Abstract
Assimilatory nitrate reductase (NR; EC 1.7.1.1-3) catalyzes the reduction of nitrate to nitrite. This enzyme has a conserved structure common to fungi, algae and plants. However, some differences in the amino acid sequence between plant and algal NR suggest that the activity regulation mechanisms have changed during plant evolution. Since only NRs from angiosperms have been studied, the search and analysis of NR genes and proteins from the moss Physcomitrella patens, a basal land plant, was performed to widen the knowledge of land plant NR structure. A family of three nr genes, named ppnia1;1, ppnia1;2 and ppnia2, was localized in the P. patens genome. The predicted proteins are canonical NRs with the conserved domains Molybdene-Cytochorme b –Cytochrome b reductase and possess 20 amino acid residues important for the enzymatic function conserved in plant and algal NRs. Interestingly, moss NRs lack a consensus sequence, common to angiosperm NRs, that is a target for posttranslational regulation. A phylogenetic tree with embryophyte and green algae NR sequences was constructed and P. patens NRs localized at the base of embryophyte NR evolution. The data presented here suggest that bryophytes and vascular plants have different systems to regulate NR activity.
Introduction
Nitrogen is a fundamental macronutrient present in nucleic acids, proteins and cofactors in all organisms. An important way by which plants incorporate nitrogen to their metabolism is the assimilation of nitrate from the soil; nitrate is then converted to ammonia and further incorporated into amino acids. The first enzyme in this process is assimilatory nitrate reductase (NR; EC 1.7.1.1–3), a protein present in fungi, algae and plants.Citation1 NR is a dimeric soluble protein of around 100 kDa composed of three conserved domains forming an internal electron transport system. A Cyt b reductase domain, located at the C-terminus of the protein, takes electrons from NAD(P)H through its FAD cofactor; the electrons are channeled through a Cyt b domain to the MoCo domain, in the N-terminus of the protein, where they are used to reduce nitrate to nitrite.Citation2-Citation4 Beside its nitrate assimilatory function, NRs also use nitrite as substrate to produce nitric oxide (NO) in higher plants.Citation5,Citation6 During the last decade, it has been shown that NO derived from NR is involved in root growth, stomatal closure, flower development and other responses in higher plants.Citation7-Citation9 The green algae Chlamydomonas reinhardtii also produce NO in a nitrite-dependent manner; a mutant line lacking functional NR lost the capacity to produce NO, indicating that green algae NRs also reduce nitrate to NO.Citation10 Thus, there is evidence that NR activity is important not only for nitrogen metabolism, but also for NO production in photosynthetic eukaryotes.
Expression of NR is tightly regulated by external and internal cues such as nitrate and sugar levels, photosynthesis rate and light.Citation1,Citation11 In higher plants, NR activity is downregulated by low CO2 levels and transition from light to dark.Citation11,Citation12 The mechanism for NR inactivation in all known plant NRs is the reversible binding of a 14–3-3 protein to a phosphorylated Serine residue located in a conserved consensus sequence between the Cyt b and Cyt b reductase domains (see the review by MacKintosh and Meek).Citation13 NR enzymes have a long evolutionary history; Stolz and BasuCitation14 analyzed the phylogenetic relations of NRs from all organisms, demonstrating that eukaryotic NR have a monophyletic origin and that a three domain protein structure is conserved among fungi, algae and land plants, as well as the amino acid residues important for nitrate reduction. However, there is evidence that the NR gene structure and protein activity regulation has changed during plant evolution. The NR from the green alga C. reinhardtii is not regulated by a 14–3-3 protein.Citation15 Moreover, NRs from C. reinhardtii and other green, brown and red algae lack the consensus sequence recognized by 14–3-3 proteins,Citation16,Citation17 suggesting that this regulation mechanism is exclusive of plant NRs. However, the activity of red and green algae is also regulated at the posttranscriptional level by unknown mechanisms.Citation16,Citation18-Citation20
Furthermore, green algae nr genes are interrupted by a variable number of introns,Citation17,Citation20,Citation21 while angiosperm nr genes have only tree introns in conserved positions,Citation22 indicating that during land plant evolution, the intron number in nr genes has been reduced.
In view of the fundamental role of NR in nitrogen metabolism and differences in gene structure and posttranslational regulatory mechanisms between green algae and angiosperms, the interesting question of how these characteristics evolved during land plant evolution emerges. In virtue of this, we present the first analysis of NRs from a basal embryophyte, as a way to better understand the NRs evolution in land plants. Bryophytes (liverworts, hornworts and mosses) emerged ~450 million years ago and are considered the basal clade in the land plant tree of life.Citation23,Citation24 Among the bryophytes, the mosses are the most diverse group and have been studied since 1930s. During the past decade, the moss Physcomitrella patens has emerged as very useful model for the analysis of all aspects of plant biology and evolution, reviewed by Cove et al.Citation25 and Cove,Citation26 and the release of the P. patens complete genome sequenceCitation27 boosted its use as model plant. In this work we present the identification of a family of three nr genes from P. patens, named ppnia1;1, ppnia1;2 and ppnia2. The predicted NR sequences showed that P. patens NRs are canonical NR proteins composed by the three conserved domains, MoCo, Cyt b and Cyt b reductase and posses 20 conserved amino acid residues important for enzyme function. Interestingly, P. patens NRs lack the consensus sequence recognized by 14–3-3 proteins common to all studied angiosperms, suggesting that its activity is not downregulated by this mechanism.
Results and Discussion
Identification of the nitrate reductase genes from Physcomitrella patens genome. The nitrate reductase 1 from Nicotiana benthamiana (accession number BAE46746.1) was used as probe to perform a search for nr genes in the P. patens genome database cosmoss (www.cosmoss.org). Four putative genes were found, two of them in scaffold 58 (named Pp1s58_249v6.1 and Pp1s58_252v6.1) and two in scaffold 79 (named Pp1s79_76v6.1 and Pp1s79_76v6.2). The last two sequences occupy the same position in the scaffold and the sequences are identical, demonstrating that only one gene exists in that position. Thus, Pp1s79_76v6.1 was used to continue this work. The protein sequence deduced from Pp1s58_252v6.1 was used to search for similar genes in the GenBank database. The sequences annotated as PpNia1;1 (accession number BAE19754.1) and PpNia1;2, (accession number BAE19755.1) corresponded with Pp1s58_252v6.1 and Pp1s79_76v6.1, respectively. A third sequence (accession number XP_001762979.1) corresponded to sequence Pp1s58_249v6.1 from the cosmoss database and we called it PpNia2. The size and position of the three nr genes from P. patens are shown in . The genes ppnia1;1 and ppnia2 are localized in the scaffold 58, they are 50.89 kb apart and have opposite translation orientation; ppnia1;2 is located in a different scaffold. The three genes are predicted to have six introns located at the same positions. Interestingly, nr genes in angiosperms only have three introns of variable length in conserved positions;Citation22 the only data available from a non-seed vascular plant, the Lycophyte Selaginella moellendorffii, indicate that its nr genes have five and four introns in nia1 and nia2, respectively (http://genome.jgi-psf.org/Selmo1/Selmo1.home.html); finally, the number of introns in nr genes from green algae varies from two in Dunaliella tertiolectaCitation17 to 10 and 18 in Volvox carteri and Chlorella vulgaris, respectively.Citation20,Citation21 This suggests that during Viridiplantae evolution the nr genes have lost some introns. The study of introns loss/gain has become a useful tool to analyze gene family evolution.Citation28 Thus, the apparent tendency to the reduction of intron number in nr genes during land plant evolution deserves further research as new nr gene sequences from non-seed vascular plants and bryophytes are available.
Figure 1. Map of the Physcomitrella patens nitrate reductase gene family. Scaffolds correspond to the P. patens genome database (http://www.cosmoss.org/). Arrows show the translational orientation of the genes. Gene length includes the 5′and 3′untranslated regions. Map not drawn to scale.
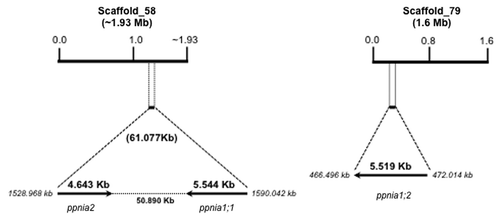
Amino acid sequences analysis of NRs from P. patens. The predicted amino acid sequence from P. patens NRs were obtained and compared. PpNia1;1 and PpNia2 have 892 amino acids, their sequences are almost identical (99.8%), they differ only in two amino acids at positions 39 and 61 where Asn and Thr are found in PpNia1;1 while Lys and Ala are found in PpNia2, respectively. PpNia1;2 has 893 amino acids and has 89.5% similarity and 80.1% identity with PpNia1;1. The sequence of PpNia1;1 and PpNia1;2 were compared with Selaginella moellendorffii NR 1, (Protein ID XP_002972481.1) and with selected angiosperm species (). The alignment shows that P. patens NRs share the general structure of canonical eukaryotic NRs, they contain the MoCo-Cyt b-Cyt b reductase domains. P. patens NRs possess 20 conserved amino acids involved in NR function ( and ). Among them the most important are a Cys residue (C200) that binds the MoCo; two His residues (H592 and H615) in the Cyt b domain that coordinate the heme iron, and three amino acids in the Cyt b reductase domain that are involved in the NAD(P)H union, two of them, K757 and G820, are conserved in the three P. patens enzymes, but C919 is absent in PpNia1;2. This residue is considered to be the active site for electron transfer from NAD(P)H to FAD. Studies with Cyt b reductase fragment mutants from corn NR and Neurospora crassa NADPH:NR showed that any substitution of the invariant Cys severely reduce the NAD(P)H oxidase activity (Reviewed by Campbell).Citation1 These data suggest that PpNia1;2 may have different catalytic rates than the other two enzymes.
Figure 2. Amino acid sequence comparison between P. patens NR and selected vascular plant NR. Underlined sections indicate the protein domains: MoCo, solid line; Cyt b, dotted line and Cyt b reductase, dashed line. Conserved amino acid residues important for NR function are underlined and in bold type. Gray shaded sequence indicates the binding site for 14–3-3 proteins, conserved in vascular plants. Note that P. patens NRs lack that sequence and have E instead of S in the phosphorylation site. Sequences were obtained from the GenBank database using the following accession numbers: Nicotiana benthamiana nia1 (BAE46746.1); Cucumis sativus nia2 (ADN96689.1); Arabidopsis thaliana nia1 (NP_177899.1); Zea mays nia1 (AAD38068.1); Selaginella moellendorffii nia1 (XP_002972481.1); Physcomitrella patens nia1;1 (BAE19754.1) and nia1;2 (BAE19755.1). Amino acid numbering corresponds to the N. benthamiana sequence.
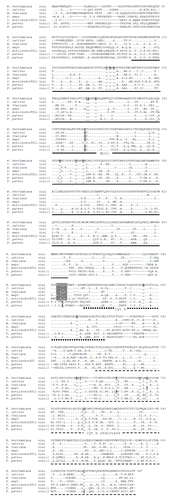
An interesting difference between P. patens and vascular plant NRs is that moss enzymes lack the consensus sequence (K/R)(S/T)XS*(T/S)XP recognized by a 14–3-3 protein during NR activity downregulation (amino acids 544 to 549 in ).Citation29,Citation30 P. patens NRs have Glu in position 547 instead of the Ser which is phosphorylated before union with a 14–3-3 protein in NR from vascular plants. When this Ser residue was changed for an Asp in NR from Nicotiana plumbaginifolia the mutated plant had constitutive NR activity even in the dark and produced more NO than wild type, demonstrating the importance of posttranslational regulation of NR by this mechanism.Citation31,Citation32 On the other hand, NRs from green algae also lack the 14–3-3 recognition sequence.Citation17,Citation20,Citation21 Moreover, it was demonstrated that C. reinhardtii synthesizes a 14–3-3 protein that does not regulate its own NR but can bind and inactivate spinach NR.Citation15 However, red and green algae downregulate NRs activity during light/dark transitions, high nitrate and ammonium levels. Upregulation of NRs activity in Rhodophyta, green algae and higher plants is mainly directed by expression of the nr genes and de novo synthesis of the protein.Citation33,Citation34 The downregulation of NR in red and green algae that lack the 14–3-3 mechanism for degradation could be due to other mechanisms for protein degradation, inhibition by Mg+, phosphorylation or structural modification of the protein.Citation35 However, none of those mechanisms has been proved to operate in green algae. Furthermore, there are no studies on Streptophytes NRs, the clade of green algae considered the sister group of embryophytes,Citation36 leaving a gap of information about its mechanisms for NR posttranslational regulation.
The results presented here are startling because they suggest that the known mechanism that regulates NRs activity in vascular plants did not appear when the embryophytes emerged. Due to the novelty of this finding it is necessary to start experimental work to exhaustively analyze the P. patenes NRs regulation at the transcriptional and posttranslational level to unravel the mechanisms that downregulate NR activity in mosses and green algae.
Evolutionary relationships of NRs from land plants. A phylogenetic analysis of NR from Viridiplantae, including green algae, P. patens, S. moellendorffi and angiosperms was performed (). The sequences used to construct the phylogenetic tree are listed on Table 2. Tree topology is consistent with the known algae a plant evolutionary history; embyophytes share a common ancestor with green algae.Citation23,Citation36 Then, at the base of land plants, NRs from P. patens form a first branch that separated before the division of non-seed vascular plants and angiosperms. The position of ppnia1;1 and ppnia2 genes in the P. patens genome (), the high identity (99.8%) of their amino acid sequences and their position in the tree suggest a recent duplication of this gene, as they form a group separated from ppnia1;2. Vascular plants are separated in two branches, one containing non-seed vascular plants, represented by S. moellendorffii, and another that includes all angiosperms. The topology of angiosperm and algae branches in is similar to those reported before.Citation14,Citation16 The result presented here, that summarizes the evolutionary history of Embryophytes NRs will be better defined in the basal branches as more sequences of Bryophytes and Pteridophytes become available.
Figure 3. Phylogenetic tree of algae and land plants NR amino acid sequences. Only full NR sequences were included in this analysis, the GenBank accession numbers used are indicated in Table 2. The evolutionary tree was constructed with the MEGA5 software.Citation40 Bootstrap analysis of 1000 replications was performed on the trees inferred from the neighbor-joining method. Bootstrap values are noted in the nodes, those below 50% are not shown.
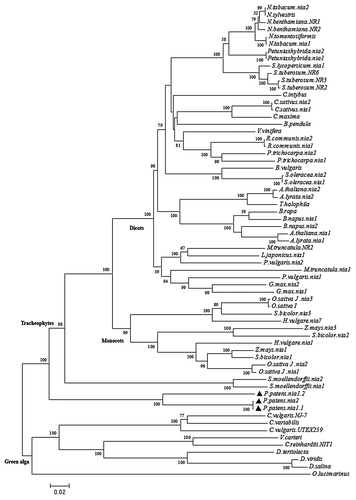
The data presented here provides compelling evidence that during land plant evolution the nr genes have lost introns, from six in mosses to three in angiosperms. Moreover, our analysis shows that the known mechanism to downregulate NRs activity in angiosperms could be common to vascular plants, but not with the most basal land plants, the mosses. To confirm this it will be necessary to analyze the NR sequences from other bryophytes that unfortunately, are not available at the moment. Furthermore, this work opens the door for future detailed research on the mechanisms that regulate P. patens NRs activity that may be common to green algae. Finally, we addressed interesting questions on Viridiplantae NR evolution that deserve to be investigated.
Methods
Search for NR genes. The protein sequence of Nicotiana benthamiana NR1 (GenBank accession number BAE46746.1) was used as probe to run a BLASTPCitation37 search to find putative nr genes in the P. patens genome database (www.cosmoss.org). The accession numbers of the retrieved sequences were used to get the complete gene sequences. The position in the genome and the structure of the genes was analyzed.
Analysis of NR protein sequences. Deduced amino acid sequences from the three P. patens NRs were aligned using the ClustalX2 softwareCitation38 and the percentage of similarity and identity between the sequences were determined using the MatGAT2.01 software.Citation39
To compare the protein sequence of P. Patens NRs with other plant NRs, a BLASTP 37 search was done using the PpNia1;1 protein sequence (Accession number BAE19754.1) in the GenBank database (www.ncbi.nlm.nih.gov). Some of the retrieved sequences, four from angiosperms and one from a Lycophyte were aligned with PpNia1;1 and PpNia1;2 using the ClustalW software.Citation38 An evolutionary tree of algae and land plants NRs amino acid sequences was constructed using the MEGA5 software.Citation40 A bootstrap analysis with 1000 replications was carried on the trees inferred from the neighbor-joining method.Citation41
Abbreviations: | ||
Cyt b | = | cytochrome b |
MoCo | = | molybdenum cofactor |
NO | = | nitric oxide |
NR | = | nitrate reductase |
Disclosure of Potential Conflicts of Interest
No potential conflicts of interest were disclosed.
Acknowledgments
Authors are grateful to an anonymous reviewer and editor in chief of CIB for they enlighten comments that help us to improve this work. Also we want to thank to Dr. Jorge Luis Folch Mallol and Dr. Ernesto Ortiz Suri for they helpful comments to this manuscript.
References
- Campbell WH. Nitrate reductase structure, function and regulation: bridging the gap between biochemistry and physiology. Annu Rev Plant Physiol Plant Mol Biol 1999; 50:277 - 303; http://dx.doi.org/10.1146/annurev.arplant.50.1.277; PMID: 15012211
- Campbell WH, Kinghorn KR. Functional domains of assimilatory nitrate reductases and nitrite reductases. Trends Biochem Sci 1990; 15:315 - 9; http://dx.doi.org/10.1016/0968-0004(90)90021-3; PMID: 2204158
- Fischer K, Barbier GG, Hecht H-J, Mendel RR, Campbell WH, Schwarz G. Structural basis of eukaryotic nitrate reduction: crystal structures of the nitrate reductase active site. Plant Cell 2005; 17:1167 - 79; http://dx.doi.org/10.1105/tpc.104.029694; PMID: 15772287
- Lu G, Campbell WH, Schneider G, Lindqvist Y. Crystal structure of the FAD-containing fragment of corn nitrate reductase at 2.5 A resolution: relationship to other flavoprotein reductases. Structure 1994; 2:809 - 21; http://dx.doi.org/10.1016/S0969-2126(94)00082-4; PMID: 7812715
- Dean JV, Harper JE. The conversion of nitrite to nitrogen oxide(s) by the constitutive NAD(P)H-Nitrate reductase enzyme from soybean. Plant Physiol 1988; 88:389 - 95; http://dx.doi.org/10.1104/pp.88.2.389; PMID: 16666314
- Yamasaki H, Sakihama Y, Takahashi S. An alternative pathway for nitric oxide production in plants: new features of an old enzyme. Trends Plant Sci 1999; 4:128 - 9; http://dx.doi.org/10.1016/S1360-1385(99)01393-X; PMID: 10322545
- Garcia-Mata C, Gay R, Sokolovski S, Hills A, Lamattina L, Blatt MR. Nitric oxide regulates K+ and Cl- channels in guard cells through a subset of abscisic acid-evoked signaling pathways. Proc Natl Acad Sci U S A 2003; 100:11116 - 21; http://dx.doi.org/10.1073/pnas.1434381100; PMID: 12949257
- Kolbert Z, Bartha B, Erdei L. Exogenous auxin-induced NO synthesis is nitrate reductase-associated in Arabidopsis thaliana root primordia. J Plant Physiol 2008; 165:967 - 75; http://dx.doi.org/10.1016/j.jplph.2007.07.019; PMID: 17936409
- Seligman K, Saviani EE, Oliveira HC, Pinto-Maglio CAF, Salgado I. Floral transition and nitric oxide emission during flower development in Arabidopsis thaliana is affected in nitrate reductase-deficient plants. Plant Cell Physiol 2008; 49:1112 - 21; http://dx.doi.org/10.1093/pcp/pcn089; PMID: 18540030
- Sakihama Y, Nakamura S, Yamasaki H. Nitric oxide production mediated by nitrate reductase in the green alga Chlamydomonas reinhardtii: an alternative NO production pathway in photosynthetic organisms. Plant Cell Physiol 2002; 43:290 - 7; http://dx.doi.org/10.1093/pcp/pcf034; PMID: 11917083
- Kaiser WM, Huber SC. Post-translational regulation of nitrate reductase: mechanism, physiological relevance and environmental triggers. J Exp Bot 2001; 52:1981 - 9; http://dx.doi.org/10.1093/jexbot/52.363.1981; PMID: 11559733
- Riens B, Heldt HW. Decrease of nitrate reductase activity in spinach leaves during a light-dark transition. Plant Physiol 1992; 98:573 - 7; http://dx.doi.org/10.1104/pp.98.2.573; PMID: 16668679
- MacKintosh C, Meek SEM. Regulation of plant NR activity by reversible phosphorylation, 14-3-3 proteins and proteolysis. Cell Mol Life Sci 2001; 58:205 - 14; http://dx.doi.org/10.1007/PL00000848; PMID: 11289302
- Stolz JF, Basu P. Evolution of nitrate reductase: molecular and structural variations on a common function. Chembiochem 2002; 3:198 - 206; http://dx.doi.org/10.1002/1439-7633(20020301)3:2/3<198::AID-CBIC198>3.0.CO;2-C; PMID: 11921398
- Pozuelo M, MacKintosh C, Galván A, Fernández E. Cytosolic glutamine synthetase and not nitrate reductase from the green alga Chlamydomonas reinhardtii is phosphorylated and binds 14-3-3 proteins. Planta 2001; 212:264 - 9; http://dx.doi.org/10.1007/s004250000388; PMID: 11216847
- Falcão RF, Oliveira MC, Colepicolo P. Molecular characterization of nitrate reductase gene and its expression in the marine red alga Gracilaria tenuistipitata (Rhodophyta). J Appl Phycol 2010; 22:613 - 22; http://dx.doi.org/10.1007/s10811-010-9501-2
- Song B, Ward BB. Molecular characterization of the assimilatory nitrate reductase gene and its expression in the marine green alga Dunaliella tertiolecta (chlorophyceae). J Phycol 2004; 40:721 - 31; http://dx.doi.org/10.1111/j.1529-8817.2004.03078.x
- Coyne KJ. Nitrate reductase (NR1) sequence and expression in the harmful alga Heterostigma akashiwo (Raphidophyceae). J Phycol 2010; 46:135 - 42; http://dx.doi.org/10.1111/j.1529-8817.2009.00781.x
- Young EB, Dring MJ, Berges JA. Distinct patterns of nitrate reductase activity in brown algae: light and ammonium sensitivity in Laminaria digitata is absent in Fucus species. J Phycol 2007; 43:1200 - 8; http://dx.doi.org/10.1111/j.1529-8817.2007.00403.x
- Dawson HN, Pendleton LC, Solomonson LP, Cannons AC. Cloning and characterization of the nitrate reductase-encoding gene from Chlorella vulgaris: structure and identification of transcription start points and initiator sequences. Gene 1996; 171:139 - 45; http://dx.doi.org/10.1016/0378-1119(96)00063-7; PMID: 8666264
- Gruber H, Goetinck SD, Kirk DL, Schmitt RD. The nitrate reductase-encoding gene of Volvox carteri: map location, sequence and induction kinetics. Gene 1992; 120:75 - 83; http://dx.doi.org/10.1016/0378-1119(92)90011-D; PMID: 1398126
- Howarth DG, Baum DA. Phylogenetic utility of a nuclear intron from nitrate reductase for the study of closely related plant species. Mol Phylogenet Evol 2002; 23:525 - 8; http://dx.doi.org/10.1016/S1055-7903(02)00035-0; PMID: 12099803
- Mishler BD, Oliver MJ. Putting Physcomitrella patens on the Tree of Life: The evolution and ecology of mosses. In: Knight C, Perroud PF, Cove D eds. Annual Plant Reviews Volume 36: The Moss Physcomitrella patens. West Sussex, UK: Wiley-Blackwell, 2009:1-15.
- Shaw J, Renzaglia K. Phylogeny and diversification of bryophytes. Am J Bot 2004; 91:1557 - 81; http://dx.doi.org/10.3732/ajb.91.10.1557; PMID: 21652309
- Cove D, Bezanilla M, Harries P, Quatrano R. Mosses as model systems for the study of metabolism and development. Annu Rev Plant Biol 2006; 57:497 - 520; http://dx.doi.org/10.1146/annurev.arplant.57.032905.105338; PMID: 16669772
- Cove DJ. The moss Physcomitrella patens. Annu Rev Genet 2005; 39:339 - 58; http://dx.doi.org/10.1146/annurev.genet.39.073003.110214; PMID: 16285864
- Rensing SA, Lang D, Zimmer AD, Terry A, Salamov A, Shapiro H, et al. The Physcomitrella genome reveals evolutionary insights into the conquest of land by plants. Science 2008; 319:64 - 9; http://dx.doi.org/10.1126/science.1150646; PMID: 18079367
- Irimia M, Roy SW. Spliceosomal introns as tools for genomic and evolutionary analysis. Nucleic Acids Res 2008; 36:1703 - 12; http://dx.doi.org/10.1093/nar/gkn012; PMID: 18263615
- Bachmann M, Huber JL, Liao P-C, Gage DA, Huber SC. The inhibitor protein of phosphorylated nitrate reductase from spinach (Spinacia oleracea) leaves is a 14-3-3 protein. FEBS Lett 1996; 387:127 - 31; http://dx.doi.org/10.1016/0014-5793(96)00478-4; PMID: 8674533
- Huber SC, Bachmann M, Huber JL. Post-translational regulation of nitrate reductase activity: a role for Ca2+ and 14-3-3 proteins. Trends Plant Sci 1996; 1:432 - 8; http://dx.doi.org/10.1016/S1360-1385(96)10046-7
- Lea US, Ten Hoopen F, Provan F, Kaiser WM, Meyer C, Lillo C. Mutation of the regulatory phosphorylation site of tobacco nitrate reductase results in high nitrite excretion and NO emission from leaf and root tissue. Planta 2004; 219:59 - 65; http://dx.doi.org/10.1007/s00425-004-1209-6; PMID: 14767769
- Lillo C, Lea US, Leydecker M-T, Meyer C. Mutation of the regulatory phosphorylation site of tobacco nitrate reductase results in constitutive activation of the enzyme in vivo and nitrite accumulation. Plant J 2003; 35:566 - 73; http://dx.doi.org/10.1046/j.1365-313X.2003.01828.x; PMID: 12940950
- Balandani T, Aparicio PJ. Regulation of nitrate reductase in Acetabularia mediterranea. J Exp Bot 1992; 43:625 - 31; http://dx.doi.org/10.1093/jxb/43.5.625
- Chow F, Cabral de Oliveira M. Rapid and slow modulation of nitrate reductase acivity in the red macro alga Gracilaria chilensis (Gracilariales, Rhodophyta): influence of different nitrogen sources. J Appl Phycol 2008; 20:775 - 82; http://dx.doi.org/10.1007/s10811-008-9310-z
- Kaiser WM, Spill D. Rapid Modulation of Spinach Leaf Nitrate Reductase by Photosynthesis : II. In Vitro Modulation by ATP and AMP. Plant Physiol 1991; 96:368 - 75; http://dx.doi.org/10.1104/pp.96.2.368; PMID: 16668195
- Becker B, Marin B. Steptophyte algae and the origin of embryophytes. Ann Bot (Lond) 2009; 103:999 - 1004; http://dx.doi.org/10.1093/aob/mcp044
- Altschul SF, Madden TL, Schäffer AA, Zhang J, Zhang Z, Miller W, et al. Gapped BLAST and PSI-BLAST: a new generation of protein database search programs. Nucleic Acids Res 1997; 25:3389 - 402; http://dx.doi.org/10.1093/nar/25.17.3389; PMID: 9254694
- Thompson JD, Gibson TJ, Plewniak F, Jeanmougin F, Higgins DG. The CLUSTAL_X windows interface: flexible strategies for multiple sequence alignment aided by quality analysis tools. Nucleic Acids Res 1997; 25:4876 - 82; http://dx.doi.org/10.1093/nar/25.24.4876; PMID: 9396791
- Campanella JJ, Bitincka L, Smalley J. MatGAT: an application that generates similarity/identity matrices using protein or DNA sequences. BMC Bioinformatics 2003; 4:29; http://dx.doi.org/10.1186/1471-2105-4-29; PMID: 12854978
- Tamura K, Peterson D, Peterson N, Stecher G, Nei M, Kumar S. MEGA5: molecular evolutionary genetics analysis using maximum likelihood, evolutionary distance, and maximum parsimony methods. Mol Biol Evol 2011; 28:2731 - 9; http://dx.doi.org/10.1093/molbev/msr121; PMID: 21546353
- Saitou N, Nei M. The neighbor-joining method: a new method for reconstructing phylogenetic trees. Mol Biol Evol 1987; 4:406 - 25; PMID: 3447015