Abstract
The nuclear pore complex (NPC) is one of the largest protein machines in the cell and forms the sole conduit for nucleocytoplasmic transport in eukaryotes. The NPC is composed of an eightfold radially symmetric scaffold of architectural proteins that anchor a set of phenylalanine-glycine (FG) repeat proteins that form the transport barrier. As a step toward elucidating the molecular architecture of the NPC, we solved the structure of nucleoporin 85 (Nup85) in complex with Seh1, a module in the heptameric Nup84 subcomplex. We define a new tripartite protein element, the ancestral coatomer element ACE1, which Nup85 specifically shares with several other nucleoporins and vesicle coat proteins. We predicted and verified functional sites on nucleoporin ACE1 members based on analogy to ACE1 interactions that propagate the COPII vesicle coat. Thus, we provide the first experimental evidence for evolution of the NPC and vesicle coats from a common ancestor. We propose that the NPC structural scaffold, like vesicle coats, is a polygonal network composed of vertex and edge elements that forms a molecular lattice upon which additional nucleoporins assemble. Here we further discuss our findings and elaborate on our lattice model of the nuclear pore complex.
All nucleocytoplasmic transport in a cell proceeds through nuclear pore complexes (NPCs). NPCs are composed of an eight-fold radially symmetric structural scaffold that anchors a group of FG-repeat-containing proteins that form the transport barrier.Citation1–Citation3 Elucidating the three dimensional structure of the NPC is critical for understanding its roles in nucleocytoplasmic transport and cellular homeostasis. A path towards an atomic resolution structure of the 40–60 MDa NPC is made possible by the realization that the NPC is a modular assembly.Citation4 The NPC is composed of ∼30 proteins (Nups) that are arranged into distinct subcomplexes, each present in multiple copies.Citation5 The structural scaffold contains the most stably attached nups and comprises two subcomplexes in yeast: the heptameric Nup84 subcomplex (composed of Nup133, Nup84, Nup145C, Sec13, Nup85, Seh1 and Nup120) and the heteromeric Nic96 subcomplex (likely composed of Nic96, Nup192, Nup188, Nup157/170, Nup59 and Nup53).Citation6
In an attempt to better understand the architecture of the NPC, we solved the crystal structure of Nup85 in complex with Seh1.Citation7 Nup85 interacts with Seh1 via insertion of an N-terminal blade into the open six-bladed β-propeller of Seh1. The remainder of Nup85 forms a uniquely arrayed J-shaped α-helical block with two distinct units we term “crown” and “trunk”. The fold is notably different from the regular α-helical solenoid that was predicted.Citation8,Citation9
We found that this fold is shared in four other proteins of known structure: the nucleoporins Nup145C, Nup84 and Nic96 and the COPII vesicle coatomer Sec31.Citation10–Citation13 While a related architecture between the NPC and vesicle coats has been proposed based on similar fold composition,Citation8 we provide the first experimental and structural evidence of a common ancestry.Citation7 Comparison of the structures shows a shared core composed of three modules: the crown, trunk and C-terminal tail. We termed this tripartite fold the ancestral coatomer element 1 (ACE1). While the overall organization and topology is identical, there are significant differences in the relative orientation of the modules between members. This suggests the boundaries between ACE1 modules may function as hinges. This relationship was not previously predicted due to low sequence conservation and was initially obscured at the structural level by differences in relative orientation of the modules.Citation12
We used characterized interactions of ACE1 proteins to predict functional sites on other members. First, in COPII vesicle coats, Sec31·Sec13 dimers form edge elements through Sec31 crown·crown homodimerization ().Citation11 We predicted and demonstrated that Nup145C likewise interacts crown·crown with its binding partner in the Nup84 complex, Nup84. Second, we predicted and verified that Nup85 and Nup145C tails interact with Nup120 as the Nup84 tail module interacts with Nup133.Citation10 These and other data were used to construct an improved model of the Nup84 subcomplex ().
We propose that the nuclear pore complex scaffold has a lattice structure assembled from vertex and edge elements similar in principle to vesicle coats. We can envision at least two alternative models for this lattice. In the first (), two rings of the Nup84 subcomplex sandwich an inner ring of the Nic96 subcomplex. This arrangement would generate a scaffold of ∼50–80 nm diameter, consistent with the observed pore size in yeast.Citation14,Citation15 Alternatively (), the scaffold may consist of two stacked rings of the Nup84 subcomplex without an intervening Nic96 subcomplex ring, which may be sufficient to traverse the ∼30–50 nm pore height. Uncertainty about the exact arrangement arises from still incompletely understood stoichiometries of components and limited information about overall NPC size. Future clarification of the connectivity between scaffold subcomplexes will additionally help to discern the possible lattice arrangements. Homology to COPII coatomers suggests ACE1-containing subcomplexes will be edge elements in the NPC lattice. The nature of the vertex elements in the NPC is less clear, though it may well also involve β-propeller-β-propeller interactions.
Our lattice model of the NPC prompts a number of additional potential parallels to vesicle coats. First, vesicle coatomers do not directly contact membranes, but use adapter protein complexes to span the ∼8 nm gap and recruit cargo.Citation16 Consistently, a ∼8 nm gap has been observed between the structural scaffold of the NPC and the nuclear membrane.Citation17 Conceivably, other nups fill corresponding adapter complex roles by linking the lattice to transmembrane nups, or transmembrane nups could act directly as adapters. Second, COPII vesicle coats size flexibility is made possible largely by hinges at coat vertices.Citation18 It may be that analogous hinges as well as those between ACE1 modules in the NPC lattice confer plasticity that may be used for pore dilation or NPC (dis)assembly.
Recent work has produced two conflicting models of the molecular organization of the NPC. A computationally generated model that integrates a wealth of localization, interaction and other primary data similarly places the Nup84 subcomplex in two peripheral NPC rings flanking an inner ring composed of the Nic96 subcomplex.Citation19,Citation20 In contrast, a model based on crystal packing interactions in Nup145C·Sec13 places the Nup84 subcomplex in four stacked rings organized by hetero-octameric poles of Nup145C·Sec13 and Nup85·Seh1 units.Citation12 A tube of 32 Nup84 subcomplexes was proposed to envelope inner cylinders of the Nic96 subcomplex and FG Nups generating a “concentric cylinder” model of the NPC.Citation12,Citation21
Our model is incompatible with the “concentric cylinder” model for the NPC. Specifically, the demonstrated crown·crown interaction between Nup84 and Nup145C overlaps with Nup145C crystal contacts necessary for the propagation of the models hetero-octameric poles. Exposed hydrophobic surfaces tend to form crystal-packing contacts; whether or not they are physiologically relevant needs to be addressed by additional experiments.Citation22 Careful analysis of packing interactions in crystals can unveil biologically important protein interfaces, especially if the crystallized proteins are part of a higher-order assembly in vivo, as nups are. Crystal contacts with at least some hydrophobic character are also observed in the structures of Nup85·Seh1, Nup107·Nup133 and Nic96,Citation7,Citation10,Citation13,Citation23 which are all fragments of larger assemblies. While some of these interactions can likely be ruled out as crystal artifacts, i.e., because they involve surfaces created by the use of truncated proteins that would otherwise be buried in the hydrophobic core of the protein, others may be indicative of real functional sites. Weak interactions observed in crystals may point to inter-subcomplex contact areas that are important for self-assembly and to date have not been observed in solution.
We have provided the first structural evidence of a common ancestry of vesicle coats and the nuclear pore complex and provide a lattice model of the NPC based on this commonality. Our lattice model is generally consistent with the computational model of the NPC,Citation20 though the absence of additional structural knowledge precludes a detailed comparison. Our model provides a framework upon which further structural and cell biological studies can be placed in an effort to more fully understand the assembly principles and function of the NPC.
Figures and Tables
Figure 1 A lattice model of the NPC. Sec31, Nup85, Nup145C, Nup84 and Nic96 ACE1 proteins are colored with crowns blue, trunks orange and tail modules green. Other protein folds are shown in grey. (A) Schematic organization of the COPII outer vesicle coat. On the left, an edge element consisting of two Sec31·Sec13 heterodimers is shown. Two Sec31 molecules interact crown·crown. On the right, an entire COPII cuboctahedron coat composed of 24 edge elements is shown unwrapped and laid flat.Citation11 Vertex elements are formed where two Sec31 and two Sec13 β-propellers interact. (B) Alternative organizations of the NPC lattice. On the left, the Nup84 subcomplex is shown in schematic fashion illustrating how ACE1 interactions organize the Y-shaped structure. Nup145C and Nup84 also interact crown·crown. On the right, the entire NPC structural scaffold is shown unwrapped and laid flat. Two rings of the Nup84 subcomplex form the lattice of the NPC scaffold either with an intervening ring of the Nic96 subcomplex (i) or alone (ii). Both the identity and organization of the vertex elements and the Nic96 subcomplex in the pore lattice are unknown and are shown half-transparent. The presented organization is not meant to predict relative positions of proteins or the structure per se, but rather emphasizes the principally similar lattice organization of NPCs and vesicle coats.
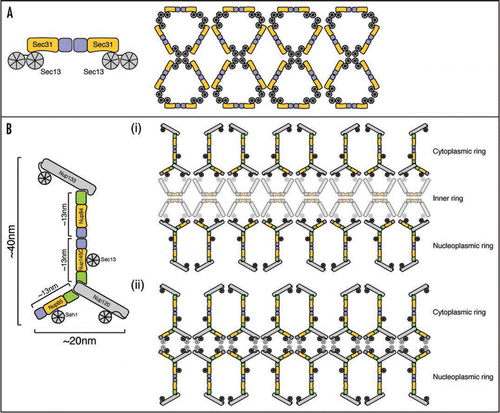
Acknowledgements
This work was supported by NIH Grant GM77537 (Thomas U. Schwartz), a Pew Scholar Award (Thomas U. Schwartz), a Koch Fellowship Award (Stephen G. Brohawn) and a Vertex Scholarship (Stephen G. Brohawn).
Addendum to:
References
- D'Angelo MA, Hetzer MW. Structure, dynamics and function of nuclear pore complexes. Trends Cell Biol 2008; 18:456 - 466
- Tran EJ, Wente SR. Dynamic nuclear pore complexes: life on the edge. Cell 2006; 125:1041 - 1053
- Weis K. Regulating access to the genome: nucleocytoplasmic transport throughout the cell cycle. Cell 2003; 112:441 - 451
- Schwartz TU. Modularity within the architecture of the nuclear pore complex. Curr Opin Struct Biol 2005; 15:221 - 226
- Rout MP, Aitchison JD, Suprapto A, Hjertaas K, Zhao Y, Chait BT. The yeast nuclear pore complex: composition, architecture and transport mechanism. J Cell Biol 2000; 148:635 - 651
- Rabut G, Doye V, Ellenberg J. Mapping the dynamic organization of the nuclear pore complex inside single living cells. Nat Cell Biol 2004; 6:1114 - 1121
- Brohawn SG, Leksa NC, Spear ED, Rajashankar KR, Schwartz TU. Structural evidence for common ancestry of the nuclear pore complex and vesicle coats. Science 2008; 322:1369 - 1373
- Devos D, Dokudovskaya S, Alber F, Williams R, Chait BT, Sali A, et al. Components of coated vesicles and nuclear pore complexes share a common molecular architecture. PLoS Biol 2004; 2:380
- Devos D, Dokudovskaya S, Williams R, Alber F, Eswar N, Chait BT, et al. Simple fold composition and modular architecture of the nuclear pore complex. Proc Natl Acad Sci USA 2006; 103:2172 - 2177
- Boehmer T, Jeudy S, Berke IC, Schwartz TU. Structural and functional studies of Nup107/Nup133 interaction and its implications for the architecture of the nuclear pore complex. Mol Cell 2008; 30:721 - 731
- Fath S, Mancias JD, Bi X, Goldberg J. Structure and organization of coat proteins in the COPII cage. Cell 2007; 129:1325 - 1336
- Hsia KC, Stavropoulos P, Blobel G, Hoelz A. Architecture of a coat for the nuclear pore membrane. Cell 2007; 131:1313 - 1326
- Jeudy S, Schwartz TU. Crystal structure of nucleoporin Nic96 reveals a novel, intricate helical domain architecture. J Biol Chem 2007; 282:34904 - 34912
- Kiseleva E, Allen TD, Rutherford S, Bucci M, Wente SR, Goldberg MW. Yeast nuclear pore complexes have a cytoplasmic ring and internal filaments. J Struct Biol 2004; 145:272 - 288
- Yang Q, Rout MP, Akey CW. Three-dimensional architecture of the isolated yeast nuclear pore complex: functional and evolutionary implications. Mol Cell 1998; 1:223 - 234
- Owen DJ, Collins BM, Evans PR. Adaptors for clathrin coats: structure and function. Annu Rev Cell Dev Biol 2004; 20:153 - 191
- Beck M, Lucic V, Förster F, Baumeister W, Medalia O. Snapshots of nuclear pore complexes in action captured by cryo-electron tomography. Nature 2007; 449:611 - 615
- Stagg SM, LaPointe P, Razvi A, Gürkan C, Potter CS, Carragher B, et al. Structural basis for cargo regulation of COPII coat assembly. Cell 2008; 134:474 - 484
- Alber F, Dokudovskaya S, Veenhoff LM, Zhang W, Kipper J, Devos D, et al. Determining the architectures of macromolecular assemblies. Nature 2007; 450:683 - 694
- Alber F, Dokudovskaya S, Veenhoff LM, Zhang W, Kipper J, Devos D, et al. The molecular architecture of the nuclear pore complex. Nature 2007; 450:695 - 701
- Debler EW, Ma Y, Seo HS, Hsia KC, Noriega TR, Blobel G, et al. A fence-like coat for the nuclear pore membrane. Mol Cell 2008; 32:815 - 826
- Kobe B, Guncar G, Buchholz R, Huber T, Maco B, Cowieson N, et al. Crystallography and protein-protein interactions: biological interfaces and crystal contacts. Biochem Soc Trans 2008; 36:1438 - 1441
- Schrader N, Stelter P, Flemming D, Kunze R, Hurt E, Vetter IR. Structural basis of the nic96 subcomplex organization in the nuclear pore channel. Mol Cell 2008; 29:46 - 55