules stored in MVBs may become immediately accessible upon contact with the cytosol. Moreover being packed already in small membranous domains could facilitate signaling initiation. Currently, back-fusion in MVBs has only been described for invading infectious agents and thus concerns entry, rather then re-entry of the cytosol.Citation20,Citation21 The process of back-fusion might be facilitated by local concentrations of specific lipids such as lysobisphosphatidic acid (LBPA) and/or its putative effectors Alix and/or tetraspanins CD9 and CD81 known to mediate membrane fusion events.Citation14,Citation22 A delicate balance between inward budding and back-fusion of ILVs/TEMs would allow precise and defined localized control over signaling potency. Alternatively, back-fusion may enable MVBs to function as a repository for proteins needed for acute responses, i.e., temporary storage for signaling receptors, which may allow rapid adjustment to changing environments (). So far systematic studies into the endocytic pathway showed that mTORC1 signals from MVBs,Citation23 and a recently proposed model for Notch activation by Delta assigned a crucial role to MVBs in Notch signaling that critically relies on its multi-vesicular morphology.Citation24
Samuel Liégeois et al.Citation25 showed that mutations in H+-ATPase (V-ATPase) that is required for exosome and morphogen (Hedgehog-related) release in C. elegans led to accumulation of MVBs and impaired larval development.
Before entry into MVBs most (RTK) receptors are activated at the plasma-membrane by ligand-binding and are subsequently endocytosed (internalized) into early endosmes.Citation26 The vesicular-mediated transport of receptors from the plasma membrane is considered to lower the amount of receptors exposed on the cell’s surface thereby controlling signaling strength. Recent findings however suggest this is only partly true, and focus is now shifting toward endosomal vesicles themselves as important platforms for initiation, elongation, and termination of receptor signaling.Citation27 Joffre et al.Citation28 showed that Met, the receptor for Hepatocyte Growth Factor (HGF), requires endocytosis for downstream activation of its pathway. Strikingly, the transforming phenotype of a tumor-associated Met-activating mutation was inhibited by an endocytosis inhibitor, while maintaining its activation status. Taken together, accumulating evidence now shows that not the mere presence of signaling molecules in subcellular membranes determines signaling, but that association within specific localized microdomains plays a prominent role.Citation29
In addition, endocytosis and subsequent loading into MVBs of non-RTK signaling complexes such as the Wnt/GSK3 receptor complex for instance, is critical for Wnt-signaling. However, here GSK3 is a negative regulator that is excluded from its cytosolic substrates such as β-catenin.Citation30 In light of the recent findings by Chairoungdua et al., MVBs may play a dual role in the regulation of Wnt signaling. It is unclear whether Wnt/GSK3 receptor complex containing MVBs function as temporary storage or whether they are destined for secretion or lysosomal degradation.Citation30 ()
LMP1 trafficking and signaling
It was long presumed that LMP1, being a mimic of the surface receptor CD40 localizes to and signals from the plasma membrane. Yet mutants that are directed specifically to the plasma membrane, do not reveal increased signaling potency. One deletion mutant of LMP1 that lacks the first two trans-membrane regions of LMP1 (ΔTM1–2 LMP1) is prominently present in the PM. ΔTM1–2 LMP1 is devoid of a putative lipid-raft anchoring domain ‘FWLY’ and seems to preferentially accumulate in the plasma membrane () compared with wild type LMP1. Accordingly, expression of WT-LMP1 in HEK293 cells leads to a ~30% decrease in CD63 surface levels (SD 0,08%) measured by FACS, while this was not seen with ΔTM1–2 LMP1 (95%; SD 1,37%) (). Since we do not observe much LMP1 protein without CD63 in vesicles at the ER/Golgi region, we reason that LMP1 probably associates with CD63 before reaching the plasma membrane. What could be a biological explanation for LMP1 mimicking CD40 signaling from intracellular compartments rather than the plasmamembrane? Being a ligand-independent receptor there seems no apparent need for LMP1 to traffic to the surface, as opposed to EGFR (, in red) or CD40. It is more likely that LMP1 is transported away from the Golgi, directly to MVBs. Indeed we and others found that endogenous LMP1 accumulates in ILVs of MVBsCitation1,Citation31 (). In fact, endogenous LMP1 is hardly detected at all on the surface of B cells. However, if LMP1 is rapidly internalized via lipid-rafts, it remains possible that the plasma membrane has a role in LMP1 physiology (). Ultimately however, endogenous and exogenously expressed LMP1 are sorted into endosomes and exosomes for secretion, raising the important question, why LMP1 seems preferentially secreted instead of being degraded?
Figure 3. LMP1 reduces CD63 surface expression. (A) HEK293 cells labeled for LMP1, showing cytoplasmic staining pattern of LMP1-wt, and mainly plasmamembrane localized LMP1-dTM12 expression. (B) CD63 surface expression (mean fluorescence intensity, MFI) in HEK293 cells transfected with LMP1-wt or LMP1-dTM12 together with GFP-N1, or control (ctrl, GFP-negative).
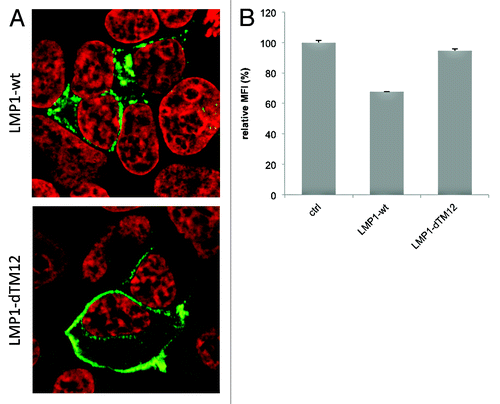
Figure 2. LMP1 localizes predominantly to the intraluminal vesicles of late endosomes in the cytoplasm. Immuno-histochemical staining of LMP1 in Hodgkins Disease lymphoid tissue, showing a characteristic heterogeneous expression of LMP1 in EBV containing Reed-Sternberg cells (upper left; nuclei in blue, LMP1 brown staining). Confocal staining of LMP1 (Alexa594) and CD63 (FITC) in LCLs, showing (upper right). Electron microscopy images on ultrathin cryosection of LCL, showing a late endosome containing ILVs labeled for LMP1 (lower left). (bar is 100nm) Immunogold labeling for LMP1 on purified exosomes isolated from LCL cultures by differential ultracentrifugation(lower right).
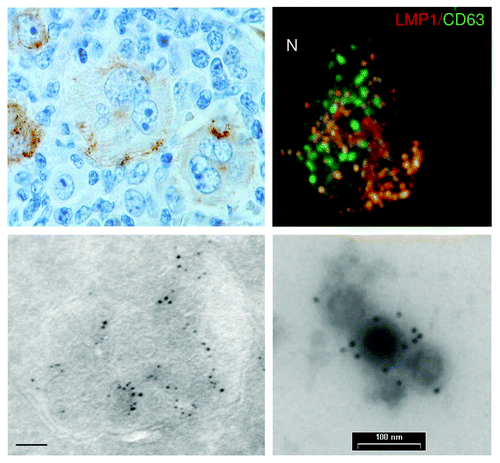
Conclusions
Future questions that need to be addressed regarding the incorporation of signaling proteins into the endo-exosomal pathway are plentiful. What would be the benefit of secretion instead of degradation of proteins, in controlling signaling? A logical answer could be that secreted proteins serve an additional purpose after binding and/or uptake by recipient cells. Indeed, Wnt is known to be transferred trans-synaptically via exosomes and is able to signal In Trans.Citation32 In this scenario the protein is actively sorted and secreted in exosomes. Taken together, current in vitro findings support a new concept for receptor regulation via shared trafficking with tetraspanins in the endosomal-exosomal pathway, involving defined subdomains of palmitoylated proteins, tetraspanins and certain lipids. A challenge for the future would be to translate these models into in vivo studies and defining a role for exosome secretion of signaling intermediates in health and disease.
References
- Verweij FJ, van Eijndhoven MAJ, Hopmans ES, Vendrig T, Wurdinger T, Cahir-McFarland E, et al. LMP1 association with CD63 in endosomes and secretion via exosomes limits constitutive NF-κB activation. EMBO J 2011; 30:2115 - 29; http://dx.doi.org/10.1038/emboj.2011.123; PMID: 21527913
- Chairoungdua A, Smith DL, Pochard P, Hull M, Caplan MJ. Exosome release of β-catenin: a novel mechanism that antagonizes Wnt signaling. J Cell Biol 2010; 190:1079 - 91; http://dx.doi.org/10.1083/jcb.201002049; PMID: 20837771
- Wang D, Liebowitz D, Kieff E. An EBV membrane protein expressed in immortalized lymphocytes transforms established rodent cells. Cell 1985; 43:831 - 40; http://dx.doi.org/10.1016/0092-8674(85)90256-9; PMID: 3000618
- Victora GD, Schwickert TA, Fooksman DR, Kamphorst AO, Meyer-Hermann M, Dustin ML, et al. Germinal center dynamics revealed by multiphoton microscopy with a photoactivatable fluorescent reporter. Cell 2010; 143:592 - 605; http://dx.doi.org/10.1016/j.cell.2010.10.032; PMID: 21074050
- Dirmeier U, Neuhierl B, Kilger E, Reisbach G, Sandberg ML, Hammerschmidt W. Latent Membrane Protein 1 Is Critical for Efficient Growth Transformation of Human B Cells by Epstein-Barr Virus. Cancer Res 2003; 63:2982 - 9; PMID: 12782607
- Guasparri I, Bubman D, Cesarman E. EBV LMP2A affects LMP1-mediated NF-kappaB signaling and survival of lymphoma cells by regulating TRAF2 expression. Blood 2008; 111:3813 - 20; http://dx.doi.org/10.1182/blood-2007-03-080309; PMID: 18230756
- Gires O, Zimber-Strobl U, Gonnella R, Ueffing M, Marschall G, Zeidler R, et al. Latent membrane protein 1 of Epstein-Barr virus mimics a constitutively active receptor molecule. EMBO J 1997; 16:6131 - 40; http://dx.doi.org/10.1093/emboj/16.20.6131; PMID: 9359753
- Cadigan KM, Nusse R. Wnt signaling: a common theme in animal development. Genes Dev 1997; 11:3286 - 305; http://dx.doi.org/10.1101/gad.11.24.3286; PMID: 9407023
- Boon EMJ, van der Neut R, van de Wetering M, Clevers H, Pals ST. Wnt signaling regulates expression of the receptor tyrosine kinase met in colorectal cancer. Cancer Res 2002; 62:5126 - 8; PMID: 12234972
- Arce L, Yokoyama NN, Waterman ML. Diversity of LEF/TCF action in development and disease. Oncogene 2006; 25:7492 - 504; http://dx.doi.org/10.1038/sj.onc.1210056; PMID: 17143293
- Polakis P. Wnt signaling and cancer. Genes Dev 2000; 14:1837 - 51; PMID: 10921899
- Escola J-M. Selective Enrichment of Tetraspan Proteins on the Internal Vesicles of Multivesicular Endosomes and on Exosomes Secreted by Human B-lymphocytes. J Biol Chem 1998; 273:20121 - 7; http://dx.doi.org/10.1074/jbc.273.32.20121; PMID: 9685355
- Rubinstein E, Le Naour F, Lagaudrière-Gesbert C, Billard M, Conjeaud H, Boucheix C. CD9, CD63, CD81, and CD82 are components of a surface tetraspan network connected to HLA-DR and VLA integrins. Eur J Immunol 1996; 26:2657 - 65; http://dx.doi.org/10.1002/eji.1830261117; PMID: 8921952
- Simons M, Raposo G. Exosomes–vesicular carriers for intercellular communication. Curr Opin Cell Biol 2009; 21:575 - 81; http://dx.doi.org/10.1016/j.ceb.2009.03.007; PMID: 19442504
- Fitzner D, Schnaars M, van Rossum D, Krishnamoorthy G, Dibaj P, Bakhti M, et al. Selective transfer of exosomes from oligodendrocytes to microglia by macropinocytosis. J Cell Sci 2011; 124:447 - 58; http://dx.doi.org/10.1242/jcs.074088; PMID: 21242314
- Mittelbrunn M, Gutiérrez-Vázquez C, Villarroya-Beltri C, González S, Sánchez-Cabo F, González MÁ, et al. Unidirectional transfer of microRNA-loaded exosomes from T cells to antigen-presenting cells. Nat Commun 2011; 2:282; http://dx.doi.org/10.1038/ncomms1285; PMID: 21505438
- Pegtel DM, Cosmopoulos K, Thorley-Lawson DA, van Eijndhoven MAJ, Hopmans ES, Lindenberg JL, et al. Functional delivery of viral miRNAs via exosomes. Proc Natl Acad Sci USA 2010; 107:6328 - 33; http://dx.doi.org/10.1073/pnas.0914843107; PMID: 20304794
- Engering A, Kuhn L, Fluitsma D, Hoefsmit E, Pieters J. Differential post-translational modification of CD63 molecules during maturation of human dendritic cells. Eur J Biochem 2003; 270:2412 - 20; http://dx.doi.org/10.1046/j.1432-1033.2003.03609.x; PMID: 12755696
- Zöller M. Tetraspanins: push and pull in suppressing and promoting metastasis. Nat Rev Cancer 2009; 9:40 - 55; http://dx.doi.org/10.1038/nrc2543; PMID: 19078974
- Abrami L, Lindsay M, Parton RG, Leppla SH, van der Goot FG. Membrane insertion of anthrax protective antigen and cytoplasmic delivery of lethal factor occur at different stages of the endocytic pathway. J Cell Biol 2004; 166:645 - 51; http://dx.doi.org/10.1083/jcb.200312072; PMID: 15337774
- Le Blanc I, Luyet P-P, Pons V, Ferguson C, Emans N, Petiot A, et al. Endosome-to-cytosol transport of viral nucleocapsids. Nat Cell Biol 2005; 7:653 - 64; http://dx.doi.org/10.1038/ncb1269; PMID: 15951806
- Murk JLAN, Humbel BM, Ziese U, Griffith JM, Posthuma G, Slot JW, et al. Endosomal compartmentalization in three dimensions: implications for membrane fusion. Proc Natl Acad Sci USA 2003; 100:13332 - 7; http://dx.doi.org/10.1073/pnas.2232379100; PMID: 14597718
- Flinn RJ, Backer JM. mTORC1 signals from late endosomes: taking a TOR of the endocytic system. Cell Cycle 2010; 9:1869 - 70; http://dx.doi.org/10.4161/cc.9.10.11679; PMID: 20436274
- Traub LM. The reverse logic of multivesicular endosomes. EMBO Rep 2010; 11:79 - 81; http://dx.doi.org/10.1038/embor.2009.281; PMID: 20062001
- Liégeois S, Benedetto A, Garnier J-M, Schwab Y, Labouesse M. The V0-ATPase mediates apical secretion of exosomes containing Hedgehog-related proteins in Caenorhabditis elegans. J Cell Biol 2006; 173:949 - 61; http://dx.doi.org/10.1083/jcb.200511072; PMID: 16785323
- Sorkin A, von Zastrow M. Endocytosis and signalling: intertwining molecular networks. Nat Rev Mol Cell Biol 2009; 10:609 - 22; http://dx.doi.org/10.1038/nrm2748; PMID: 19696798
- Vieira AV, Lamaze C, Schmid SL. Control of EGF Receptor Signaling by Clathrin-Mediated Endocytosis. Science 1996; 274:2086 - 9; http://dx.doi.org/10.1126/science.274.5295.2086; PMID: 8953040
- Joffre C, Barrow R, Ménard L, Calleja V, Hart IR, Kermorgant S. A direct role for Met endocytosis in tumorigenesis. Nat Cell Biol 2011; 13:827 - 37; http://dx.doi.org/10.1038/ncb2257; PMID: 21642981
- Sadowski L, Pilecka I, Miaczynska M. Signaling from endosomes: location makes a difference. Exp Cell Res 2009; 315:1601 - 9; http://dx.doi.org/10.1016/j.yexcr.2008.09.021; PMID: 18930045
- Taelman VF, Dobrowolski R, Plouhinec J-L, Fuentealba LC, Vorwald PP, Gumper I, et al. Wnt signaling requires sequestration of glycogen synthase kinase 3 inside multivesicular endosomes. Cell 2010; 143:1136 - 48; http://dx.doi.org/10.1016/j.cell.2010.11.034; PMID: 21183076
- Lam N, Sugden B. LMP1, a viral relative of the TNF receptor family, signals principally from intracellular compartments. EMBO J 2003; 22:3027 - 38; http://dx.doi.org/10.1093/emboj/cdg284; PMID: 12805217
- Korkut C, Ataman B, Ramachandran P, Ashley J, Barria R, Gherbesi N, et al. Trans-synaptic transmission of vesicular Wnt signals through Evi/Wntless. Cell 2009; 139:393 - 404; http://dx.doi.org/10.1016/j.cell.2009.07.051; PMID: 19837038