Abstract
Cells respond and adapt to various extracellular changes. Environmental stresses, such as high osmolarity and acute glucose deprivation, rapidly and transiently shut down translation initiation and actin polarization in the yeast Saccharomyces cerevisiae. Certain clinical drugs, such as local anesthetics and antipsychotic phenothiazines, and cationic surfactants also cause shutdowns similar to those triggered by environmental stresses. These compounds all have an amphiphilic structure, a cationic hydrophilic region, surfactant activity, and the ability to lyse yeast cells. Since low concentrations of these compounds shut down intracellular reactions in the absence of cell lysis, the compounds might change the state of the cell’s membrane by intercalating into the membrane and thus generate signals for the shutdown, as do environmental stresses. The intracellular shutdowns caused by stresses might essentially be the same as the paralysis caused by clinical drugs at the cellular level.
Environmental Stresses Shut Down Intracellular Reactions
Translation is governed by the interaction between a number of different structural elements of mRNAs and the translation machinery. Environmental stresses, such as high osmolarity,Citation1 glucose deprivation,Citation2 amino acids starvation,Citation3 oxidative stressCitation4 and nitrogen starvationCitation5 rapidly shut down bulk protein synthesis at the initiation level in the yeast Saccharomyces cerevisiae. The actin cytoskeleton in the yeast provides the structural basis for cell polarity by forming cortical patches and cables during the budding phase of the cell cycle.Citation6 Mild heat shock rapidly and transiently depolarizes the actin cytoskeleton without resulting in the shutdown of translation initiation, whereas amino acids starvation shuts down translation initiation without causing the rapid depolarization of actin.Citation7,Citation8 High osmolarity and glucose deprivation transiently and simultaneously shut down translation initiation and actin polarization.Citation1,Citation8,Citation9
Many mRNAs and RNA-binding proteins are reported to localize to specific compartments, along with cytoskeletal elements, in a variety of eukaryotic cells.Citation10,Citation11 In S. cerevisiae, numerous mRNAs are localized to produce proteins in specific compartments, such as the daughter cell or the mitochondrion.Citation12–Citation15 These observations suggest that individual mRNAs may consistently be transported to specific areas in the yeast cell. Thus the biological significance of transient shutdown in response to stresses is considered to be the following ().Citation8 When cells are exposed to certain stresses, the cells immediately suspend both the transportation of mRNAs to specific compartments, by the loss of actin polarization, and the production of proteins by the shutdown of translation initiation. During the shutdown, the transcriptional profile of mRNAs is dramatically changed in response to the stress.Citation16–Citation18 The shutdown of translation at the initiation but not the elongation step would increase the proportion of mRNAs that are not protected by the ribosome. This would facilitate, via mRNA decay pathways, the rapid degradation of mRNAs that are unnecessary for the stressed condition.Citation19 Consequently, the cell can easily adapt the intracellular pattern of mRNAs, to meet the demands of the stressed condition, by altering in transcriptional regulation and/or mRNA stability during the shutdown. Subsequently, some mRNAs are relocated to specific areas, where their translation products are required for the stressed condition, by repolarization of the actin cytoskeleton. Simultaneously, the cell can produce the proteins encoded by the mRNAs by resuming the translation process.Citation20,Citation21 Therefore, it seems likely that the dramatic changes in both the actin cytoskeleton and protein synthesis, form part of a system that facilitates the spatial rearrangement of gene expression in response to environmental stress.
Transient shutdowns in response to stresses are divided into two reactions. One is the rapid shutdown, which is indirectly regulated by Reg1 and A-kinase (in the case of glucose deprivation),Citation2,Citation8 and the other is the slow adaptation to shutdowns via the Msn2/4 transcription factors, the Snf1 kinase (in the case of glucose deprivation),Citation8 or the Hog1 MAP kinase (in the case of high osmolarity).Citation1 However, the precise mechanism underlying the rapid shutdown of both translation initiation and actin polarization has yet to be determined.
Common Features of the Drugs that Elicit Intracellular Shutdown
Recently, we demonstrated that, similar to environmental stress, local anesthetics and antipsychotic phenothiazines also cause the rapid shutdown of translation initiation and actin polarization, in addition to the rapid nuclear localization of the Msn2 transcription factor in yeast.Citation22 Local anesthetics are amphiphilic molecules consisting of a hydrophilic tertiary amine that is joined to a hydrophobic aromatic ring by an ester or an amide linkage,Citation24 and these drugs interrupt nerve transmission in the central nervous system (CNS) by blocking sodium conductance.Citation23 Phenothiazine tranquilizers elicit a variety of neuroleptic effects, and are widely used as antipsychotic drugs. For example, chlorpromazine (CPZ), one of the best-known phenothiazines, exerts its antipsychotic effects by blocking dopamine receptors.Citation25 Phenothiazine compounds also have an amphiphilic structure; the tricyclic ring structure is hydrophobic, while the tertiary propylamine tail is hydrophilic.Citation24 Growth of budding yeast is inhibited by the administration of tetracaine (TC), a local anesthetic, and the genes involved in the sensitivity to TC have been identified.Citation26,Citation27 The effects of phenothiazines on ion transport in yeast have also been investigated.Citation28 These reports suggest that yeast is a useful experimental system to track the direct cellular effects of the clinical drugs. Similarly, surfactants are also examples of amphiphilic compounds, with a great variety of structures in both the hydrophilic head and the hydrophobic tail, and are categorized into four groups (namely, the cationic, anionic, nonionic and zwitterionic group), based on the charge associated with the hydrophilic head. The structures of representative amphiphilic compounds, local anesthetics (e.g., TC), phenothiazines (e.g., CPZ), cationic surfactants (e.g., benzethonium chloride, BC), and anionic surfactants (e.g., sodium dodecyl sulfate, SDS), which elicit intracellular shutdowns, are shown in .
Local anesthetics and phenothiazines have surfactant activities, and cationic surfactants show the shutdown activities that are similar to, and more potent than, those induced by the clinical drugs, indicating that the chemical features required for shutdown are amphiphilicity and surfactant activity.Citation22 However, surfactant activity is not sufficient for shutdown, because even high concentrations of CHAPS (3-[(3-Cholamidopropyl) dimethylammonio]1-propanesulfonate), a zwitterionic surfactant, do not depolarize the actin cytoskeleton, and SDS depolarizes actin, but does not inhibit translation initiation.Citation22 The quaternary ammonium base in the cationic surfactants (e.g., BC), and the tertiary amine in both local anesthetics and phenothiazines are positively charged under physiological conditions.Citation24,Citation29 Thus a cationic charge in the hydrophilic region might also be important, in addition to surfactant activity, for inducing all the shutdown reactions.
TC has been reported to associate with segment 6 in domain IV of the voltage-dependent sodium channelCitation30 and CPZ blocks GABA,Citation31 nicotinic ACh,Citation32 and dopamine receptors in mammalian cells.Citation25,Citation33 However, these proteins are not present in yeast, and are thus not likely to be the target of the local anesthetics. While the biological effects of the local anesthetics, phenothiazines and cationic surfactants are similar in yeast, these compounds have distinct molecular structures (). Hence, it is unlikely that these compounds bind to a common target protein or to similar regions of the target proteins, if present, in yeast. Phospholipids are also amphiphilic compounds, forming the lipid bilayer of cell membranes. Amphiphilic molecules, such as surfactants, are thought to interact with the membrane by intercalating into the lipid bilayer, altering the membrane's properties, and eventually disrupting the cell's membranes.Citation24 Local anesthetics and phenothiazines also have surfactant properties, such as an intrinsic critical micellar concentration,Citation24,Citation34 surfactant activity, and the ability to lyse yeast cells at high concentrations.Citation22 These observations indicate that these clinical drugs are a type of surfactants, and thus suggest that the lipid bilayer is the primary target of the clinical drugs in the shutdown response. Low concentrations of the clinical drugs and cationic surfactants shut down the intracellular reactions without cell lysis, suggesting that these compounds might change the state of the cell membrane by intercalating into the membrane.
The Correlation between Stresses and Drugs on Intracellular Shutdown
Mild heat stress transiently depolarizes the actin cytoskeleton without affecting translation initiation,Citation1,Citation7 as seen in cells treated with a low concentration of SDS.Citation22 In contrast, high osmolarity and glucose deprivation inhibit actin polarization and translation initiation,Citation1,Citation2,Citation7,Citation8 as do low concentrations of TC, CPZ and BC.Citation22 Morphological studies using erythrocytes suggest that anionic amphiphiles intercalate into the outer leaflet of the lipid bilayer (), while cationic amphiphiles preferentially intercalate into the inner leaflet ().Citation35,Citation36 These observations suggest that a model where heat stress perturbs the outer leaflet, in a manner similar to an anionic surfactant (), whereas high osmolarity or glucose deprivation perturbs the inner or both leaflets of the membrane, in a manner similar to cationic surfactants and clinical drugs (). Cell membranes perturbed by environmental stresses can usually recover, but those treated with a high concentration of drugs will eventually be disrupted. During perturbation of the outer leaflet of the plasma membrane, signals required for the shutdown of actin polarization and for the cytoplasmic retention of Msn2 might be generated (, shutdown). Perturbation of the inner or both leaflets might generate a signal to shut down translation initiation, in addition to the signal that controls actin polarization and Msn2 localization (, shutdown). This model is consistent with the observations that TC, CPZ and BC act on a pathway distinct from heat stress, but partially overlapping with osmotic stress, whereas SDS acts on a pathway distinct from osmotic stress, but partially overlapping with heat stress.Citation22
Outlook
Inhibition of bulk protein synthesis or actin polarization, but not a change of gene expression, might directly reflect a shutdown of cellular activity, as occurs during cellular paralysis. Trifluoperazine and chlorpromazine, representative phenothiazines, are known to inhibit translation in both mammalian cells and budding yeast cells,Citation37,Citation38 indicating that the intracellular shutdown caused by clinical drugs is a conserved mechanism. Thus the shutdowns caused by environmental stresses may, at the cellular level, essentially be the same as the paralysis induced by clinical drugs. To test this hypothesis, details of the drug-induced and stress-induced intracellular shutdown should be investigated in mammalian cells. High concentrations of local anesthetics are required to produce shutdown effects similar to those produced by phenothiazines or cationic surfactants in yeast, providing an explanation for the unique ability of local anesthetics to paralyze only the area surrounding the injection site. Therefore, cationic surfactants, which produce effect at lower concentrations than the phenothiazines, may have an antipsychotic effect on the nervous system rather than a local anesthetic effect, although neither anesthetic nor antipsychotic effects have been confirmed for the cationic surfactants that we examined. Even if the cationic surfactants do not produce such effects, membrane permeabilization is likely to be a common effect of local anesthetics, phenothiazines and cationic surfactants, and thus it might contribute to the toxicity or side effects caused by the excessive administration of these drugs, as reported previously for local anesthetics.Citation34 The yeast system may be useful for elucidating correlations between drug structure and toxicity at the cellular level.
Figures and Tables
Figure 1 Transient shutdown in response to environmental stresses. The intracellular reaction in budding yeast upon exposure to stress, such as high osmolarity or glucose deprivation, is separated into three steps: the normal situation before exposure to stress, the shutdown phase just after exposure to stress, and the adaptation phase in the continued presence of the stress.
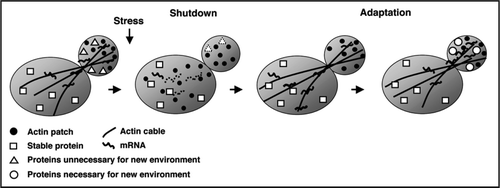
Figure 2 The structures of compounds eliciting intracellular shutdowns. The structures of a local anesthetic, a phenothiazine, a cationic surfactant, and an anionic surfactant are shown. TC, CPZ, BC and SDS indicate tetracaine, chlorpromazine, benzethonium chloride, sodium dodecyl sulfate, respectively.
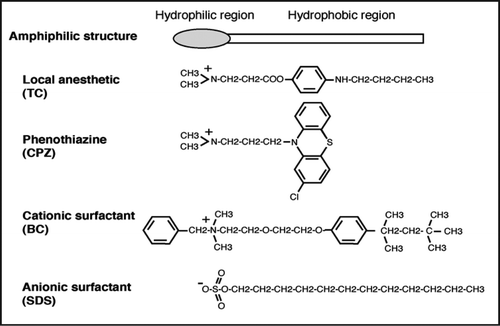
Figure 3 A model for shutdown via membrane perturbation induced by drug or environmental stress. (A) The outer leaflet of the plasma membrane is perturbed by low concentrations of anionic surfactants, but is disrupted at high concentrations, whereas the leaflet is transiently perturbed by mild heat stress. During either perturbation, a putative signal for shutdown of both actin polarization and cytoplasmic retention of Msn2 is generated. (B) Inner or both leaflets of the membrane are perturbed by low concentrations of cationic surfactants, local anesthetics or phenothiazines, but are disrupted by high concentrations, whereas the leaflets are transiently perturbed by osmotic stress or glucose deprivation. These perturbations generate a signal for shutdown of translation initiation and actin polarization, and bring about the rapid nuclear localization of Msn2.
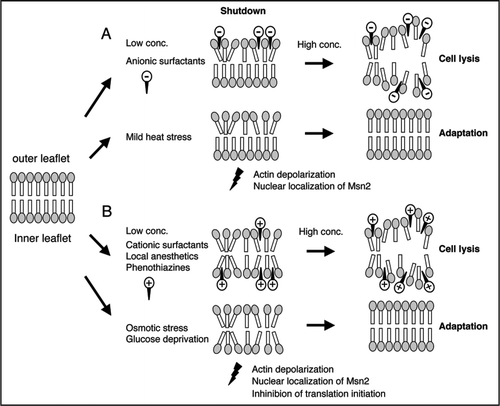
Acknowledgements
I would like to thank Tomoyuki Araki and Akio Toh-e for their useful suggestions. The author's work was supported by the Japan Society for the Promotion of Science.
References
- Uesono Y, Toh-e A. Transient inhibition of translation initiation by osmotic stress. J Biol Chem 2002; 277:13848 - 13855
- Ashe MP, De Long SK, Sachs AB. Glucose depletion rapidly inhibits translation initiation in yeast. Mol Biol Cell 2000; 11:833 - 848
- Hinnebusch AG. Translational regulation of GCN4 and the general amino acid control of yeast. Annu Rev Microbiol 2005; 59:407 - 450
- Shenton D, Smirnova JB, Selley JN, Carroll K, Hubbard SJ, Pavitt GD, et al. Global translational responses to oxidative stress impact upon multiple levels of protein synthesis. J Biol Chem 2006; 281:29011 - 29021
- Ibrahimo S, Holmes LE, Ashe MP. Regulation of translation initiation by the yeast eIF4E binding proteins is required for the pseudohyphal response. Yeast 2006; 23:1075 - 1088
- Botstein D, Amberg D, Mulholland J, Huffaker T, Adams A, Drubin D, et al. Pringle JR, Broach JR, Jones EW. The yeast cytoskeleton. The Molecular and Cellular Biology of the yeast Saccharomyces. Cell Cycle and Cell Biology 3 1997; Cold Spring Harbor, NY Cold Spring Harbor Laboratory Press 1 - 45
- Delley PA, Hall MN. Cell wall stress depolarizes cell growth via hyperactivation of RHO1. J Cell Biol 1999; 147:163 - 174
- Uesono Y, Ashe MP, Toh-e A. Simultaneous yet independent regulation of actin cytoskeletal organization and translation initiation by glucose in Saccharomyces cerevisiae. Mol Biol Cell 2004; 15:1544 - 1556
- Chowdhury S, Smith KW, Gustin MC. Osmotic stress and the yeast cytoskeleton: phenotype-specific suppression of an actin mutation. J Cell Biol 1992; 118:561 - 571
- Jansen RP. mRNA localization: message on the move. Nat Rev Mol Cell Biol 2001; 2:247 - 256
- Kloc M, Zearfoss NR, Etkin LD. Mechanisms of subcellular mRNA localization. Cell 2002; 108:533 - 544
- Corral-Debrinski M, Blugeon C, Jacq C. In yeast, the 3′ untranslated region or the presequence of ATM1 is required for the exclusive localization of its mRNA to the vicinity of mitochondria. Mol Cell Biol 2000; 20:7881 - 7892
- Takizawa PA, DeRisi JL, Wilhelm JE, Vale RD. Plasma membrane compartmentalization in yeast by messenger RNA transport and a septin diffusion barrier. Science 2000; 290:341 - 344
- Shepard KA, Gerber AP, Jambhekar A, Takizawa PA, Brown PO, Herschlag D, et al. Widespread cytoplasmic mRNA transport in yeast: identification of 22 bud-localized transcripts using DNA microarray analysis. Proc Natl Acad Sci USA 2003; 100:11429 - 11434
- Paquin N, Chartrand P. Local regulation of mRNA translation: new insights from the bud. Trends Cell Biol 2008; 18:105 - 111
- Gancedo JM. Yeast carbon catabolite repression. Microbiol Mol Biol Rev 1998; 62:334 - 361
- Causton HC, Ren B, Koh SS, Harbison CT, Kanin E, Jennings EG, et al. Remodeling of yeast genome expression in response to environmental changes. Mol Biol Cell 2001; 12:323 - 337
- Gat-Viks I, Shamir R. Refinement and expansion of signaling pathways: the osmotic response network in yeast. Genome Res 2007; 17:358 - 367
- Sheth U, Parker R. Decapping and decay of messenger RNA occur in cytoplasmic processing bodies. Science 2003; 300:805 - 808
- Blomberg A. Global changes in protein synthesis during adaptation of the yeast Saccharomyces cerevisiae to 0.7 M NaCl. J Bacteriol 1995; 177:3563 - 3572
- Norbeck J, Blomberg A. Protein expression during exponential growth in 0.7 M NaCl medium of Saccharomyces cerevisiae. FEMS Microbiol Lett 1996; 137:1 - 8
- Uesono Y, Araki T, Toh-E A. Local anesthetics, antipsychotic phenothiazines and cationic surfactants shut down intracellular reactions through membrane perturbation in yeast. Biosci Biotechnol Biochem 2008; 72:2884 - 2894
- Butterworth JF, Strichartz GR. Molecular mechanisms of local anesthesia. Anesthesiology 1990; 72:711 - 734
- Schreier S, Malheiros SV, de Paula E. Surface active drugs: self-association and interaction with membranes and surfactants: physicochemical and biological aspects. Biochim Biophys Acta 2000; 1508:210 - 234
- Seeman P. Brain dopamine receptors. Pharmacol Rev 1980; 32:229 - 313
- Toh-e A, Oguchi T. Isolation and characterization of the yeast las21 mutants, which are sensitive to a local anestheticum, tetracaine. Genes Genet Syst 1998; 73:365 - 375
- Araki T, Uesono Y, Oguchi T, Toh-e A. LAS24/KOG1, a component of the TOR complex 1 (TORC1), is needed for resistance to local anesthetic tetracaine and normal distribution of actin cytoskeleton in yeast. Genes Genet Syst 2005; 80:325 - 343
- Eilam Y. Membrane effects of phenothiazines in yeasts I. Stimulation of calcium and potassium fluxes. Biochim Biophys Acta 1983; 733:242 - 248
- Malheiros SV, Meirelles NC, de Paula E. Pathways involved in trifluoperazine-, dibucaine- and praziquantel-induced hemolysis. Biophys Chem 2000; 83:89 - 100
- Nau C, Wang GK. Interactions of local anesthetics with voltage-gated Na+ channels. J Membr Biol 2004; 201:1 - 8
- Mozrzymas JW, Barberis A, Michalak K, Cherubini E. Chlorpromazine inhibits miniature GABAergic currents by reducing the binding and by increasing the unbinding rate of GABAA receptors. J Neurosci 1999; 19:2474 - 2488
- Lee IS, Park TJ, Suh BC, Kim YS, Rhee IJ, Kim KT. Chlorpromazine-induced inhibition of catecholamine secretion by a differential blockade of nicotinic receptors and L-type Ca2+ channels in rat pheochromocytoma cells. Biochem Pharmacol 1999; 58:1017 - 1024
- Snyder SH, Banerjee SP, Yamamura HI, Greenburg D. Drugs, neurotransmitters and schizophrenia. Science 1974; 184:1243 - 1253
- Kitagawa N, Oda M, Totoki T. Possible mechanism of irreversible nerve injury caused by local anesthetics: detergent properties of local anesthetics and membrane disruption. Anesthesiology 2004; 100:962 - 967
- Deuticke B. Transformation and restoration of biconcave shape of human erythrocytes induced by amphiphilic agents and changes of ionic environment. Biochim Biophys Acta 1968; 163:494 - 500
- Sheetz MP, Singer SJ. Biological membranes as bilayer couples: a molecular mechanism of drug-erythrocyte interactions. Proc Natl Acad Sci USA 1974; 71:4457 - 4461
- Kumar RV, Panniers R, Wolfman A, Henshaw EC. Inhibition of protein synthesis by antagonists of calmodulin in Ehrlich ascites tumor cells. Eur J Biochem 1991; 195:313 - 339
- De Filippi L, Fournier M, Cameroni E, Linder P, De Virgilio C, Foti M, et al. Membrane stress is coupled to a rapid translational control of gene expression in chlorpromazine-treated cells. Curr Genet 2007; 52:171 - 185