Abstract
From a structural standpoint, living organisms are organized like a nest of Russian matryoshka dolls, in which structures are buried within one another. From a temporal point of view, this type of organisation is the result of a history comprised of a set of time backcloths which have accompanied the passage of living matter from its origins up to the present day. The aim of the present paper is to indicate a possible course of this passage through time and suggest how today’s complexity has been reached by living organisms. This investigation will employ three conceptual tools, namely the Mosaic, Self-Similarity Logic, and the Biological Attraction principles. Self-Similarity Logic indicates the self-consistency by which elements of a living system interact, irrespective of the spatio-temporal level under consideration. The term Mosaic indicates how, from the same set of elements assembled according to different patterns, it is possible to arrive at completely different constructions: hence, each system becomes endowed with different emergent properties. The Biological Attraction principle states that there is an inherent drive for association and merging of compatible elements at all levels of biological complexity. By analogy with the gravitation law in physics, biological attraction is based on the evidence that each living organism creates attractive ‘field’ around itself. This field acts as a sphere of influence that is actively attracting similar fields of other biological systems, thereby modifying salient features of interacting organisms. Three specific organizational levels of living matter, namely the molecular, cellular, and supracellular levels, have been considered in order to analyse and illustrate the interpretative as well as the predictive roles of each of these three explanatory principles.
Very deep is the well of the past. Should we not call it bottomless?
Bottomless indeed, if — and perhaps only if — the past we mean is the past merely of the life of mankind, that riddling essence of which our own normally unsatisfied and quite abnormally wretched existences form a part; whose mystery, of course, includes our own and is the alpha and omega of all our questions, lending burning immediacy to all we say, and significance to all our striving. For the deeper we sound, the further down into the lower world of the past we probe and press, the more we do find that the earliest foundations of humanity, its history and culture, reveal themselves unfathomable. No matter to what hazardous lengths we let out our line they still withdraw again, and further, into the depths.
-Thomas Mann, Die Geschichten Jaakobs.Citation1 (English translation by H.T. Lowe-Porter)
Premise
It is not possible to probe the past of our species to its very depth, since, as described so eloquently by Thomas Mann in the quotation above, it appears to run towards a bottomless abyss. However, an attempt at this impossible task is a necessity if we are to penetrate the enigma of our ‘Dasein’, i.e., of our “being there” (as postulated by HeideggerCitation2). Some biologists have arbitrarily chosen that the bottom of the abyss corresponds with the origin of living matter, even if the terms ‘living’ and ‘life’ have not yet been adequately defined (for an old, but perceptive argumentation, see MitchellCitation3). This blurring of the term ‘life’ implies also that it is not possible to define the way to the bottom of the ‘Well of Being’ and, as discussed below, the same can be said when one considers ‘matter’, whose origin is equally mysterious an emanation from the ‘hyle’ at the interface between existence and non-existence ().
The aim of the present paper is to suggest a possible journey in the course of which we suggest how the complexity of present-day organisms has been arrived at. This investigation will employ two conceptual tools which have been proposed in previous papers, as well as a new tool which is introduced here in the present paper. These can be summarized as follows:
The ‘Self-Similarity Logic’ principle (Box 1). To clarify this principle, we take advantage of the definition of a dissipative system formed from several nested hierarchical levels. Auto-similar structures define each of these levels, and they lie buried within each other. Hence, they are structures of different degrees of scale or miniaturization.
The Mosaic principle. This principle can be illustrated by means of an analogy with the mosaic in art. The principle of mosaic will then be analysed especially at the macro-molecular level. However, it can also play a role at higher structural levels. Indeed, the concept may, at least in part, justify the existence of a gratuitous order in nature and might, therefore, play a role in so-called ‘evolutionary tinkering’.Citation4
The ‘Biological Attraction’ principle is a newly proposed principle (Box 1). It states that the assembly of basic biological units into a more complex system is navigated by an inherent drive for spontaneous attraction and merging of lowerorder complementary biological units/systems to generate higher organization level units/systems.
Self-Similarity Logic principle
As a new principle, ‘Self-Similarity Logic’ can be proposed. This is actually a new formulation of the old dictum of Carl von Linné : “Natura non facit saltus” (1751) and is paraphrased thus: “Naturae Logica non facit saltus”. This new principle means that the same rules for carrying out logical operations hold at any level of miniaturization: that is, within each of the ‘Russian Dolls’ which are nested within each other in the building up of a living organism.
Biological Attraction principle
Another new principle, that of ‘Biological Attraction’, is analogous to Newton’s Law of Gravitation in which objects with physical mass attract one another. Biological Attraction acts within and between biological systems. Biological activities, processes, or patterns are all deemed to be mutually attractive, and often may follow partial or full fusion of the attracted structures and/or systems. The biological ‘drive’ of attraction is inherent to living and evolving systems and is the results of their inherent biological activities. Attraction within the biological sphere operates irrespective of physical mass. It is proposed that this biological attractive force is intrinsic to living organisms and manifests itself through the propensity of any living organism to act, without necessarily any direct contact, on other living organisms. By analogy with the aforementioned gravitation law, biological attraction is based on the evidence that each living organism creates a sphere (or field) of influence around itself; i.e., it is capable of active modification of some of the salient features of the environment (niche) in which it lives. Living systems are, therefore, acting on other living organisms, which are sensitive to these features. Sensitivity to this biological attraction seems to increase in biological systems under stress. Depending on the context, the biological attraction may depend on the physical distance between interacting organisms, on the shapes, intensities and patterns of the created fields, on the corresponding features of the fields which are affected and, finally, on the sensitivities of the organisms present within that field. In biological evolution, this Biological Attraction manifests itself, inter alia, as co-evolution, convergent evolution, symbiogenesis, lateral gene transfer and manipulation at distance (such as attraction of pollinators by flowering plants). The Biological Attraction principle has both explanatory as well as predictive powers. For instance, it can explain the evolutionary origin of eukaryotic cells, multicellular organisms, and complex ecosystems. Also, it predicts a further tightening of bonds in our society, especially when exposed to stress situations. Biological Attraction explains all the above-mentioned horizontal aspects of biological evolution which are responsible for a network-like (reticulate) character of biological evolution, that has to be added to the vertical tree-like character of Darwinian evolution, as it is understood today.
From Definition of Dissipative System to Self-Similarity Logic and Mosaic Principles
Definition of nested dissipative system
Let us start by defining the term ‘nested system’, when applied to a living organism (see BungeCitation5 for example). According to Jacob,Citation6 from a structural standpoint, the living organism is organized as a complex of Russian Matryoshka Dolls. Hence, smaller structures are buried within larger ones (). From a functional standpoint, some nested systems have the extraordinary, if still obscure, quality of being ‘alive’. Thus, we arrive at the problem of having to define what we mean by the term ‘life’ or, less ambiguously, perhaps, ‘living system’. Certainly, a key characteristic of a living system is the one proposed by Prigogine,Citation7 who stated that a living individual is a dissipative system operating as a thermodynamically open system capable of maintaining a stable state far from equilibrium (see JantschCitation8); such a system can even evolve. In other words, a dissipative system absorbs energy from the environment and from it creates order within the system while passing disorder, or entropy, to the outside. This process has been summarized by Erwin Schroedinger who stated that a living organism acquires “neg-entropy”.Citation9
In the present paper, we suggest that it is possible to apply the model of a dissipative system not only, as indicated by Prigogine and Schroedinger,Citation7,Citation9 to the living individual but also, in a complex organism, to its main organs (), especially to the Central Nervous System (CNS).Citation10,Citation11 Actually, it is further suggested that organs absorb energy from the internal milieu to keep their entropy at a low level. The more they (tissues and organs) are organized, the more energy they consume to maintain their organization and perform their functions.
It has been surmised, also, that the other important conceptual proposal by Jacob, namely the nested structural organization of living organisms — animals and plants (and with regard to nested levels of organization in plants see in BarlowCitation12) — can be applied to the CNS.Citation13,Citation14 As a matter of fact, especially (but not only) for the CNS, it is possible to recognize several hierarchic levels, which are nested each within other ones.Citation13,Citation14 It has also been proposedCitation13 that some autosimilarity prevails at each nested level: the different elements interact, whatever their degree of miniaturization, according to similar rules (see the Self-Similarity Logic principle below).
Each level is characterized by its elements and by their interrelationships, not only at the level n under scrutiny but also at the other levels, n-1 and n+1, above and below (lesser and greater degrees of overall scale). It should also be noted that the notation n-1, n, and n+1 for the levels indicates a hierarchy. The nested set of dissipative system has, therefore, a depth due to the number of overlapping, or embedded, levels. Although this depth is in some respects the result of the power of our techniques to probe the system, it is useful at present to identify organizational levels such as organelles, cell, organ, organism, etc., since these seem to be common to very many kinds of living organism (fungi, plants, animals, etc.); and, in particular, to humans, and show similarities of structure and function.
Another aspect to be considered is the time dimension. All living beings contain the traces of a ‘history’ whose steps (evolutionary ‘events’) are the ‘time backcloths’ (geological eras) that have accompanied living matter in its passage towards the present day. As described in an extraordinary way by Thomas Mann in his masterpiece, ‘Tales of Jacob’,Citation1 great uncertainty prevails with respect to the time dimension. As a matter of fact, moving back along time’s arrow by means of the scientific investigation brought to bear on each backcloth, one reaches a more ancient backcloth located in the far-away past; and the process has, apparently, no end.
The Self-Similarity Logic principle has been introduced as as conceptual tool to investigate aspects of the above-mentioned spatio-temporal relationships, which occur inside the hierarchical levels, as well as within each time backcloth. This logic is based on the assumption: Natura non facit saltus. This assumption implies some uniformity in the logic according to which the elements of a living system interact in time and space. And in this respect, a fundamental principle of evolution at each level seems to be the mosaic assemblage of its components, i.e. the remodeling of the available materials to obtain new specializations of old structures, or the stabilization of formerly transient complexes to provide new functional operations. Let us consider, for the sake of clarity, the spatial dimension of the two conceptual tools, namely the Self-Similarity Logic (Box 1) and the Mosaic.
Self-Similarity Logic as a conceptual tool to investigate the spatial levels of a ‘nested dissipative system’.
The relevance of the term Self-Similarity Logic can be grasped by the fact that it conveys both the concept of miniaturization as well as multi-level organization of circuits. As a matter of fact, we have introduced the term Self-Similarity Logic to describe networks of networks (i.e., miniaturization) in the CNS (see ) where, at the various levels of the nested system, the same rules (logic) apply to each network’s characteristic operation.Citation13,Citation14
This view of a Self-Similarity Logic principle is in agreement with the statement of Russell and AloyCitation15 that a key concept in biological system design is modularity: nature duplicates and reuses existing parts and design principles again and again. This feature is apparent at nearly all levels, from the four nucleotide bases making up the genetic code to the hierarchical organization of ecosystems.Citation15 A well known example is the feed-back circuit found in metabolic networks where the amount of the final product inhibits its further production by acting directly upon a certain rate-limiting step. Feedback is also found in neuronal circuits, e.g., in the circuit which maintains muscle length, as well as in arterial blood pressure control.Citation16
On the basis of the Self-Similarity Logic principle we have proposed that (see Agnati et al.Citation13,Citation14) the way in which information is handled at one of the nested levels predicts, and sheds light on, the way in which similar operations are carried out at other levels. In the case of the CNS, the best investigated level is the neural network level; hence modes of information handling at this level could clarify aspects of information handling at, e.g., the molecular level. Information-handling by neural networks has a long story behind it. In this regard, it is enough to cite the classical article by McCulloch and PittsCitation17 and the published papers of the first year (1975–1976) of the Center for Systems Neuroscience.Citation18 Recently, an application of organization models present in neural networks, such as the ‘fringe’, has been applied at the molecular level.Citation14
Mosaic principle as conceptual tool to investigate the spatial levels of a ‘nested dissipative system’.
The concept of mosaic can be easily grasped from the mosaic as it is defined in figurative art: as a result of the process of making pictures or designs by inlaying small bits of coloured stones (tesserae) (). The term indicates that, in the assemblage, the elements are in physical contact with each other according to a structural plan (‘Bauplan’). The resulting pattern, moreover, fulfils an aim — for example, to represent an image envisaged by an artist or creator (, the left panel). Each single tessera, or a group of them, is endowed with emergent properties which reveal themselves by investigation of the completed mosaic. Exactly this, as well as the ‘energy’ required to make constructions of different cellular mosaic patterns, was explored for the case of plant meristems.Citation19 Thus, the term mosaic has the advantage of indicating how, from a set of elements, it is possible to achieve completely different patterns according to different artistic intentions represented by different Bauplanen. Similar sets of elements are, hence, endowed with different emergent properties according to the rules for their interaction (, the right panel). Two important implications should be mentioned:
Shapes of tesserae impose limits to their assemblage with other tesserae, since spatial matching has to be respected
This has an effect on the auto-assemblage of tesserae and it may give a hint for understanding the gratuitous order and, importantly, the spontaneous tendency of living matter towards order. It is becoming clearer and clearer that self-organization is widespread in nature and observed on all scales leading to a gratuitous order.Citation20–Citation22 As will be discussed below, the self-organization considered in the present paper is not only that of spontaneous assembly, which can occur at the molecular level (e.g., the capability of proteins to assembly in macromolecular complexes (protein mosaics) with highly different functions), even if formed by the same set of monomers,Citation23,Citation24 but also at the cellular level. In the latter case, an assemblage of prokaryotes within a bounded space has led to the appearance of eukaryotes.Citation25,Citation26 It occurred not necessarily by direct contact between free-living prokaryotes where different cells became different organelles on account of their position within the whole (for an analogy here, see the cellular rafts or globes of eukaryotic coenobia such as VolvoxCitation27) but rather it was their contiguity, as types of biological tesserae, due to their sharing of the same environment, such as the host cytoplasm.Citation28 This sharing of a common environment perhaps provided an extra tier of information for the working out of the Bauplan of the biological tesserae. In the case of the eukaryotic cell, it was one of the tesserae (host pre-karyote) which provided a boundary and which also provided a home within which other tesserae (guest prokaryotes) could reside and work out their respective fates as organelles.
Spatial location (topology) of the tesserae creates a pattern, which may be of importance in defining emergent properties of a set of elements
The term mosaic will be used to describe not only the physical contact and assemblage of elements, but also (as mentioned above) at a later more developed conceptual step, the assemblage achieved when the building blocks are in a such strict contiguity (substantial overlap of the respective spheres of influence) that they have become functionally interrelated, as is the case of cellular organelles within the cytoplasm.
According to the Self-Similarity Logic principle, it is also possible to give a further meaning to the proposal of Jacob concerning ‘evolutionary tinkering’.Citation4 Jacob proposed that evolution acts not as an engineer but as a tinker: evolution assembles new entities better fitted to the overall landscape (‘adaptation’) by putting together in new ways materials that have already been usefully developed. This way of proceeding by tinkering is present from the molecular level up to the level of complex organisms, and even to the level of ecosystems. Jacob describes this phenomenon as follows. Evolution tinkers together contraptions: it is natural selection combined with biological attraction (see below) that gives direction to changes, orients chance, and slowly and progressively produces more complex structures, new organs, and new species.Citation4 Novelties come from previously unseen associations of old material. Thus, evolution tinkers together new contraptions from the parts of older ones (for plant body evolution see ref. Citation29).
The mosaic principle could hold all the way from molecular to ecological systems. As far as the human brain is concerned, this way of thinking could be applied to the evolutionary process that has led to complex organization as well as to the moment-to-moment recruiting of different brain areas to organize a computational system capable of fulfilling an impending task. This point of view is an extension of Sherrington’s concept of ‘integration’Citation30 by which he meant the interaction of neural networks for a definite purpose. The functional interactions among neural networks, however, could be different from those moment-to-moment interactions needed to carry out specific tasks. It is interesting to notice that this view, although proposed recently by Damasio,Citation31 was also proposed, but in a different context, by Camillo GolgiCitation32 at the beginning of the last century.
“I (i.e., Golgi) have the strong belief that neurons operate (more or less) as larger groups of cells; sometimes entire ‘provinces’ of the brain are recruited and the output of these neuronal assemblies is integrated by their diffuse networks”.
Thus, the collective actions of neurons control the functions of the peripheral organs. Let us analyse more in detail how these two conceptual tools allow the probing of the Mosaic and the Self-Similarity Logic of living matter of past eras.
Interpretative Roles of Mosaic and Self-Similarity Logic Principles at Molecular Levels
On interpretative role of Mosaic principle
In the 1950s, Linus Pauling,Citation33 whose views were at variance with the accepted notions concerning the chemical structure of proteins, proposed that proteins have a well defined three-dimensional (3D) structure; and if two protein structures are compatible, then a multimeric complex can be assembled. This innovative view has been recently updated by stating that some domains or even, in some instances, the entire protein can be ‘unstructured’.Citation34
The presence of unstructured domains enhances protein flexibility and malleability. Protein flexibility depends not only upon the ‘disorder’ of the domains but also upon the characteristics of the amino acids forming these domains. Disorder enables the unstructured domains to serve as linkers which connect large modular domains in multimeric proteins (). The linkers are rich in polar uncharged amino acids which bias the sequence towards an extended flexible conformation. It is interesting to note that linkers of significant length are mainly found in eukaryotic proteins.Citation35 In the last decade, our view on protein-ligand interactions, hence also on protein-protein interactions, has been profoundly changed, and our group has proposed a general scheme for protein-protein interactions.Citation23,Citation24 It has been surmised that at least three main mechanisms for monomer assemblage (tesserae of the mosaic) within a multimeric protein complex can play a role ():
Lock-and-Key interactionsCitation36: Assembly concerns proteins (tesserae) with complementary 3D-structures. The proteins approach each other in the proper spatial fashion. This is typically a process involving globular proteins and, hence, it occurs between two “rigid” tesserae.
The induced-fit interactionsCitation37: Assembly involves interaction of a plastic protein (typically an Intrinsically Disordered Protein (IDP) hence a “malleable” tessera) with a 3D-structured protein (hence a “rigid” tessera) which acts as a structural template. Obviously, even if not reported in the scheme, a reciprocal induced-fit interaction can take place between two malleable proteins.
The glue-mediated interactionsCitation38: Assembly involves two non-complementary 3D-structured proteins (sterically incompatible rigid tesserae) thanks to the interposition of an IDP (malleable tessera), which acts as a “glue” between the two “rigid” tesserae, which otherwise could not fit with each other.
It should be noted that all the abovementioned mechanisms are based on the concept of mosaic. The difference between them resides in the malleability of the partners (tesserae) of the mosaic:
In the first case, the two partners have rigid, but complementary, 3D structures.
In the second case, one partner has a rigid 3D structure, but the other has a malleable 3D structure which can take a suitable conformation to match the rigid structure.
In the third case, the two partners have rigid and non-complementary 3D structures, but a malleable third partner is interposed which “glues” the other two together.
On interpretative roles of Self-Similarity Logic principle.
On the basis of the Self-Similarity Logic principle, it is possible to apply circuitry schemes not only at the level of neuronal networks but also at the level of molecular networks.Citation14
Let us examine two of these circuitry schemes: a circuitry scheme based on ‘lateral inhibition’: a filtering and sharpening action () can be obtained by means of a molecular circuit organization reminiscent of the “lateral inhibition” in neuronal circuits.Citation39 Actually, lateral inhibition has been extensively studied in neuronal circuits where it has been shown that, at every level of the brain, there are special mechanisms of lateral inhibition whose specific function is to exaggerate differences of activity between neighbouring neurons and thus to enhance or amplify any patterns that may exist.Citation39 As illustrated in a previous article,Citation14 the classical representation of lateral inhibition for neural circuits can be (, upper panel) transferred to molecular networks, in particular to the Receptor Mosaic (RM) formed by A2A and D2 receptors (lower panel). Thus, beside “physical compartmentalisation” of molecular networks that allows a very efficient spatial segregation of signalling pathways, a functional segregation, via lateral inhibition, can also produce a similar effect.Citation14
The fact that a similar organization of circuits can be recognized for lateral inhibition both at the neural network level and at the molecular network level, again underlines the potential heuristic value of Self-Similarity Logic.Citation14
The scheme of “fringe” for molecular networks:
Sherrington’s studies allowed the assessment of some basic criterions for the operations of neural circuits, in particular, the concept of “fringe” (). A fringe is an area of overlap between two networks and it leads to facilitation or occlusion. In this Figure, the facilitation in a neuronal circuit is shown (upper panel), and the same basic scheme is applied to mosaics formed by A2A receptors and D2 receptors. Thus, if a threshold release of Adenosine takes place at terminal 1 and 2, then only Adenylyl Cyclase 1 (AC 1) and Adenylyl Cyclase 2 (AC 2) are activated, since the amount of Adenosine transmitter (1a + 2a) is not sufficient to trigger the response of AC3. However, when both terminal 1 and terminal 2 are highly activated, the amount of Adenosine transmitter released can trigger the activation of AC 3. Hence, fringes at the molecular network can cause “facilitation” (higher overall response) and, in a similar way (not shown, but see the corresponding scheme of Agnati et al.Citation14), it can be shown that they can cause “occlusion”.
In conclusion, the concept of fringe can be introduced in the study molecular networks, just as other classical types of controls such as feed-back or feed-forward and spatial or temporal summation have been already used in molecular networks. The concept of fringe is of particular importance since it can suggest mechanisms which at the molecular network level, regulate cross-talk between pathways, and which can also play a role in allowing the production of complex cellular responses.Citation14
Interpretative roles of Mosaic and Self-Similarity Logic principles at the cellular level
On interpretative roles of the Mosaic principle.
It is intuitive to apply the concept of mosaic at the level of the single cell, and even at the multicellular organism level. As a matter of fact, in the latter case already the great encyclopedist Denis Diderot observed: Have you seen a swarm of bees that runs away from a beehive? Have you seen them cluster together at the end of a tree branch forming a big bunch, where all of them are attached to each other by means of their small legs? This bunch behaves as a single living being! As a matter of fact, if you disturb one of these bees the entire bunch will react by changing shapes and with an intense noise.Citation40 It is a well accepted notion that any complex living being is a ‘bunch of cells’ which are interconnected in such a way that they operate as a functional whole. Furthermore, it is now also clear that any eukaryotic cell is a ‘bunch of prokaryotes’ which share the same environment (the cytosol) and are interconnected in such a way as to operate as a functional whole. It should be noted that most multi-cellular organisms live in close association with microbes (bacteria) that either inhabit certain tissues of their hosts, or live externally on their surfaces in a close physiological relationship. Examples include bacteria that live on the skin or within the digestive tracts of animals. The term symbiosis refers to a mutually beneficial relationship that involves two or more biological partners. It is important to underline that formerly beneficial relationships may evolve into pathological ones. The term “endosymbiosis” refers to cases where one symbiont lives harmoniously within the cytoplasm of its unicellular or multicellular partner. It is now a well accepted theory that endosymbiosis has played a fundamental role in the evolution of complex organisms.
The ‘Serial Endosymbiotic Theory’ proposed by Lynn MargulisCitation25 maintains that, at certain points in time known as endosymbiotic events, one prokaryotic cell engulfed another. In each case, the result was a new kind of cell having a new kind of internal support system. Each endosymbiotic event would have been revealed as a major evolutionary step. An engulfed body could, for example, have been a bunch of the bacteria-like organisms and, after this engulfment, the latter could have developed to become the contemporary symbiotic mitochondria which absorb cellular oxygen and synthesise products that are used by the host cell. For the engulfed cell, this transition from being an autonomous proto-bacterium to becoming a host- (nuclear-) controlled organelle would have been pivotal in the evolution of the eukaryotic cell.Citation25,Citation26
In conclusion, the merging of formerly free-living unicellular organisms to recombine as more complex cells is in agreement with our proposal of the assembling of tesserae to create complexity and novelty. It should be remarked that the assemblage of prokaryotes (as tesserae) in the buildingup of the eukaryotic cell, and making use of the restricted cytosolic environment of the host, can confer not only an advantage for the continuity of reproduction of these proto-organelles but also for their contiguity in relation to their overall function.
On interpretative roles of Self-Similarity Logic principle.
It is not so easy to find clear-cut examples of the Self-Similarity Logic principle at the cellular level. However, let us use Jacob’s proposalCitation4 of evolution as a ‘tinker’ who, with the help of the ‘sieve of the natural selection’, creates cells with the most efficient combinations of tesserae. It can be surmised also that at the cellular level (as well as for molecular modules at the sub cellular level) there is a ‘sieving’ of the results of ‘trial-and-error’ events which involved the tinkering together of previously free-living organisms. Thus, a selective sieve permits optimal associations of endosymbiotic organelles which fit together to form a functional mosaic; and this mosaic, provided that it is also endowed with negentropy, is accordingly also endowed with emergent properties. This view is in agreement with our view that nature reuses both design principles (i.e., uses a Self-Similarity Logic) as well as the same building elements to assemble and re-assemble different contraptions. Thus, present-day eukaryotic cells are a hierarchical mosaic of cooperating specialized units and dissipative processes. In a previous paper,Citation41 on the basis of the obvious deduction that a hierarchical order of modules within a cell is a “must” for the global functioning of the system, it has been proposed that sets of ‘maternal mitochondria’ are the master entities of each eukaryotic cell. We reached such a conclusion on the basis of the following criteria relating to mitochondria:
Their unbroken linear descent through successive cell generations.
Their control of energy production.
Possibility of their by-passing, and therefore of surviving, the consequences of cell death.
Their regulation of cell death, life being the default position.
All these data and speculations lead to the proposal of the maternal mitochondria as the ‘sun’ around which all the other organelles of the eukaryotic cell revolve.Citation41
Against this background, a proposal for the interpretation of an old ‘idea’, namely that of the ‘eternal feminine’, has been put forward. It was introduced as the Ewig-Weibliche Theory (L. Agnati, unpublished lecture) according to Goethe’s verses of the ‘Chorus Mysticus’ with which he closes his masterpiece ‘Faust’.
All that is transitory is but a likeness; the unfulfilled here is attained; the inexpressible here is accomplished; the eternal feminine still leads us on.
-Goethe JW. Faust; 12110-11
As stated in the Premise, it is not possible to probe the past of our species to its depth, since it appears as a bottomless abyss. However, an attempt at this impossible task is a ‘must’, since the task would also reveal the solution to the enigma of our ‘Dasein’. Great poets—even stretching back to the beginnings of literature with the ‘Epic of Gilgamesh’—have proposed this task in some of their most famous masterpieces, even if only by making use of powerful metaphors; and the plastic arts too, have given us images which bring us some hints that bear on this problem. In literature we especially recall the masterpieces of Dante and Goethe, both of whom invoked the emblematic image of the feminine principle. These are now patrimonies of our culture and reflect one of the deepest feelings of our species. Thousands of years ago, the latter led to the religion of the Great Mother.Citation42
It can be speculated that our proposal of the maternal mitochondria as the ‘sun’ around which all the organelles of the eukaryotic cell revolveCitation41 is actually a cellbiological manifestation of this Feminine principle. As a matter of fact, it should also be mentioned that maternal mitochondria, because of their role in controlling nDNA, affect cognition.Citation43 Furthermore, it has been shown that mtDNA, via interactions with nDNA, can affect complex patterns of behavior, e.g., reproductive behaviors, especially in females. Actually, it has been proposed that mitochondria could influence female responses to male manipulations.Citation44 More recently, a proposal was made that mutant mitochondria can completely unbalance the human organism affected with Alzheimer disease.Citation45
Thus, we find here the possible ‘red thread’ of our life and reach that same awareness which inspired both Dante and Goethe: of the role of the feminine permeating the life and fate of our species. In fact, it has been observed that in the poems of both authors there is a pilgrimage towards a superior comprehension of our life which strives to contact an ideal world, possibly the one envisaged by Plato in which resides his conjectured Eternal Forms.
Goethe describes the ascension from the love of Margarete full of devotion and capability of self-sacrifice, to Helene’s love capable of spreading beauty and sensual joy, and finally to absolute love. By means of this trinity, of Eros, Agape and Philia, human beings and humanity make contact with a supernal world above the senses.
Dante describes Beatrice — that is the God Sapience; and when she uncovers her face and the light of the Truth illuminates Dante, he acquires a superior awareness.
“Turn, Beatrice, O turn thy holy eyes,” Such was their song, “unto thy faithful one, Who has to see thee ta’en so many steps. In grace do us the grace that thou unveil Thy face to him, so that he may discern The second beauty which thou dost conceal.”
-Dante Alighieri. Purgatorio Canto XXXI; vv. 133–38 (English translation by H. Wadsworth Longfellow)
Biological Attraction Principle: An Explanatory Proposal for Both Mosaic and Self-Similarity Logic Principles with a Powerful Predictive Potential
Something else is suggested by the Mosaic and Self-Similarity Logic principles. It is the question of the driving force which pushes unanimated matter towards life and then drives living systems towards ever higher complexity. This aspect has been recognized as an inherent ‘gratuitous order’Citation20 within matter. By itself, however, order, even when coupled with self-replication, is not sufficient to explain the emergence of properties having a survival value. Thus, the idea of ‘gratuitous order’ may be reformulated in a wider way as a Biological Attraction principle which drives the assemblage of units towards a higher level of complexity; and out of this complexification new, self-sufficient, or autonomous, higher levels of organization emerge. Higher degrees of inherent order are products of the complementarities possessed by lower-order, simpler or smaller scale units/systems; the latter are the units which associate/merge—show biological attraction—with other similar units/systems. In other words, the Biological Attraction principle states that, at each given level of organization, each biological units/systems acts as an attractor for other units/systems. If structurally and functionally compatible and complementary, these assemble into new, higher-order assemblages.Citation24 The pattern and order of these new and more complex assemblages are dictated by the pattern and order of the lower-order units/systems. Ultimately, the assemblage culminates in a system endowed with emergent properties of new higher-level complexity, endowed with high survival value, and having high efficiency for the exploration and exploitation of adjacent environmental spaces. The behavior of each of these new biological units/systems is, moreover, decided by the powerful sieve of natural selection that presides over the emergence of complex organisms and, in general, regulates a suitable cost/benefit ratios for the whole system and it component parts. Modular construction and nested levels of organization, by means of the various modes of internal communication, may supply these benefits.
In this sense, the Biological Attraction principle is a new concept for biology with a powerful explanatory and predictive potential. It encompasses the inherent drive of biological units, at all levels of biological complexity, to associate into higher-order assemblages. Summing up, as specified above, such a drive is not blind. On the contrary, it is navigated by lower-level attractors as well as oriented by an attractor located at a higher complexity level. Several examples of convergent evolutionCitation46–Citation49 indirectly implicate such higher-order attractors in evolution. Thus, more and more complex assemblages occur. On the Earth, they have culminated in Gaia.Citation50,Citation51 It might also be surmised that such a view can also have an interpretative value for, at least some fundamental aspects of human society. The Biological Attraction principle explains why the priciples of the Mosaic and Self-Similarity Logic are valid for all biological units and systems (Box 1). Biological Attraction can be considered as the biological variant of the attractional force in Physics. It gives us a prospect to unify several concepts in Biology and Physics (Box 1). It could, therefore, serve as a powerful frame of reference for future research. In fact, recent discoveries have revealed that galaxies also obviously evolve; they seem to be shaped by their surroundings, and form through hierarchical process such as the merging of smaller galaxies to form bigger one,Citation52–Citation54 as well as by possible reproductive fission events. Self-organization is not just a biological phenomenon but, in its dealing with the nature of space and time, it has validity also for fundamental physics.Citation8,Citation52
There are several examples of the inherent attractive, or associative, drive of biological units/systems at the sub-cellular, cellular, and supra-cellular levels. At the sub-cellular level, we can mention association of genes into genomes, proteins into higher proteinaceous complexes, binding of ligands with their receptors, polymerization of cytoskeletal elements such as microtubules and actin filaments from monomeric subunits using multiple accessory proteins, assembly of cellular superstructures such as ribosomes, proteasomes, signalosomes, spliceosomes, and cytoskeletal motors binding their cargoes to the cytoskeletal tracks in the performance of vectorial work. The Biological Attraction principle explains the evolutionary drive to transfer DNA from cytoplasmic organelles into the nuclear DNA. At the cellular level, this principle has the power to explain why the historical unicellular organisms became merged, and by so doing led to the formation of contemporary eukaryotic cells; and even why multicellularity was invented numerous times independently in diverse organisms.Citation27,Citation55 At the supracellular level, the Biological Attraction principle explains why complex multi cellular organisms show a strong tendency to, and drive for, cooperation and association, forming social communities. This principle also predicts that this drive is continuing at present, and will continue to do so in the future, as evidenced by recent developments in human societies which accomplish the apparent dramatic tightening of social bonds and communication via internet and mobile phones. In plant ecology, new data indicate that plants live in social communities, relying for their extensive interactions on communication systems involving, inter alia, diverse volatiles.Citation56–Citation58 Moreover, many species of plants merge with mycorrhizal fungi which connect individual plants into highly integrated fungal-plant superorganism.Citation59,Citation60 Grafting of roots into a supra-organismic root system for maximal survivalCitation61 is another example of the Biological Attraction principle. The significance of these consortia is still not fully understood but might have a predictive power for human civilization, too. It might be proposed, for example, that when communication by these means reaches a critical threshold then a phase-change will be accomplished, in part by means of new and diverse technological artefacts, and will lead to a new quality of the assemblages that compose both Self and SocietyCitation62 at their respective high levels of biological complexity and organization.Citation62
Final Comments
The three biological principles, which we have outlined here, are closely interlinked. They obviously reflect different aspects of the same biological phenomena. They also have the power to explain perplexing features of biological systems such as their inherent capabilities for self-assembly and self-organization. In evolution, besides the ‘supra-cellular’ nature of the eukaryotic cells,Citation25,Citation26 there are several examples of the merging of genomes into a supra-genome and the subsequent individualization of an allopolyploid organism. Aggregations with subsequent individualization, so-called Beklemishev cycles, as well as chromosomal and gene duplications (see OhnoCitation63), were proposed also for the ancient rise of animals.Citation64,Citation65 Furthermore, there are examples of unities between pairs of otherwise independent organisms. We can mention diatoms as mosaic organisms which have completed their merging,Citation66–Citation68 and corals and lichens as examples of unified organisms which are in the process of merging.Citation69–Citation71 Corals represent a symbiotic cnidarian-dinoflagellate partnership, while lichens are evidence of another type of ‘attractional’ association — here between fungus and alga/cyanobacteria to form composite plant-like superorganisms.Citation69 These latter organisms are able to survive extreme conditions, even that harshest of environments afforded by interplanetary SpaceCitation70 through which organisms may, from time to time, travel. Organismal associations are often preceded by parasitism and infection.Citation71–Citation73 By means of these infective processes, RNAi was evolved as defence against viruses and transposable elements,Citation72,Citation73 providing biological evolution with yet another tool for generating higher complexity.Citation74 Even evolution of sex can be explained via parasitic infection and merging of proto-eukaryotic hosts and symbionts.Citation75 The examples given also reveal the robustness of composite organisms generated according to the principles which we have discussed: the Mosaic, Self-Similarity Logic and the Biological Attraction principles. Moreover, the Biological Attraction principle explains all the above-mentioned horizontal aspects of the biological evolution which are responsible for the network-like (reticulate) character of biological evolution (Box 1) and which has to be added to the tree-like character of Darwinian evolution, as it is basically accepted today.Citation76–Citation78
Finally, it is essential to keep in mind our subjective perspective which gives us access only to an incomplete understanding of reality.Citation79–Citation83 All our theories, concepts, and principles, as derived from our subjective senses,Citation79–Citation85 and are limited by constraints of our embodied cognition. Citation79,Citation84–Citation88 This embodied cognition is heavily loaded with an anthropocentrism and other cognitive traps due to the subjectivity of the observer perspective.Citation88
Note
This article is dedicated to Gianpiero Turrin, great friend and man of great culture.
Abbreviations
AC | = | Adenylyl Cyclase |
Gi | = | heterotrimeric G-protein of the Gi family |
Gs | = | heterotrimeric G-protein of the Gs family |
IDP | = | Intrinsically Disordered Protein |
Figures and Tables
Figure 3 Schematic representation of miniaturization and hierarchic criteria. The four main miniaturization levels, i.e., the macro-, meso-, micro- and nano-scales are illustrated. Furthermore, mechanisms for the plastic adjustment of the 3D-conformations of proteins are outlined in view of their functional importance since as stated by JacobCitation6: “the overall coordination of the cellular function is founded on the geometrical deformation of proteins”.Citation6
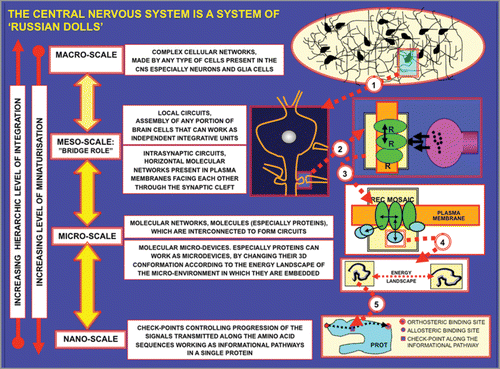
Figure 4 Schematic representation of the ‘Mosaic Principle’. The left panel shows the mosaic in Ravenna of Empress Teodora made up by small cubes of coloured marble (tesserae). As the right panel shows, from the same set of protein monomers (tesserae) it is possible to assemble completely different multimeric proteins.
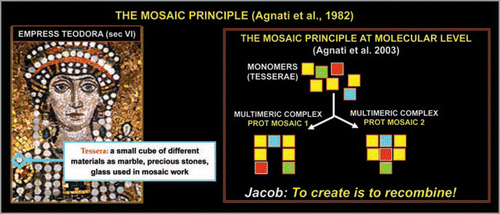
Figure 6 Schematic representation of the possible basic mechanisms involved in the assemblage of multimeric proteins.
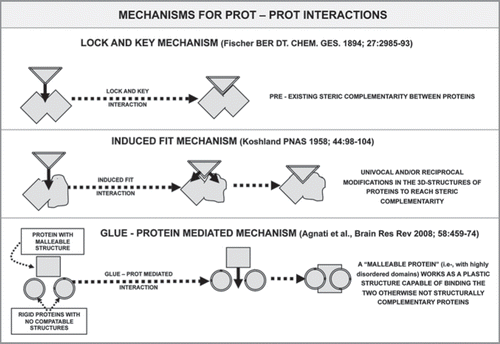
Figure 7 Schematic representation of the model of lateral inhibition transferred according to the ‘Self-Similarity Logic’ principle: from neural networks to molecular networks. Upper panel: Lateral inhibition leads to focusing of activity in a few computational neurons in neural circuits.Citation39 Lower panel: The scheme illustrates the possible application of this basic model of circuit organization to a complex formed by a A2A^D2 heterodimers and A2A homodimers. Activation of A2A receptors by Adenosine leads to a reduction in the affinity of D2 receptors in the heterodimers, hence in a sharpening of the signalling via the A2A homodimers. For further details, see text.
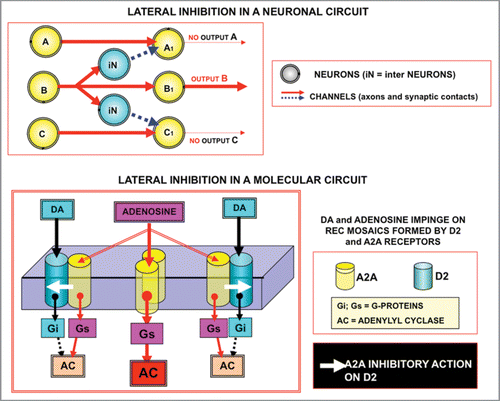
Figure 8 Schematic representation of the concept of fringe. Two input neurons, A and B, directly acting on two output neurons (A1 and B1) are considered. It is surmised that A, but not B (upper left panel), gives an above-threshold input to its target neuron A1 (upper left panel). However, if neuron A fires at a high frequency it can lower the threshold for the firing of B1. The term ‘mosaic’ will be used to describe not only the physical contact and assemblage of elements, but also (as mentioned above) at a later more developed organimal complexity when individual building blocks become functionally inter-related and inter-dependent, as is the case of cellular organelles within the cytoplasm. Thus, the subthreshold input of B to B1 is now sufficient to cause the firing of neuron B1, hence two output neurons fire (upper right panel). Thus, these panels show “facilitation” of A on the firing of neuron B1, and indicate that B1 is localised in the subliminal fringe of A. The same basic scheme is applied (lower panel) to receptor mosaics formed by A2A and D2 receptors. A low concentration of Adenosine can activate only AC1 and AC2, however high concentrations of Adenosine can diffuse until the trimer A2A^D2^A2A inhibiting, via receptor-receptor interactions, the activation by D2 of the Gi. Thus, it can be observed that the activation is not simply of AC1 and AC2, but also of AC3, which is no longer inhibited by the Gi
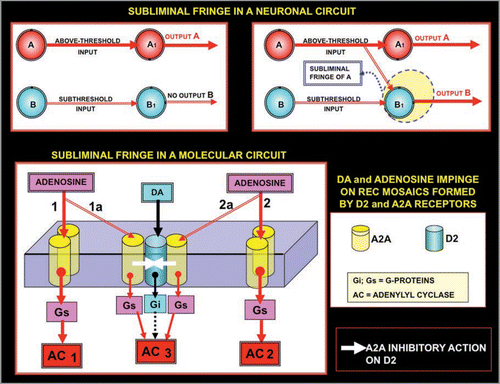
References
- Mann T. The Tales of Jacob 1933; (Die Geschichten Jaakobs)
- Heidegger M. Sein und Zeit 1927; Oxford Basil Blackwell (translated as Being and Time by John Macquarrie and Edward Robinson), 1978
- Mitchell PM. Materialism and Vitalism in Biology. The Herbert Spencer Lecture 1930; Oxford, Clarendon Press Delivered at Oxford, 3 June.
- Jacob F. Evolution and tinkering. Science 1977; 196:1161 - 1166
- Bunge M. Levels: a semantical preliminary. Rev Metaphys 1960; 13:396 - 406
- Jacob F. La logique du vivant. Une histoire de l’hérédité 1970; Paris Editions Gallimard
- Prigogine L. From Being to Becoming 1980; St. Francisco Freeman WH
- Jantsch E. The self-organizing Universe. Scientific and Human Implications of the Emerging Paradigm of Evolution 1980; Oxford Pergamon Press,
- Schroedinger E. What is Life?. The Physical Aspects of the Living Cells 1944; Cambridge Cambridge University Press
- Agnati LF, Fuxe K. New concepts on the structure of the neural networks: the miniaturization and hierarchical organization of the central nervous system. Biosci Rep 1984; 4:93 - 98
- Agnati LF, Guidolin D, Fuxe K. The brain as a system of nested but partially overlapping networks. Heuristic relevance of the model for brain physiology and pathology. J Neural Transm 2007; 114:3 - 19
- Barlow PW. Iqbal M. From cell to system: Reptetitive units of growth in the development of shoots and roots. Growth Patterns in Vascular Plants 1994; Portland, Oregon Dioscorides Press, 19 - 58
- Agnati LF, Santarossa L, Genedani S, Canela EI, Leo G, Franco R, et al. Erdi P, Esposito A, Marinaro M, Scarpetta S, et al. On the nested hierarchical organization of CNS: basic characteristics of neural molecular networks. Computational neuroscience: cortical dynamics, lecture notes in computer sciences 2004; Berlin, Heidelberg, New York Springer 24 - 54
- Agnati LF, Guidolin D, Carone C, Dam M, Genedani S, Fuxe K. Understanding neuronal molecular networks builds on cellular network architecture. Brain Res Rev 2008; 58:379 - 399
- Russell RB, Aloy P. Targeting and tinkering with interaction networks. Nat Chem Biol 2008; 4:666 - 673
- Guyton AC, Cowley AW. Cardiovascular Physiology II International Review of Physiology Vol 9 (Series Editor: Arthur C. Guyton) 1976; Baltimore University Park Press,
- McCulloch WS, Pitts WH. A logical calculus of the ideas immanent in neural nets. Bull Math Biophys 1943; 5:115 - 133
- Metzler J. Systems Neuroscience 1977; New York Academic Press,
- Barlow PW. Evolution of structural initials in apical meristems of plants. J Theor Biol 1994; 169:163 - 177
- Kauffman SA. The Origin of Order 1993; New York Oxford University Press
- Luisi PL. Contingency and determinism. Philos Transact A Math Phys Eng Sci 2003; 361:1141 - 1147
- Harold FM. Molecules into cells: specifying spatial architecture. Microbiol Mol Biol Rev 2005; 69:544 - 564
- Agnati LF, Franzen O, Ferré S, Leo G, Franco R, Fuxe K. Possible role of intramembrane receptorreceptor interactions in memory and learning via formation of long-lived heteromeric complexes: focus on motor learning in the basal ganglia. J Neural Transm Suppl 2003; 65:1 - 28
- Agnati LF, Guidolin D, Genedani S, Ferré S, Bigiani A, Woods AS, et al. How proteins come together in the plasma membrane and function in macromolecular assemblies: focus on receptor mosaics. J Mol Neurosci 2005; 26:133 - 154
- Margulis L. Serial endosymbiotic theory (SET) and composite individuality. Transition from bacterial to eukaryotic genomes. Microbiol Today 2004; 31:172 - 174
- Baluška F, Volkmann D, Barlow PW. Eukaryotic cells and their Cell Bodies: Cell Theory revisited. Ann Bot 2004; 94:9 - 32
- Kirk DL. Volvox. Molecular-genetic Origins of Multicelluarity and Cellular Differentiation 1998; Cambridge, Cambridge University Press
- Agnati LF, Fuxe K, Baluška F, Guidolin D. Implications of the ‘Energide’ concept for communication and information handling in the central nervous system. J Neural Trans 2009; 116:1037 - 1052
- Dolan L. Body building on land—morphological evolution of land plants. Curr Opin Plant Biol 2009; 12:4 - 8
- Sherrington C. The Integrative Action of the Nervous System 1906; New Haven Yale University Press
- Damasio A, Meyer K. Behind the looking-glass. Nature 2008; 454:167 - 168
- Golgi C. La moderna evoluzione delle dottrine e delle conoscenze sulla vita. Rendiconti Regio Istituto Lombardo 1914 Milano Vol. XLVII fasc. 1
- Pauling L. Protein interactions. Aggregation of globular proteins. Discus Faraday Soc 1953; 13:170 - 176
- Dunker AK, Brown CJ, Lawson JD, Iakoucheva LM, Obradovic Z. Intrinsic disorder and protein function. Biochemistry 2002; 41:6573 - 6582
- Dyson HJ, Wright PE. Intrinsically unstructured proteins and their functions. Nat Rev Mol Cell Biol 2005; 6:197 - 208
- Fisher E. Einfluss der Configuration auf die Wirkung der Enzyme. Berichte der deutschen chemischen Gesellschaft 1894; 27:2985 - 2995
- Koshland DE. Application of a theory of enzyme specificity to protein synthesis. Proc Natl Acad Sci USA 1958; 44:98 - 104
- Agnati LF, Leo G, Genedani S, Andreoli N, Marcellino D, Woods A, et al. Structural plasticity in G-protein coupled receptors as demonstrated by the allosteric actions of homocysteine and computerassisted analysis of disordered domains. Brain Res Rev 2008; 58:459 - 474
- Carpenter RHS. Neurophysiology 2003; IV edition London Arnold
- Diderot D. Rêve de d’Alembert (Essay composed in the 1769 but published only after Diderot’s death in the 1830)
- Agnati LF, Barlow PW, Baldelli E, Baluška F. Are maternal mitochondria the shellfish entities that are masters of the cells of eukaryotic multicellular organisms?. Commun Integr Biol 2009; 2:1 - 7
- Ward T. Savage Breast. One Man’s Search for the Goddess 2006; Winchester O Books,
- Roubertoux PL, Marcet B, Sluyter F, Verrier B. Mitochondrial DNA (mtDNA) and behavior: interaction between mitochondrial and nuclear genes, preliminary results from microarrays. Behav Genet 2003; 33:717
- Zeh DA, Zeh JW. Maternal inheritance, sexual conflict and the maladapted male. Trends Genet 2005; 21:281 - 286
- Agnati LF, Guidolin D, Baluška F, Fuxe K, Carone C, Genedani S. A new hypothesis on pathogenic mechanisms founded on the possible ‘liberation’ war of endo-symbiotic organelles. Curr Alzh Res 2009; In press
- Zakon HH. Convergent evolution on the molecular level. Brain Behav Evol 2002; 59:250 - 261
- Emery NJ. The mentality of crows: convergent evolution of intelligence in corvids and apes. Science 2004; 306:1903 - 1907
- Conway Morris S. Evolutionary convergence. Curr Biol 2006; 16:R826 - R827
- Baluška F, Mancuso S. Baluška F. Plants and animals: convergent evolution in action?. Plant-Environment Interactions: From Sensory Plant Biology to Active Plant Behavior 2009; 285 - 301
- Lovelock JE. Gaia: A New Look at Life on Earth 1979;
- Lovelock JE. The living Earth. Nature 2003; 426:769 - 770
- Smolin L. The self-organization of space and time. Phil Trans R Soc Lond A 2009; 361:1081 - 1088
- Smolin L. Cosmological natural selection as the explanation for the complexity of the universe. Physica A 2004; 340:705 - 713
- Cho A. The tales told by lonely galaxies. Science 2009; 324:1262 - 1263
- Rokas A. The molecular origins of multicellular transitions. Curr Opin Genet Dev 2008; 18:472 - 478
- Karban R. Plant behaviour and communication. Ecol Letts 2008; 11:727 - 739
- Dicke M. Chemical complexity of volatiles from plants induced by multiple attack. Nat Chem Biol 2009; 5:317 - 324
- Heil M. Baluška F. Airborne induction and priming of defenses. Plant-Environment Interactions: From Sensory Plant Biology to Active Plant Behavior 2009; 137 - 152
- Giovannetti M, Avio L, Fortuna P, Pellegrino E, Sbrana C, Strani P. At the root of the Wood Wide Web: self recognition and nonself incompatibility in mycorrhizal networks. Plant Signal Behav 2006; 1:1 - 5
- Seddas P, et al. Baluška F, et al. Communication and signaling in the plant-fungus symbiosis: the mycorrhiza. Plant-Environment Interactions: From Sensory Plant Biology to Active Plant Behavior 2009; 45 - 71
- Keeley JE. Population variation in root grafting and a hypothesis. Oikos 1988; 52:364 - 366
- Kováč L. Science, an essential part of culture. EMBO Rep 2006; 7:128 - 132
- Ohno S. Evolution by Gene Duplication 1970; Berlin Allen and Unwin, London/Springer-Verlag,
- Beklemishev WN. Principles of Comparative Anatomy of Invertebrates 1969; 1 Chicago University of Chicago Press,
- Dewel RA. Colonial origin for Eumetazoa: major morphological transitions and the origin of bilaterian complexity. J Morph 2000; 243:35 - 74
- Nisbet RE, Kilian O, McFadden GI. Diatom genomics: genetic acquisitions and mergers. Curr Biol 2004; 14:R1048 - R1050
- Bowler C, Allen AE, Badger JH, Grimwood J, Jabbari K, Kuo A, et al. The Phaeodactylum genome reveals the evolutionary history of diatom genomes. Nature 2008; 456:239 - 244
- De Martino A, Amato A, Bowler C. Mitosis in diatoms: rediscovering an old model for cell division. BioEssays 2009; 31:874 - 884
- Sanders WB. A feeling for the superorganism: expression of plant form in the lichen thallus. Bot J Linn Soc 2006; 150:89 - 99
- Sancho LG, et al. Lichens survive in Space: results from the 2005 LICHENS experiment. Astrobiology 2007; 7:443 - 454
- Baluška F. Cell-cell channels, viruses, and evolution: via infection, parasitism and symbiosis towards higher levels of biological complexity. Ann NY Acad Sci 2009; 1178:106 - 119
- Bagasra O, Prilliman KR. RNA interference: the molecular immune system. J Mol Histol 2004; 35:545 - 553
- Obbard DJ, Gordon KH, Buck AH, Jiggins FM. The evolution of RNAi as a defence against viruses and transposable elements. Philos Trans R Soc Lond B Biol Sci 2009; 364:99 - 115
- Heimberg AM, Sempere LF, Moy VN, Donoghue PCJ, Peterson KJ. MicroRNAs and the advent of vertebrate morphological complexity. Proc Natl Acad Sci USA 2008; 105:2946 - 2950
- Sterrer W. On the origin of sex as vaccination. J Theor Biol 2002; 216:387 - 396
- Doolittle WF, Bapteste E. Pattern pluralism and the Tree of Life hypothesis. Proc Natl Acad Sci USA 2008; 104:2043 - 2049
- Archibald JM. The eocyte hypothesis and the origin of eukaryotic cells. Proc Natl Acad Sci USA 2008; 105:20049 - 20050
- Ragan MA, McInerney JO, Lake JA. The network of life: genome beginnings and evolution. Phil Trans R Soc B 2009; 364:2169 - 2175
- Vrobel S. Fractal time, observer perspectives and levels of description in nature. Electr J Theor Phys 2007; 4:275 - 302
- Eagleman DM. Human time perception and its illusions. Curr Opin Neurobiol 2008; 18:131 - 136
- Piccolino M, Wade NJ. Galileo’s eye: a new vision of the senses in the work of Galileo Galilei. Perception 2008; 37:1312 - 1340
- Piccolino M, Wade NJ. Galileo Galilei’s vision of the senses. Trends Neurosci 2008; 31:585 - 590
- Wittmann M, van Wassenhove V. The experience of time: neural mechanisms and the interplay of emotion, cognition and embodiment. Phil Trans R Soc B 2009; 364:1809 - 1813
- Lyon P. The biogenic approach to cognition. Cogn Process 2005; 7:11 - 29
- Kováč L. Life, chemistry and cognition. EMBO Rep 2006; 7:562 - 566
- Kováč L. Information and knowledge in biology: time for reappraisal. Plant Signal Behav 2007; 2:65 - 73
- Kováč L. Bioenergetics: A key to brain and mind. Commun Integr Biol 2008; 1:114 - 122
- Pauling L, Zuckerkandl E. Rohlfing DL, Oparin AI. Chance in evolution — some philosophical remarks. Molecular Evolution: Prebiological and Biological 1972; New York Plenum Press, 113 - 126